Abstract
The altered activity of the hypothalamic–pituitary–adrenal (HPA) axis is often observed in stress-related disorders. According to the literature, about 60% of patients with major depressive disorder elicit high levels of cortisol. It is still unclear why high cortisol levels are not observed in all patients. In this study, we used the chronic mild stress (CMS) rat model of depression, which is based on continuous exposure to unpredictable stressors, to track longitudinal changes in HPA function using fecal corticosterone metabolites (FCM) as a read out. The dexamethasone suppression test was used to assess negative feedback inhibition of the HPA axis. Our results show (1) a disturbance in diurnal corticosterone rhythm measured as fluctuations of the diurnal FCM peak, (2) differences in corticosterone levels between stress-susceptible and stress-resilient animals, (3) recovery of diurnal corticosterone rhythm after 8 weeks of CMS, and (4) alterations in sensitivity to dexamethasone in negative feedback regulation of corticosterone secretion during the time course of CMS. Thus, a disruption of HPA axis circadian rhythmicity coincides with the initial state in the development of depression-like behavior. This chronobiological abnormality, as well as the hypersecretion of corticosterone, is state, rather than trait, dependent.
Introduction
The secretion of glucocorticoids (predominantly cortisol in humans and corticosterone in rats) is the important endocrine component of the stress response, and is necessary for successful adaptation. The acute response, preparing the body to fight or run away in response to an immediate perceived threat, has a time course of 1–2 h. The chronic response, when the body encounters a situation of perceived threat in the state of ongoing physiological arousal, has a time course of days or weeks (Checkley Citation1996).
Cortisol/corticosterone (Cort) secretion by the adrenal cortex is regulated by the hypothalamic–pituitary–adrenal (HPA) axis. HPA axis activity is governed by the secretion of corticotropin-releasing hormone (CRH) and arginin-vasopressin (AVP) from the hypothalamus. CRH is produced by small-bodied neurons in the paraventricular nucleus. Neurons store CRH in secretory vesicles located in synaptic terminals in the median eminence of the hypothalamus and can release CRH acutely in the absence of new synthesis. After release into the interstitial fluid of the median eminence, CRH enters the hypophysial portal venous plexus and travels to the anterior pituitary, where through the G-protein-coupled receptor on the cell membrane of corticotroph cells leads to the exocytosis of preformed adrenocorticotropic hormone (ACTH). The large-diameter hypothalamic neurons located in the supraoptic and paraventricular nuclei of the hypothalamus synthesize AVP and oxytocin and then transport these hormones along their axons to the site of release in the posterior pituitary. Although CRH is the major regulator of ACTH secretion, AVP is also a potent ACTH secretagogue and plays a physiological role in the regulation of ACTH secretion in various stress states (Barrett Citation2009). ACTH stimulates the secretion of the glucocorticoids from the adrenal cortex. Glucocorticoids interact with their receptors in multiple target tissues including the HPA axis and limbic system, where they are responsible for feedback inhibition of CRH and AVP in the hypothalamus and have a direct effect on the secretion of ACTH from the pituitary (De Kloet et al. Citation1998). Considering its role at the interface between stress and brain functioning, the HPA axis has been found to be abnormal in psychiatric disorders and particularly in major depressive disorder (MDD). For example, a significant percentage of depressed patients have increased cortisol levels in the saliva, plasma, and urine and increased pituitary and adrenal gland size as well as activity (Nemeroff and Vale Citation2005). This abnormality is often associated with the disruption of the HPA axis negative feedback regulation (Kathol et al. Citation1989).
Secretion of Cort has a circadian rhythm. In healthy individuals, peak Cort secretion appears at ‘waking’ hours: in humans in the morning and in nocturnal laboratory rats about the time point when the facility lighting switches off. The lowest level of Cort secretion is normally observed in humans in the late afternoon (Streeten et al. Citation1984) and in rodents about the time point when facility lighting switches on (Lepschy et al. Citation2007). In some patients with MDD, circadian rhythm is disturbed, i.e. Cort secretion is elevated at the time period when it should be low (Deuschle et al. Citation1997; Wong et al. Citation2000). Furthermore, there is a correlation between emotional state and changes in Cort secretion (Dinan Citation1994). It has been shown in contrast to melancholic depression that atypical depression is not associated with hypercortisolemia (Gold et al. Citation1995). Furthermore, phase shifts in Cort peak secretion and ACTH nadir have been reported in depression by some authors (Linkowski et al. Citation1987; Rubin et al. Citation1987; Antonijevic et al. Citation2000; Koenigsberg et al. Citation2004). Studies reporting a change in Cort circadian rhythm in depression are few, include small sample sizes, and draw conclusions based on a non-representative patient group (Linkowski et al. Citation1987) or on small phase shifts (without pronounced increase in plasma Cort) (Koenigsberg et al. Citation2004). Therefore, these data do not justify the conclusion that Cort circadian rhythms change in a specific subtype of major depression (Antonijevic Citation2008).
This study is among the first to look at the diurnal variation of Cort in the context of stress-induced abnormalities. Stetler and Miller's (Citation2011) quantitative meta-analysis of more than 400 studies of depression and HPA axis activity show a complex picture of HPA activity during depression thereby pointing to heterogeneity of Cort response within a single diagnostic label that should be taken into account when existing theories of disease are refined and new ones emerge in the field of psychosomatic medicine.
Endogenous glucocorticoids serve as potent negative regulators of HPA axis activity by binding to their cognate receptors in HPA-regulatory regions. Data supporting the notion that glucocorticoid-mediated feedback inhibition is impaired in major depression come from a multitude of studies (Carroll Citation1982; Pariante and Miller Citation2001; Calfa et al. Citation2003), demonstrating impaired HPA axis suppression following pharmacological stimulation of the glucocorticoid receptors with the synthetic glucocorticoid dexamethasone. By contrast, even a small dose of dexamethasone induces a potent feedback inhibition of the HPA axis in healthy subjects, leading to reduced cortisol levels for up to 24 h (Pariante Citation2006). Interestingly, successful antidepressant treatment is associated with restoration of negative feedback on the HPA axis by glucocorticoids (reviewed in Pariante Citation2006; Surget et al. Citation2011).
Some publications indicate that the different subtypes of MDD, e.g. melancholic versus psychotic, may be related to a suppression or non-suppression of endogenous Cort response to dexamethasone injection (Caroff et al. Citation1983; Banki et al. Citation1986; Antonijevic Citation2008). Such a classification could be a considerable help in clinical practice for the diagnosis of MDD.
In this study, we used a validated chronic mild stress (CMS) rat model of depression based on continuous exposure to unpredictable mild stressors. The great advantage of the CMS model is the possibility to differentiate individual stress responses on the basis of the sucrose consumption test (SCT) and physiological and behavioral reactions. In our study, we categorize animals as ‘stress-resilient’ when they demonstrate a persistent intake of sucrose solution during the CMS and do not fall in the SCT by more than 10% during the experiment. Animals with a drop in SCT more than 40% are taken to indicate ‘anhedonic-like’ behavior. The remaining group of rats with a modest decrease in sucrose intake is designated as an intermediate group (Henningsen et al. Citation2009; Palacios et al. Citation2011). Advantages and disadvantages of using the CMS model to mimic symptoms of MDD are discussed elsewhere (Willner Citation2005). However, the model is validated for the study of mechanisms underlying the development of depression-like conditions as well as antidepressant treatment (Maes Citation1999; Jayatissa et al. Citation2006; Pittenger and Duman Citation2008). Therefore, the CMS paradigm was selected as a tool to investigate, in detail, a stress-induced reaction in a chronic experiment by following diurnal rhythms of endogenous Cort secretion and suppression of Cort secretion by dexamethasone in rats exposed to unpredictable stressors during an 8-week period.
The achievement of this goal was made possible by developing a non-invasive technique to monitor stress hormones in small laboratory animals (Touma et al. Citation2003). The method is based on a determination by enzyme immunoassay (EIA) of fecal corticosterone metabolites (FCM) that allow for time-integrated sampling over several hours and for the acquisition of samples, independent of any invasive sampling methods that may provoke an acute stress effect. The methodical reliability has been clearly demonstrated by pharmacological stimulation and suppression of adrenocortical activity, which was accurately reflected by means of corticosterone metabolite measurements in the feces of male and female mice and rats (Touma et al. Citation2004; Lepschy et al. Citation2007). This method has also been used to assess potential differences in the maximal capacity of the adrenal cortex in a murine model of affective disorder (Touma et al. Citation2008). Together, these findings allow and indicate that fecal corticosterone determinations can be used to evaluate the function of the HPA axis during the development of the CMS-induced depression-like condition in rats and achieve reliable results.
Materials and methods
Male Wistar rats were purchased from Taconic (Ry, Denmark). Animal weight was about 200 g when adaptation for sucrose consumption was initiated, and was approximately 350 g at the start of the stress regime. The animals were singly housed, except when grouping was applied as a stressor. Food and water were available ad libitum except when food or/and water deprivation were applied as a stressor. The standard 12-h light/dark cycle (light phase 6:00–18:00) was only changed in the course of the stress regime.
The first two weeks after arrival, animals were adapted to animal facilities. The following two weeks all animals were trained to drink palatable sucrose solution. Throughout the training period, the rats were exposed to the SCT semiweekly, consisting of 1-h exposure to a bottle with sucrose solution. Afterwards, SCTs were conducted once a week until the end of the experiment. Permission for conducting the animal experiments was obtained from the Danish National Committee for Ethics in Animal Experimentation.
In the current study, 28 rats were randomly chosen and, in eight of them, the diurnal rhythm and effect of the saline injection were tested prior to starting the CMS protocol. For this purpose, animals were adapted to a grid floor during a 3-day period. Another 20 rats were exposed to CMS. Fecal sample collection and dexamethasone suppression test (DST) were performed as described below.
Sucrose consumption test
SCT is a weekly test where the sucrose intake of individual animals is measured after 1-h exposure to a bottle with 1.5% sucrose solution (Jayatissa et al. Citation2006). To achieve a comparable level of thirst and hunger prior to the SCT, animals were deprived of food and water for 14 h prior to the test. The baseline sucrose intake was calculated as an average intake from three consequent SCTs applied prior to starting the CMS protocol. For each SCT during the 8 weeks of the experiment, the sucrose index (SI) was calculated as a percentage of the current intake value relative to the baseline intake. Averaged sucrose index (avSI) was then calculated and used as a parameter to evaluate individual hedonic status.
CMS protocol
The applied stress procedure was described in detail previously (Jayatissa et al. Citation2006; Henningsen et al. Citation2009; Palacios et al. Citation2011). In short, the stress protocol with a 14-day cycle consisted of: one period of intermittent illumination, stroboscopic light, grouping, food or water deprivation; two periods of soiled cage and no stress; and three periods of 45° box tilting. During grouping, rats were housed in pairs with different partners alternately serving as resident or intruder. All stressors lasted 10–14 h. During the period of fecal samples collection, the animals were placed in grid-floor cages 12 h prior to the first sampling procedure. This replacement was used as a stressor in the CMS protocol.
Collection of samples for FCM measurement
Fecal samples for the measurement of diurnal FCM rhythm and the DST in 20 animals were collected on weeks 2, 4, and 8 of CMS. Animals were transferred to grid-floor cages at 18:00 on Sunday of the corresponding week. The first sampling plate was inserted under the cages at 9:00 and the collection of samples started at 12:00, continuing for 57 h with 3-h intervals. The collection of samples during the dark phase of the light cycle was performed under dim red light. To conduct DST, 1 mg/kg of dexamethasone was injected i.p. at 9:00 into all animals within 30 min. We defined the sampling point as a time midpoint between two sampling procedures.
Fecal corticosterone metabolites
The collected fecal samples were analyzed for immunoreactive FCM using a 5α-pregnane-3β,11β,21-triol-20-one EIA (Touma et al. Citation2003), validated for rats as described by Lepschy et al. (Citation2007, Citation2010). Fecal samples were homogenized and aliquots of 0.25 g were extracted with 5 ml of 80% methanol. The EIA used a double-antibody technique and was performed on anti-rabbit-IgG-coated microtiter plates. After overnight incubation (at +4°C) of standards (range: 0.8–200 pg/well) and samples with steroid antibody and biotinylated label, plates were emptied, washed, and blotted dry. A streptavidin horseradish peroxidase conjugate was then added, and after an additional 45 min the plates were again emptied, washed, and blotted dry. The substrate (tetramethylbenzidine) was added and incubated for another 45 min at +4°C until the reaction was stopped with 1 M sulfuric acid. Optical densities (at 450 nm) were recorded using an automatic plate reader, and the hormone concentrations were calculated. The inter-assay coefficients of variation were 9.6% and 11.9% for low and high concentration pool samples, respectively.
Statistical analysis
Q–Q plots, descriptive statistics, and one-way ANOVA followed by post hoc comparison were used to validate the SCT results.
FCM analyses were carried out with non-parametric statistics, since not all data followed normal distribution as tested by Shapiro–Wilk (Shapiro and Wilk Citation1965) and Jarque–Bera (Jarque and Bera Citation1980) tests. All tests were two tailed and were calculated using XLSTAT package version 2010 from Addinsoft compatible with MS Excel.
ANOVA on ranks (Friedman's test) was used to evaluate the difference in repeated samples between weeks of CMS, and the Nemenyi's (Citation1963) procedure was used for the multiple pairwise comparisons. Two dependent samples were compared using the Wilcoxon signed-rank test. Two independent samples were compared by the Mann-Whitney U-test, while differences between more than two independent samples were compared with the Kruskal–Wallis test (KW test), followed by Dunn's post-test where appropriate. For all tests, differences were considered significant if their probability of occurring by chance was < 5% (p < 0.05).
Results
Sucrose consumption test
Twenty rats exposed to CMS were used in this study. Based on SCT, four animals with avSI of 1.01 ± 0.06 were identified as resilient (Res), five animals with avSI of 0.56 ± 0.03 were identified as anhedonic-like (Anh), and other 11 animals with avSI of 0.71 ± 0.03 were identified as intermediate (IM, ). The segregation was significant (F(1,19) = 4.053; p < 0.001) throughout the entire period of exposure to CMS. In addition, the SI of the animals from the resilient group was significantly higher compared to both groups of the anhedonic-like (F(1,8) = 4.769, p < 0.001) and intermediate (F(1,8) = 2.529, p < 0.03) animals.
Figure 1. Effect of CMS on sucrose index, calculated as a ratio between weekly sucrose intake and baseline values of sucrose consumption measured before the onset of stress. The CMS protocol was applied during 8 weeks and animals were divided into resilient (Res, n = 4), anhedonic-like (Anh, n = 5), and intermediate (IM, n = 11) groups according to their hedonic status evaluated by averaged SI calculated throughout the experiment. Gray bars indicate periods of time of fecal sampling. Animals in Anh and IM groups had a significantly lower SI during the entire experiment confirmed by ANOVA and Dunnett's post hoc comparison. Data are shown as mean ± SEM, ***p < 0.001; *p < 0.05 between groups during the time course of the experiment.
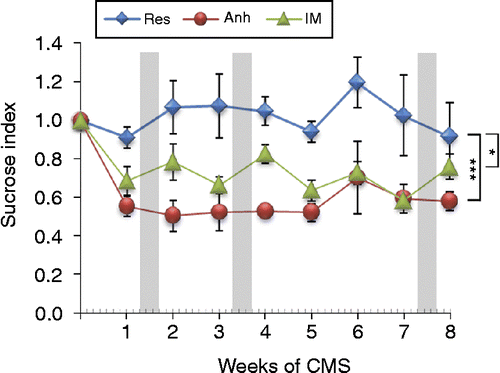
FCM concentrations: diurnal rhythm and CMS
Baseline FCM concentration before the onset of the stress protocol
The circadian rhythm in FCM was tested in eight animals prior to the CMS procedure before and after saline injection (). Peak FCM levels occurred at sampling point 22:30 in 62% of animals during the first 24 h (Day 0) and in 75% of animals during the second 24 h (Day 1) of the experiment. The average peak values of FCM were 5.8 ± 0.93 and 6.2 ± 0.84 μg/g, respectively. The lowest (nadir) level of FCM occurred at intervals 15:30 and 19:30 with an average value of 2.2 ± 0.25 μg/g FCM on Day 0 and 1.8 ± 0.28 μg/g FCM on Day 1. Only one animal out of eight showed highest/lowest FCM levels at sampling point 10:30/16:30 on Day 0 and 22:30/1:30 on Day 1. The difference in FCM between peak and nadir levels was 3.6 ± 2.2 μg/g.
Figure 2. FCM measured with the 5α-pregnane-3β,11β,21-triol-20-one EIA in a random group (n = 8) before CMS. Animals were adapted to the grid floor during 3 days before fecal sampling. To control the effect of injection on diurnal rhythm, the sterile saline solution was injected (1 ml/rat) at 9:00 on Day 1 (as indicated). Data are given as box plots (showing medians by lines in boxes), 25% and 75% (boxes), and 10% and 90% (whiskers). Dark background corresponds to diurnal variation 24 h prior to the saline injection, lighter background indicates Day 1 after saline injection. The dark phase (horizontal bar) is indicated at the top of the panel.
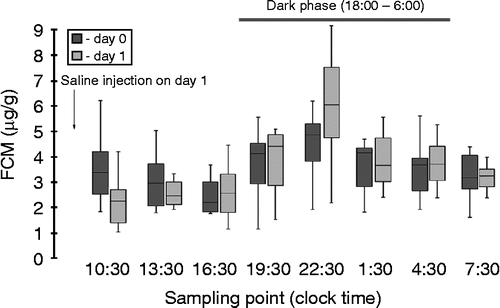
Effect of CMS on diurnal FCM concentrations
Distinct circadian rhythm variations in the secretion of glucocorticoids were observed in all stress animals during the experiment (; ). Highest FCM concentrations were measured during the dark phase, while relatively low levels were observed during the light phase of a 12-h light cycle. The comparison of peak FCM levels revealed significant differences between weeks 2 and 8 (Q(5.991) = 9.1, p < 0.05). Significant differences between nadir FCM values were observed between weeks 2 and 4 (Q(5.991) = 8.04, p < 0.01).
Figure 3. Histograms of frequency distribution for animals exposed to 8-week CMS and divided by the groups according to their hedonic status: anhedonic-like (Anh, n = 5), stress resilient (Res, n = 4), and intermediate (IM, n = 11) for FCM peak (upper panel) and FCM nadir (lower panel) concentrations by week 2 (left column), week 4 (middle column), and week 8 (right column) of CMS.
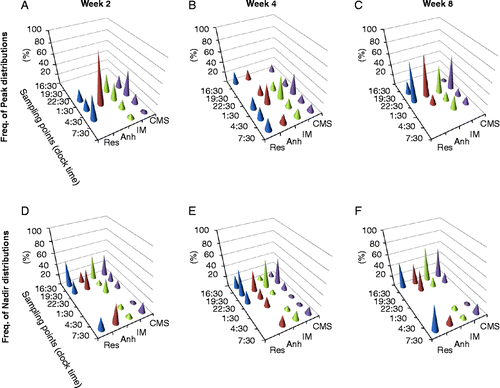
Table I. FCM concentrations for peak, nadir and their differences (Delta) in CMS groups during the 8-week CMS protocol.
By week 2 of CMS, 3-h phase delay in peak FCM was observed. Only 15% of CMS animals exhibited peak FCM secretion at the sampling point 22:30. The majority of animals (40%) exhibited a peak secretion of FCM at the sampling point 1:30; 10% and 5% of animals had their peaks at the sampling points 4:30 and 7:30, respectively (). In the anhedonic-like group, all animals had their peak FCM concentrations at the sampling point 1:30 collected between 0:00 and 3:00, while most of the animals from the resilient group (50%) had their peak FCM concentrations at the sampling point 4:30, collected between 3:00 and 6:00. The peak of FCM concentrations in animals from the intermediate group was observed at the sampling points 22:30–7:30 with 36% at both 13:30 and 16:30.
By the fourth week of CMS, the distribution of FCM peak concentrations was even more inhomogeneous and spread between sampling points 16:30 and 7:30, but 40% of CMS animals had a peak FCM concentration at the sampling point 1:30. Eighty percent of anhedonic-like animals had their peaks at two sampling points at 16:30 and 1:30. Peaks of FCM concentrations in animals from the resilient group were equally distributed between the sampling points 16:30, 1:30, 1:30, 4:30, and 7:30. Most of animals in the intermediate group (40%) had peak FCM concentrations at the sampling point 1:30 (). Interestingly, only individuals from resilient and anhedonic-like, but not intermediate group had a peak of FCM at sampling point 16:30, where animals tested prior to the CMS elicited the lowest levels of FCM ().
The distribution of FCM peak activity by week 8 of CMS was still heterogeneous, but most animals (60%) demonstrated a peak activity at the sampling point 22:30 (), demonstrating that the rhythmic activity in FCM excretion returned to the basal level.
The analysis of diurnal distribution of the FCM nadirs also indicated a disturbance in circadian rhythm in animals exposed to CMS. By the second stress week, 40% of CMS animals exhibited nadirs at the sampling point 16:30 (). Fifty percent of resilient animals had their nadir in FCM concentrations at the sampling point 16:30; 80% of anhedonic-like animals elicited a nadir in FCM levels at 19:30 and 7:30; and 45% of animals from intermediate group had their nadir concentrations at the sampling point 16:30. By week 4 of CMS, 50% of CMS animals had their nadirs at the sampling point 19:30 (). Despite the heterogeneity of frequency distribution of lowest FCM diurnal concentrations, the majority of animals in all three groups demonstrated their nadir FCM values at the sampling point 19:30 (). By week 8 of CMS, the majority of animals (55%) demonstrated the lowest FCM concentrations at the sampling point 16:30 similar to the results obtained prior to the CMS ().
The analysis of differences in hours between nadir and peak levels of FCM secretion revealed the distribution of these differences between 3 and 21 h with the greatest frequency of differences in 6 h in 45% of CMS animals by week 2 of CMS; in 25% of animals by week 4, and in 30% of animals by week 8 of stress (data not shown).
The tendency toward lower concentrations of FCM in the resilient group was observed already by week 2 of CMS (). The FCM comparisons in the resilient and anhedonic-like groups revealed significantly higher FCM concentrations in the anhedonic-like group at the sampling point 1:30 partly due to shifted FCM peaks in some of the resilient animals (). By week 4 of the CMS, the differences between resilient and anhedonic-like groups became obvious (). The level of significance between the groups was calculated as 0.07, but Dunn's post-Kruskal–Wallis multiple pairwise comparisons revealed significant differences at sampling points 16:30, 22:30, and 4:30. By week 8 of the CMS, all animals demonstrated identical patterns in FCM diurnal changes indicative of habituation of the HPA axis function to incessant mild stress regardless of individual hedonic status ().
Figure 4. Diurnal FCM distribution at different time points during CMS and effect of DST. FCM measured with the 5α-pregnane-3β,11β,21-triol-20-one EIA in rats from resilient (Res; n = 4), anhedonic-like (Anh; n = 5), and intermediate (IM; n = 11) by week 2 (A), week 4 (B), and week 8 (C) of CMS. Data for the measurements from all CMS animals are given as box plots (showing medians by lines in boxes), 25% and 75% (boxes), and 10% and 90% (whiskers). Diamonds, circles, and triangles indicate medians for FCM distribution in the resilient, anhedonic-like, and intermediate groups, respectively. 1 mg/kg of dexamethasone (Dex) was injected at 9:00 on Day 1 as indicated by the arrow. The dark phase (horizontal bar) is indicated at the top of the panel. Statistical differences between the three groups were analyzed using KW test following Dunn's post comparison. *p < 0.05 Anh vs. Res.
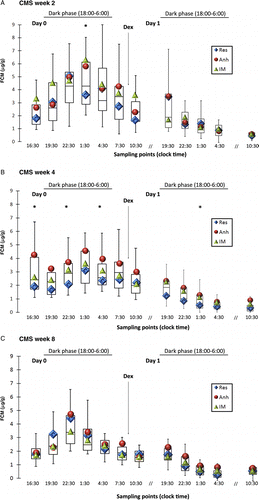
Analysis of repeated measures in CMS groups
To simplify repeated measures comparisons, the areas under curves (AUC) were calculated (). During the 8-week experiment, the application of CMS protocol had a significant effect on the FCM excretion (Q(5.991) = 10.0, p < 0.01). Furthermore, multiple pairwise comparisons revealed a significant decline in FCM in CMS animals between weeks 2 and 8. Distinct analysis within CMS groups revealed a significant decrease in AUC in the resilient group between weeks 2 and 4, and in the intermediate group between weeks 2 and 8. The significance in the discrepancy between resilient and anhedonic-like rats at week 4 was confirmed by comparison of the differences in AUC between the groups.
Table II. AUC for individual FCM measures in CMS groups.
Dexamethasone suppression test
A DST was used to stimulate the glucocorticoid receptors, thereby testing the stress sensitivity of feedback inhibition of the HPA axis activity. Through competitive binding to glucocorticoid receptors, dexamethasone stimulates negative feedback mechanisms. Multidrug resistance P-glycoprotein prevents dexamethasone from passing through the blood–brain barrier. However, this does not completely block dexamethasone from entering the brain. Therefore, both pituitary and brain sites of the HPA axis negative feedback regulation could be activated by a high dose of drug, such as 1 mg/kg.
The effect of DST on FCM concentrations was measured in fecal samples collected during a period of 27 h after i.p. injection of 1 mg/kg of dexamethasone. Dexamethasone solution was injected at 9:00 on Day 1 as indicated in . At all three periods of CMS, the injection of dexamethasone significantly reduced the excretion of FCM in all three groups of animals (). The detailed analysis of dexamethasone-induced suppression in FCM in three CMS groups revealed significantly lower post-dexamethasone FCM values by 4 weeks of CMS in the resilient group (0.34 ± 0.07 μg/g), in comparison to both the anhedonic-like (0.8 ± 0.4 μg/g, p < 0.05) and intermediate (0.7 ± 0.4 μg/g, p < 0.05) groups. Interestingly, the pharmacological effect of dexamethasone, identified as a negative difference in FCM before and after drug injection, at the second week of CMS was observed at the sampling point 19:30 in resilient and anhedonic-like groups (), but at the sampling point 16:30 in the intermediate group (data not shown). By weeks 4 and 8 of stress, the animals in the anhedonic-like and resilient groups demonstrated this effect at the sampling point 16:30 (data not shown). The statistical analysis revealed significant changes in the DST efficiency in all animals exposed to CMS throughout the entire experiment (Q(5.991) = 8.74, p < 0.05). Dunn's post-KW comparison identified an increase in efficiency between weeks 2 and 4 (p = 0.004). Differences in the dexamethasone effect between the resilient and anhedonic-like groups reached significance at week 8 of CMS at sampling point 19:30 (p < 0.013). A tendency toward an increased effect of dexamethasone during CMS in the resilient group was observed according to sampling points 19:30 and 22:30 (). In the anhedonic-like group, the greatest effect of DST was observed at the sampling point 1:30 on weeks 2 and 4 and at 22:30 on week 8 of CMS. It is important to mention that the strongest effect of dexamethasone (the lowest FCM concentration) was measured mostly in samples collected between 15:00 and 18:00 on week 8 of CMS, which was 3 h earlier than on weeks 2 and 4 (Q(5.991) = 14.85, p < 0.001). Post-KW comparisons revealed significant differences between weeks 2 and 8: 22 ± 3 h vs. 19 ± 4 h (p < 0.05) and weeks 4 and 8: 24 ± 3 h vs. 19 ± 4 h (p < 0.001).
Table III. Efficiency of DST calculated as a difference in FCM before and after dexamethasone injection at selected sampling points.
Discussion
The CMS model of depression was used as a tool to investigate the pattern of changes in circadian rhythmic activity and sensitivity of the HPA axis to exogenous glucocorticoids during the period of developing a depression-like state in rats. After a period of adaptation to a palatable sucrose solution, rats demonstrated a typical diurnal activity in Cort secretion, measured as a FCM concentration, with peak activity occurring at sampling point 22:30 (corresponding to an average sample collected between 21:00 and 24:00) and nadir FCM levels measured at sampling points 16:30 and 19:30. These results are in line with previous findings demonstrated by Lepschy et al. (Citation2010), where the peak FCM secretion was measured at sampling points 13.5–16.5 (sampling intervals 22:30–1:30).
Our next step was to study the CMS effect on the circadian Cort rhythmic activity and changes in peak and nadir FCM concentrations. Results indicate persistent diurnal activity in FCM during all 8 weeks of chronic stress exposure; however, stress-affected diurnal variations and a consequent shift in peak and nadir FCM excretion were observed within the 12:12-h light cycle. shows shifted distributions of peak appearance along the time axis by weeks 2 and 4 of CMS. By 8 weeks of CMS, the distributions were comparable to those seen before the onset of CMS, with the most frequently observed peak values at the sampling point 22:30. The 8-week recovery in FCM distributions is considered as a marker for functional habituation of the stress-sensitive hormonal system to chronic stress, also reported by other authors (Willner Citation1997). It is known that stress can affect both the rate of metabolism and the rate of endogenous Cort secretion (Gronli et al. Citation2004; Grippo et al. Citation2005). Therefore, it is reasonable to consider possible variations in FCM concentrations due to these two processes. Taking into consideration the stress effect on intestinal transit time (Barone et al. Citation1990), the effect of CMS on excretion of FCM can be estimated indirectly by a lag between the time point of i.p. injection of dexamethasone and the sampling point, where the lowest FCM concentrations were measured. Correlation analysis on sampling points where diurnal peaks were measured and sampling points where lowest post-dexamethasone FCM concentrations were measured during the CMS did not reveal any significant interactions between either CMS groups or time of CMS exposure. Therefore, it is unlikely that the shift in the peak FCM distributions during CMS was due to altered intestinal transit time only.
Another parameter used to characterize the diurnal Cort variations is an absolute difference between peak and nadir FCM concentrations. In our study, a greater difference in this parameter was observed at week 2 of CMS ().
A major advantage of the CMS model is the possibility to evaluate individual hedonic status by the assessment of sucrose consumption with respect to physiological and behavioral reactivity. In our study, 20% of rats exposed to CMS show signs of stress resilience, which is defined by a persistent intake of sucrose solution during CMS. Twenty-five percent of CMS rats decreased in SCT by more than 40% indicating an anhedonic-like behavior. The remaining 55% of rats with a modest decrease in sucrose intake were assigned to the intermediate group.
Comparison of FCM concentrations in rats chronically exposed to unpredictable mild stressors did not reveal significant changes between CMS groups at the second week of CMS, thus indicating a normal stress reaction in all CMS animals. By week 4 of CMS, animals in the anhedonic-like group excreted significantly higher amounts of FCM at 5 from 7 measured sampling points compared to the stress-resilient group indicating persistent sensitivity to CMS-induced increase in endogenous Cort level in the resilient group and decreased endogenous Cort sensitivity in anhedonic-like animals. By week 8 of CMS, there were no differences between the CMS groups in diurnal FCM excretion, thus indicating the adaptive nature of stress reaction in individuals exposed to chronic stress.
Dexamethasone injections induced a significant suppression in FCM during the time course of CMS, indicating a conserved sensitivity of the HPA axis to exogenous glucocorticoids. Analysis of the lag in maximal dexamethasone effect revealed significantly delayed response in all animals at weeks 2 and 4 compared to the results obtained at week 8 of CMS. Lepschy et al. (Citation2007, Citation2010) reported a 6–9-h delay between Cort appearance in blood and increased FCM. Assuming the peak blood Cort concentration close to the time point when lighting in facilities changes from light to dark phase (18:00), the peak FCM concentrations were expected in samples collected between 21:00 and 24:00 with a 6-h delay. Indeed, the abundant peaks in FCM concentrations were obtained at the sampling point 22:30 before the initiation of the stress protocol and at week 8 of CMS.
From , it follows that according to the expected delay in FCM excretion, the peak Cort concentration in the blood can be expected either between 15:00 and 18:00 or 3 h later. The lowest FCM concentrations were measured most frequently at the sampling point 16:30 and could be related to the blood values between 9:00 and 12:00 with a 6-h delay or between 6:00 and 9:00 with a 9-h delay. Deviations in detection of the nadir FCM concentrations were observed in samples collected at week 4 of CMS in the resilient and intermediate groups, while at week 8 of CMS, all rats demonstrated a similar pattern to the baseline.
Analyzing the efficiency of DST calculated as a difference in FCM concentrations before and after dexamethasone injection (), the greatest differences in FCM were calculated for sampling point 22:30 in all CMS groups at week 8 of CMS and 3 h later at week 4 of CMS. In the resilient group the greatest differences during the experiment were observed at sampling point 22:30 on week 8. In both, the anhedonic-like and intermediate, groups the greatest differences we observed at sampling point 1:30 on week 2 (). Taken together, these observations indicate that studying the functional presence of negative feedback is most efficient by measuring the dexamethasone-induced suppression in FCM at the sampling point falling exactly 24 h after the sampling point where the FCM peak concentrations were observed with the present protocol of dexamethasone administration.
The advantage of using the animal models of affective diseases lies in the possibility to mimic only one parameter involved in the pathology of disease (Willner Citation1997). In the case of the CMS model, we investigate the effect of chronic stress. Our study has shown that habituation of the HPA hormonal system to chronic stress is not correlated with a hedonic status of individuals. However, animals with indices of anhedonia manifest higher FCM levels during a limited period of CMS protocol. Thus, a high level of endogenous glucocorticoids could be considered as a marker for certain stages in the development of long-lasting anhedonic-like condition. Moreover, we have shown that HPA axis sensitivity to high doses of exogenous glucocorticoid is not affected by stress. Despite our report on significant differences between resilient and anhedonic-like animals at some sampling points, this parameter would not be helpful to confirm a hedonic state of individuals due to the small differences between the groups and the relatively high variability within the groups. Nevertheless, the finding that animals exposed to chronic stress demonstrate differential, but persistent, sensitivity to relatively high dose of dexamethasone, and a positive response to DST independently of their hedonic status could be useful in the diagnosis of stress-related disorders. However, we acknowledge that sub-maximal doses of dexamethasone may reveal differential effects on CMS animals.
In conclusion, this study described the rhythmic activity of the HPA axis and its negative feedback regulation in the CMS model of depression. Results indicate the activation of the HPA axis in response to CMS and increased levels of FCM in animals exhibiting anhedonic-like symptoms by 4 weeks of CMS exposure. At the same time, the differences between anhedonic-like and stress-resilient rats were prominently recorded as a shift in peak FCM secretion during the diurnal cycle. Independently of the stress response, all animals demonstrate sensitivity to the exogenous glucocorticoid dexamethasone as an indication for the functional presence of the negative feedback mechanism, but the efficiency of DST significantly decreased in animals with symptoms of anhedonia during the time course of CMS. Thus, our results demonstrate that a disturbance of circadian rhythmicity of the HPA axis activity characterizes an initial state in the development of depression-like behavior and that this chronobiological abnormality, as well as the hypersecretion of corticosterone, is state, rather than trait, dependent.
Acknowledgements
The authors wish to express their gratitude to Kim Henningsen and Stine Dhiin Heide Hansen for running the CMS model of depression and Edith Klobetz-Rassam for excellent technical assistance with EIA. Also, we are grateful to David Farr and Buffy Illum for their careful reading of the manuscript. The project is supported by the regional foundation for the support of basic research in psychiatry (Region Midtjylland, Denmark) and the University of Veterinarian Medicine, Vienna, Austria.
Declaration of interest: The authors report no conflicts of interest. The authors alone are responsible for the content and writing of the paper.
References
- Antonijevic I. 2008. HPA axis and sleep: Identifying subtypes of major depression. Stress. 11:15–27.
- Antonijevic IA, Murck H, Frieboes RM, Steiger A. 2000. Sexually dimorphic effects of GHRH on sleep-endocrine activity in patients with depression and normal controls—part II: Hormone secretion. Sleep Res Online.. 3:15–21.
- Banki CM, Arato M, Papp Z, Rihmer Z, Kovacs Z. 1986. Associations among dexamethasone non-suppression and TRH-induced hormonal responses: Increased specificity for melancholia?. Psychoneuroendocrinology. 11:205–211.
- Barone FC, Deegan JF, Price WJ, Fowler PJ, Fondacaro JD, Ormsbee HSIII. 1990. Cold-restraint stress increases rat fecal pellet output and colonic transit. Am J Physiol. 258:G329–G337.
- Barrett EJ. 2009. Organization of endocrine control. In: Boron WF, Boulpaep EL. editors. Medical physiology. Philadelphia, PA: Saunders/Elsevier1003–1017.
- Calfa G, Kademian S, Ceschin D, Vega G, Rabinovich GA, Volosin M. 2003. Characterization and functional significance of glucocorticoid receptors in patients with major depression: Modulation by antidepressant treatment. Psychoneuroendocrinology. 28:687–701.
- Caroff S, Winokur A, Rieger W, Schweizer E, Amsterdam J. 1983. Response to dexamethasone in psychotic depression. Psychiatry Res.. 8:59–64.
- Carroll BJ. 1982. Clinical applications of the dexamethasone suppression test for endogenous depression. Pharmacopsychiatria.. 15:19–25.
- Checkley S. 1996. The neuroendocrinology of depression and chronic stress. Br Med Bull. 52:597–617.
- De Kloet ER, Vreugdenhil E, Oitzl MS, Joels M. 1998. Brain corticosteroid receptor balance in health and disease. Endocr Rev. 19:269–301.
- Deuschle M, Schweiger U, Weber B, Gotthardt U, Korner A, Schmider J, Standhardt H, Lammers CH, Heuser I. 1997. Diurnal activity and pulsatility of the hypothalamus–pituitary–adrenal system in male depressed patients and healthy controls. J Clin Endocrinol Metab. 82:234–238.
- Dinan TG. 1994. Glucocorticoids and the genesis of depressive illness. A psychobiological model. Br J Psychiatry. 164:365–371.
- Gold PW, Licinio J, Wong ML, Chrousos GP. 1995. Corticotropin releasing hormone in the pathophysiology of melancholic and atypical depression and in the mechanism of action of antidepressant drugs. Ann NY Acad Sci. 771:716–729.
- Grippo AJ, Francis J, Beltz TG, Felder RB, Johnson AK. 2005. Neuroendocrine and cytokine profile of chronic mild stress-induced anhedonia. Physiol Behav. 84:697–706.
- Gronli J, Murison R, Bjorvatn B, Sorensen E, Portas CM, Ursin R. 2004. Chronic mild stress affects sucrose intake and sleep in rats. Behav Brain Res. 150:139–147.
- Henningsen K, Andreasen JT, Bouzinova EV, Jayatissa MN, Jensen MS, Redrobe JP, Wiborg O. 2009. Cognitive deficits in the rat chronic mild stress model for depression: Relation to anhedonic-like responses. Behav Brain Res. 198:136–141.
- Jarque CM, Bera AK. 1980. Efficient tests for normality, homoscedasticity and serial independence of regression residuals. Econ Lett. 6:255–259.
- Jayatissa MN, Bisgaard C, Tingstrom A, Papp M, Wiborg O. 2006. Hippocampal cytogenesis correlates to escitalopram-mediated recovery in a chronic mild stress rat model of depression. Neuropsychopharmacology. 31:2395–2404.
- Kathol RG, Jaeckle RS, Lopez JF, Meller WH. 1989. Pathophysiology of HPA axis abnormalities in patients with major depression: An update. Am J Psychiatry. 146:311–317.
- Koenigsberg HW, Teicher MH, Mitropoulou V, Navalta C, New AS, Trestman R, Siever LJ. 2004. 24-h Monitoring of plasma norepinephrine, MHPG, cortisol, growth hormone and prolactin in depression. J Psychiatr Res. 38:503–511.
- Lepschy M, Touma C, Palme R. 2010. Faecal glucocorticoid metabolites: How to express yourself—comparison of absolute amounts versus concentrations in samples from a study in laboratory rats. Lab Anim. 44:192–198.
- Lepschy M, Touma C, Hruby R, Palme R. 2007. Non-invasive measurement of adrenocortical activity in male and female rats. Lab Anim. 41:372–387.
- Linkowski P, Mendlewicz J, Kerkhofs M, Leclercq R, Golstein J, Brasseur M, Copinschi G, Van CE. 1987. 24-hour profiles of adrenocorticotropin, cortisol, and growth hormone in major depressive illness: Effect of antidepressant treatment. J Clin Endocrinol Metab. 65:141–152.
- Maes M. 1999. Major depression and activation of the inflammatory response system. Adv Exp Med Biol. 461:25–46.
- Nemenyi PB. 1963. Distribution-free multiple comparisons. Princeton University: Doctoral thesis.
- Nemeroff CB, Vale WW. 2005. The neurobiology of depression: Inroads to treatment and new drug discovery. J Clin Psychiatry. 66:5–13.
- Palacios R, Campo A, Henningsen K, Verhoye M, Poot D, Dijkstra J, Van AJ, Benveniste H, Sijbers J, Wiborg O, Van der LA. 2011. Magnetic resonance imaging and spectroscopy reveal differential hippocampal changes in anhedonic and resilient subtypes of the chronic mild stress rat model. Biol Psychiatry. 70:449–457.
- Pariante CM. 2006. The glucocorticoid receptor: Part of the solution or part of the problem?. J Psychopharmacol. 20:79–84.
- Pariante CM, Miller AH. 2001. Glucocorticoid receptors in major depression: Relevance to pathophysiology and treatment. Biol Psychiatry. 49:391–404.
- Pittenger C, Duman RS. 2008. Stress, depression, and neuroplasticity: A convergence of mechanisms. Neuropsychopharmacology.. 33:88–109.
- Rubin RT, Poland RE, Lesser IM, Winston RA, Blodgett AL. 1987. Neuroendocrine aspects of primary endogenous depression. I. Cortisol secretory dynamics in patients and matched controls. Arch Gen Psychiatry. 44:328–336.
- Shapiro SS, Wilk MB. 1965. An analysis of variance test for normality (complete samples). Biometrika. 52:591–611.
- Stetler C, Miller GE. 2011. Depression and hypothalamic–pituitary–adrenal activation: A quantitative summary of four decades of research. Psychosom Med. 73:114–126.
- Streeten DH, Anderson GHJr, Dalakos TG, Seeley D, Mallov JS, Eusebio R, Sunderlin FS, Badawy SZ, King RB. 1984. Normal and abnormal function of the hypothalamic–pituitary–adrenocortical system in man. Endocr Rev. 5:371–394.
- Surget A, Tanti A, Leonardo ED, Laugeray A, Rainer Q, Touma C, Palme R, Griebel G, Ibarguen-Vargas Y, Hen R, Belzung C. 2011. Antidepressants recruit new neurons to improve stress response regulation. Mol Psychiatry. 16:1177–1188.
- Touma C, Bunck M, Glasl L, Nussbaumer M, Palme R, Stein H, Wolferstatter M, Zeh R, Zimbelmann M, Holsboer F, Landgraf R. 2008. Mice selected for high versus low stress reactivity: A new animal model for affective disorders. Psychoneuroendocrinology. 33:839–862.
- Touma C, Fenzl T, Ruschel J, Palme R, Holsboer F, Kimura M, Landgraf R. 2009. Rhythmicity in mice selected for extremes in stress reactivity: Behavioural, endocrine and sleep changes resembling endophenotypes of major depression. PLoS. One.. 4:e4325.
- Touma C, Palme R, Sachser N. 2004. Analyzing corticosterone metabolites in fecal samples of mice: A noninvasive technique to monitor stress hormones. Horm Behav. 45:10–22.
- Touma C, Sachser N, Mostl E, Palme R. 2003. Effects of sex and time of day on metabolism and excretion of corticosterone in urine and feces of mice. Gen Comp Endocrinol. 130:267–278.
- Willner P. 1997. Validity, reliability and utility of the chronic mild stress model of depression: A 10-year review and evaluation. Psychopharmacology (Berl). 134:319–329.
- Willner P. 2005. Chronic mild stress (CMS) revisited: Consistency and behavioural-neurobiological concordance in the effects of CMS. Neuropsychobiology.. 52:90–110.
- Wong ML, Kling MA, Munson PJ, Listwak S, Licinio J, Prolo P, Karp B, McCutcheon IE, Geracioti TD, Jr., DeBellis MD, Rice KC, Goldstein DS, Veldhuis JD, Chrousos GP, Oldfield EH, McCann SM, Gold PW. 2000. Pronounced and sustained central hypernoradrenergic function in major depression with melancholic features: Relation to hypercortisolism and corticotropin-releasing hormone. Proc Natl Acad Sci USA. 97:325–330.