Abstract
OFA hr/hr (OFA) rats present a major lactation deficit that impairs offspring survival. To explore whether abnormal stress responsiveness causes this deficit, we compared their hormonal (prolactin, progesterone, and corticosterone) responses to stress (room change and 2-min ether exposure) with those of Wistar and Sprague Dawley (SD) rats. We tested responses during the estrous cycle, pregnancy, lactation, after ovariectomy, and ovarian steroid hormone priming, and responses to suckling. We evaluated hypothalamic expression of receptors for prolactin (PRLRlong) and the isoforms of receptors for progesterone (PRA and B) and estrogen (ERα and β) in late pregnancy. We tested whether administration of an anxiolytic (diazepam) improved lactation. Ether exposure increased circulating levels of the three hormones in the three strains of rats, cycling and ovariectomized, but was less effective in pregnancy and lactation. Elevated estrogen level (estrus and estradiol-treated ovariectomized rats) potentiated the prolactin response more in SD and OFA rats than in Wistar rats. Elevated progesterone level (late pregnancy, lactation, progesterone-treated ovariectomized rats) inhibited the prolactin response less in OFA than in SD or Wistar rats. Ether exposure inhibited the prolactin and oxytocin responses to suckling only in OFA rats. Diazepam treatment increased pup survival rate and the prolactin response to suckling. Hypothalamic total PR mRNA content, assayed by RT-PCR, was higher in pregnant OFA rats compared with SD and Wistar rats, but the PRB/PRA protein ratio determined by Western blot was lowest in Wistar rats, intermediate in OFA rats, and highest in SD rats. The heightened sensitivity to stress of lactating OFA rats may contribute to their lactational deficit and be caused by a combination of hypoprolactinemia and reduced inhibitory capacity of progesterone.
Introduction
The OFA hr/hr (OFA) hairless strain of rats presents deficient lactation associated with an elevated activity of the dopamine system in the mediobasal hypothalamus (MBH, comprising the arcuate and periventricular nuclei and the median eminence) and overexpression of tyrosine hydroxylase (TH). Dopamine neurones in the MBH are the key inhibitory regulators of prolactin secretion, and consequently in OFA rats suckling-induced prolactin secretion, and also oxytocin release, is partially blocked when compared with Sprague Dawley (SD) rats, the strain of origin. However, this partial blockade may not be solely responsible for lactation failure, as once lactogenesis has been triggered, only moderate amounts of prolactin are sufficient to maintain adequate milk synthesis which seems to be normal in this strain (Valdez et al. Citation2007). The OFA strain bears a large intragenic deletion of the desmoglein-4 gene (Bazzi et al. Citation2004), which codes for a protein belonging to the desmoglein family, cell adhesion molecules related to cadherins. This strain does not present the severe impairment of the immune system present in the “nude” strains, as it does not show increased susceptibility to infectious disease.
Anxiety is a common cause of lactation failure in women (Weichert Citation1979; Lonstein Citation2007), most probably due to powerful inhibition of the nursing-induced secretion of oxytocin and prolactin. This effect of stress has been demonstrated in several rat strains (Wistar, Holzmann and SD) and in guinea pigs (Cross Citation1955; Chaudhury et al. Citation1961; Grosvenor and Mena Citation1967; Higuchi et al. Citation1989), and is mediated by cerebral cortex activation (Taleisnik and Deis Citation1964; Voloschin and Tramezzani Citation1984). However, the response to stress in terms of behavior and secretion of hypothalamic–pituitary–adrenal (HPA) axis hormones (adrenocorticotrophic hormone [ACTH], corticosterone) is attenuated in lactating rats, while the prolactin response is practically abolished (Higuchi et al. Citation1989; Banky et al. Citation1994; Windle et al. Citation1997). This phenomenon probably evolved as an adaptative mechanism promoting survival of the offspring, and is also seen in breast-feeding postpartum women (Altemus et al. Citation1995).
Stress, besides its recognized role as a potent activator of the HPA axis, is a powerful stimulus to prolactin secretion in rodents and other mammals (Gala Citation1990; Malarkey et al. Citation1991; Grasselli et al. Citation1992) and seems not to act solely by inhibition of dopaminergic neurons, since dopamine agonists do not suppress completely stress-induced prolactin release (Shin Citation1980; Jahn and Deis Citation1986).
Ovarian steroids modulate the prolactin response to stress (Poletini et al. Citation2006). Progesterone and its 5α-reduced metabolites are powerful inhibitors of the HPA axis stress response (Jahn and Deis Citation1986; Deis et al. Citation1989; Brunton et al. Citation2008). The central and peripheral levels of the progesterone metabolite, allopregnanolone, are elevated during pregnancy, and are responsible for the attenuation of the HPA axis response to stress through activation of opioid tone (Brunton et al. Citation2009). Progesterone may also act directly as this steroid modulates hypothalamic hypophysiotropic dopamine neuron activity (Arbogast and Voogt Citation2002). However, progesterone does not seem to influence ACTH significantly and only affects corticosterone slightly when administered to estradiol-primed rats (Figueiredo et al. Citation2007). Although estrogens reduce anxiety through a potentiation of the action of progestogens or their metabolites (Walf and Frye Citation2006), they seem to potentiate stress-induced prolactin release (Poletini et al. Citation2006). Estrogens also blunt the ACTH response to restraint stress, but the corticosterone response is enhanced (Figueiredo et al. Citation2007).
Thus, it is possible that the lactation failure of OFA rats may be caused, in part at least, by an abnormal sensitivity to stress which may be mediated through alterations in the ovarian steroid modulation of the stress response.
In order to test this hypothesis, we have investigated the sensitivity to stress of the OFA strain and its regulation by ovarian steroids. For this purpose, we studied the relative responses of OFA, SD and Wistar rats in terms of prolactin, progesterone, and corticosterone release to moderate stressors (moving to another room and brief exposure to ether). Wistar rats show normal lactation, like SD rats, but display several neuroendocrine differences from SD rats, such as greater susceptibility to estrogenic actions of mammary carcinogens (el Abed et al. Citation1987; Garcia-Segura et al. Citation1992), lower copulatory responses to sex steroid hormones (Emery and Larsson Citation1979), and reduced hypothalamic aromatase activity (Mathias et al. Citation1999). In order to determine whether any differences in stress responses are due to differential responses to ovarian hormones, we tested stress responses in different hormonal states (estrous cycle, pregnancy, lactation, and steroid hormone replacement in ovariectomized rats). We also determined the sensitivity of lactating OFA rats to stress by comparing the effect of stress on the milk ejection reflex in mid-lactation in the three strains of rats. We also determined whether administration of an anxiolytic (diazepam) improves lactational performance. Finally, to determine possible causes of the differential sensitivity to ovarian hormones observed between strains, we measured hypothalamic expression of prolactin (the long form, PRLRlong) and the two isoforms of the progesterone (PR A and B) and estrogen (ERα and β) receptors in late pregnancy, when the attenuation of the stress response may be maximal (Brunton et al. Citation2008).
Materials and methods
Animals
Adult female (200–230 g) and male (250–300 g) of 3–4-month-old Wistar, SD and OFA rats bred in our laboratory were used. Rats were kept in a light (lights on 06.00–20.00 h) and temperature (22–24°C) controlled room, six to eight per cage unless indicated otherwise. Rat chow (Cargill, Cordoba, Argentina) and tap water were available ad libitum. Vaginal smears were taken daily from the rats and the stage of the estrous cycle was determined by microscopic observation of the vaginal cytology on the fresh smears. Female rats showing regular 4-day cycles were used. The presence of spermatozoa in the vaginal smears, the morning after caging with a fertile male in the night of proestrus, was indicative of pregnancy and this day counted as day 0 of pregnancy. Two or 3 days before delivery, the rats were caged individually and kept in the individual cages with the litter until weaning or euthanasia. The time of delivery, litter size, and weight were recorded. On day 1 postpartum, litter sizes were standardized to eight pups. As most of the pups from the OFA rats died within the first 3 days of lactation, for breeding purposes they were fostered from the first day postpartum to Wistar or SD mothers that had given birth at the same time as the OFA rats, and the OFA rats were given litters of eight SD or Wistar pups of the same birth date; dead pups were replaced with Wistar or SD pups of the appropriate age to maintain litters of eight pups during all the experiments. The surviving fostered pups given to the OFA mothers achieved normal growth rates. Animal maintenance and handling was performed according to the NIH guide for the Care and Use of Laboratory Animals (NIH publication No. 86-23, revised 1985 and 1991) and the UK requirements for ethics of animal experimentation (Animals (Scientific Procedures) Act 1986). All the experimental procedures were approved by the Institutional Animal Ethics Committee.
Induction of stress
Groups of eight rats were moved to a contiguous room within the animal house and were rapidly exposed to ether vapor as a physical stressor (Neill Citation1970), by individually placing the rats in a jar containing saturated ether vapor for 2 min (Jahn and Deis Citation1986; Deis et al. Citation1989). Rats were bled (approximately 0.8–1 ml) from the tail vein during the 2-min ether exposure (ether) and 5 min after ether exposure (5 min after ether). A transverse cut was first made with a sterile scalpel blade of 1–2 mm from the tip of the tail, and gentle massaging was applied from the base to the tip of the tail to increase blood flow initially. Basal samples were taken under light ether anesthesia in the animal house from other groups of rats in the same experimental situation. These blood samples were always taken within 30–45 s after removing the rat from its cage and placing it in the ether jar; we compared the results with hormone values obtained from decapitated rats in the different physiological states, and found no significant differences confirming that the values obtained represent those of unstressed animals. Thus, the stress procedure involved a combination of moving to the adjacent room and inhaling ether vapor. Blood was collected without anticoagulant, and serum separated by centrifugation and stored at − 30°C for prolactin, progesterone, and corticosterone RIA.
Experimental design
Experiment 1
Hormonal responses to stress during the estrous cycle, late pregnancy, and mid-lactation.
Rats in proestrus, estrus, and diestrus I and II, on day 19 of pregnancy (G19) or day 11 of lactation (L11), were stressed as described above between 11.00 and 12.00 h, with the exception of the lactating rats that were isolated from their litters at 08.00 h and stressed between 15.30 and 16.00 h. The choice of the sampling times was based on the following: (a) in the non-lactating rats, prolactin levels are at nadir at midday, i.e. this is a time outside the circadian or ovarian steroid-induced peaks; (b) lactating rats were sampled at 16 h because this is also an off-peak hour, allows for the separation of the litter during 8 h to be performed during the lights-on period, and we have determined previously that at this hour the suckling-induced prolactin secretion is maximal, whereas at midday it is minimal (unpublished).
Experiment 2
Hormonal response to stress in ovariectomized rats after steroid hormone administration.
Adult female rats were ovariectomized through two dorsolateral incisions under ketamine–xylazine anesthesia (ketamine hydrochloride 80 mg/kg, xylazine 4 mg/kg dissolved in saline, i.p.). Two weeks after surgery groups of eight ovariectomized rats were injected with estradiol benzoate (5 ug/rat s.c., in 0.2 ml sunflower seed oil 48 h before stress; ovariectomized + estradiol), progesterone (5 mg/rat s.c. in 0.2 ml sunflower seed oil at 16 and 2 h before ether exposure; ovariectomized + progesterone), ovariectomized plus estradiol and progesterone (ovariectomized + estradiol + progesterone) or oil. All the rats were stressed as described above between 11.00 and 12.00 h, 48 h after the start of treatments.
Experiment 3
Effect of stress on suckling-induced milk transfer and circulating hormones.
Groups of 8–10 mothers from the three strains, nursing litters of eight Wistar or SD pups, were isolated from the litter at 08.00 h for 8 h on 3 consecutive days (L10, 11, and 12). On all 3 days, the litters were weighed before reunion with their mothers at 16.00 h and weighed again after 30 min, 2, and 4 h of vigorous suckling. To avoid the weight error resulting from loss of urine produced by maternal licking, the bladders of the pups were evacuated manually before weighing and reunion with the mothers. On the second day, dams were exposed to ether vapor for 2 min between 15.40 h and 15.50 h. The pups were returned to their dams at 16.00 h, and after 30 min suckling the mothers were bled from the tail vein under light ether anesthesia to measure prolactin, progesterone, and oxytocin concentrations. Other groups of rats (controls) were not subjected to stress and were bled 30 min after replacing the litters or, to measure basal levels of hormones, were bled at 16.00 h before replacing the pups, all on the second day.
Experiment 4
Effect of diazepam on lactation performance of OFA hr/hr rats.
Lactating OFA rats were given diazepam (4 mg/100 ml) in the drinking water from G19 to L15. Water intake was about 20 ml/day in dams weighing about 250 g, giving an approximate dose of diazepam of 3 mg/kg/day. Control OFA rats were given water only. Timing and duration of delivery were not affected by diazepam. After delivery, the OFA pups were replaced with eight newborn Wistar or SD pups as described above. To determine the survival rate of the pups, in this case dead pups were not replaced. The number and weight of surviving pups were measured thrice weekly. To determine the pattern of prolactin secretion during lactation, other groups of control or diazepam-treated rats were bled by the tail vein under light ether anesthesia at 16.00 h on days 2 and 7 of lactation, and were subjected to an acute suckling experiment on days 10–12 as described for Experiment 3, without subjecting them to stress. For these experiments, the mothers were provided with a litter of eight pups, of the appropriate age, for the 30-min suckling period, so as to homogenize the suckling stimulus.
Hormone determinations
Prolactin was measured by double antibody radioimmunoassay, using materials provided by Dr A. F. Parlow and the NHPP (National Hormone and Pituitary Program, Harbor-UCLA Medical Center, Torrance, CA, USA) according to the manufacturer instructions as described previously (Valdez et al. Citation2007). The results were expressed in terms of the rat prolactin RP-3 standard preparation. Assay sensitivity was 0.2 ng/tube and the inter-assay and intra-assay coefficients of variation were less than 10%. Oxytocin was measured in unextracted sera by double antibody radioimmunoassay as described previously (Valdez et al. Citation2007), using an antibody and purified oxytocin generously provided by Dr N. Hagino and by Novartis Argentina, respectively. The standard curve was prepared using the same preparation of purified oxytocin used for radioiodination. To maximize sensitivity of the assay, the standards and serum samples were incubated 24 h at 4°C with appropriate dilution of the antibody (1/104–2 × 104), subsequently the labeled hormone (8–10 × 103 cpm) was added and the tubes incubated overnight at 4°C before addition of the second antibody. Assay sensitivity was 3.2 pg/tube and the intra-assay coefficient of variation was less than 10%. All the samples were measured in the same assay by duplicate.
Serum progesterone was measured in unextracted sera, according to the manufacturer's instructions, using commercial kits (DSL-3400 double antibody RIA, from Diagnostic Systems Laboratories, Webster TX, USA) as described previously (Valdez et al. Citation2007). Assay sensitivity was less than 70 fmol/tube and the inter-assay and intra-assay coefficients of variation were less than 10%. Serum corticosterone was measured in serum aliquots extracted with diethyl ether using a radioimmunoassay developed in our laboratory (Jahn et al. Citation1995). Briefly, 50 μl aliquots of serum were extracted twice with 0.5 ml diethyl ether. The pooled organic phases were evaporated and resuspended in 500 μl PBS. Appropriate aliquots of the resuspended samples were incubated overnight with antisera (at dilutions between 1/5000 and 1/104) prepared in our laboratory, raised in rabbits against corticosterone-6-CMO-bovine serum albumin conjugate and 3H corticosterone (1,2,6,7-3H Corticosterone, New England Nuclear, Boston, MA, USA). Hormone–antibody complexes were separated from free hormone by the carbon dextran method and the bound hormone counted in a liquid scintillation counter. Assay sensitivity was lower than 70 fmole/tube. Recovery rates were higher than 90% and inter-assay and intra-assay coefficients of variation were less than 10%.
Determination Of Hypothalamic Prlrlong, Er And Pr Mrna Abundance By Rt Pcr
Groups of six rats on G19 were decapitated without anesthesia, the brains rapidly removed, placed on an ice-cold stainless steel slicer (model RBM-4000C, ASI, Instruments, Inc., Warren, Michigan, USA), the MBH dissected, and stored at − 80°C until processed for total RNA and protein isolation with TRIzol (GIBCO-BRL, Gaithersburg, Maryland, USA) according to the manufacturer's instructions.
Approximately 2 μg samples of total RNA were reverse transcribed (RT) at 37°C for 60 min with Moloney murine leukemia virus retrotranscriptase and random hexamer primers (Invitrogen/Life Technologies, Buenos Aires, Argentina) in 20-μl reaction mixture. Appropriate aliquots of the reverse transcription reaction mix cDNA were amplified with the rat-specific primers and in the conditions shown in . The conditions and quantities of cDNA added were such that the amplification was in the exponential phase, and the assay was linear with respect to the amount of input cDNA. The PCR products were analyzed on 1.5% agarose gels containing 0.5 mg/ml ethidium bromide. Appearance of a single band of the correct molecular size was confirmed. Gels were photographed using a Kodak DC 290 Zoom Digital Camera. Band intensities of RT-PCR products were quantified using NIH Image software. Each PCR assay included two negative controls, without reverse transcription or without template in the PCR reaction. The gene expression patterns of PR, ERα, ERβ, and PRLRlong were normalized to that of β-actin cDNA.
Table I. Primer sequences and reaction conditions used in the PCR amplification of the various cDNAs.
Determination of hypothalamic PR protein abundance by Western blot
TRIzol protein fractions were dissolved, quantified by the Bradford method, and 20 μg protein aliquots separated by SDS-PAGE and electrotransferred to Hybond membranes as described previously (Valdez et al. Citation2007). After rinsing and blocking with 2% BSA and 2% horse serum (Valdez et al. Citation2007), the membranes were probed with anti-PR (1/1000 rabbit polyclonal, sc539, Santa Cruz Biotechnology, Inc., California, USA; this antibody recognizes equally the two isoforms, which are a product of alternative splicing in rat, human, mouse, and monkey tissue samples) using horseradish peroxidase-conjugated secondary antisera (1/3000 polyclonal goat anti-rabbit, Dakocytomation, CA, USA) and chemiluminiscence (Amersham ECL™, GE Healthcare, Buenos Aires, Argentina) to detect the specific PRA and PRB isoform bands that were quantified by densitometry using NIH Image 1.6/ppc freeware program. The membranes were stripped and reprobed with anti-β-actin (1/10,000 mouse monoclonal antibody, Sigma, St Louis, MO, USA) and horseradish peroxidase-conjugated secondary antisera (1/3000 polyclonal goat anti-mouse immunoglobulins, Dakocytomation) as loading and transfer controls.
Statistics
Statistical analysis was performed using one- or two-way analysis of variance followed by the τ-test for comparison between means (Snedecor and Cochran Citation1968). Results are expressed as means ± SEM, and differences between means were considered significant at the P < 0.05 level.
Results
Hormone release in response to stress in female rats during the estrous cycle, late pregnancy, and mid-lactation (Experiment 1)
Exposure to ether produced significant increases in circulating serum concentrations of prolactin, progesterone, and corticosterone in the three strains in most of the situations studied.
Prolactin
On all the days of the cycle, two-way ANOVA analysis () showed that exposure to ether significantly elevated circulating prolactin concentration (effect of ether exposure, at least P < 0.05 for all days of the cycle, ), and that there were significant differences in response among the three strains (effect of strain, at least P < 0.001 for all days of the cycle, ). Basal prolactin concentrations were similar in the three strains and between the different days of the estrous cycle, but there were significant differences in the magnitude of the response to ether exposure among them, particularly in the SD strain, in which the maximal response was observed on estrus and the minimal on diestrus II (). The responses of the three strains were similar in proestrus and diestrus II, with significant increases in circulating prolactin during ether exposure that persisted for at least 5 min (). However, there were differences in the extent of the response among the three strains on the other days of the cycle. At estrus, ether exposure induced the smallest prolactin increase in Wistar rats, the highest and earliest increase in SD rats, and OFA rats had intermediate values (). At diestrus I, the response of the Wistar rats was similar to that in estrus, while both OFA and SD rats achieved maximal and similar prolactin values during ether exposure ().
Table II. Statistical parameters of the two-way ANOVAS corresponding to the results presented in .
Figure 1. Changes in serum concentrations of prolactin, progesterone, and corticosterone in response to ether exposure in OFA, SD, and Wistar rats during the estrous cycle, late pregnancy, and mid-lactation. Panel A: prolactin (PRL), Panel B: progesterone (P4), Panel C: corticosterone. Groups of 8–10 rats were moved to an experimental room and exposed to ether vapor for 2 min. Tail vein blood samples were collected as indicated; basal samples were taken under light ether anesthesia. Hormones were measured by radioimmunoassay in serum. Values are mean ± SEM. *P < 0.05 vs. basal concentration in the same strain, #P < 0.05 vs. Wistar rats at same time point, ‡P < 0.05 vs. SD rats at same time point; two-way ANOVA and τ-test.
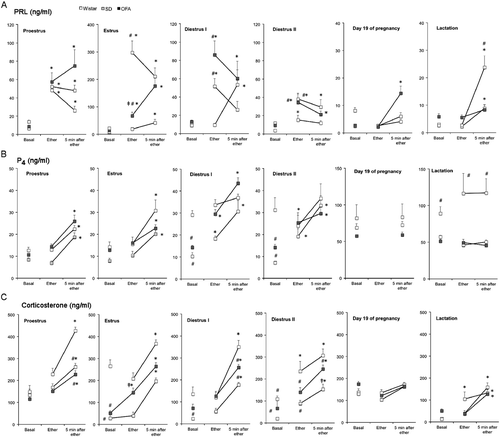
In late pregnancy, in Wistar and SD rats, prolactin secretion did not increase with ether exposure, while the OFA rats had elevated serum prolactin concentration at 5 min after ether. Two-way ANOVA showed a significant effect of strain (P < 0.0038). The mid-lactation rats of the three strains separated from their pups had significantly elevated prolactin concentrations only 5 min after ether exposure, but the response of the OFA rats was significantly greater. Two-way ANOVA showed significant effects of strain (P < 0.01) and of ether exposure (P < 0.0001) ( and ).
Progesterone
Two-way ANOVA showed significant effects of strain (at least P < 0.01) and of ether exposure on all days of the cycle (at least P < 0.01; ). Serum progesterone concentrations increased similarly in stressed rats of the three strains at proestrus and estrus (). In diestrus I and II, basal progesterone concentrations were higher in Wistar rats compared with SD and OFA, which resulted in an attenuated response in this strain (). On G19 and in lactation, stress did not modify the high basal progesterone concentrations, but SD rats had higher concentrations compared with the others ( and ).
Corticosterone
Serum corticosterone concentrations varied with estrous cycle stage and strain (effect of strain, P < 0.0001), and ether exposure significantly (P < 0.0001) increased these values ( and ). Wistar rats had consistently higher basal values, with the highest values at estrus (P < 0.01). SD and OFA rats had elevated basal corticosterone in proestrus relative to the other days (P < 0.01). In diestrus, OFA rats showed slightly higher basal values compared with SD rats. However, the corticosterone response to ether exposure was similar for the three strains, although Wistar and SD rats tended to have the highest and lowest responses, respectively. On G19, as for prolactin and progesterone, stress did not elicit corticosterone release. By contrast, in lactation, basal corticosterone concentrations were low and the response to stress was restored and similar in the three strains (P < 0.0001), but basal values were higher (P < 0.01) in OFA rats compared with the other strains.
Hormone release in response to stress in ovariectomized rats treated with ovarian steroids (Experiment 2)
In order to explore the role of ovarian steroids on the different responses to ether exposure observed in cycling, pregnant, and lactating rats, we determined the effect of stress on ovariectomized rats (which have minimal circulating levels of estrogens and progesterone) and the effect of estrogen and/or progesterone administration. Ovariectomy and steroid replacement affected the prolactin response to stress more markedly than the progesterone or corticosterone responses.
Prolactin
In ovariectomized rats, the prolactin response to stress was significant (P < 0.0002) but modest in the three strains. However, there were strain differences (P < 0.0001), since ether exposure elevated serum prolactin concentrations significantly in SD and OFA rats but not in Wistar rats (; ANOVA data in ).
Figure 2. Changes in serum concentrations of prolactin, progesterone, and corticosterone in response to ether exposure in ovariectomized rats after steroid hormone administration. Panel A: prolactin (PRL), Panel B: progesterone (P4), Panel C: corticosterone. Two weeks after ovariectomy rats were divided into four groups: ovariectomized with oil administration (OVX); ovariectomized treated with estradiol benzoate (5 μg/rat s.c. 48 h previously, OVX+E2); ovariectomized treated with progesterone (5 mg/rat s.c. 14 and 2 h previously, OVX + P4); ovariectomized treated with estradiol and progesterone (OVX + E2P4). Groups of eight rats were moved to an experimental room and exposed to ether vapor for 2 min. Tail vein blood samples were collected as indicated; basal samples were taken under light ether anesthesia. Hormones were measured by radioimmunoassay in serum. Values are mean ± SEM. *P < 0.05 vs. basal concentrations of the same strain, #P < 0.05 vs. Wistar rats at the same time point, ‡P < 0.05 vs. SD rats at the same time point; two-way ANOVA and τ-test.
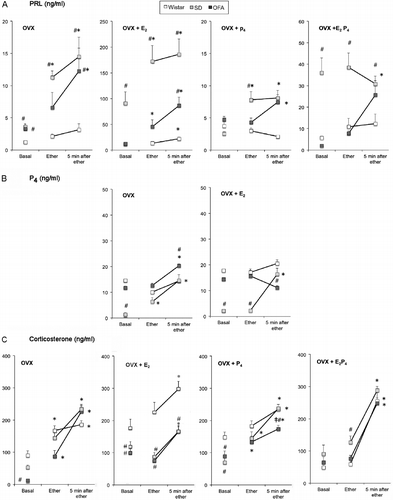
Estradiol administration increased basal serum prolactin concentrations in all three strains, but the effect was more marked in SD rats (approximately 30-fold vs. 4-fold and 10-fold in the OFA and Wistar strains, respectively; , ). There were significant effects of strain (P < 0.0001, ) and of ether exposure (P < 0.0002, ). SD rats also showed the greatest response to ether exposure (). OFA rats also showed significantly increased prolactin values but with markedly lower values than in SD rats (). Wistar rats had the smallest response, but in contrast with the ovariectomized group, the increase in circulating prolactin was significant ().
Administration of two doses of progesterone did not modify significantly the pattern of response to ether exposure in ovariectomized rats of the three strains. Thus, there were strain differences (P < 0.005) in the response: serum prolactin concentration after ether exposure was increased in SD and OFA rats but not in Wistar rats ( and ). Administration of progesterone to estrogen-treated ovariectomized rats lowered basal serum prolactin concentrations in the three strains (), and there were strain differences (P < 0.0001, ): the response to ether was blocked in SD and Wistar rats, but OFA rats showed an increase in prolactin secretion similar to that seen in the ovariectomized group ( and ).
Progesterone
Basal progesterone concentrations in ovariectomized rats were significantly lower in SD rats compared with the other strains (). Two-way ANOVA showed significant effects of strain (P < 0.0001) and of ether exposure (P < 0.0001, ). The progesterone response to ether exposure was similar to the prolactin response, with a significant increase in SD and OFA rats and no response in the Wistar strain (). Administration of estrogen did not modify basal values when compared with ovariectomized rats, but blocked the effect of ether exposure on progesterone secretion in OFA but not in SD rats, and Wistar rats did not respond ( and ). Administration of progesterone to ovariectomized or estrogen-treated ovariectomized rats resulted in serum progesterone concentrations between 100 and 200 ng/ml, which were not modified by ether exposure (results not shown).
Corticosterone
The effect of ovariectomy and steroid hormone replacement on the corticosterone response to ether exposure was qualitatively different from the effects on prolactin and progesterone ( and ). Regardless of the steroid hormone treatment, there were significant strain differences (P < 0.05 or less, ) and significant responses to ether exposure (P < 0.0001, ). As in cycling rats, basal values were higher in Wistar rats compared with the other strains, except in the ovariectomized+estradiol+progesterone-treated rats, where the three strains had similar values (). The magnitude of the response to ether exposure was also similar in the three strains, and ovarian steroid treatments did not modify this response significantly except in the ovariectomized+estradiol Wistar rats that had significantly higher values at all times ().
Effect of ether exposure on serum prolactin, progesterone, and oxytocin concentrations in response to the suckling stimulus in rats of the three strains (Experiment 3)
As stress inhibits lactation and the lactating OFA rats had greater hormonal responses than the other strains, we investigated whether a previous stress episode affects suckling-induced hormone release and milk transfer. For this, we performed an acute suckling experiment over 3 days, exposing the mothers to ether as a stressor on the second day, 10–20 min before initiation of suckling.
Hormone release induced by 30 min suckling was partially (prolactin) or totally (oxytocin and progesterone) blocked in control OFA compared to SD and Wistar rats (; ANOVA data in ). Ether exposure did not affect suckling-induced hormone secretion in SD and Wistar rats, but produced a profound inhibition of prolactin release in OFA rats ( and ).
Figure 3. Effect of ether exposure on suckling-induced milk ejection and circulating hormones. Panel A: effect of stress on suckling-induced hormone release in lactating Wistar (Wi), SD, and OFA rats. Litters were separated from the mothers at 08.00 h for 8 h on 3 days. On day 2, dams were exposed to ether vapor for 2 min before reunion. Tail vein blood samples were collected under light ether anesthesia before pups were returned and after suckling for 30 min. Hormones were measured by radioimmunoassay in serum. Panel B: amount of milk drawn per pup nursed by non-stressed (day 1 and 3) and stressed mothers (day 2). The litters were weighed before reunion with their mothers at 16.00 h (0) and after 0.5, 2, and 4 h of suckling. Data are weight change between weighings. Panel C: total amount of milk obtained per pup in the 4 h suckling period. Data are mean ± SEM; n = 8–10 rats per group. Data were analyzed by two-way ANOVA and τ-test.
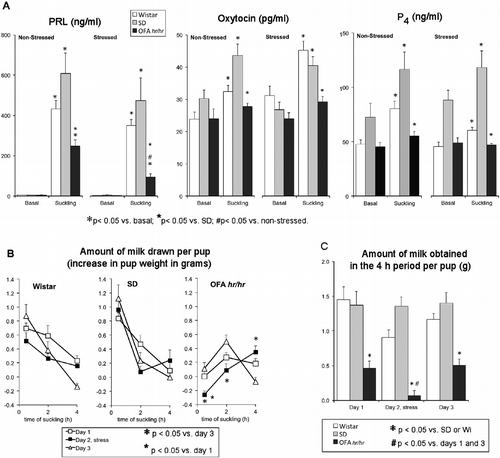
Table III. Statistical parameters of the two-way ANOVAS corresponding to the results presented in .
In SD and Wistar rats, the pups obtained most milk during the first 30 min of suckling (effect of time P < 0.0001, ). Ether exposure had no significant effect on this pattern nor on the total amount of milk obtained in the 4 h period in SD and Wistar rats ( and ). By contrast, pups suckling control OFA rats obtained significantly less milk at later times (effect of time P < 0.0001, ), compared with the other strains ( and ) and ether exposure blocked milk transfer further: the pups obtained even less milk and later ( and ). When the total amount of milk drawn per pup in the 4-h suckling period was analyzed, there were significant differences between the OFA and the other two strains (P < 0.0001) and a significant effect of stress (P < 0.017) that was limited to the OFA strain ( and ). Milk transfer is a reliable indicator of milk ejection and oxytocin release, as the release of oxytocin induced by suckling is responsible for the contraction of the myoepithelial cells around the alveoli, which ejects the milk. Thus, these findings indicate a significant impairment in the milk ejection reflex in the OFA strain that is accentuated by stress.
Effect of diazepam administration on the lactational performance of OFA rats (Experiment 4)
In order to test the hypothesis that heightened susceptibility to stress during lactation contributes to lactation failure in OFA rats, we studied the effect of administration of the anxiolytic diazepam during late pregnancy and lactation on survival and growth rates of the pups. Diazepam treatment significantly improved lactational performance in OFA rats, increasing the number of surviving litters to almost 90% and increasing the number of surviving pups per litter (P < 0.0001; , ). In both groups, the surviving pups grew at a normal rate () confirming previous results (Valdez et al. Citation2007). Furthermore, serum prolactin concentration was significantly greater on L2 and L7 in the diazepam-treated rats and the response to 30-min acute suckling was also significantly augmented (P < 0.0001, , ). However, milk ejection, measured as the change in litter weight after 30 min suckling, was not improved (controls 0.08 ± 0.06 g/pup, diazepam 0.03 ± 0.10 g/pup). Diazepam also elevated basal levels of prolactin in the non-suckled rats (). There were no differences in survival rates and growth curves of the litters of the groups of rats that were sampled for prolactin determination and those that were not.
Figure 4. Effect of diazepam on the lactation performance of OFA hr/hr rats. Panel A: the percentage of surviving litters. Panel B: number of surviving pups per litter. Panel C: pup weight gain. Panel D: serum prolactin concentrations on days 2 (L2) and 7 (L7) and after 8 h separation (separated) and 30 min suckling (suckled) on day 12 of lactation (L12). Lactating OFA rats were given diazepam (DZP) in the drinking water or water alone (Co) from day 19 of pregnancy until day 15 of lactation. OFA dams nursed eight SD foster pups. Data are mean ± SEM of groups of 18 rats for panels A–C and 8–10 rats for panel D. *P < 0.05 vs. control rats; two-way ANOVA and τ-test.
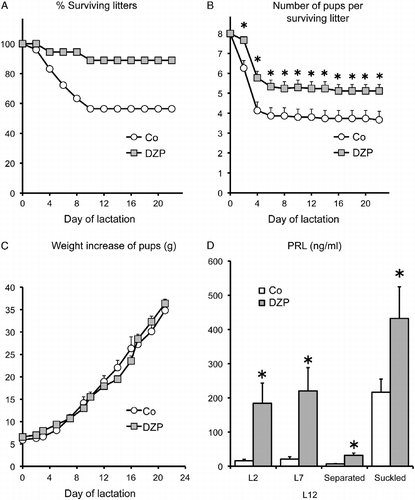
Hypothalamic expression of PR, ER, and PRLRlong mRNA and PR proteins in the three strains
As our results indicated that SD rats may have increased response to estrogens, while OFA rats evidently had reduced sensitivity to the inhibitory effects of progesterone on stress responses, we measured by semiquantitative RT-PCR the expression relative to β-actin of estrogen (ERα and β), progesterone (total and PRB), and prolactin (PRLRlong) receptor mRNAs in the MBH (which includes the arcuate and periventricular nuclei, important in the control of prolactin secretion). This experiment was done on G19, when the response to stress was blocked in SD and Wistar but still present in OFA rats (). The OFA strain showed significantly higher expression of total PR mRNA, while there were no significant differences in ERs nor in PRLRlong mRNA levels (). By contrast, at the protein level, total PR expression was similar in the three strains, although the ratio of the short A form to the long B form was strikingly different between strains (). Thus, in Wistar rats PRB protein abundance was low, in SD rats the abundance of the two forms was similar, while in OFA rats the mean abundance of the A form was approximately 50% higher than that of the B form ().
Figure 5. Hypothalamic prolactin (long form, PRLRlong), estradiol (ER), and progesterone (PR) receptor mRNA abundance by RT PCR and progesterone receptor protein by Western blot at the end of pregnancy in OFA, SD, and Wistar rats. Panel A: representative photograph of RT-PCR products run in 1.5% agarose gel of three samples of each strain of rats. Panel B: semiquantitative PCR analysis of total PR, ERα, ERβ, and PRLRlong mRNA abundance relative to β-actin in mediobasal hypothalamus (MBH). Panel C: representative immunoblots of hypothalamic PR isoforms and β-actin in the three rat strains. Panel D: expression relative to β-actin of PR isoforms A and B, PR isoform ratio, and total PR. Data are mean ± SEM; n = 8 rats of each strain. #P < 0.05 vs. Wistar and SD rats, *P < 0.05 vs. Wistar rats, ‡P < 0.05 vs. SD rats; two-way ANOVA and τ-test.
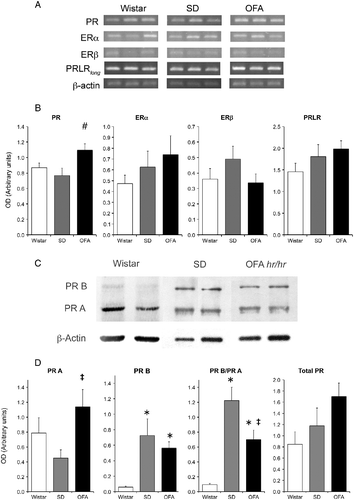
Discussion
The present results reveal that Wistar, SD, and OFA rats have different responses to stress, particularly in terms of prolactin release, which depend on the reproductive state and on the differential sensitivity of each strain to the particular steroidal hormone milieu of each state. The exaggerated hormonal responsiveness to stress observed in the OFA rats during lactation may be partially responsible for the lactation failure of these rats. This is indicated by the higher prolactin response to stress in pregnant and lactating OFA rats, by the capacity of a mild stress episode to block the milk ejection reflex in OFA but not in the other strains and by the capacity of an anxiolytic drug to improve lactation performance in this strain.
The differences in the prolactin response to stress observed during the estrous cycle, pregnancy, and lactation can be linked to the variations in circulating estradiol and progesterone levels, where estrogens potentiate the response and progesterone, perhaps acting through its 5α-reduced metabolites, inhibits it. This conclusion is supported by the results obtained with the estrogen- and/or progesterone-treated ovariectomized rats.
SD rats, compared with Wistar rats, showed elevated sensitivity to estrogens in terms of prolactin release, evidenced by higher basal levels and greater prolactin responses to acute stress during estrus and in estrogen-treated ovariectomized rats. This difference confirms that SD and Wistar strains are not equivalent in terms of their neuroendocrine responses that are influenced by estrogens. Compared to Wistar rats, OFA rats evidently share the elevated sensitivity to estrogen of their parent strain, but to a lesser degree. This difference could be accounted for by the increased activation of the dopaminergic system by elevated prolactin levels previously described (Valdez et al. Citation2007). The end of pregnancy and lactation are characterized by a desensitization of the neuroendocrine dopaminergic neurons to elevated prolactin levels that will allow the maintenance of chronically elevated prolactin levels during lactation (Andrews et al. Citation2001). The diminution of tuberoinfundibular dopamine (TIDA) neuronal activity is evidenced during late pregnancy and lactation as a fall in TH expression and DA turnover (Andrews et al. Citation2001; Grattan et al. Citation2008); this fall is not observed in OFA rats and may be one cause of the reduced prolactin levels observed during lactation (Valdez et al. Citation2007).
Our results indicate that OFA and SD rats are more sensitive in their prolactin response to stress than Wistar rats, especially when under the influence of estrogen. Estrogens have stimulatory action on prolactin release at the pituitary and central nervous system level, and some of these actions are mediated through modulation of TIDA neuron activity (Arbogast and Voogt Citation1993). Although MBH ERα or ERβ expression at the mRNA level was similar in the three strains, we cannot exclude strain differences in ER expression at other sites or in the neuronal responsiveness to estrogen signaling that may account for the differential sensitivity to estrogens.
The present study confirms the inhibiting effects of elevated circulating progesterone levels on the prolactin response to stress demonstrated previously in proestrous Wistar rats (Jahn and Deis Citation1986) and extends this finding to SD and OFA rats. However, the inhibiting effect of progesterone was weaker in OFA estradiol-treated ovariectomized rats, indicating a reduced sensitivity of this strain to progestogens that may explain also their conserved responses during pregnancy and lactation. Although pregnant OFA rats had higher total MBH PR mRNA content when compared with the parent strain, at the protein level they had significantly higher content of PRA and lower PRB/PRA ratio. It has been postulated that PRB mediates most of the genomic actions of progesterone, while PRA may act as a negative regulator of PRB action (Giangrande and McDonnell Citation1999). There is evidence that a differential PR isoform ratio is essential for the cell-specific functional role of these proteins (Turgeon and Waring Citation2006). For example, an appropriate PRB/PRA expression ratio is critical for mammary development, and an unbalanced ratio accompanies structure disorganization (Simian et al. Citation2009). The reduced PRB/PRA ratio in pregnant OFA compared with SD rats may be one cause of the reduced sensitivity of the former to the inhibiting effect of progesterone on stress responses, which results in a conserved response to stress in OFA rats in late pregnancy and lactation.
However, in addition to the reduced sensitivity to estrogens, Wistar rats had the lowest PRB expression and PRB/PRA ratio, but the response to stress during pregnancy and lactation was similar to that in SD rats. Wistar rats also had lower response to estrogens in terms of basal- or stress-induced prolactin release. The higher basal corticosterone levels may be responsible, through negative feedback, for the reduced prolactin response to stress in the Wistar rats compared with the other two strains, and this may have compensated for the low PRB/PRA ratio. However, we cannot preclude the existence of strain differences in responsiveness to ovarian steroids at a level different from that of steroid receptor expression in the MBH, for example, PR expression in other brain areas.
Although the increase in circulating progesterone induced by stress is of adrenal origin (Deis et al. Citation1989), the stress episode did not elicit parallel progesterone and corticosterone responses in all the different experimental situations. On the contrary, the progesterone response was more similar to that of prolactin than to the corticosterone response, especially in the ovariectomized and estradiol-treated ovariectomized groups, indicating that the prolactin released by the stress episode may be responsible for the progesterone release by the adrenals, while corticosterone release is driven by ACTH. Supporting this conclusion, previous evidence showed that prolactin releases adrenal progesterone and corticosterone (Chang et al. Citation1999; Lo and Wang Citation2003), directly and through potentiation of ACTH action (Figueiredo et al. Citation2007; Jaroenporn et al. Citation2007). However, in states with elevated circulating progesterone levels of luteal origin, such as pregnancy, lactation, or diestrus (only in Wistar rats), the high basal levels of progesterone may have masked any increase in adrenal progesterone release induced by stress. It is interesting to note that basal corticosterone levels also differed among the strains, with Wistar>OFA>SD in most situations. Wistar rats also displayed higher basal progesterone levels in diestrus I and II, indicating that the luteal phase in Wistar rats may start earlier and end later than in the other strains.
Although pregnancy and lactation are characterized by hyporeactivity of the HPA axis, stress is a powerful blocker of the suckling reflex, by a direct inhibition of oxytocin and prolactin release or indirectly through activation of adrenergic afferents to the central nervous system (Feldman Citation1985; Lau Citation2001). Likewise, inhibition of prolactin secretion is intimately related to maternal lactation failure (Weichert Citation1979), hence stress is considered a major hindrance for a successful lactation in humans.
The reduced sensitivity of the HPA axis to an acute stressor, observed during pregnancy and lactation (Stern et al. Citation1973; Lightman and Young Citation1989; Neumann et al. Citation1998; Johnstone et al. Citation2000), may be due to elevated levels of circulating progesterone, lactogenic hormones, and oxytocin. The elevated circulating progestogens during pregnancy and lactation have anxiolytic effects and reduce hormonal responses to stress (Patchev et al. Citation1994, Citation1996). Progesterone, through conversion to allopregnanolone, reduces activation of the corticotropin-releasing hormone neurons in the paraventricular nucleus through suppression of noradrenaline release from terminals originating in the brain stem by an upregulated endogenous opioid mechanism (Brunton et al. Citation2008, Citation2009). Another mechanism mediating the suppression of the HPA axis in late pregnancy is the interaction of allopregnanolone with GABAA receptors (Bitran et al. Citation1993), whose activation in the periaqueductal gray has also been implicated in anxiety reduction (Miller et al. Citation2010). Thus, although the altered PRB/PRA ratio observed in the MBH of OFA rats in late pregnancy may play a role in the conserved sensitivity to stress during pregnancy and lactation in this strain, we cannot discard alterations in opioid or GABA modulation by progestogens and their metabolites.
Concerning the effect of lactogenic hormones, prolactin has anxiolytic effects (Carter and Lightman Citation1987) and attenuates the corticoid response to stress (Schlein et al. Citation1974). During pregnancy and lactation there is an increase in prolactin and PRLR expression in several brain areas that play a key role in the decrease of the reactivity of the HPA axis during lactation (Torner et al. Citation2001, Citation2002; Torner and Neumann Citation2002). The possible mechanisms mediating these anxiolytic actions may be through inhibition of ACTH release (Figueiredo et al. Citation2007; Jaroenporn et al. Citation2007) and stimulation of 5α-reductase expression, the enzyme that converts progesterone to allopregnanolone, since it has been shown that hyperprolactinemia increases brain mRNA levels of this enzyme (Sánchez et al. Citation2008, Citation2009). The low circulating prolactin levels of the lactating OFA rats, produced by the elevated hypothalamic dopaminergic tone in late pregnancy and lactation, may result in a higher reactivity of the HPA axis mediated by direct actions of prolactin and also by reduced 5α-reductase activity and diminished responsiveness to the anxiolytic effects of elevated circulating progesterone.
Oxytocin, released centrally by the suckling stimulus, also contributes to suppress anxiety in the postpartum through activation of its receptors in the periaqueductal gray (Figueira et al. Citation2008), and thus, the low suckling-induced oxytocin release into the circulation observed in the OFA rats (Valdez et al. Citation2007 and ) may indicate an additional factor contributing to the elevated sensitivity to stress observed in lactating rats of this strain.
Any mild stress suffered by the lactating OFA rats may impair the suckling reflex and reduce still further circulating prolactin and oxytocin levels, thereby increasing further the sensitivity to stress and anxiety levels in a positive feedback loop resulting in defective lactation. The increased pup survival and restoration of suckling-induced prolactin release produced by diazepam administration to lactating OFA rats supports this hypothesis. Hence, we found high anxiogenic parameters in OFA rats examined on the elevated plus maze that were lowered by diazepam treatment (Gonzalez et al. Citation2007). As diazepam has no significant effect on circulating prolactin level in non-lactating female rats (Grandison Citation1983; Lacau de Mengido et al. Citation1989), it is quite probable that the restoration of suckling-induced prolactin release is more related to the anxiety lowering properties of diazepam than to direct neuroendocrine effects. However, the elevated basal prolactin level observed in the non-suckled OFA group seems to be a phenomenon specific to this strain or to the lactating state that may be related to other neuroendocrine effects of diazepam yet to be elucidated.
It is interesting to compare OFA rats with other rat models of altered susceptibility to stress such as the Hatano and Roman high (HA) and low (LA) avoidance rats, which are SD- and Wistar-derived strains, respectively. The LA substrains have increased prolactin release in response to stressful stimuli, with small differences in corticosterone release, although they present marked differences in ACTH regulation and adrenal sensitivity to ACTH (Walker et al. Citation1989; Driscoll et al. Citation1998; Asai et al. Citation2004a). Furthermore, when compared with the respective HA substrains, Hatano LA rats show impaired suckling reflex and maternal behavior and a mild decrease in pup growth rate (Asai et al. Citation2004b), while Roman LA rats show decreased brain 5α-reduced progesterone metabolites (Steimer et al. Citation1997), suggesting low 5α-reductase activity. Thus, the LA substrains may share with the OFA rats an elevated susceptibility to stress through a mechanism in which prolactin itself and the regulation of 5α-reductase may play a central role.
In conclusion, the present results show that OFA rats have an enhanced sensitivity to stress during lactation that may cause their lactation deficit. This increased sensitivity to stress may result from a combination of lower circulating prolactin and oxytocin levels during lactation, and altered responsiveness to ovarian steroids, in particular, a lower capacity of elevated circulating progesterone to inhibit the hormonal stress responses. Many of the neuroendocrine adaptations that will enable a successful lactation develop during late pregnancy, among them decreased hypothalamic dopaminergic responses to elevated prolactin and hyporesponsiveness to stress (Brunton et al. Citation2008; Grattan et al. Citation2008). Both of these adaptations are abnormal in the OFA rats (Valdez et al. Citation2007 and present results), highlighting their importance for normal lactation and ultimately, for reproductive success and adequate development of the young.
Acknowledgements
The authors are grateful to Dr N. Hagino for oxytocin antibody and to Dr Marta Soaje for critical reading of this article. This paper is dedicated to the memory of Dr Ricardo P. Deis, deceased on 26 April 2008, who was a pioneer in lactation physiology and whose mentorship, counsel, and suggestions greatly enriched this work.
Declaration of interest: This work has been supported by grants PIP 5795/05 and 2298/09 from CONICET (Consejo nacional de investigaciones científicas y técnicas, Argentina) and PICT-R 32529 from the Agencia de Promoción Científica y Tecnológica, Argentina. GAJ and SRV are Career Scientists from CONICET, and MMB has a fellowship from CONICET. The authors report no conflicts of interest. The authors alone are responsible for the content and writing of the paper.
References
- Altemus M, Deuster PA, Galliven E, Carter CS, Gold PW. 1995. Suppression of hypothalmic–pituitary–adrenal axis responses to stress in lactating women. J Clin Endocrinol Metab. 80:2954–2959.
- Andrews ZB, Kokay IC, Grattan DR. 2001. Dissociation of prolactin secretion from tuberoinfundibular dopamine activity in late pregnant rats. Endocrinology. 142:2719–2724.
- Arbogast LA, Voogt JL. 1993. Progesterone reverses the estradiol-induced decrease in tyrosine hydroxylase mRNA levels in the arcuate nucleus. Neuroendocrinology. 58:501–510.
- Arbogast LA, Voogt JL. 2002. Progesterone induces dephosphorylation and inactivation of tyrosine hydroxylase in rat hypothalamic dopaminergic neurons. Neuroendocrinology. 75:273–281.
- Asai S, Ohta R, Shirota M, Watanabe G, Taya K. Differential responses of the hypothalamo-pituitary–adrenocortical axis to acute restraint stress in Hatano high- and low-avoidance rats. J Endocrinol. 2004a; 181:515–520.
- Asai S, Ohta R, Shirota M, Tohei A, Watanabe G, Taya K. Endocrinological responses during suckling in Hatano high- and low-avoidance rats. J Endocrinol. 2004b; 182:267–272.
- Banky Z, Nagy GM, Halasz B. 1994. Analysis of pituitary prolactin and adrenocortical response to ether, formalin or restraint in lactating rats: Rise in corticosterone, but no increase in plasma prolactin levels after exposure to stress. Neuroendocrinology. 59:63–71.
- Bazzi H, Kljuic A, Christiano AM, Panteleyev AA. 2004. Intragenic deletion in the Desmoglein 4 gene underlies the skin phenotype in the Iffa Credo “hairless” rat. Differentiation. 72:450–464.
- Bitran D, Purdy RH, Kellogg CK. 1993. Anxiolytic effect of progesterone is associated with increases in cortical allopregnanolone and GABAA receptor function. Pharmacol Biochem Behav. 45:423–428.
- Brunton PJ, Russell JA, Douglas AJ. 2008. Adaptive responses of the maternal hypothalamic–pituitary–adrenal axis during pregnancy and lactation. J Neuroendocrinol. 20:764–776.
- Brunton PJ, McKay AJ, Ochedalski T, Piastowska A, Rebas E, Lachowicz A, Russell JA. 2009. Central opioid inhibition of neuroendocrine stress responses in pregnancy in the rat is induced by the neurosteroid allopregnanolone. J Neurosci. 29:6449–6460.
- Carter DA, Lightman SL. 1987. Oxytocin responses to stress in lactating and hyperprolactinaemic rats. Neuroendocrinology. 46:532–537.
- Chang LL, Lo MJ, Kan SF, Huang WJ, Chen JJ, Kau MM, Wang JL, Lin H, Tsai SC, Chiao YC, Yeh JY, Wun WS, Wang PS. 1999. Direct effects of prolactin on corticosterone release by zona fasciculata-reticularis cells from male rats. J Cell Biochem. 73:563–572.
- Chaudhury RR, Chaudhury MR, Lu FC. 1961. Stress-induced block of milk ejection. Br J Pharmacol Chemother. 17:305–309.
- Cross BA. 1955. Neurohormonal mechanisms in emotional inhibition of milk ejection. J Endocrinol. 12:29–37.
- Deis RP, Leguizamon E, Jahn GA. 1989. Feedback regulation by progesterone of stress-induced prolactin release in rats. J Endocrinol. 120:37–43.
- Driscoll P, Escorihuela RM, Fernández-Teruel A, Giorgi O, Schwegler H, Steimer T, Wiersma A, Corda MG, Flint J, Koolhaas JM, Langhans W, Schulz PE, Siegel J, Tobeña A. 1998. Genetic selection and differential stress responses: The Roman lines/strains of rats. Ann N Y Acad Sci. 851:501–510.
- el Abed A, Kerdelhue B, Castanier M, Scholler R. 1987. Stimulation of estradiol-17 beta secretion by 7,12-dimethylbenz (a) anthracene during mammary tumor induction in Sprague-Dawley rats. J Steroid Biochem. 26:733–738.
- Emery DE, Larsson K. 1979. Rat strain differences in copulatory behavior after para-chlorophenylalanine and hormone treatment. J Comp Physiol Psychol. 93:1067–1084.
- Feldman S. 1985. Neural pathways mediating adrenocortical responses. Fed Proc. 44:169–175.
- Figueiredo HF, Ulrich-Lai YM, Choi DC, Herman JP. 2007. Estrogen potentiates adrenocortical responses to stress in female rats. Am J Physiol Endocrinol Metab. 292:E1173–E1182.
- Figueira RJ, Peabody MF, Lonstein JS. 2008. Oxytocin receptor activity in the ventrocaudal periaqueductal gray modulates anxiety-related behavior in postpartum rats. Behav Neurosci. 122:618–628.
- Gala RR. 1990. The physiology and mechanisms of the stress-induced changes in prolactin secretion in the rat. Life Sci. 46:1407–1420.
- Garcia-Segura LM, Diolez-Bojda F, Lenoir V, Naftolin F, Kerdelhue B. 1992. Estrogen-like effects of the mammary carcinogen 7,12-dimethylbenz(alpha)anthracene on hypothalamic neuronal membranes. Brain Res Bull. 28:625–628.
- Giangrande PH, McDonnell DP. 1999. The A and B isoforms of the human progesterone receptor: Two functionally different transcription factors encoded by a single gene. Recent Prog Horm Res. 54:291–313.
- Gonzalez AS, Rodriguez Echandía EL, Valdez SR, Jahn GA. Differential stress response in Sprague Dawley (SD), Wistar (W) and OFA hr/hr (O) female rats. 2007 31st Göttingen Neurobiology Conference and 7th Meeting of the German Neuroscience Society, Göttingen, Germany.
- Grandison L. 1983. Actions of benzodiazepines on the neuroendocrine system. Neuropharmacology. 22:1505–1510.
- Grasselli F, Gaiani R, Tamanini C. 1992. Seasonal variation in the reproductive hormones of male goats. Acta Endocrinol (Copenh). 126:271–275.
- Grattan DR, Steyn FJ, Kokay IC, Anderson GM, Bunn SJ. 2008. Pregnancy-induced adaptation in the neuroendocrine control of prolactin secretion. J Neuroendocrinol. 20:497–507.
- Grosvenor CE, Mena F. 1967. Effect of auditory, olfactory and optic stimuli upon milk ejection and suckling-induced release of prolactin in lactating rats. Endocrinology. 80:840–846.
- Higuchi T, Negoro H, Arita J. 1989. Reduced responses of prolactin and catecholamine to stress in the lactating rat. J Endocrinol. 122:495–498.
- Jahn GA, Deis RP. 1986. Stress-induced prolactin release in female, male and androgenized rats: Influence of progesterone treatment. J Endocrinol. 110:423–428.
- Jahn GA, Moya G, Jammes H, Rosato RR. 1995. Effect of chronic thyroid hormone treatment on cycling, ovulation, serum reproductive hormones and ovarian LH and prolactin receptors in rats. Endocrine. 3:121–127.
- Jaroenporn S, Nagaoka K, Ohta R, Watanabe G, Taya K. 2007. Direct effects of prolactin on adrenal steroid release in male Hatano high-avoidance (HAA) rats may be mediated through Janus kinase 2 (Jak2) activity. J Reprod Dev. 53:887–893.
- Johnstone HA, Wigger A, Douglas AJ, Neumann ID, Landgraf R, Seckl JR, Russell JA. 2000. Attenuation of hypothalamic–pituitary–adrenal axis stress responses in late pregnancy: Changes in feedforward and feedback mechanisms. J Neuroendocrinol. 12:811–822.
- Lacau de Mengido IM, Diaz-Torga GS, Libertun C. 1989. Diazepam: Endocrine effects and hypothalamic binding sites in the developing male and female. Life Sci. 45:567–575.
- Lau C. 2001. Effects of stress on lactation. Pediatr Clin North Am. 48:221–234.
- Lightman SL, Young WSIII. 1989. Lactation inhibits stress-mediated secretion of corticosterone and oxytocin and hypothalamic accumulation of corticotropin-releasing factor and enkephalin messenger ribonucleic acids. Endocrinology. 124:2358–2364.
- Lo MJ, Wang PS. 2003. Relative and combined effects of estradiol and prolactin on corticosterone secretion in ovariectomized rats. Chin J Physiol. 46:103–109.
- Lonstein JS. 2007. Regulation of anxiety during the postpartum period. Front Neuroendocrinol. 28:115–141.
- Malarkey WB, Hall JC, Pearl DK, Kiecolt-Glaser JK, Glaser R. 1991. The influence of academic stress and season on 24-h concentrations of growth hormone and prolactin. J Clin Endocrinol Metab. 73:1089–1092.
- Mathias LJ, Jacobson NA, Rhees RW, Lephart ED. 1999. Brain aromatase in control versus castrated Norway Brown, Sprague-Dawley and Wistar adult rats. Proc Soc Exp Biol Med. 221:126–130.
- Miller SM, Piasecki CC, Peabody MF, Lonstein JS. 2010. GABA(A) receptor antagonism in the ventrocaudal periaqueductal gray increases anxiety in the anxiety-resistant postpartum rat. Pharmacol Biochem Behav. 95:457–465.
- Neill JD. 1970. Effect of “stress” on serum prolactin and luteinizing hormone levels during the estrous cycle of the rat. Endocrinology. 87:1192–1197.
- Neumann ID, Johnstone HA, Hatzinger M, Liebsch G, Shipston M, Russell JA, Landgraf R, Douglas A. 1998. Attenuated neuroendocrine responses to emotional and physical stressors in pregnant rats involve adenohypophysial changes. J Physiol. 508 Pt 1: 289–300.
- Patchev VK, Shoaib M, Holsboer F, Almeida OF. 1994. The neurosteroid tetrahydroprogesterone counteracts corticotropin-releasing hormone-induced anxiety and alters the release and gene expression of corticotropin-releasing hormone in the rat hypothalamus. Neuroscience. 62:265–271.
- Patchev VK, Hassan AH, Holsboer DF, Almeida OF. 1996. The neurosteroid tetrahydroprogesterone attenuates the endocrine response to stress and exerts glucocorticoid-like effects on vasopressin gene transcription in the rat hypothalamus. Neuropsychopharmacology. 15:533–540.
- Poletini MO, Szawka RE, Franci CR, Anselmo-Franci JA. 2006. Ovarian steroids but not the locus coeruleus regulate stress-induced prolactin secretion in female rats. J Neuroendocrinol. 18:938–948.
- Sánchez P, Torres JM, Vílchez P, Del Moral RG, Ortega E. 2008. Effects of sulpiride on prolactin and mRNA levels of steroid 5alpha-reductase isozymes in adult rat brain. Neurochem Res. 33:820–825.
- Sánchez P, Torres JM, Vílchez P, Del Moral RG, Ortega E. 2009. Effects of metoclopramide on mRNA levels of 5alpha-reductase isozymes in rat brain. Neuroreport. 20:93–96.
- Schlein PA, Zarrow MX, Denenberg VH. 1974. The role of prolactin in the depressed or “buffered” adrenocorticosteroid response of the rat. J Endocrinol. 62:93–99.
- Shin SH. 1980. Physiological evidence for the existence of prolactin releasing factor: Stress-induced prolactin secretion is not linked to dopaminergic receptors. Neuroendocrinology. 31:375–379.
- Simian M, Bissell MJ, Barcellos-Hoff MH, Shyamala G. 2009. Estrogen and progesterone receptors have distinct roles in the establishment of the hyperplastic phenotype in PR-A transgenic mice. Breast Cancer Res. 11:R72.
- Snedecor GW, Cochran WG. 1968. Statistical methods. Ames: Iowa State University Press.
- Steimer T, Driscoll P, Schulz PE. 1997. Brain metabolism of progesterone, coping behaviour and emotional reactivity in male rats from two psychogenetically selected lines. J Neuroendocrinol. 9:169–175.
- Stern JM, Goldman L, Levine S. 1973. Pituitary–adrenal responsiveness during lactation in rats. Neuroendocrinology. 12:179–191.
- Taleisnik S, Deis RP. 1964. Influence of cerebral cortex in inhibition of oxytocin release induced by stressful stimuli. Am J Physiol. 207:1394–1398.
- Torner L, Neumann ID. 2002. The brain prolactin system: Involvement in stress response adaptations in lactation. Stress. 5:249–257.
- Torner L, Toschi N, Pohlinger A, Landgraf R, Neumann ID. 2001. Anxiolytic and anti-stress effects of brain prolactin: Improved efficacy of antisense targeting of the prolactin receptor by molecular modeling. J Neurosci. 21:3207–3214.
- Torner L, Toschi N, Nava G, Clapp C, Neumann ID. 2002. Increased hypothalamic expression of prolactin in lactation: Involvement in behavioural and neuroendocrine stress responses. Eur J Neurosci. 15:1381–1389.
- Turgeon JL, Waring DW. 2006. Differential expression and regulation of progesterone receptor isoforms in rat and mouse pituitary cells and LbetaT2 gonadotropes. J Endocrinol. 190:837–846.
- Valdez SR, Penissi AB, Deis RP, Jahn GA. 2007. Hormonal profile and reproductive performance in lactation deficient (OFA hr/hr) and normal (Sprague-Dawley) female rats. Reproduction. 133:827–840.
- Voloschin LM, Tramezzani JH. 1984. Relationship of prolactin release in lactating rats to milk ejection, sleep state, and ultrasonic vocalization by the pups. Endocrinology. 114:618–623.
- Walf AA, Frye CA. 2006. A Review and Update of Mechanisms of Estrogen in the Hippocampus and Amygdala for Anxiety and Depression Behavior. Neuropsychopharmacology. 31:1097–1111.
- Walker CD, Rivest RW, Meaney MJ, Aubert ML. 1989. Differential activation of the pituitary–adrenocortical axis after stress in the rat: Use of two genetically selected lines (Roman low- and high-avoidance rats) as a model. J Endocrinol. 123:477–485.
- Weichert CE. 1979. Lactational reflex recovery in breast-feeding failure. Pediatrics. 63:799–803.
- Windle RJ, Wood S, Shanks N, Perks P, Conde GL, Da Costa AP, Ingram CD, Lightman SL. 1997. Endocrine and behavioural responses to noise stress: Comparison of virgin and lactating female rats during non-disrupted maternal activity. J Neuroendocrinol. 9:407–414.