Abstract
During the juvenile period rodents are particularly sensitive to stressors. Aversive events encountered during this period may have enduring effects that are not evident among animals initially stressed as adults. Interestingly, experiencing stressor during juvenile period was found to elicit a biphasic behavioral pattern over the course of development. During the juvenile period, the expression of several GABAA receptor subunits is subject to elevated plasticity, rendering the GABAergic system sensitive to stressors. In the present investigation, animals were exposed to a juvenile variable stressor regimen (JUV-S) at 27–29 postnatal days (PND): 27 PND—acute swim stress (10 min), 28 PND—elevated platform stress (3 sessions × 30 min each), and 29 PND—restraint (2 h). One hour following the last exposure to stressor or in adulthood (60 PND), anxiety-related behaviors were assessed in a 5-min elevated plus maze test. The western blotting technique was used to evaluate whether the juvenile stress induced behavioral pattern will be accompanied by respective changes in GABAA α1, α2, and α3 protein expression in male rats. Our findings further established that juvenile stressor elicits hyper-reactivity when rats were tested as juveniles, whereas rats exhibited reduced activity and increased anxiety when tested as adults. Additionally, the effects of juvenile stressor on α1, α2, and α3 were more pronounced among juvenile stressed rats that were challenged as adults compared with rats that were only challenged as juveniles. Interestingly, the stress-induced modulation of the subunits was particularly evident in the amygdala, a brain region closely associated with anxiety. Thus, age- and region-specific alterations of the α subunits may contribute to the age-specific behavioral alterations observed following juvenile stress exposure.
Introduction
There has been a resurgence of interest regarding γ-aminobutyric acid (GABA) functioning in relation to major depressive disorder. The view that this transmitter contributes to depressive illness (Emrich et al. Citation1980; Massat et al. Citation2000) has been reinforced by reports indicating that GABAA subunit gene expression differed in brain regions of depressed individuals who died by suicide relative to that evident among individuals who died of other causes (Tunnicliff and Malatynska Citation2003; Merali et al. Citation2004; Choudary et al. Citation2005; Rupprecht et al. Citation2006; Sequeira and Turecki Citation2006; Sanacora and Saricicek Citation2007).
GABA exerts its effects by binding to particular GABAA or GABAB receptors. The structure of the GABAA receptor comprises pentameric protein made up of complexes (comprising subunit proteins) derived from 21 different proteins/genes arranged in a helical column to form the chloride ion channel (Parsian and Cloninger Citation1997; Liberzon et al. Citation2003). Combinations of this gene cassette are expressed in virtually all central nervous system (CNS) neurons and are fundamental in controlling brain excitability (Fritschy et al. Citation2003). These subunit types differ with respect to their sensitivity to GABA and are relevant to the pharmacological differences observed between drugs, such as benzodiazepines (BZDs), which interact with GABAA receptor.
Of the different subunits that make up the GABAA receptor, the α subunits are suggested to be preferentially associated with the induction of certain types of behavior; GABAA receptor α1 subunit is suggested to mediate sedation and anxiolytic effects are mediated by GABAA receptor α2 and maybe GABAA receptor α3 subunits (Rudolph et al. Citation1999; Gulinello et al. Citation2001). In this regard, unique GABAA receptor isoforms in specific brain regions are thought to differentially modulate anxiety (Crestani et al. Citation1999; Menard and Treit Citation1999), although a role for other subunits (e.g. γ2) has also been implicated in this disorder (Mohler et al. Citation1995a, Citation1995b; Sanders and Shekhar Citation1995; Crestani et al. Citation1999; CitationCaldji et al. 2003). Modulation of α subunits in the amygdala following exposure to stress might be particularly important, given that the amygdala is believed to serve as an interface between the environment and effector organs generating behavioral responses associated with anxiety (Da Cunha et al. Citation1992). Interestingly, the possibility exists that the nature of the GABA involvement in anxiety may vary with developmental age, given that marked α subunits changes occur with development. α2, α3, and α5 subunits are predominant during early periods of the rat, whereas α1 is most prominent thereafter (Laurie et al. Citation1992; Poulter et al. Citation1992; Fritschy et al. Citation1994; Paysan et al. Citation1994; Hornung and Fritschy Citation1996; Poulter et al. Citation1999).
Given the strong association between the α subunits of the GABA receptor complex and the development of anxiety, considerable efforts have been devoted to assess the effects on GABA receptors composition of factors that influence or moderate stress, including early life stress experiences. Early life stressors (particularly maternal neglect of pups) were found to influence the adult response to stressors and also to affect GABA receptor expression (CitationCaldji et al. 2003). Focus has been mainly on the postnatal period, but more recently stressful events during the post-weaning, pre-puberty (juvenile) period (Days 27–29 in rats) have been demonstrated to profoundly influence adult stress responses (Avital and Richter-Levin Citation2005; Tsoory et al. Citation2007). Indeed, we found region- and subunit-specific regulation of GABAA receptor expression profile within the limbic system in adult rats that had been stressed as juveniles and emotionally challenged in adulthood (Jacobson-Pick et al. Citation2008). However, it appeared that stress experienced during the juvenile period elicited biphasic behavioral alterations (Jacobson-Pick and Richter-Levin Citation2010). Unlike the behavioral suppression evident when stressed juveniles were tested as adults, stressed juveniles demonstrated increased arousal, characterized by increased levels of activity and more time spent in the “unsafe“ regions of the open field and elevated plus maze when tested as juveniles, soon after the exposure to the stress experience (Jacobson-Pick and Richter-Levin Citation2010).
In light of these behavioral results, together with the fact that the expression of these subunits varies with development, we examined in the present study the immediate and long-term effects of juvenile exposure to a stressor on the expression of α1, α2, and α3 GABAA receptor subunits.
Material and methods
Animals
Male Sprague-Dawley rats, 22 days old on delivery, weighing 35–49 g supplied by Harlan Laboratories, Jerusalem, were maintained on a 12-h light–dark cycle with lights on at 7 a.m.; room temperature 22 ± 2°C, with free access to food and water (Teklad Global Diet 2018S, Harlan Teklad Ltd, Madison, WI, USA). Rats were maintained four per cage (3560 × 18 cm) containing sawdust bedding. All procedures were approved by the Institutional Animal Care Committee and adhered to the guidelines set out in the National Institutes of Health (NIH) Guide for the Care and Use of Laboratory Animals.
General procedure
At the age of 27–29 postnatal days (PND), animals were exposed to a juvenile variable stressor regimen (JUV-S) or were undisturbed. Half the animals in each condition were assessed in an elevated plus maze test on 29 PND (1 h following the last exposure to stress), whereas the remaining rats were not tested and maintained undisturbed. The remaining half of the rats were examined in adulthood (60 PND). Half the rats in each condition were assessed in an elevated plus maze test, whereas the remaining rats were not tested. In effect, this experiment comprised a 2 (juvenile stress × no stress) × 2 (assessed 1 h later or in adulthood, 30 days later) × 2 (plus maze test vs. no test); N = 8–11 per group.
The basolateral amygdala (left and right pooled together) and the whole hippocampus were collected 24 h after the elevated plus maze challenge or at a similar time point for non-stressed rats as described previously (Jacobson-Pick et al. Citation2008; Tsoory et al. Citation2008). Immediately following decapitation, the brain was placed on an ice-cooled glass dish. First, the hippocampus was dissected out. Then a thick (∼1 mm) coronal slice was cut ∼6 mm anterior of the interaural plane (indicated by the rostal end of the cerebellum), and the basolateral amygdala was cut out: (1) cutting the ventral part of the slice just below the base of the optic tract, (2) cutting orthogonally to the first cut and in parallel to the corpus callosum about 2 mm medial to the rhinal fissure, and (3) cutting out a small isosceles (∼1.5 mm) triangle.
Juvenile stressor paradigm
The juvenile stressor procedure comprised exposure to a different stressor on each of three consecutive days. On PND 27, rats were individually placed in a circular water tank (diameter 0.5 m, height 0.5 m, and water depth 0.4 m) for 10 min (water temperature 22 ± 2°C). Immediately after the swim stress terminated, rats were returned to their home cage to dry off. On PND 28, rats were placed individually on a small 12 × 12 cm elevated platform, 70 cm above floor level, located in the middle of a small room for three sessions of 30 min each, separated by 60-min intervals in the home cage. Finally on PND 29, rats were placed in a metal mesh restraining box (11 × 5 × 4 cm) that prevented forward–backward movement and limited side-to-side mobility, but did not otherwise discomfort the animal. Rats remained in the restraining box for 2 h at 25 ± 2°C under dim illumination. All rats were stressed between 11:00 and 17:00 h by the same experimenter. Following completion of the stressor procedure on the third day, rats were returned to their home cage and were not handled until the behavioral tests, except for weekly cage maintenance.
Elevated plus maze test
The elevated plus maze test was conducted as previously described (Pellow et al. Citation1985). Briefly, the plus-shaped maze was elevated 50 cm from the floor and consisted of two open arms and two closed arms (the latter had 30-cm high Plexiglas walls and no roof). At the time of testing, each animal was placed in the center of the maze facing an open arm and allowed to explore for a 5-min session while their behavior was videotaped. Each of the recorded sessions was later analyzed by “blind” experimenters, who charted the exploration course of the rats and measured the time spent in the open arena of the apparatus. The percentage of time spent in the open arms represented primary index of anxiety. In addition, each arm of the plus maze was demarcated by sections (each arm containing five equivalent sized sections) and the number of sections crossed (which correlated with the frequency of arm entries) provided an index of anxiety and of gross motor activity. These measures were also collected for the closed arms to determine whether behaviors were broadly affected or were unique to the anxiety-provoking open arms. All rats were tested between 11:00 and 17:00 h by the same experimenter.
Western blot analysis
Brains of rats were removed 24 h subsequent to the anxiety tests. The hippocampus and amygdala were immediately dissected and homogenized in a glass Teflon homogenizer in 300 μl (amygdala) or 600 μl (hippocampus) lysis buffer (10 mM HEPES, 2 mM EDTA, 2 mM EGTA, 0.5 mM DTT, 10 μl/ml leupeptin, and 10 μl/ml aprotinin). After the protein levels in each sample were determined using the Bradford assay (Bio-Rad protein dye reagent; Bio-Rad, Hercules, CA, USA), they were diluted in SDS sample buffer (10% glycerol, 5% β-mercaptoethanol, and 2.3% SDS in 62.5 mM Tris–HCl, pH 6.8), and boiled (100°C) for 5 min. Samples were then stored at − 80°C until further analysis.
Aliquots in SDS sample buffer were subjected to SDS-PAGE (10% polyacrylamide) and immunoblot analysis. Each lane was loaded with equal amount of protein sample as determined by Bradford assay (Bio-Rad protein dye reagent; Bio-Rad, Hercules, CA, USA).
Following blotting to a nitrocellulose membrane, lysate homogeneity lanes were stained by Ponceau staining. Blots were blocked with 5% BSA+5% Blotto solution (Chemicon, Temecula, CA, USA) for 1 h at room temperature, followed by 1 h incubation with the primary antibody at room temperature. Following three short washes in TBST washing buffer (0.9% w/v NaCl, 0.05% v/v Tween-20, and 100 mM Tris–HCl, pH 7.6), the blots were subsequently incubated for 1 h with a horseradish peroxidase (HRP)-linked secondary antibody at room temperature before reacted with enhanced chemiluminescense (ECL+) substrate (Amersham, Piscataway, NJ).
Reagents
Antibodies. GABAA receptor α1 subunit Cat. # AGA-001 (1:200) rabbit polyclonal (Alomone Labs, Jerusalem, Israel). GABAA receptor α2 subunit Cat. # AGA-002 (1:200) rabbit polyclonal (Alomone Labs). GABAA receptor α3 subunit Cat. # AGA-003 (1:200) rabbit polyclonal (Alomone Labs). Actin (N-19) Cat. # sc-1616 (1:1500) polyclonal goat antibody (Santa Cruz Biotechnology, Santa Cruz, CA, USA). Rabbit anti-goat (IgG) HRP conjugated (Santa Cruz Biotechnology). Goat anti-rabbit (IgG) HRP conjugated and the ECL+ kit was obtained from Amersham. All other chemicals were of analytical grade or the highest grade available.
Quantification
Quantification was performed using a CCD camera (XRS Biorad, Hercules, CA, USA). The expression level for each depicted protein was calculated as the ratio between the band intensity of the protein divided by that of the normalized actin, a cytoskeletal protein was used as an internal control. No differences were detected in actin levels between the different groups.
Statistical methods
Results are expressed as means ± SEM. The behavioral measures in the elevated plus maze were assessed by a 2 (juvenile stressor or no stress) × 2 (age: juvenile vs. adult) between groups analysis of variance (ANOVA). Comparisons of means comprising significant interactions were conducted through Tukey's “Honestly Significant Difference” (HSD) post-hoc tests. As the GABAA receptor α subunits of juvenile and adult animals were analyzed against different baselines, their protein expressions were assessed individually through ANOVA.
Results
Immediate effects of juvenile variable stress on exploratory and anxiety indices
shows performance in the plus maze as a function of the treatments rats received as well as their age of testing. The analysis of the time spent in the open arms and section crossings in the open arms revealed significant stressor condition × age of testing interactions, F(1,36) = 46.82 and 34.90, ps < 0.001. The follow-up tests revealed that the number of section crossings in the open arms among non-stressed adult animals exceeded that of non-stressed young rats. Among the adults, the juvenile stressor experience reduced the number of section crossings in the open arms and time in the open arms, whereas among rats tested in juvenility the same stressor resulted in precisely the opposite effect.
Figure 1. Short- and long-term effects of juvenile variable stressor regimen (JUV-S) on exploratory and anxiety behavior in an elevated plus maze. Data were analyzed through a 2 (juvenile stressor vs. no stress) × 2 (age: juvenile vs. adult) between-groups ANOVA followed by HSD Tukey's post-hoc tests. Data are expressed as means ± SEM (n = 8–11/group). Compared with controls, JUV-S rats (A) spent more time in the open arms of the elevated plus maze when tested in juvenility and less time in the open arms of the apparatus when tested in adulthood, (B) were more active in the open arms when tested in juvenility and less active when tested in adulthood, (C) spent less time in the closed arms when tested in juvenility but no differences were observed when tested in adulthood, and (D) were more active in the closed arms when tested in juvenility but these effects were absent when rats were tested as adults; *, represents significantly different from controls, p < 0.05; •, represents significantly different from the same group juveniles, p < 0.05.
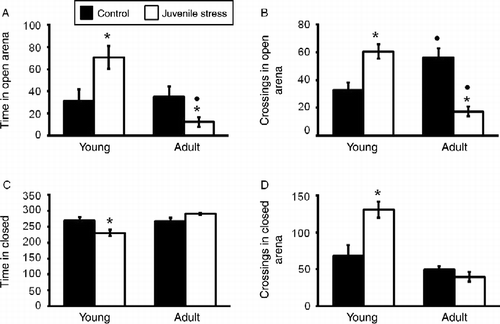
The time spent in the closed arms also varied as a function of the juvenile stressor treatment × age of test interaction, F(1,33) = 11.39, p < 0.001, as did the number of section crossings in the closed arms, F(1,33) = 13.32, p < 0.001. In both instances, when tested soon after the exposure to the juvenile stress, rats spent less time in the closed arms, but were more active when they were in that arm than did animals that were not stressed. However, these effects were absent when rats were tested as adults, indicating that the primary effect on adult behavior in rats that had been stressed as juveniles was evident in relation to their entries to the open arms of the maze.
Immediate- and long-term effects of juvenile variable stress on GABAA receptor α subunit expression in the hippocampus
shows the α1, α2, and α3 subunit expression in the hippocampus of juvenile rats 24 h following the exposure to the juvenile stress, as a function of their exposure to stress and the plus maze experience. Neither the juvenile stressor nor the plus maze experience influenced hippocampal α1 or α2 subunits, whereas α3 subunit expression varied as a function of the juvenile stress × plus maze experience interaction, F(1,28) = 8.68, p < 0.01. Follow-up comparisons revealed that α3 expression was elevated in rats that had been stressed as juveniles and then tested in the plus maze relative to rats that received either of these treatments alone.
Figure 2. The short-term effects of juvenile variable stressor regimen (JUV-S) on GABAA receptor α subunit expression in the hippocampus. Data were analyzed through a 2 (juvenile stressor vs. no stress) × 2 (age: juvenile vs. adult) between-groups ANOVA followed by HSD Tukey's post-hoc tests. Data are expressed as means ± SEM (n = 8–11/group). Neither the juvenile stressor nor the plus maze experience influenced hippocampal α1 or α2 subunits, whereas α3 subunit expression was elevated in rats that had been stressed as juveniles and then tested in the plus maze relative to control and rats that received either of these treatments alone; *, represents significantly different from controls, p < 0.05; (C) #, represents significantly different from elevated plus maze challenge group C (+), p < 0.05; ^, represents significantly different from juvenile stress group (J), p < 0.05.
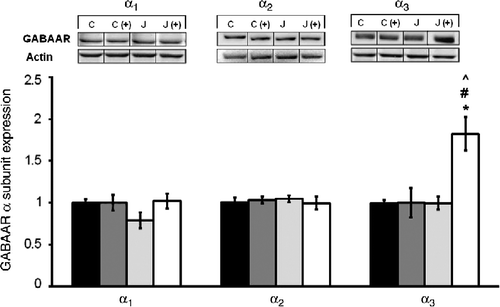
GABAA subunit expression assessed among adult rats was very different from that evident in juveniles (). The juvenile stress experience decreased hippocampal α1 expression when measured in adulthood, F(1,28) = 7.57, p < 0.01. Whereas α2 expression within the hippocampus varied as a function of the juvenile stressor × plus maze test interaction, F(1,28) = 10.98, p < 0.001. Follow-up tests for this interaction indicated that in rats that received both treatments (juvenile stressor and adult plus maze exposure), the expression of the α2 subunit was elevated relative to rats that had received either the juvenile stressor or the plus maze exposure alone. Finally, expression of the α3 subunit in the hippocampus was affected by neither of the treatments nor their interaction.
Figure 3. The long-term effects of juvenile variable stressor regimen (JUV-S) on GABAA receptor α subunit expression in the hippocampus. Data were analyzed through a 2 (juvenile stressor vs. no stress) × 2 (age: juvenile vs. adult) between-groups ANOVA followed by HSD Tukey's post-hoc tests. Data are expressed as means ± SEM (n = 8–11/group). GABAA subunit expression assessed among adult rats was very different from that evident in juveniles. In rats that received juvenile stressor and adult plus maze exposure, the expression of the α1 subunit was decreased compared to controls, α2 subunit was elevated relative to rats that had received either the juvenile stressor or the plus maze exposure alone. Finally, expression of the α3 subunit was neither affected by either of the treatments nor their interaction; *, represents significantly different from controls (C), p < 0.05; #, represents significantly different from elevated plus maze challenge group C (+), p < 0.05; ^, represents significantly different from juvenile stress group (J), p < 0.05.
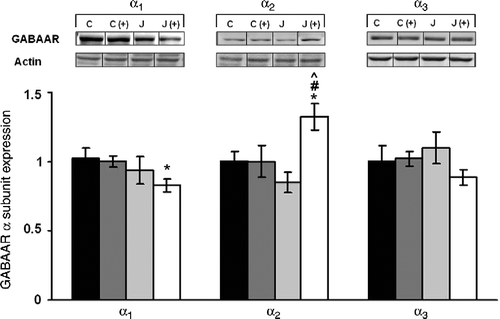
Immediate- and long-term effects of juvenile variable stress on GABAA receptor α subunit expression in the amygdala
Analyses of the amygdala in juvenile animals indicated that neither α1 nor α3 expression was affected by either of the treatments, whereas α2 expression was lower in those stressed juvenile animals relative to non-stressed rats, F(1,28) = 4.65, p < 0.05 (). In contrast, appreciably more dramatic and opposite effects on amygdala subunit expression were apparent in rats tested in adulthood (). Specifically, the expression of the α1 subunit was reduced among rats that had been exposed to a juvenile stressor, F(1,28) = 6.06, p < 0.05, and the adult stressor experience (placement on a plus maze) had a similar effect. Furthermore, both the α2 and α3 subunit expression varied as a function of the interaction between the juvenile stressor experience and that experienced as an adult, F(1,28) = 7.60 and 27.38, ps < 0.01. Follow-up tests indicated that neither the juvenile stress experience nor the plus maze test alone elicited a change in the expression of these receptor subunits. However, in rats that had initially been stressed as juveniles and then exposed to the adult stressor, the levels of these two subunits were significantly increased.
Figure 4. The short-term effects of juvenile variable stressor regimen (JUV-S) on GABAA receptor α subunit expression in the amygdala. Data were analyzed through a 2 (juvenile stressor vs. no stress) × 2 (age: juvenile vs. adult) between-groups ANOVA followed by HSD Tukey's post-hoc tests. Data are expressed as means ± SEM (n = 8–11/group). Among rats that were tested as juveniles, neither the juvenile stressor nor the plus maze test affected either α1 or α3 subunit expression within the amygdala, whereas the juvenile stressor experience reduced α2 to a moderate, but significant extent; *, represents significantly different from controls (C), p < 0.05.
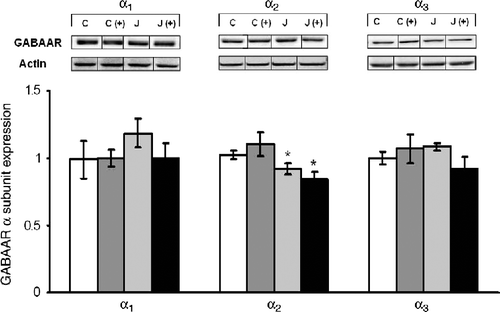
Figure 5. The long-term effects of juvenile variable stressor regimen (JUV-S) on GABAA receptor α subunit expression in the amygdala. Data were analyzed through a 2 (juvenile stressor vs. no stress) × 2 (age: juvenile vs. adult) between-groups ANOVA followed by HSD Tukey's post-hoc tests. Data are expressed as means ± SEM (n = 8–11/group). More dramatic effects on amygdala subunit expression were apparent in rats tested in adulthood. Among rats that had initially been stressed as juveniles and then exposed to the adult stressor, the levels of α2 and α3 were significantly increased while those of α1 were decreased. Nevertheless, neither the juvenile stressor experience nor the plus maze test alone elicited a change of these receptor subunits; *, represents significantly different from controls (C), p < 0.05; #, represents significantly different from elevated plus maze challenge group C (+), p < 0.05; ^, represents significantly different from juvenile stress group (J), p < 0.05.
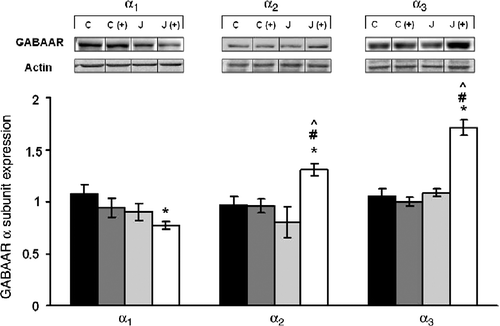
Discussion
It has been reported that the juvenile period is one in which rodents are especially sensitive to stressors (Doremus et al. Citation2003; Spear Citation2009). Aversive events encountered during this period may have protracted effects on behavior which are not evident among animals initially stressed as adults (Avital and Richter-Levin Citation2005; Tsoory et al. Citation2007).
Interestingly, in response to juvenile stressors, a biphasic behavioral pattern was reported over the course of development. Juvenile stress was found to elicit hyper-reactivity or impulsivity when rats were tested soon after the stress experience, whereas rats revealed reduced activity and increased anxiety when tested as adults (Jacobson-Pick and Richter-Levin Citation2010). The same biphasic behavioral pattern was observed in the present investigation. Adult rats that had been exposed to a juvenile stressor displayed decreased levels of activity and spent less time in the “unsafe” regions of the elevated plus maze than rats that had not been stressed previously. In contrast, rats that were tested soon after the exposure to the juvenile stressor exhibited increased levels of activity and spent more time in the “unsafe” regions of the elevated plus maze than rats that had not been stressed.
These differential effects of the stressor between juveniles and adults are reminiscent of findings from clinical studies showing that although children might experience anxiety and depression in much the same way as adults do, children display and react to those symptoms differently (Bostic et al. Citation2005). For example, a child with major depression may display irritable mood and anxiety rather than overt depression as adults do (Volkmar Citation2002).
We propose here that the differential behavioral effects of the exposure to stress are attributable to the age at which the animals were tested. However, the possibility that the differential outcomes stemmed from the differential passage of time between the two treatments (i.e. 1 h in rats tested as juveniles vs. 30 days for rats tested as adults) should be considered. It has been shown that the apparent impulsivity evident 1 h after juvenile stress was not a simple result of arousal associated with the very brief post-stress period at which rats were tested, since a similar outcome was apparent when juveniles were tested 6 h after the exposure to the stressor (Jacobson-Pick and Richter-Levin Citation2010). It has been reported that a stressful experience may elicit behavioral and neurochemical effects that intensify with the passage of time. For example, the expression of arginine vasopressin (AVP) within corticotropin-releasing hormone (CRH) terminals located in the external zone of the median eminence was markedly greater 2–4 weeks than 1 day after a stressor (Bartanusz et al. Citation1993; Schmidt et al. Citation1996; Tilders and Schmidt Citation1999). As AVP and CRH synergistically increase pituitary Adrenocorticotropic hormone (ACTH) release, it was suggested that this might account for the progressive increase of anxiety-like behaviors associated with the stressor. Whether the co-expression of these peptides varies as a function of the age at which animals were stressed is yet to be studied.
In view of the relationship between anxiety and GABA functioning (Mohler et al. Citation1995a, Citation1995b; Sanders and Shekhar Citation1995; Crestani et al. Citation1999; CitationCaldji et al. 2003), it had been of interest to determine the effects of juvenile stressors on later variations of GABAA subunit expression. In this regard, it had previously been reported that exposing juvenile rats to a stressor influenced GABA receptor subunit expression upon subsequent stressor exposure (Jacobson-Pick et al. Citation2008). The present investigation, however, indicated that the nature of the effects observed varied with the age (i.e. as a juvenile or as an adult) at which rats were subsequently assessed and also as a function of whether rats were again exposed to a stressor. Specifically, a juvenile stress experience reduced amygdala α2 subunit expression during the juvenile period, an effect that was not apparent when animals were tested in adulthood. As well, hippocampal α3 was increased if rats had been exposed to the juvenile stressor and then again exposed to a stressor during the juvenile period. This effect, being uniquely evident with respect to α3 expression in the hippocampus, was unexpected but was a reliable effect, being evident in successive replications of the study.
The profile of subunit responses to the stressors was very different in adult animals relative to those apparent in juveniles. Specifically, in adults that had been exposed to the juvenile stressor, α1 expression was reduced in both the hippocampus and amygdala, attesting to the long-term influence of the juvenile experience. In contrast, the juvenile stress experience did not have direct effects on α2 or α3 expression in the hippocampus or amygdala. However, among rats that had initially encountered the juvenile stressor, later exposure to a moderate stressor (placement in the elevated plus maze) during adulthood markedly increased α2 and α3 expression in the amygdala and α2 expression in the hippocampus. In effect, the juvenile stressor may have established a form of long-term meta-plasticity, so that the subunit response to later exposure to a stressor was altered.
Behavioral alterations associated with variations of GABAA subunit expression have been observed in other studies. For example, Poulter et al. (Citation2010) have shown that GABAA receptor α2 subunit was increased among stress-reactive mice compared with stress-resilient mice (Poulter et al. Citation2010). Pinna et al. (Citation2006) have demonstrated altered locomotor activity and alteration in sensitivity to BZDs in mice subjected to protracted social isolation (Pinna et al. Citation2006).
The fact that a sensitization effect was apparent when previously stressed rats were tested as adults may reflect the high level of stressor sensitivity associated with the juvenile period (Tsoory et al. Citation2007). Alternatively, as described earlier regarding the sensitization of corticosterone responses (Bartanusz et al. Citation1993; Schmidt et al. Citation1996; Tilders and Schmidt Citation1999), it is possible that passage of time was necessary for a sensitized response to develop.
It will be recalled that the α2 and α3 subunits predominate in young animals and the α1 subunit is more predominant afterward (Laurie et al. Citation1992; Poulter et al. Citation1992). Although these initial reports essentially suggested that these developmental changes were complete by PND 30, it was subsequently shown that the α3 and α5 subunits continuously decreased in the adult and aging brain (Yu et al. Citation2006). Moreover, α1 subunits that were only expressed to a limited extent in the early postnatal brain progressively increased with age, stabilizing in the adult and aging brain (Yu et al. Citation2006). Albeit speculative, these developmental differences in the expression of the subunits raise the possibility that the differential sensitization responses might be linked to these subunits expression during development. Thus, the presence of α2 and α3 subunits during early development might make them more amenable to becoming sensitized by environmental triggers, such as stressors, whereas the relative paucity of α1 subunits at this age might limit the sensitization of this subunit.
The mechanisms responsible for this outcome are uncertain, but the results suggest that altered GABAA receptor α subunits expression contributes to the underlying mechanism of the biphasic age-dependent behavioral alterations observed following juvenile stress exposure.
Acknowledgments
We thank Prof. Hymie Anisman for valuable comments on this paper.
Declaration of interest: This work was funded by a Deutsche Israel Project cooperation grant to G.R-L and by a grant from the Institute for the Study of Affective Neuroscience, University of Haifa, which was endowed by the Hope for Depression Research Foundation, to G.R-L. The authors report no conflicts of interest. The authors alone are responsible for the content and writing of the paper.
References
- Avital A, Richter-Levin G. 2005. Exposure to juvenile stress exacerbates the behavioural consequences of exposure to stress in the adult rat. Int J Neuropsychopharmacol. 8:163–173.
- Bartanusz V, Jezova D, Bertini LT, Tilders FJ, Aubry JM, Kiss JZ. 1993. Stress-induced increase in vasopressin and corticotropin-releasing factor expression in hypophysiotrophic paraventricular neurons. Endocrinology. 132:895–902.
- Bostic JQ, Rubin DH, Prince J, Schlozman S. 2005. Treatment of depression in children and adolescents. J Psychiatr Pract. 11:141–154.
- Caldji C, Diorio J, Meaney MJ. 1959. Variations in maternal care alter GABA(A) receptor subunit expression in brain regions associated with fear. Neuropsychopharmacology. 28 11: 1950–1950.
- Choudary PV, Molnar M, Evans SJ, Tomita H, Li JZ, Vawter MP, Myers RM, Bunney WEJr, Akil H, Watson SJ, Jones EG. 2005. Altered cortical glutamatergic and GABAergic signal transmission with glial involvement in depression. Proc Natl Acad Sci USA. 102 43: 15653–15658.
- Crestani F, Lorez M, Baer K, Essrich C, Benke D, Laurent JP, Belzung C, Fritschy JM, Luscher B, Mohler H. 1999. Decreased GABAA-receptor clustering results in enhanced anxiety and a bias for threat cues. Nat Neurosci. 2:833–839.
- Da Cunha C, Wolfman C, Levi de Stein M, Ruschel AC, Izquierdo I, Medina JH. 1992. Anxiogenic effects of the intraamygdala injection of flumazenil, a benzodiazepine receptor antagonist. Funct Neurol. 7:401–405.
- Doremus TL, Brunell SC, Varlinskaya EI, Spear LP. 2003. Anxiogenic effects during withdrawal from acute ethanol in adolescent and adult rats. Pharmacol Biochem Behav. 75:411–418.
- Emrich HM, Zerssen DV, Kissling W, Möller HJ, Windorfer A. 1980. Effect of sodium valproate on mania: The GABA-hypothesis of affective disorders. Arch Psychiatr Nervenkr. 229:1–16.
- Fritschy JM, Paysan J, Enna A, Mohler H. 1994. Switch in the expression of rat GABAA-receptor subtypes during postnatal development: An immunohistochemical study. J Neurosci. 14:5302–5324.
- Fritschy JM, Schweizer C, Brünig I, Lüscher B. 2003. Pre- and post-synaptic mechanisms regulating the clustering of type A gamma-aminobutyric acid receptors (GABAA receptors). Biochem Soc Trans. 31 Pt 4: 889–892.
- Gulinello M, Gong QH, Li X, Smith SS. 2001. Short-term exposure to a neuroactive steroid increases alpha4 GABA(A) receptor subunit levels in association with increased anxiety in the female rat. Brain Res. 910:55–66.
- Hornung JP, Fritschy JM. 1996. Developmental profile of GABAA-receptors in the marmoset monkey: expression of distinct subtypes in pre- and postnatal brain. J Comp Neurol. 367:413–430.
- Jacobson-Pick S, Elkobi A, Vander S, Rosenblum K, Richter-Levin G. 2008. Juvenile stress-induced alteration of maturation of the GABAA receptor alpha subunit in the rat. Int J Neuropsychopharmacol. 11 7: 891–903.
- Jacobson-Pick S, Richter-Levin G. 2010. Differential impact of juvenile stress and corticosterone in juvenility and in adulthood, in male and female rats. Behav Brain Res. 214 2: 268–276.
- Laurie DJ, Wisden W, Seeburg PH. 1992. The distribution of thirteen GABAA receptor subunit mRNAs in the rat brain. III. Embryonic and postnatal development. J Neurosci. 12:4151–4172.
- Liberzon I, Britton JC, Phan KL. 2003. Neural correlates of traumatic recall in posttraumatic stress disorder. Stress. 6:151–156.
- Massat I, Souery D, Papadimitriou GN, Mendlewicz J. 2000. The GABAergic hypothesis of mood disorders. In: Soares JC, Gershon S. editors. Bipolar disorders. New York: Marcel Dekker143–165.
- Menard J, Treit D. 1999. Effects of centrally administered anxiolytic compounds in animal models of anxiety. Neurosci Biobehav Rev. 23:591–613.
- Merali Z, Du L, Hrdina P, Palkovits M, Faludi G, Poulter MO, Anisman H. 2004. Dysregulation in the suicide brain: mRNA expression of corticotropin-releasing hormone receptors and GABA(A) receptor subunits in frontal cortical brain region. J Neurosci. 24 6: 1478–1485.
- Mohler H, Benke D, Benson J, Luscher B, Fritschy JM. GABAA-receptor subtypes in vivo: Cellular localization, pharmacology and regulation. Adv Biochem Psychopharmacol. 1995a; 48:41–56.
- Mohler H, Knoflach F, Paysan J, Motejlek K, Benke D, Luscher B, Fritschy JM. Heterogeneity of GABAA-receptors: Cell-specific expression, pharmacology, and regulation. Neurochem Res. 1995b; 20:631–636.
- Parsian A, Cloninger CR. 1997. Human GABAA receptor alpha 1 and alpha 3 subunits genes and alcoholism. Alcohol Clin Exp Res. 21:430–433.
- Paysan J, Bolz J, Mohler H, Fritschy JM. 1994. GABAA receptor alpha 1 subunit, an early marker for area specification in developing rat cerebral cortex. J Comp Neurol. 350:133–149.
- Pellow S, Chopin P, File SE, Briley M.. 1985. Validation of open: closed arm entries in an elevated plus-maze as a measure of anxiety in the rat.. J Neurosci Methods. 14:149–167.
- Pinna G, Agis-Balboa RC, Zhubi A, Matsumoto K, Grayson DR, Costa E, Guidotti A. 2006. Imidazeni and diazepam increase locomotor activity in mice exposed to protracted social isolation. Proc Natl Acad Sci USA. 103 11: 4275–4280.
- Poulter MO, Barker JL, O'Carroll AM, Lolait SJ, Mahan LC. 1992. Differential and transient expression of GABAA receptor alpha-subunit mRNAs in the developing rat CNS. J Neurosci. 12:2888–2900.
- Poulter MO, Brown LA, Tynan S, Willick G, William R, McIntyre DC. 1999. Differential expression of alpha1, alpha2, alpha3, and alpha5 GABAA receptor subunits in seizure-prone and seizure-resistant rat models of temporal lobe epilepsy. J Neurosci. 19:4654–4661.
- Poulter MO, Du L, Zhurov V, Merali Z, Anisman H. 2010. Plasticity of the GABA(A) receptor subunit cassette in response to stressors in reactive versus resilient mice. Neuroscience. 165 4: 1039–1051.
- Rudolph U, Crestani F, Benke D, Brunig I, Benson JA, Fritschy JM, Martin JR, Bluethmann H, Mohler H. 1999. Benzodiazepine actions mediated by specific gamma-aminobutyric acid(A) receptor subtypes. Nature. 401:796–800.
- Rupprecht R, Eser D, Zwanzger P, Moller HJ. 2006. GABAA receptors as targets for novel anxiolytic drugs. World J Biol Psychiatry. 7:231–237.
- Sanacora G, Saricicek A. 2007. GABAergic contributions to the pathophysiology of depression and the mechanism of antidepressant action. CNS Neurol Disord Drug Targets. 6:127–140.
- Sanders SK, Shekhar A. 1995. Regulation of anxiety by GABAA receptors in the rat amygdala. Pharmacol Biochem Behav. 52:701–706.
- Schmidt ED, Binnekade R, Janszen AW, Tlders FJ. 1996. Short stressor induced long-lasting increases of vasopressin stores in hypothalamic corticotropin-releasing hormone (CRH) neurons in adult rats. J Neuroendocrinol. 8:703–712.
- Sequeira A, Turecki G. 2006. Genome wide gene expression studies in mood disorders. OMICS. 10:444–454.
- Spear LP. 2009. Heightened stress responsivity and emotional reactivity during pubertal maturation: Implications for psychopathology. Dev Psychopathol. 21 1: 87–97.
- Tilders FJH, Schmidt ED. 1999. Cross-sensitization between immune and non-immune stressors. A role in the etiology of depression?. Adv Exp Med Biol. 461:179–197.
- Tsoory M, Cohen H, Richter-Levin G. 2007. Juvenile stress induces a predisposition to either anxiety or depressive-like symptoms following stress in adulthood. Eur Neuropsychopharmacol. 17 4: 245–256.
- Tsoory MM, Vouimba RM, Akirav I, Kavushansky A, Avital A, Richter-Levin G. 2008. Amygdala modulation of memory-related processes in the hippocampus: Potential relevance to PTSD. Prog Brain Res. 167:35–51.
- Tunnicliff G, Malatynska E. 2003. Central GABAergic systems and depressive illness. Neurochem Res. 28:965–976.
- Volkmar FR. 2002. Changing perspectives on mood disorders in children. Am J Psychiatry. 159:893–894.
- Yu ZY, Wang W, Fritschy JM, Witte OW, Redecker C. 2006. Changes in neocortical and hippocampal GABAA receptor subunit distribution during brain maturation and aging. Brain Res. 1099:73–81.