Abstract
Seizure causes autonomic, neuroendocrine and stress responses. We examined the effects of kainic acid (KA)-induced seizures on the expression of the arginine vasopressin (AVP)-enhanced green fluorescent protein (eGFP) in the locus coeruleus (LC), an area known to contain noradrenergic cells, in AVP-eGFP transgenic male and female rats, with the rationale to identify stressors which induce AVP synthesis in the LC. Subcutaneous (s.c.) administration of KA caused a progressive development of seizure behavior within 24 h. AVP-eGFP fluorescence in the LC was detected 6, 24, and 48 h and 1 week after administration of KA (12 mg/kg). From a nearly undetectable level, it reached a maximum at 48 h after s.c. administration of KA and returned to the basal levels after 2 weeks. AVP-eGFP fluorescence in the LC after s.c. administration of KA was significantly reduced by the pretreatment with MK-801 (nonselective N-methyl-d-aspartate (NMDA) receptor antagonist). In the KA-administered rats, immunohistochemistry for tyrosine hydroxylase (TH) revealed that the eGFP fluorescence was co-localized with TH-immuno-reactivity in the LC. These results suggest that the synthesis of AVP-eGFP is potentially up-regulated in noradrenergic neurons in the LC after KA-induced seizures through the activation of NMDA receptors.
Introduction
The neuropeptide arginine vasopressin (AVP) is known to act as a neurotransmitter/neuromodulator in the central nervous system (CNS) as well as a systemic anti-diuretic hormone at the level of the kidney. AVP is synthesized not only in the supraoptic and paraventricular nuclei (PVN) of the hypothalamus but also in extra-hypothalamic areas. In the latter context, the use of the AVP-enhanced green fluorescent protein (eGFP) transgenic rat (Ueta et al. Citation2005), which expresses an AVP-eGFP fusion gene under the control of AVP regulatory sequences, has greatly facilitated the description of extra-hypothalamic vasopressinergic brain regions. Thus, novel AVP-expressing neuronal groups have been described in the olfactory bulb (Tobin et al. 2010) and the locus coeruleus (LC; Todoroki et al. Citation2010). The LC, the largest group of noradrenergic neurons in the CNS, projects extensively to the widespread areas of the brain. Exposure to stress is associated with an enhanced LC activity and with an increased noradrenaline release (Caffe et al. Citation1985; Samuels and Szabadi Citation2008a,Citationb).
However, the stressors which induce AVP synthesis in the LC remain unknown. Todoroki et al. (Citation2010) examined the effect of several types of stressors on the expression of the AVP-eGFP fusion gene in the LC of transgenic rats (Todoroki et al. Citation2010). Sleep deprivation, noxious, and inflammatory stimuli (peripheral formalin injection), as well as inescapable restraint stress had no effect. However, the i.c.v. administration of colchicine induced AVP synthesis in the LC. Colchicine treatment is known to block axonal transport, resulting in peptide accumulation in the cell body, but it remained unclear whether colchicine acts not only as a blocker of axonal transport but also as a stressor.
In order to identify other stressors which induce AVP synthesis in the LC in a physiological context, we examined the consequences of subcutaneous (s.c.) administration of kainic acid (KA), an excitotoxic analog of glutamate, which is widely used in the study of seizure (Collins et al. Citation1980; Ben-Ari et al. Citation1982), on the expression of the AVP-eGFP transgene in the LC. Seizures are known to activate the hypothalamic–pituitary–adrenal (HPA) axis, causing autonomic, neuroendocrine, and stress responses, as well as intensive recurrent neuronal discharge and neurochemical changes (Gallagher et al. Citation1984; Piekut and Phipps Citation1998; Sakamoto et al. Citation2008). It has also been reported that KA-induced seizures induced the expression of the pituitary adenylate cyclase-activating polypeptide gene in the medial parvocellular part of the PVN of rats (Nomura et al. Citation2000). Furthermore, in the LC, several kinds of seizures induced the expression of Fos immunoreactivity (Silveira et al. Citation1998a,Citationb), elevation of tyrosine hydroxylase (TH) and norepinephrine transporter mRNAs (Bengzon et al. Citation1999), and increased concentrations of noradrenaline, dopamine, and serotonin (Shouse et al. Citation2001). These results indicate that noradrenergic neurons in the LC are activated by seizure. Therefore, we hypothesized that seizure may induce the expression of the AVP gene in the LC.
Thus, in the present study, we examined the effects of s.c. administration of KA on AVP-eGFP fluorescence in the LC and in other brain areas that contain noradrenergic cells.
Materials and methods
Animals
Adult male and female AVP-eGFP, Wistar transgenic rats weighing 150–380 g were bred and maintained as described previously (Ueta et al. Citation2005). Rats were housed under standard laboratory conditions (12:12 h light/dark cycle, lights on 07.00–19.00 h) with food and drinking water available ad libitum. All experiments were performed in strict accordance with the guidelines set out by the Physiological Society of Japan and approved by the Ethics Committee of Animal Care and Experimentation, University of Occupational and Environmental Health, Japan. All rats were genotyped by polymerase chain reaction (PCR) analysis of genomic DNA extracted from rat ear biopsies before use (Ueta et al. Citation2005). The PCR was performed using the oligonucleotide primers (sense sequence, 5′-CAC CAT CTT CTT CAA GGA CGA C-3′; antisense sequence, 5′-ATG ATA TAG ACG TTG TGG CTG TTG T-3′). Males and females were initially analyzed separately, but as there was no statistical difference between the males and females in regard to AVP-eGFP fluorescence in the LC, future experiments have included both genders.
KA-induced seizures
KA and MK-801 were purchased from Nacalai Tesque, Inc. (Kyoto, Japan) and Tocris Cookson Ltd. (Ballwin, MO, USA), respectively. Rats were injected s.c. with KA dissolved in 0.9% (w/v) saline (12 ml/kg body weight, 4 mg/kg body weight). Seizures were rated hourly according to a previously defined scale (Stages 1–6; Racine Citation1972; Schauwecker and Steward Citation1997): Stage 1, immobility; Stage 2, forelimb and/or tail extension, rigid posture; Stage 3, repetitive movements, head bobbing; Stage 4, rearing and falling; Stage 5, continuous rearing and falling; and Stage 6, severe tonic–clonic seizures. Rats that exhibited seizure scale from Stages 3 to 6 were perfused 3 h (n = 4), 6 h (n = 5), 24 h (n = 5), 48 h (n = 5), 1 week (n = 6), or 2 weeks (n = 4) after s.c. administration of KA between 13:00 and 14:00. Four rats were s.c. injected with corresponding amounts of 0.9% (w/v) saline and used as controls.
Pretreatment with MK-801
Rats were injected s.c. with (i) MK-801 (1 mg/kg; n = 5) 5 min before administration of KA (12 mg/kg s.c.), (ii) MK-801 (1 mg/kg; n = 3) 5 min before administration of vehicle (0.9% w/v saline s.c.), or (iii) vehicle (0.9% saline) 5 min before administration of KA (12 mg/kg s.c.; n = 7) or (iv) two injections of vehicle (0.9% w/v saline s.c.; n = 3). Rats were perfused 24 h after the last injection between 13.00 and 14.00 h.
Fluorescent microscopic observation after s.c. administration of KA and vehicle
After s.c. administration of KA or vehicle, the rats were terminally anaesthetized by the administration of sodium pentobarbital (50 mg/kg body weight i.p.), and were perfused transcardially with 100 ml of 0.1 M phosphate buffer (PB; pH 7.4) containing heparin (1000 U/l) followed by 150 ml of 4% (w/v) paraformaldehyde in 0.1 M PB. The brains and adrenal glands were removed, the brain was cut coronally into three blocks (forebrain, hypothalamus, and brainstem). These tissues were then post-fixed with the same fixative for 48 h at 4°C, and then immersed in 0.1 M PB containing 20% (w/v) sucrose for 48 h at 4°C for cryoprotection. Fixed brains were cut at 40 μm with a microtome (Komatsu Electronics, Hiratsuka, Japan). The sections were rinsed with 0.1 M PB and spread on the glass slides. The sections were observed under a fluorescent microscope (Eclipse E 600, Nikon, Tokyo, Japan) with a filter for GFP-B (excitation filter; 460–500, dichromatic mirror; 505, barrier filter; 510–560; Nikon) for AVP-eGFP fluorescence. Fluorescent micrographs were obtained with a digital camera (Digital Sight DS-L2, Nikon). AVP-eGFP fluorescence was quantified by outlining the entire region of LC using Image J software (National Institutes of Health, Bethesda, MD, USA).
Immunohistochemistry for TH
In a separate group of rats, sections of brains including the LC were rinsed four times with PBS containing 0.2% (v/v) Triton X. Sections were incubated at 4°C for 2 days with a rabbit antiserum (1: 2000) directed against TH (Chemicon, Billerica, MA, USA). After a 60 min wash in PBS+0.3% (v/v) Triton X, the sections were incubated for 2 h with Alexa Fluor 546-conjugated anti-rabbit IgG (Molecular Probes, Eugene, OR, USA; 1:2000). The sections were mounted onto gelatin-coated glass slides, air dried, and coverslipped with mounting medium for fluorescence (Gel/Mount: Vectashield, Vector Laboratories, Burlingame, CA, USA) and observed under the confocal laser scanning microscopy (Axiovert 200 M; Carl Zeiss Co. Ltd., Jena, Germany) and acquisition system (LSM 5 PASCAL; Carl Zeiss) for screening for TH and eGFP expression.
Statistical analysis
The results are expressed as means ± SEM. Each group within an experiment was compared with the control group. The data were analyzed using a one-way ANOVA followed by Bonferroni or Holm correction for multiple comparisons. Statistical significance was defined as p < 0.05.
Results
KA-induced seizures
S.c. administration of KA (12 mg/kg) in AVP-eGFP transgenic rats elicited immobility or rigid posture that appeared after 30–45 min, and repetitive movements began 2 h after administration. Seizures were rated at hourly intervals after an injection of KA according to a previously defined scale (Stages 1–6; Racine Citation1972; Schauwecker and Steward Citation1997): Stage 1, immobility; Stage 2, forelimb and/or tail extension, rigid posture; Stage 3, repetitive movements, head bobbing; Stage 4, rearing and falling; Stage 5, continuous rearing and falling; and Stage 6, severe tonic–clonic seizures. In the present study, 47 animals received an injection of KA (12 mg/kg s.c.) and 38 of the 47 KA-treated rats exhibited wet dog shake (WDS). Furthermore, 9 out of the 38 rats that showed WDS died due to the severe tonic–clonic seizures, before the completion of the time course. No rats exhibited seizures above Stage 3 at later timepoints. Rats that exhibited seizures between Stages 3 and 6 were included in the analysis (29 rats in all). The peak of the seizures was observed 3 h after s.c. administration of KA ().
Effects of s.c. administration of KA or vehicle on AVP-eGFP fluorescence in the LC by time course
Rats that displayed seizure activity were divided into six groups and perfused 3 h (n = 4), 6 h (n = 5), 24 h (n = 5), 48 h (n = 5), 1 week (n = 6), and 2 weeks (n = 4) after s.c. administration of KA. Control vehicle-treated rats (n = 4) were perfused 24 h after s.c. administration. No AVP-eGFP fluorescence was observed in the LC of control animals (). However, although there was no observed fluorescence in the LC of AVP-eGFP rats 3 h and a little after 6 h (NS) after KA administration (), robust AVP-eGFP fluorescence was localized in the LC in KA-administered rats 24 h (p < 0.05) and 48 h (p < 0.01) after KA administration, reducing to non-significant levels by 1 week (). Statistical significance was determined by one-way ANOVA, following by Holm correction for multiple comparisons (F = 10.781, p < 0.0001)). The expression of AVP-eGFP fluorescence in the LC reached its highest level 48 h after KA administration ().
Figure 2. Effects of s.c. administration of vehicle or KA on eGFP fluorescence in (A-a∼g) the LC, (B-a∼g) the NTS, (C-a∼g) the VLM, and (D-a∼g) the AM. Sections (A-a, B-a, C-a, D-a) were obtained from animals 24 h after s.c. administration of vehicle. Scale bars = 200 μm.
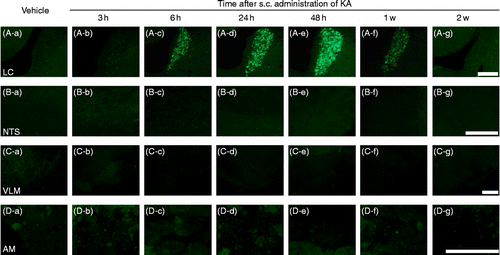
Figure 3. Time course of eGFP fluorescence in the LC after s.c. administration of vehicle or KA. **p < 0.01, *p < 0.05 compared with vehicle. Values represent means ± SEM. Number of rats at each point; vehicle (n = 4), 3 h (n = 4), 6 h (n = 5), 24 h (n = 5), 48 h (n = 5), 1 week (n = 6), and 2 weeks (n = 4). Mean density (100% of control) was calculated from the sections obtained from animals 24 h after s.c. administration of vehicle. Statistical significance was determined by one-way ANOVA, following by Holm correction for multiple comparisons (F = 10.781, p < 0.0001).
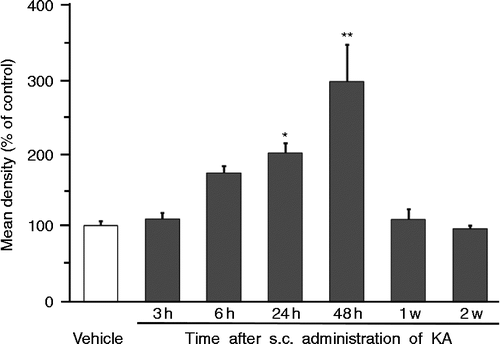
Effects of s.c. administration of KA or vehicle on AVP-eGFP fluorescence in the brainstem and adrenal medulla by time course
No AVP-eGFP fluorescence was observed in the nucleus tractus solitary (NTS; ), in the ventrolateral medulla (VLM; ), and in the adrenal medulla (AM; ) of either vehicle or KA-treated rats at any time.
Effects of pretreatment with MK-801 on AVP-eGFP fluorescence in the LC following KA-induced seizures
Pretreatment with the nonselective N-methyl-d-aspartate (NMDA) receptor antagonist MK-801 suppressed abnormal behavior induced by KA, such as WDS, rearing, and falling. Twenty-four hours after administration of KA (following a pretreatment with vehicle), AVP-eGFP fluorescence was observed in the LC compared with the rats receiving vehicle pretreatment and followed by vehicle control for the KA treatment (p < 0.01; ). By contrast, the pretreatment with MK-801 significantly suppressed AVP-eGFP fluorescence in the LC compared with the administration of KA with the pretreatment of vehicle (p < 0.01; ). No effects on the induction of the AVP-eGFP fluorescence in the LC were observed after s.c. administration of vehicle pretreatment with vehicle () or MK-801 (). Statistical significance was determined by one-way ANOVA, followed by Bonferroni correction for multiple comparisons (F = 22.957, p < 0.0001).
Figure 4. Effects of s.c. administration of vehicle or KA (12 mg/kg) with pretreatment of vehicle or MK-801 (1 mg/kg) on eGFP fluorescence in the LC. Sections were obtained from (A) vehicle-administered rats pretreated with vehicle or (B) MK-801 (1 mg/kg) and (C) KA-administered rats pretreated with vehicle or (D) MK-801 (1 mg/kg). Scale bar = 50 μm. 4 V, fourth ventricle.
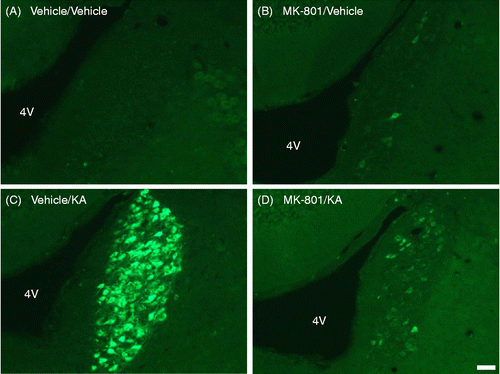
Figure 5. Effect of s.c. administration of vehicle or KA (12 mg/kg) with pretreatment of vehicle or MK-801 (1 mg/kg) on eGFP fluorescence in the LC. **p < 0.01 compared with KA-administered rats pretreated with vehicle. Values represent means ± SEM. Number of rat: vehicle+vehicle (n = 3), MK-801+vehicle (n = 3), vehicle+KA (n = 7), and MK-801+KA (n = 5). Statistical significance was determined by one-way ANOVA, following by Bonferroni correction for multiple comparisons (F = 22.957, p < 0.0001).
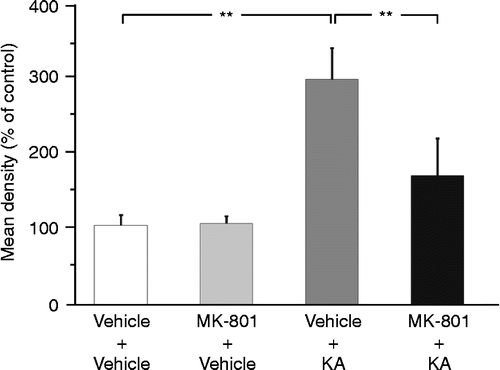
Co-existence of AVP-eGFP fluorescence and TH-immunoreactivity in the LC after KA-induced seizures
AVP-eGFP fluorescence in the LC was observed 24 h after KA administration (), but not the vehicle administration (). TH is the enzyme responsible for the synthesis of DOPA, a precursor for dopamine, which, in turn, is a precursor for noradrenaline (Westfall and Westfall Citation2006). TH-immunoreactivity (red fluorescence) was observed in the LC of control () and KA-administered () rats. AVP-eGFP fluorescence and TH-immunoreactivity were co-localized in the LC neurons only in KA-administered rats (). Almost all AVP-eGFP fluorescence exhibited in TH-immunoreactive neurons in the LC 24 h after KA administration ().
Figure 6. Effect of s.c. administration of vehicle (control) or KA (12 mg/kg) on (A, D) eGFP fluorescence and (B, E) TH-immunoreactivity in the LC. (B, E) TH-antibody complexes were visualized as red fluorescence, using Alexa Fluor 546-conjugated secondary antibody. (C, F) Merged view of fluorescence of eGFP and Alex Fluor 546 is seen as yellow. Sections were obtained from (A–C) control or (D–F) KA-treated rats. Boxed areas are enlarged in D, E, and F. Scale bars = 50 μm.
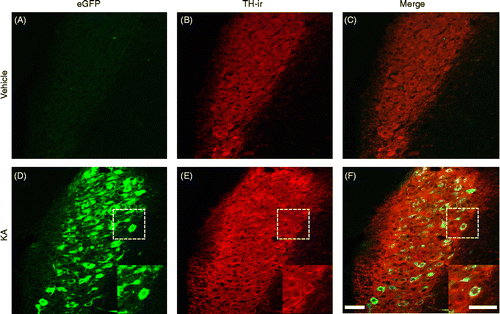
Discussion
The present study has revealed a marked induction of eGFP fluorescence in the LC after KA-induced seizures in AVP-eGFP transgenic rats. Furthermore, pretreatment with MK-801 (a nonselective NMDA receptor antagonist) significantly suppressed both the seizures and the expression of AVP-eGFP fluorescence in the LC after KA administration.
A number of neuropeptides including AVP are released by stress in specific neuronal populations and contribute to the stress response (Landgraf and Neumann Citation2004; Koob Citation2008). Stressful conditions cause marked increases of AVP expression in the parvocellular division of the PVN, which plays an important role in the activation of the HPA axis (Aguilera and Rabadan-Diehl Citation2000). CRH is released in response to stress from axon terminals in the hypothalamic median eminence and acts on receptors in the pituitary (Swanson et al. Citation1983). However, it is now also known to be expressed in neuronal populations in the amygdala (Swanson et al. Citation1983; Koob Citation2008), the hippocampus (Chen et al. Citation2001), and the LC (Valentino and Van Bockstaele Citation2008). Several studies reported that noradrenergic neurons in the LC were activated by seizures (Silveira et al. Citation1998a,Citationb; Bengzon et al. Citation1999; Shouse et al. Citation2001). Thus, we hypothesized that AVP synthesis might be induced in the LC by seizures.
In our previous study (Todoroki et al. Citation2010), we tried to induce AVP-eGFP expression in the LC using several different stressful stimuli. Only i.c.v. administration of colchicine induced expression, and it remains unclear as to whether colchicine is acting as a blocker of axonal transport or a stressor. In contrast, this study has robustly demonstrated induction of AVP-eGFP expression in the LC after KA-induced seizures.
S.c. administration of KA in the AVP-eGFP transgenic rats caused a progressive development of seizure behavior within 24 h. AVP-eGFP fluorescence in the LC was observed between 6 and 24 h after administration of KA, reaching a maximum after 48 h. Interestingly, the peak of eGFP fluorescence did not coincide with that of seizure behavior at 3 h after KA administration, at which point no transgene expression was evident. It has previously been reported that AVP mRNA levels in rat hypothalamus are increased after KA-induced seizures (Sun et al. Citation1996). In addition to a direct effect of KA on hypothalamic neurons, the activation and perturbations of other neurotransmitter systems, such as glutamate, catecholamine, serotonin, and somatostatin following KA-induced seizures could well contribute indirectly to the alterations, i.e. AVP expression (Sun et al. Citation1996). Furthermore, one well-known feature of the KA seizure model is the occurrence of progressive neuronal damage of limbic structures (Ben-Ari Citation1985); most vulnerable are the CA1 (Kim et al. Citation2000) and CA3 pyramidal cell and interneurons of the hilus of the dentate gyrus of the hippocampus. Therefore, it can be speculated that KA-induced seizures activate the HPA axis via the loss of the inhibitory influence of the hippocampus on the HPA axis (Gallagher et al. Citation1984), even after the cessation of seizure behavior (Guoying et al. Citation1997).
Next, we examined the possible role of NMDA receptors in LC noradrenergic neurons. A previous rat study showed that MK-801 at a dose of 3 mg/kg elicited neurotoxicity in the cerebral cortex, and individual death with a dose of 10–20 mg/kg (Ahmed et al. Citation2003). It is unlikely that our low dose of MK-801 (1 mg/kg) would be neurotoxic or act as a stressor. On the contrary, MK-801 protected rats from KA neuropathological syndrome by using an appropriate dose (1 mg/kg; Virgili et al. Citation1992). It also implies that the synthesis of AVP may be upregulated in noradrenergic neurons in the LC through NMDA receptors and play a role in response to severe stress. As to the cause of the significant suppression of the AVP-eGFP fluorescence by pretreatment with MK-801, due to its role as an antagonist of NMDA receptor or a protector against KA or a combination of both, further studies should be investigated.
We addressed the neurochemical identity of the AVP-eGFP cells in the LC by seeking co-localization with TH, the biosynthetic enzyme which is involved in the conversion of tyrosine to dopamine. TH can be used to identify noradrenergic cells within the LC, because cell bodies containing dopamine and adrenaline are absent in the LC (Berod et al. Citation1984). In our experiment, in the LC all neurons were TH-immunoreactive, indicating that the LC contains a large quantity of noradrenergic neurons, and we found that AVP-eGFP fluorescence was colocalized in TH-immunoreactive cells in the LC after administration of KA. In the NTS, the VLM, and the AM, eGFP fluorescence was absent in both control and KA-treated rats although they are also known to contain noradrenergic cells (Kalia et al. Citation1985). This indicates that noradrenaline does not necessarily coexist with AVP in the central and peripheral noradrenergic cells. The specific mechanisms by which the AVP gene is expressed, and AVP is synthesized only in the noradrenergic neurons of the LC is yet to be determined.
There are two possibilities regarding the role of AVP in stress, the bad side and the good side. The bad side is that AVP may exert a bad influence on the trigger of the seizure (Wilkinson and Pittman Citation1994). The good side is that AVP may work to modify the stress (Caldwell et al. Citation2008), paralleling the synthesis of AVP in the parvocellular division of the PVN (Aguilera and Rabadan-Diehl Citation2000). It is therefore important to clarify the influence of LC AVP on stress.
In conclusion, using AVP-eGFP transgenic rats, we have demonstrated robust transgene expression in the noradrenergic neurons in the LC after KA treatment. We suggest that AVP is synthesized in the noradrenergic neurons in the LC only under severe stressful conditions, and affect potentially mediated by NMDA receptors, and may play a role in response to stress.
Acknowledgements
We thank Prof. David Murphy (University of Bristol, UK) for critical reading and language editing of the manuscript. We thank Kanako Shoguchi for her technical assistance. This study was supported by the University of Occupational and Environmental Health Grant for Advanced Research and Grant-in-Aid for Scientific Research on Innovative Area No. 23113518, Scientific Research (A) No. 22249025, Scientific Research (B) 22390044, Challenging Exploratory Research No. 23659127 from the Ministry of Education, Culture, Sports, Science, and Technology, Japan.
Declaration of interest: The authors report no conflicts of interest. The authors alone are responsible for the content and writing of the paper.
References
- Aguilera G, Rabadan-Diehl C. 2000. Vasopressinergic regulation of the hypothalamic–pituitary–adrenal axis: Implications for stress adaptation. Regul Pept. 96:23–29.
- Ahmed MM, Yamamoto M, Chikuma T, Rahman MM, Kato T. 2003. Dose-dependent effect of MK-801 on the level of neuropeptides processing enzymes in rat brain regions. Neurosci Res. 47:177–189.
- Ben-Ari Y. 1985. Limbic seizure and brain damage produced by kainic acid: Mechanisms and relevance to human temporal lobe epilepsy. Neuroscience. 14:375–403.
- Ben-Ari Y, Tremblay D, Riche D, Ghilini G, Naquet R. 1982. Electrographic, clinical and pathological alterations following systemic administration of kainic acid, bicuculline or pentetrazole: Metabolic mapping using the deoxyglucose method with special reference to the pathology of epilepsy. Neuroscience. 6:1361–1391.
- Bengzon J, Hansson SR, Hoffman BJ, Lndvall O. 1999. Regulation of norepinephrine transporter and tyrosine hydroxylase mRNAs after kainic acid-induced seizures. Brain Res. 842:239–242.
- Berod A, Chat M, Paut L, Tappaz M. 1984. Catecholaminergic and GABAergic anatomical relationship in the rat substantia nigra, locus coeruleus, and hypothalamic median eminence. J Histochem Cytochem. 32:1331–1338.
- Caffe AR, van Leeuwen FW, Buijs RM, de Vries GJ, Geffard M. 1985. Coexistence of vasopressin, neurophysin and noradrenaline immunoreactivity in medium-sized cells of the locus coeruleus and subcoeruleus in the rat. Brain Res. 338:160–164.
- Caldwell HK, Lee H-J, Macbeth AH, Young WSIII. 2008. Vasopressin: Behavioral Roles of an “Original” Neuropeptide. Prog Neurobiol. 84 1: 1–24.
- Chen Y, Bender RA, Frotscher M, Baram TZ. 2001. Novel and transient populations of corticotrophin-releasing hormone-expressing neurons in developing hippocampus suggest unique functional roles: Quantitative spatiotemporal analysis. J Neurosci. 21:7171–7181.
- Collins RC, McLean M, Olney JW. 1980. Cerebral metabolic response to systemic kainic acid: 14-C-deoxyglucose studies. Life Sci. 27:855–862.
- Gallagher BB, Murvin A, Flanigin HF, King DW, Luney D. 1984. Pituitary and adrenal function in epileptic patients. Epilepsia. 25:683–689.
- Guoying B, Belinda W, Pearlie H, Lei J, Zhehui F, Wanqin Z, Renjie B, Jau-Shyong H. 1997. A single dose of kainic acid elevates the levels of enkephalins and activator protein-1 transcription factors in the hippocampus for up to 1 year. Neurobiology. 94 17: 9422–9427.
- Kalia M, Fuxe K, Goldstein M. 1985. Rat medulla oblongata. II. Dopaminergic, noradrenergic (A1 and A2) and adrenergic neurons, nerve fibers, and presumptive terminal process. J Comp Neurol. 233:308–332.
- Kim HC, Jhoo WK, Kim WK, Suh JH, Shin EJ, Kato K, Ko KH. 2000. An Immunocyto-chemical study of mitochondrial manganese-superoxide in the rat hippocampus after kainite administration. Neurosci Lett. 281:65–68.
- Koob GF. 2008. A role for brain stress systems in addiction. Neuron. 59:11–34.
- Landgraf R, Neumann ID. 2004. Vasopressin and oxytocin release within the brain: A dynamic concept of multiple and variable modes of neuropeptide communication. Front Neuroendocrinol. 25:150–176.
- Nomura M, Ueta Y, Hannibal J, Serino R, Yamamoto Y, Shibuya I, Matsumoto T, Yamashita H. 2000. Induction of pituitary adenylate cyclase-activating polypeptide mRNA in the medial parvocellular part of the paraventricular nucleus of rats following kainic-acid-induced seizure. Neuroendocrinology. 71:318–326.
- Piekut D, Phipps B. 1998. Increased corticotropin-releasing factor immunoreactivity in selected brain sites following kainic elicited seizures. Brain Res. 781:100–113.
- Piekut D, Phipps B, Pretel S, Applegate C. 1996. Increased corticotropin-releasing factor neuronal systems. Brain Res. 743:63–69.
- Racine RJ. 1972. Modification of seizure activity by electrical stimulation: II. Motor seizure. Electroenceph clin Neurophysiol. 32:281–294.
- Sakamoto K, Saito T, Orman R, Koizumi K, Lazar J, Salciccioli L, Stewart M. 2008. Autonomic consequences of kainic acid-induced limbic cortical seizures in rats: Peripheral autonomic nerve activity, acute cardiovascular changes, and death. Epilepsia. 49:982–996.
- Samuels ER, Szabadi E. Functional Neuroanatomy of the noradrenergic locus coeruleus: Its roles in the regulation of arousal and autonomic function part I: Principles of functional organisation. Curr Neuropharmacol. 2008a; 6 3: 235–253.
- Samuels ER, Szabadi E. Functional Neuroanatomy of the noradrenergic locus coeruleus: Its roles in the regulation of arousal and autonomic function part II: Physiological and pharmacological manipulations and pathological alterations of locus coeruleus activity in humans. Curr Neuropharmacol. 2008b; 6 3: 254–285.
- Schauwecker PE, Steward O. 1997. Genetic determinants of susceptibility to excitotoxic cell death: Imprications for gene targeting approaches. Proc Natl Acad Sci USA. 94:4103–4108.
- Shouse MN, Staba RJ, Ko PY, Saquib SF, Farber PR. 2001. Monoamines and seizures: Microdialysis findings in locus coeruleus and amygdale before and during amygdale kindling. Brain Res. 892:176–192.
- Silveira DC, Liu Z, Holmes GL, Schomer DL, Schchter SC. Seizures in rats treated with kainic acid induce Fos-like immunoreactivity in locus coeruleus. Neuroreport. 1998a; 9:1353–1357.
- Silveira DC, Liu Z, LaCalle S, Lu J, Klein P, Holmes GL, Herzog AG. Activation of the locus coeruleus after amygdaloid kindling. Epilepsia. 1998b; 39:1261–1264.
- Sun Q, Pretel S, Applegate CD, Piekut DT. 1996. Oxytocin and vasopressin mRNA expression in rat hypothalamus following kainic acid-induced seizures. Neuroscience. 71:543–554.
- Swanson LW, Sawchenko PE, Rivier J, Vale WW. 1983. Organization of ovine corticotrophin-releasing factor immunoreactive cells and fibers in the rat brain: An immunohistochemical study. Neuroendocrinology. 36:165–186.
- Todoroki M, Ueta Y, Fujihara H, Otsubo H, Shibata M, Hashimoto H, Kobayashi M, Sakamoto H, Kawata M, Dayanithi G, Murphy D, Hiro H, Takahashi K, Nagata S. 2010. Induction of the arginine vasopressin-enhanced green fluorescent protein fusion transgene in the rat locus coeruleus. Stress. 13 4: 281–292.
- Tobin VA, Hashimoto H, Wacker DW, Takayanagi Y, Langnaese CC, Noack J, Landgraf R, Onaka T, Leng G, Meddle SL, Engelmann M, Ludwig M. 2010. An intrinsic vasopressin system in the olfactory bulb is involved in social recognition. Nature. 464 7287: 413–417.
- Ueta Y, Fujihara H, Serino R, Dayanithi G, Ozawa H, Matsuda K, Kawata M, Yamada J, Ueno S, Fukuda A, Murphy D. 2005. Transgenic expression of enhanced green fluorescent protein enables direct visualization for physiological studies of vasopressin neurons and isolated nerve terminals of the rat. Endocrinology. 146:406–413.
- Valentino RJ, Van Bockstaele E. 2008. Convergent regulation of locus coeruleus activity as an adaptive response to stress. Eur J Pharmacol. 583:215–225.
- Virgili M, Migani P, Contestabile A, Barnabei O. 1992. Protection from kainic acid neuropathological syndrome by NMDA receptor antagonists: Effect of MK-801 and CG 39551 on neurotransmitter and glial markers. Neuropharmacology. 31:469–474.
- Westfall TC, Westfall DP. 2006. Neurotransmission: The automatic and somatic motor nervous systems. In: Brunton LL, Laoz JS, Parker DP. editors. Goodman & Gilman's the pharmacological basis of therapeutics. New York, NY: McGraw-Hill137–182.
- Wilkinson MF, Pittman QJ. 1994. Alteration of the physiological responses to indomethacin by endotoxin tolerance in the rat: A possible role for central vasopressin. J Physiol. 479.3:441–449.