Abstract
Spontaneous aneurysmal subarachnoid haemorrhage (SAH) is a cause of stroke, which constitutes a severe trauma to the brain and may lead to serious long-term medical, psychosocial and endocrinological sequelae. Adrenocorticotrophic hormone deficiency, which is considered to occur in up to 20% of all survivors, is a possible consequence of bleeding. Moreover, preliminary data suggest that a poor psychosocial outcome in SAH survivors is linked to alterations in cortisol secretion. Despite these findings, investigation of diurnal cortisol profiles and the cortisol awakening response (CAR) in chronic SAH patients has not been done so far. In this study, basal serum cortisol and salivary cortisol concentration profiles were investigated in 31 SAH patients more than 1 year after the acute event and in 25 healthy controls. Additionally, low-dose dexamethasone (DEX) suppression tests were conducted, and sensitivity to stress was measured with a psychometric questionnaire (NeuropatternTM). Although significantly higher salivary cortisol concentrations were observed on waking in SAH patients (p = 0.013, ANOVA), without a CAR change, total serum cortisol concentrations were blunted, but only in patients with high levels of perceived stress (SAH high stress: 337 nmol/l, SAH low stress: 442 nmol/l, controls: 467 nmol/l; Controls vs. SAH high stress p = 0.018). DEX suppression of cortisol secretion was not significantly different between patients and controls. The results indicate that total (serum) and free (salivary) cortisol concentrations give different information about cortisol availability in patients after aneurysmal SAH. Enhanced free cortisol concentrations may reflect a meaningful biological coping mechanism in SAH patients.
Introduction
Aneurysmal subarachnoid haemorrhage (SAH) is acute bleeding into the subarachnoid space, caused by the rupture of an intracranial aneurysm (Rinkel et al. Citation1998). It affects about 3–10 of 100,000 people each year (Kolominsky-Rabas et al. Citation1998), predominantly in the fourth to sixth decade of life. Modern interventional methods to occlude the ruptured aneurysm, microneurosurgical clipping and neuroradiological coil embolization, as well as highly specialized intensive care treatment, have greatly decreased morbidity and mortality of this once-fatal disease (Lovelock et al. Citation2010). Yet, in the long term, patients after SAH frequently report persistent impairments such as lack of initiative, fatigue, irritability, personality change, loss of interests and emotional disturbances, even in the absence of any neurological deficit (Al-Khindi et al. Citation2010; Rinkel and Algra Citation2011). How aneurysmal SAH causes long-term psychosocial impairment is not yet fully elucidated. Factors relating to the severity of the haemorrhage itself (Hütter et al. Citation2001) but also post-ictal events, leading to post-traumatic stress disorder (Baisch et al. Citation2011), seem to play a role.
In recent years, a growing body of evidence indicates that disturbances of the hypothalamo–pituitary–adrenal (HPA) axis also occur in a considerable proportion of SAH survivors. The authors of a recent meta-analysis summarizing the data on this topic concluded that after acute SAH, cortisol levels may initially be supranormal, but decrease towards normal levels over time (Vespa Citation2011). During the months to years after SAH, pituitary deficiency may occur in one out of three patients. Preliminary data indicate that a deranged HPA axis in SAH patients may affect the physical and psychosocial outcome. In patients investigated in the acute phase of aneurysmal SAH, an abnormal diurnal variation of free cortisol levels, but not early morning (08:00 h) serum cortisol concentration or total daily cortisol availability (calculated free serum cortisol), correlated with poor outcome and a longer hospital stay compared with those patients with normal diurnal variation (Poll et al. Citation2010). In another study, Kreitschmann-Andermahr et al. (Citation2007) showed an association between low basal serum cortisol concentrations, poor quality of life and psychiatric syndromes such as depression in SAH patients more than 1 year after the acute event. In contrast to this finding, measures of responses of the HPA axis to hypoglycaemia in conventional endocrine function testing [insulin tolerance test (ITT)] were not related to psychological well-being in these patients. Thus, it can be inferred that the derangements of the HPA axis in some SAH patients are so subtle that the endocrine reaction to a strong pharmacological stimulus such as the ITT is preserved. This hypothesis is supported by a recent study of Klose et al. (Citation2010) who found secondary hypocortisolism as diagnosed by means of the ITT to be uncommon after aneurysmal SAH. Nevertheless, a disturbed course of diurnal cortisol output as has been shown in SAH patients in the acute phase (Poll et al. Citation2010; Shin et al. Citation2011) and an altered feedback sensitivity of the HPA axis (Fries et al. Citation2005), which has not been investigated in SAH patients so far, could still contribute to psychological symptoms of SAH patients in the long term. Two theories serve this hypothesis. Firstly, damage to the hypothalamo–pituitary area of the brain as a consequence of the haemorrhage, as observed in SAH fatalities (Crompton Citation1963), might induce a permanent dysregulation of cortisol secretion. This, in turn, could lead to psychiatric symptoms that are linked to a dysfunctional HPA axis. Another mechanism involves chronic stress as a result of the traumatic event and its social and work-related sequelae, which in turn could lead to the typical stress-related disorders in these patients. The latter explanation is the line of reasoning often employed to explain the frequent hormonal dysregulations, particularly hypocortisolism and altered feedback reactivity, in patients with stress-related disorders of other aetiology (Heim et al. Citation2000; Fries Citation2008).
In order to test our hypothesis of disturbed diurnal cortisol secretion and altered HPA-axis feedback sensitivity in SAH patients after the acute phase of the illness, we assessed basal serum cortisol concentrations, diurnal cortisol secretion and cortisol levels after awakening, including the cortisol awakening response (CAR; Pruessner et al. Citation2003; Clow et al. Citation2010) in saliva. We also conducted a low-dose dexamethasone (DEX) suppression test in SAH patients more than 1 year after the bleeding and in age- and sex-matched healthy controls. Sensitivity to stress and stress resilience were measured with psychometric questionnaires (NeuropatternTM; Hellhammer and Hellhammer Citation2008).
Materials and methods
Patients
All patients who had sustained an aneurysmal SAH at least 1 year before entering into the study were screened for eligibility during their regular clinical visits to the outpatient clinic of the Department of Neurosurgery, RWTH Aachen University Hospital over a time span of 18 months. Contemporaneously, controls were recruited via word-of-mouth recommendation of co-workers at the University Hospital Aachen and via the homepage of the University Hospital Aachen. Exclusion criteria for both groups were pregnancy, medication during the testing period known to affect cortisol concentrations, acute or chronic inflammatory diseases, glaucoma, osteoporosis, history of traumatic brain injury or brain tumours and age below 18 years and over 65 years. Controls were excluded if a free clinical interview revealed symptoms of depression or other mental disorders or known pituitary disease. Additionally, SAH patients were excluded when there was any known history of pituitary insufficiency prior to the SAH. Based on these inclusion and exclusion criteria, we were able to investigate 31 patients in the chronic phase after SAH (i.e. at least 1 year after the acute event) as well as 25 age-matched healthy participants (see ). The study was approved by the Ethics Committee of the University Hospital of the University of Technology, RWTH Aachen and was conducted according to the Declaration of Helsinki. Written informed consent was given in all cases.
Table I. Descriptive data.
Severity of the SAH at hospital admission was rated in accordance with the Hunt and Hess (HH) grading (Hunt and Hess Citation1968) and bleeding severity according to the Fisher computed tomography (FCT) scale (Fisher et al. Citation1980). Neurological outcome at the time of testing was rated using the Glasgow Outcome Scale (Jennett and Bond Citation1975). Details of patients and controls are given in .
The ruptured aneurysm could be identified in all patients. Most commonly, the aneurysm was located at the anterior communicating artery (n = 14) and at the middle cerebral artery (n = 8). Occlusion of the aneurysm was performed microsurgically in 26 patients, and 5 patients underwent endovascular coil embolization. Cerebral vasospasm, defined as mean cerebral blood flow in the middle cerebral and/or the internal carotid artery >120 cm/s measured by transcranial Doppler sonography, was detected in 15 patients. Hydrocephalus in the acute phase of SAH was present in nine patients.
Assessments
Both patients and controls underwent the same procedure. A venous blood sample (7.5 ml) was drawn from an antecubital vein at 08:00 h from each participant to assess basal fasting cortisol concentrations. Subsequently, all participants received a study pack containing pre-labelled sampling material for saliva collection (standard reaction tubes) and a capsule containing 0.25 μg DEX. The study subjects were asked to perform saliva sampling at home on 2 consecutive work-free days directly after awakening, +30,+45 and +60 min after awakening (for awakening response) as well as at 08:00, 11:00, 15:00 and 20:00 h (for diurnal pattern). All participants noted the exact times of sampling on a form, as well as their awakening time. On the following weekend or other work-free day, the participants were asked to complete the same sampling routine again after taking 0.25 μg DEX the evening before sampling (at 23:00 h). As a reminder of DEX intake, participants were contacted by telephone, short message service or e-mail, and depending on the preferences of the contacted person, on the evening prior to DEX intake, saliva was collected by passive drool into the tube. Smoking, brushing teeth, eating and drinking were prohibited 30 min prior to sampling. Stressors that could not be avoided, such as sports activity or any type of physical or psychological arousal, were noted. All instructions were given in a one-to-one talk, and all participants received a handout comprising instructions on saliva sampling as well as a protocol sheet for noting down the exact times of sampling.
Additionally, all patients completed the NeuropatternTM Diagnostic Kit (DAACRO GmbH, Trier, Germany). This diagnostic system differentiates between 23 endophenotypes of the stress response, which are ascertained by biological, psychological and symptom measures. The questionnaires encompass items regarding physical reactions to stress, some psychological state and trait characteristics of the patient, pre- and postnatal influences, chronic stress and depression. Additionally, the information gained from salivary cortisol measures and the DEX suppression test is included in the diagnosis.
Specimen handling and assays
Saliva samples were immediately frozen in the participant's domestic freezer, and once the sampling had been completed, they were stored in a freezer of the central laboratory of RWTH Aachen University Hospital at − 20°. After completion of the study protocol, all samples were shipped to the laboratory of the Department of Psychophysiology at Trier University and were analysed. After thawing, samples were centrifuged at 2000g for 6 min, which resulted in a clear supernatant of low viscosity. Cortisol concentrations were determined employing a competitive solid-phase time-resolved fluorescence immunoassay with fluorometric end-point detection (DELFIA, PerkinElmer Inc., Waltham, USA); 100 μl of saliva was used for duplicate analysis (50 μl per well). The intra-assay coefficient of variation was between 4.0% and 6.7%, and the corresponding inter-assay coefficients of variation were between 7.1% and 9.0%; assay sensitivity was 0.173 nmol/l.
Blood samples were analysed at the central laboratory of the University Hospital RWTH Aachen directly after sampling. Serum cortisol was measured via an automated immunometric chemiluminescence assay (ADVIA Centaur, Bayer Health Care Diagnostics, Fernwald, Germany, reference value: 171–536 nmol/l, and assay sensitivity: 1 μg/dl).
Statistical methods
For data analysis, IBM SPSS statistics for Windows version 20.0 (SPSS Inc., Chicago, IL, USA) was used. Descriptive statistics of interval-scaled data are expressed as mean and standard deviation (SD). To test the strength of linear dependence between the two variables, correlation coefficients were calculated (Spearman correlation for ranked variables and Pearson correlation for variables measured on interval scales). To test group differences of independent measures, Student's t-tests or Mann–Whitney U-tests were conducted as appropriate. For the comparison of cortisol secretion patterns, three-way analyses of variance with repeated measures (RM-ANOVA) were calculated separately for diurnal cortisol pattern and awakening concentrations. Between-subjects factors were groups (SAH patients/controls) and DEX administration (yes/no), while the (repeated) within-subjects factor was time of sampling. In case of statistically significant results with ANOVA, Tukey's honestly significant difference (HSD) test was used as the post-hoc test. Paired sample t-tests of the cortisol data per time point did not show any significant differences between day 1 and day 2; therefore, baseline measurements from the first 2 days were merged in order to gain mean salivary cortisol concentrations on waking and diurnal profile. In subjects who did not show an awakening response on 1 of the 2 days, we assumed that they were awake prior to sampling, without recognizing it. Thus, we only used the profile with the normal CAR. In order to create groups of stressed and non-stressed patients and controls, a two-step cluster analysis was carried out. The CAR was calculated as mean increase in cortisol values from awakening to the values 30–45 min later [‘mean increase (MnInc)’: ((t 30+t 45)/2) − t 0; Clow et al. Citation2010].
Results
Cortisol measurements
Basal serum cortisol concentrations were available for 54 subjects (31 patients and 23 controls); diurnal salivary cortisol profiles were available for all patients and controls, and the DEX suppression test was done in all patients and 23 controls. All participants stated in their questionnaires that the first saliva sample was taken immediately after awakening. Three participants did not adhere to the time schedule for saliva sampling on 1 of the 2 basal sampling days. Those three profiles were not included in the analysis. In 11 patients and 7 controls, no effect of the DEX administration could be seen (non-responders). As all of these participants stated that they took the DEX at 23:00 h the evening before, they were included in the analysis.
In the SAH group, serum cortisol concentrations were similar to the cortisol concentrations of controls (SAH: mean ± SD = 401.4 ± 126.1 nmol/l, controls: mean ± SD = 466.8 ± 144.4 nmol/l, t-test, p = 0.082). In SAH patients, serum cortisol concentrations were not associated with measures of severity of the acute illness (length of hospital stay: Pearson correlation r = 0.11, p = 0.55; FCT r = 0.09, p = 0.67; HH r = − 0.20, p = 0.27, both Spearman correlation). However, SAH patients exhibited significantly greater salivary cortisol concentrations in the morning (baseline concentrations: F (1,54) = 6.548, p = 0.013, ANOVA) than controls (). There was no evident difference in the CAR (MnInc) between the SAH group and the controls (). This was confirmed by calculating the CAR for each participant and by comparing the group means (SAH patients: 2.91 ± 4.50 nmol/l; Controls: 3.39 ± 3.95 nmol/l; t-test, p = 0.69). The difference in salivary cortisol concentrations between the SAH and control groups, as observed in the morning, did not persist for the rest of the day: the diurnal secretion of salivary cortisol was not significantly elevated in SAH patients (baseline diurnal profile F (1,53) = 3.080, p = 0.085, ANOVA; ). After administration of DEX, both the cortisol concentrations after awakening and the diurnal profile revealed significantly blunted cortisol concentrations in patients and controls (main effect, cortisol concentrations after awakening F (1,103) = 33.52 and diurnal profile F (1,99) = 35.4, both p < 0.0001, ANOVA). Statistically significant interactions between group (SAH/controls) and DEX (suppression yes/no) were observed neither for cortisol concentrations after awakening (F (1,103) = 1.727, p = 0.192, ANOVA) nor for the diurnal profile (F (1,99) = 0.007, p = 0.936, ANOVA; compare ).
Figure 1. Salivary cortisol concentrations in patients after subarachnoid haemorrhage (SAH patients) and controls. (a) Post-awakening. Patients exhibited significantly greater salivary cortisol concentrations than controls; p = 0.013, ANOVA; n = 31 SAH patients, n = 25 controls. (b) Diurnal variation. Profiles did not differ between groups; p = 0.14, ANOVA, n = 31 SAH patients, n = 24 controls. Values are mean salivary cortisol concentrations for each group; whiskers represent 95% confidence intervals. Time scale (a) is in minutes, (b) is time of day, h.
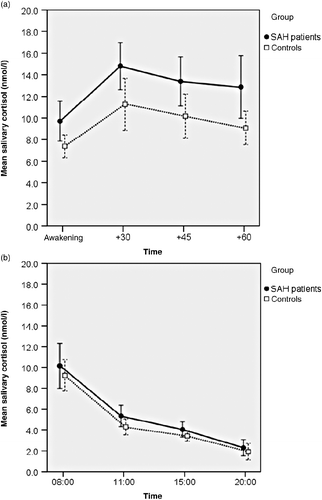
Figure 2. Salivary cortisol concentrations in patients after subarachnoid haemorrhage (SAH patients) categorized as low or high stress and controls. Stress level was determined by NeuropatternTM. (a) Post-awakening. Salivary cortisol concentrations in the SAH low-stress group (n = 19), but not the high-stress group (n = 12), were significantly greater than those in controls (n = 25); p = 0.022, ANOVA; post-hoc Tukey-HSD: p = 0.016. (b) Diurnal variation. Diurnal cortisol concentrations for patients after SAH with high stress (n = 12) or low stress (n = 19) and controls (n = 24). There were no significant differences among the three groups (p = 0.22, ANOVA). Values are mean salivary cortisol concentrations for each group; whiskers represent 95% confidence interval. Time scale (a) is in minutes, (b) is time of day, h.
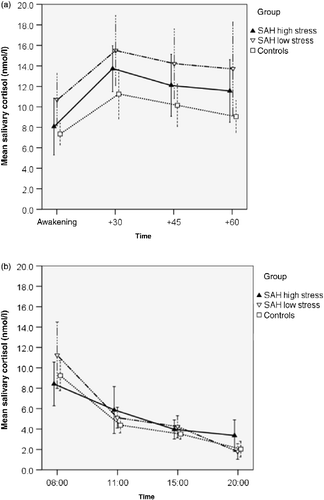
Figure 3. Salivary cortisol concentrations after administration of 0.25 μg DEX the evening before sampling (23:00 h) in patients after subarachnoid haemorrhage (SAH patients) and controls. a: Post-awakening, n = 31 SAH patients, n = 23 controls. b: Diurnal variation, n = 31 SAH patients, n = 23 controls. No statistically significant interaction between group (SAH/controls) and DEX (suppression yes/no: data for ‘no DEX’ shown are in Figure 1) was observed (Post-awakening: F (1,103) = 1.727, p = 0.19, ANOVA; diurnal profile: F (1,99) = 0.007, p = 0.94, ANOVA; compare Figure 1a,b). Values are mean salivary cortisol concentrations for each group; whiskers represent 95% confidence interval. Time scale (a) is in minutes, (b) is time of day, h.
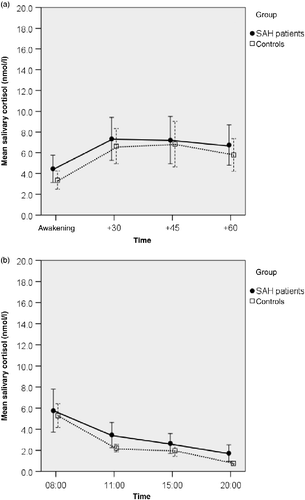
Stress load: NeuropatternTM
With respect to stress load, SAH patients showed more symptoms of stress-related physical complaints than the control group. After Bonferroni correction, 4 of the 76 scales differed between both the groups: patients achieved higher scores in excitability (patients: 43.7, controls: 22.0, p = 0.0003, t-test) and lethargy (patients: 44.7, controls: 17.2, p = 0.0001, t-test), and lower scores in vigilance (patients: 49.7, controls: 72.4, p = 0.0001, t-test) and cognitive performance (patients: 32.2, controls: 57.8, p = 0.0001, t-test). Based on these scales, a two-step cluster analysis was carried out to discriminate subgroups of patients with respect to stress load. The analysis revealed two clusters. The first cluster comprised 12 patients with a high-stress load (excitability: 61.2, lethargy: 71.4, vigilance: 27.8, performance: 17.5) and a second cluster with the remaining 19 patients, and all of the controls (low-stress load: excitability: 26.6, lethargy: 21.8, vigilance: 68.6, performance: 50.8; each comparison by t-test p < 0.0001). For further analyses, three subgroups were compared with respect to the endocrine measures: SAH high stress, SAH low stress and controls.
Cortisol and stress load
First, serum cortisol concentrations were compared among the three groups, which revealed significantly different serum cortisol values (F (2,51) = 4.15, p = 0.021, ANOVA). Interestingly, a post-hoc test showed that only highly stressed SAH patients had significantly lower serum cortisol values than controls (p = 0.018, Tukey-HSD), while no such difference was observed for the low-stressed SAH patients and controls (p = 0.816, Tukey-HSD) or between low- and high-stressed SAH patients (p = 0.077, Tukey-HSD) (mean ± SD values of controls: 466.8 ± 144.4 nmol/l; SAH low stress: 442.4 ± 138.5 nmol/l; SAH high stress: 336.50 ± 66.8 nmol/l).
Second, salivary cortisol concentrations after awakening were clearly elevated in the SAH low-stress group when compared to controls (F (2,53) = 4.093, p = 0.022, ANOVA. Tukey-HSD: p = 0.016), while neither the difference between the SAH high-stress group and controls nor the difference between high-stress and low-stress patients reached statistical significance (). The CAR was not statistically significantly different between the high- and low-stress SAH groups and the controls (: CAR, low- and high-stress SAH patients: 4.13 ± 6.63 nmol/l, 4.83 ± 3.72 nmol/l, respectively; Controls: 3.34 ± 4.62; ANOVA, p = 0.71). Also for the diurnal profile, no difference among the three groups was detected (F (2,52) = 1.565, p = 0.219, ANOVA; ).
Third, cortisol concentrations after DEX showed a similar picture, with higher cortisol concentrations after awakening in the SAH low-stressed group (). However, group differences were not significant (F (2,48) = 0.607, p = 0.549, ANOVA), Also, diurnal salivary cortisol profiles after DEX administration did not differ among the three groups (F (2,45) = 1.199, p = 0.311, ANOVA; ).
Figure 4. Salivary cortisol concentrations after administration of 0.25 μg DEX the evening before sampling (23:00 h) in patients after subarachnoid haemorrhage (SAH patients) categorized as low or high stress, and controls. Stress level was determined by NeuropatternTM. There were no statistically significant group differences (a) post-awakening. (F (2,48) = 0.607, p = 0.55, ANOVA) or (b) in the diurnal cortisol profiles (F (2,45) = 1.199, p = 0.31, ANOVA). Values are mean salivary cortisol concentrations for each group; whiskers represent 95% confidence interval. Time scale (a) is in minutes, (b) is time of day, h.
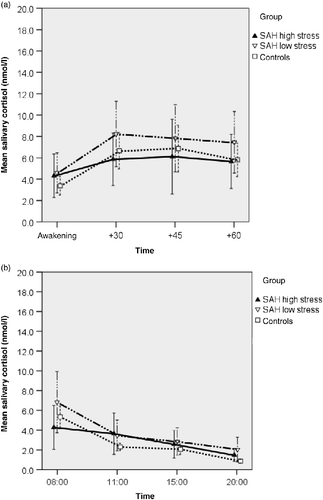
SAH patients with low and high stress did not differ with respect to HH grading scores (median: low stress: 2.0, high stress: 2.0, p = 0.89, U-test), Fisher CT-score (median: low stress: 2.5, high stress: 2.0, p = 0.31, U-test) or length of stay in the hospital (mean: low stress: 22.5, high stress: 24.5, p = 0.4, t-test).
Discussion
In accordance with previous research studies, we found a trend towards lower serum cortisol concentrations in patients in the chronic state after aneurysmal SAH, which reached significance in SAH patients with a high-stress load according to the NeuropatternTM. This difference in cortisol concentrations was not associated with clinical measures of severity of the acute haemorrhage, giving rise to the hypothesis that stress also plays a major role in blunting the cortisol acrophase in serum.
In contrast to the normal to low serum cortisol values, in this study, the free (salivary) cortisol concentrations after awakening were elevated in SAH patients, which was attributable to high cortisol concentrations in low-stressed SAH patients. These findings were unexpected and require further investigation. One possible mechanism to explain this result may pertain to altered cortisol binding as an adaptive mechanism in highly stressed SAH patients. In serum, cortisol is bound to corticosteroid-binding globulin (CBG), albumin and erythrocytes, leading to a fraction of about 10% unbound cortisol. Neither in this nor in other studies concerning chronic SAH patients have CBG data ever been investigated, and CBG data from the acute phase of brain trauma yielded different results (Savaridas et al. Citation2004; Poll et al. Citation2010). Still, it may be speculated that enhanced free cortisol concentrations may reflect a meaningful biological coping mechanism in SAH patients. The brain is the organ with the highest energy consumption, without having own energy stores. Thus, the brain enhances its own glucose supply via activation of the HPA axis (Fehm et al. Citation2006). A down-regulation of CBG level and consequent increased availability of free cortisol could help SAH patients to optimize the energy supply for the brain, and to cope with the increased daily physical and psychosocial challenges after the bleeding. This possibility is supported by animal data, in which a down-regulation of CBG was observed in acute and severely stressed animals (Garrel Citation1996), and chronic social stress led to reduced concentrations of CBG (Spencer et al. Citation1996).
Another unexpected result is that in this study the CAR was clearly not different between the SAH and control groups, and also the diurnal cortisol output was similar between SAH patients and controls. Recently, it has been supposed that the CAR is associated with the regaining of arousal after awakening, and there is evidence that the CAR may be a ‘kick-starter’ for the day (Clow et al. Citation2010). Additionally, a higher CAR is associated with less fatigue (Adam et al. Citation2006). However, here we found greater salivary cortisol levels in patients in the first complete hour after awakening, including the first measurement after awakening. Hence, no difference in the CAR was observed. Furthermore, salivary cortisol concentrations did not show differences among groups after DEX administration, indicating similar sensitivity to glucocorticoid negative feedback.
A possible explanation of the paradox of reduced serum cortisol concentrations in patients who are under chronic stress is that continuous stress seems to initially result in elevated cortisol concentrations and later in a decrease in cortisol, with low concentrations in serum and saliva (Hellhammer and Wade Citation1993). This phenomenon has been reported in a series of studies, and in most of these studies low cortisol concentrations were associated with fatigue, low vigilance and excitability (Heim et al. Citation2000), as observed in this study. Recently, Fries et al. (Citation2005) argued that a long lasting elevation of cortisol concentrations under chronic stress impairs physical and mental health. Thus, the organism may down-regulate cortisol concentrations to protect cardiovascular and metabolic functioning. Hence, the decrease in free and total cortisol concentrations may be a consequence of the severity and chronicity of stress. Consequently, it is expected that cortisol concentrations in the low-stress SAH group may follow the same time course, and eventually reach the same end point.
Although this study generated some unexpected findings, the data indicate that stress is an important mediator of HPA dysregulation in SAH patients. This notion is supported by a recent study finding that the traumatic quality of a SAH lies in post-ictal events, related to patients' adjustment and life changes after the haemorrhage, rather than ictal events (Baisch et al. Citation2011). As observed in other stress-related disorders, cortisol concentrations may follow a bi-phasic time course with an initial phase of elevated and a later phase of lowered glucocorticoid concentrations. Chronic stress seems to be a relevant initiator and promoter of this time course. Our observation of a differential regulation of free (salivary) and total (serum) cortisol concentrations indicates that CBG is a possible mediator of these effects. It is important to note that, due to the lack of CBG measurements in this study, the results of this investigation should be taken as a starting point for further studies, including CBG measurements with free and total cortisol concentrations, as well as the time course and the stress load of the patients.
The results of this study indicate that total and free serum cortisol concentrations give different information about the HPA axis in patients after aneurysmal SAH and give rise to the conclusion that the investigation of total serum cortisol concentrations either alone, or in combination with endocrinological function testing, may not be adequate for diagnosing secondary adrenal insufficiency in this patient group.
Acknowledgement
EP received a scholarship from the Konrad-Adenauer-Stiftung (KAS) for conducting this study.
Declaration of interest : The authors report no conflicts of interest. The authors alone are responsible for the content and writing of the paper.
References
- Adam EK, Hawkley LC, Kudielka BM, Cacioppo JT. 2006. Day-to-day dynamics of experience—cortisol associations in a population-based sample of older adults. Proc Natl Acad Sci USA. 103 45: 17058–17063.
- Al-Khindi T, Macdonald RL, Schweizer TA. 2010. Cognitive and functional outcome after aneurysmal subarachnoid hemorrhage. Stroke. 41 8: e519–e536.
- Baisch SB, Schenk T, Noble AJ. 2011. What is the cause of post-traumatic stress disorder following subarachnoid haemorrhage?. Post-ictal events are key. Acta Neurochir. 153 4: 913–922.
- Clow A, Hucklebridge F, Stalder T, Evans P, Thorn L. 2010. The cortisol awakening response: More than a measure of HPA axis function. Neurosci Biobehav Rev. 35 1: 97–103.
- Crompton MR. 1963. Hypothalamic lesions following the rupture of cerebral berry aneurysms. Brain. 86:301–314.
- Fehm HL, Kern W, Peters A. 2006. The selfish brain: Competition for energy resources. Prog Brain Res. 153:129–140.
- Fisher CM, Kistler JP, Davis JM. 1980. Relation of cerebral vasospasm to subarachnoid hemorrhage visualized by computerized tomographic scanning. Neurosurgery. 6 1: 1–9.
- Fries E. 2008. Hypocortisolemic disorders. In: Hellhammer DH, Hellhammer J. editors. Stress: The brain-body connection. Basel: Karger60–77.
- Fries E, Hesse J, Hellhammer J, Hellhammer DH. 2005. A new view on hypocortisolism. Psychoneuroendocrinology. 30 10: 1010–1016.
- Garrel DR. 1996. Corticosteroid-binding globulin during inflammation and burn injury: Nutritional modulation and clinical implications. Horm Res. 45 3–5: 245–251.
- Heim C, Ehlert U, Hellhammer DH. 2000. The potential role of hypocortisolism in the pathophysiology of stress-related bodily disorders. Psychoneuroendocrinology. 25 1: 1–35.
- Hellhammer DH, Hellhammer J. 2008. Stress: The brain-body connection. Basel: Karger.
- Hellhammer DH, Wade S. 1993. Endocrine correlates of stress vulnerability. Psychother Psychosom. 60 1: 8–17.
- Hunt WE, Hess RM. 1968. Surgical risk as related to time of intervention in the repair of intracranial aneurysms. J Neurosurg. 28 1: 14–20.
- Hütter BO, Kreitschmann-Andermahr I, Gilsbach JM. 2001. Health-related quality of life after aneurysmal subarachnoid hemorrhage: Impacts of bleeding severity, computerized tomography findings, surgery, vasospasm, and neurological grade. J Neurosurg. 94 2: 241–251.
- Jennett B, Bond M. 1975. Assessment of outcome after severe brain damage. Lancet. 1 7905: 480–484.
- Klose M, Brennum J, Poulsgaard L, Kosteljanetz M, Wagner A, Feldt-Rasmussen U. 2010. Hypopituitarism is uncommon after aneurysmal subarachnoid haemorrhage. Clin Endocrinol (Oxf). 73 1: 95–101.
- Kolominsky-Rabas PL, Sarti C, Heuschmann PU, Graf C, Siemonsen S, Neundoerfer B, Katalinic A, Lang E, Gassmann KG, von Stockert TR. 1998. A prospective community-based study of stroke in Germany—the Erlangen Stroke Project (ESPro): Incidence and case fatality at 1, 3, and 12 months. Stroke. 29 12: 2501–2506.
- Kreitschmann-Andermahr I, Poll E, Hutter BO, Reineke A, Kristes S, Gilsbach JM, Saller B. 2007. Quality of life and psychiatric sequelae following aneurysmal subarachnoid haemorrhage: Does neuroendocrine dysfunction play a role?. Clin Endocrinol (Oxf). 66 6: 833–837.
- Lovelock CE, Rinkel GJE, Rothwell PM. 2010. Time trends in outcome of subarachnoid hemorrhage: Population-based study and systematic review. Neurology. 74 19: 1494–1501.
- Poll EM, Boström A, Bürgel U, Reinges MH, Hans FJ, Gilsbach JM, Kreitschmann-Andermahr I. 2010. Cortisol dynamics in the acute phase of aneurysmal subarachnoid hemorrhage: Associations with disease severity and outcome. J Neurotrauma. 27 1: 189–195.
- Pruessner JC, Kirschbaum C, Meinlschmid G, Hellhammer DH. 2003. Two formulas for computation of the area under the curve represent measures of total hormone concentration versus time-dependent change. Psychoneuroendocrinology. 28 7: 916–931.
- Rinkel GJ, Algra A. 2011. Long-term outcomes of patients with aneurysmal subarachnoid haemorrhage. Lancet Neurol. 10 4: 349–356.
- Rinkel GJ, Djibuti M, Algra A, van Gijn J. 1998. Prevalence and risk of rupture of intracranial aneurysms: A systematic review. Stroke. 29 1: 251–256.
- Savaridas T, Andrews PJD, Harris B. 2004. Cortisol dynamics following acute severe brain injury. Intensive Care Med. 30 7: 1479–1483.
- Shin IY, Joo HM, Chung YG, Kim MS, Park JW, Ahn RS. 2011. Abnormal diurnal pattern of cortisol secretion in patients after aneurysmal subarachnoid hemorrhage. Stress. 14 2: 156–165.
- Spencer RL, Miller AH, Moday H, McEwen BS, Blanchard RJ, Blanchard DC, Sakai RR. 1996. Chronic social stress produces reductions in available splenic type II corticosteroid receptor binding and plasma corticosteroid binding globulin levels. Psychoneuroendocrinology. 21 1: 95–109.
- Vespa P. 2011. Endocrine function following acute SAH. Neurocrit Care. 15 2: 361–364.