Abstract
Restraint stress modulates pain and inflammation. The present study was designed to evaluate the effect of acute restraint stress on inflammatory pain induced by subcutaneous injection of bee venom (BV). First, we investigated the effect of 1 h restraint on the spontaneous paw-flinching reflex (SPFR), decrease in paw withdrawal mechanical threshold (PWMT) and increase in paw volume (PV) of the injected paw induced by BV. SPFR was measured immediately after BV injection, and PWMT and PV were measured 2 h before BV and 2–8 h after BV. The results showed that acute restraint inhibited significantly the SPFR but failed to affect mechanical hyperalgesia. In contrast, stress enhanced significantly inflammatory swelling of the injected paw. In a second series of experiments, the effects of pretreatment with capsaicin locally applied to the sciatic nerve, systemic 6-hydroxydopamine (6-OHDA), and systemic naloxone were examined on the antinociception and proinflammation produced by acute restraint stress. Local capsaicin pretreatment inhibited BV-induced nociception and inflammatory edema, and had additive effects with stress on nociception but reduced stress enhancement of edema. Systemic 6-OHDA treatment attenuated the proinflammatory effect of stress, but did not affect the antinociceptive effect. Systemic naloxone pretreatment eliminated the antinociceptive effect of stress, but did not affect proinflammation. Taken together, our data indicate that acute restraint stress contributes to antinociception via activating an endogenous opioid system, while sympathetic postganglionic fibers may contribute to enhanced inflammation in the BV pain model.
Introduction
The phenomenon of stress-induced analgesia (SIA) in humans and animals is well known (Amit & Galina, Citation1986; Butler & Finn, Citation2009). However, acute stress is also known to modulate the development of inflammation (Cikler et al., Citation2005; Peters et al., Citation2005; Theoharides & Cochrane, Citation2004). Several mechanisms are currently proposed to explain SIA, for example, opioids or non-opioids in the descending pain control system (Butler & Finn, Citation2009; Porro & Carli, Citation1988), activation of the neuroimmune or hypothalamic-pituitary-adrenal systems (Bomholt et al., Citation2004), and the release of corticotropin-releasing hormone or opioids in immune cells in the injured site (Busch-Dienstfertig & Stein, Citation2010; Lariviere & Melzack, Citation2000; Machelska, Citation2007). As to the mechanisms modulating the inflammation, these may include the activation of the sympathetic nervous system (Jänig et al., Citation1996; Lin et al., Citation2003) and degranulation of mast cells in the inflamed tissue (Cikler et al., Citation2005; Theoharides & Cochrane, Citation2004).
Acute restraint is an example of a source of stress that produces antinociception in selected animal models of pain, including the hot-plate (Amir & Amit, Citation1978; Houston et al., Citation1997) and formalin tests (Aloisi et al., Citation1998; Amit & Galina, Citation1986; Butler & Finn, Citation2009; Seo et al., Citation2006). However, other inflammatory (e.g. complete Freund’s adjuvant, and carrageenan) or neuropathic (spinal nerve ligation model) models are seldom used to study the mechanism underlying SIA and the modulatory effect of stress on inflammation, except for one study using the carrageenan model (Le Roy et al., 2011). Thus, the mechanisms underlying the effect of acute stress on nociception and inflammation in other pain models are unclear and need to be further investigated.
Subcutaneous injection of bee venom (BV) into the center of the plantar surface of a rat hind paw produces ipsilateral nociceptive responses, including persistent spontaneous nociception (PSN), prolonged heat and mechanical hyperalgesia, accompanied by obvious inflammatory responses including edema and redness of the injected paw (Chen & Chen, Citation2001; Chen & Lariviere, Citation2010). Thus, the BV model should be a suitable candidate to investigate the mechanisms of PSN, hyperalgesia and inflammation simultaneously. Our previous studies showed that capsaicin-sensitive primary afferents (CSPAs) and the sympathetic system play differential roles in BV-induced PSN, mechanical hyperalgesia, and inflammation: CSPA contribute to BV-induced PSN, mechanical hyperalgesia, and inflammation (Chen et al., Citation2006, Citation2007), while the sympathetic system contributes to BV-induced mechanical hyperalgesia and inflammation, but not PSN (Chen et al., Citation2010b). However, the effect of acute restraint stress in the BV inflammatory pain model is not clear.
Physical restraint is a model that can inflict potent psychological stress on experimental animals and thereby potentially induce physical, physiological, and psychological responses that affect noxious stimulation (Amit & Galina, Citation1986; Butler & Finn, Citation2009). Thus, the present study was designed to test the hypothesis that acute restraint stress modulates BV-induced PSN, mechanical hyperalgesia and inflammation. Furthermore, the roles of the CSPAs, endogenous opioids, and sympathetic efferent system in the BV-induced pain and inflammation responses were investigated.
Materials and methods
Animals
The experiments were performed on age-matched male Sprague–Dawley rats weighing 250–300 g. The rats were provided by the General Hospital of Shen-Yang Military Region, and use of the rats was reviewed and approved by the Animal Care and Use Committee of the Hospital. Guidelines of the International Association for the Study of Pain for research with animals were followed (Zimmermann, Citation1983). Rats were housed in plastic cages in a group of three in the colony rooms at ambient temperatures with food and water available ad libitum. A 12:12 h light dark cycle (lights on at 08:00 h) was maintained and testing was done between the hours of 09:00 h and 18:30 h. The rats were allowed to acclimate to the testing environment for at least 30 min each day for 5 d before testing. At the conclusion of each experiment, rats were euthanised by intraperitoneal (IP) injection of an over-dose of sodium phenobarbital (200 mg/kg).
Intraplantar administration of BV
The BV used in these experiments was lyophilized whole venom of Apis mellifera (Sigma, St. Louis, MO) dissolved in 0.9% sterile saline. A volume of 50 µL saline containing 0.2 mg BV was used for the whole study, because it has been shown that 0.2 mg in 50 µL was the optimal dose to produce a prolonged nociceptive behavioral response (Lariviere & Melzack, Citation1996).
Drugs and treatments
6-Hydroxydopamine (6-OHDA, in 0.9% sterile saline), capsaicin (in 10% ethanol, 10% Tween 80 and 80% saline) and naloxone (1 mg/ml in 0.9% sterile saline) were purchased from Sigma (St. Louis, MO). For chemical sympathectomy, rats were pre-treated with the neurotoxin 6-OHDA on a dosage schedule of 50 mg/kg IP each day for 2 d followed by 100 mg/kg IP each day for 3 d (Chen et al., Citation2010b; Wei et al., Citation2002).
To confirm the validity of 6-OHDA sympathectomy, noradrenaline levels in the plantar skin of the hind paws were measured, according to the methods described previously (Chen et al., Citation2010b).
For the destruction of CSPA fibers, rats in the capsaicin sub-group were anesthetized with sodium pentobarbital (50 mg/kg, IP) and the sciatic nerve was exposed in one of the hind limbs. A piece of cotton wool (0.5 cm × 1 cm) soaked with 0.15% capsaicin solution was gently wrapped around 1 cm of the target nerve for 30 min (Chen & Chen, Citation2001; Fitzgerald, Citation1983). As a control, a piece of cotton wool soaked with vehicle was gently wrapped around 1 cm of the target nerve for 30 min. The wound was washed with saline solution and sutured by layers. The whole operation was performed under strictly sterile conditions. Subsequently, a loss of sensitivity to a maximum 40 s heat stimulus (100 W, 10.5 V) applied to the hind paw 1 d after the capsaicin treatment was used to confirm the effect of capsaicin on the sciatic nerve. BV was injected into the hind paw ipsilateral to the capsaicin application.
Acute restraint stress
The rats subjected to acute restraint stress were confined in horizontal glass cylinders (5 cm diameter, 18 cm length, with ventilation holes), in which some degree of movement was allowed (such as head grooming with the forelimbs), but not with the hind limbs, and the rats were prevented from turning around or escaping. Acute restraint stress was performed after measuring baseline paw mechanical threshold and paw volume (PV).
Experimental design
The experimental protocol is illustrated in . Rats were randomly divided into five groups: BV, acute restraint stress, vehicle (Veh), drug (6-OHDA, capsaicin, or naloxone) treatment, and drug treatment + stress. Rats in the BV control group (n = 10) received a BV injection only, with no prior treatment. Those in the acute restraint stress group (stress + BV; n = 10) were restrained for 1 h prior to BV injection. The vehicle group (Veh + BV; n = 10) were administered vehicle before BV injection. Rats in the drug treatment groups (n = 8 per specific drug) were administered the respective drug prior to BV injection. The rats in the drug treatment + stress groups (n = 8–10 per specific drug) received the same drugs as the drug treatment groups, then acute restraint, followed by BV injection. To rule out the effect of restricting physical movement on the inflammatory response, an additional group of rats received only acute restraint stress (n = 8).
Figure 1. A diagram showing the experimental protocol. Chemical sympathectomy with 6-hydroxydopamine (6-OHDA) (A), local capsaicin (CAP) treatment on the sciatic nerve (B), or pretreatment with systemic naloxone (NAX) (C) was performed before restraint stress. The baseline of PWMT and PV of rats were measured (PWMT & PV, test 1) 2 h prior to BV. BV was injected subcutaneously and the effects of different treatments on BV-induced spontaneous foot lifting were assessed continuously for 1 h. The PWMT and PV were then measured 2, 4, 8 h after BV (PWMT & PV, test 2–4) to assess the effects on BV-induced mechanical hyperalgesia and edema. At 9 h after BV treatment, rats in panels A were euthanized and noradrenaline (NA) levels were measured.
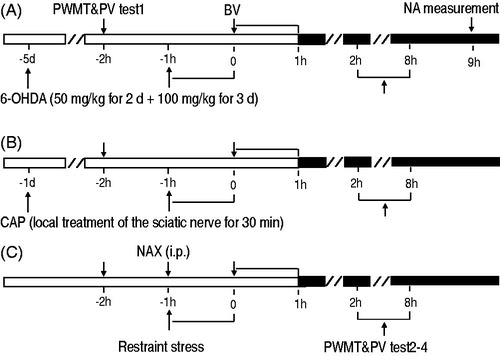
Measurement of PSN
A 30 × 30 × 30 cm3 transparent Plexiglass test box with a transparent glass floor was placed on a supporting frame 50 cm above the experimental table to allow the experimenters an unobstructed view of the paws of the rats. Each rat was placed in the test box for at least 30 min before administration of BV. The spontaneous nociceptive behavioral response was determined by the number of spontaneous paw flinching reflex (SPFR) movements during each 5 min interval of a 1 h time course following injection of BV (Chen et al., Citation2006, Citation2007).
Examination of mechanical hyperalgesia
The paw withdrawal mechanical threshold (PWMT) of both hind paws was examined 10 min before and 2, 4 and 8 h after BV injection, as described elsewhere (Chen et al., Citation2003, Citation2006). Rats were placed in individual Plexiglass chambers with mesh floors and covers (20 × 20 × 25 cm3). Von Frey filaments with bending forces of 2, 5, 7, 10, 12, 16, 20, 25, 30, 35 and 40 g were used to determine the PWMT. Each von Frey filament was applied for approximately 2–3 s to the plantar area of the tested hind paw in order to induce the end-point withdrawal reflex. A single von Frey filament was applied 10 times to each testing site (once every several seconds). The filament able to elicit a 50% withdrawal response was taken to be the PWMT (g). The change in PWMT was calculated as a percentage of the difference between the pre- and post-injection PWMTs: PWMTpre − PWMTpost/PWMTpre × 100.
Measurement of inflammation
Change in PV was used as a determinant of the BV-induced inflammatory response. PV of the injected paw was measured with the aid of a plethysmometer (ZH-YLS-7A, Zheng Hua Bioinstruments, Anhui, China) 2 h before and 2, 4 and 8 h after BV injection while the rat was immobilized (Chen et al., Citation2006, Citation2007).
Statistical analyses
All results are expressed as mean ± standard error of the mean (SEM). The data were analyzed using one-way ANOVA with Tukey’s post-hoc tests for data of normal distribution (SigmaStat™, Systat Software, San Jose, CA). Data that were not of normal distribution were analyzed by Kruskal–Wallis ANOVA by ranks. A probability value of p < 0.05 was considered statistically significant.
Results
The effect of acute restraint stress on BV-induced nociception and inflammation
In agreement with previous studies (Chen et al., Citation2006, Citation2007, Citation2008), subcutaneous injection of BV produced a persistent SPFR lasting for almost one hour (BV group in ) and a significant decrease in the PWMT of the injected side (BV group in ), indicative of mechanical hyperalgesia. In addition, there was a significant increase in the PV of the injected side throughout the 2–8 h observation period after the BV treatment (BV group in ).
Figure 2. Effect of acute stress on BV-induced nociception and inflammation. Panel A shows the effect on BV-induced persistent SPFR; Panel B shows the effect on BV-induced decrease in PWMT; Panel C shows the effect on BV-induced increase in PV. One way ANOVA analysis shows that acute restraint stress attenuated significantly BV-induced SPFR and enhanced significantly BV-induced increase in PV, but had no effect on BV-induced increase in PWMT. *p < 0.05, **p < 0.01, ***p < 0.001. Values are mean ± SEM. One-way ANOVA with Tukey’s post-hoc tests, n = 10 for BV group; n = 10 for BV + Stress group.
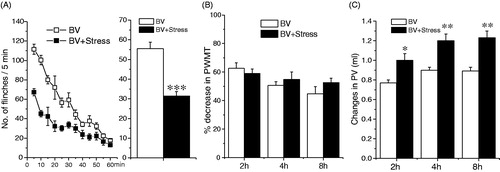
Compared with the BV group (BV + Vehicle), 1 h restraint stress treatment produced a significant inhibition of BV-induced SPFR (F (1, 19) = 62.218, p < 0.001, ), and a significant enhancement of increase in PV (2 h: F (1, 19) = 5.925, p < 0.05; 4 h: F (1, 19) = 7.547, p < 0.01; 8 h : F (1, 19) = 8.589, p < 0.01, ), but had no effect on decrease in PWMT during the 2–8 h observation period (p > 0.05, ). In rats only receiving acute restraint, PV prior to and 2–8 h after acute restraint did not differ significantly (data not shown), ruling out the effect of restricting physical movement on the inflammatory response induced by BV.
The effect of capsaicin treatment on the inhibition of BV-induced SPFR and on enhancement of BV-induced inflammation produced by acute restraint stress
In agreement with previous studies (Chen et al., Citation2007), pre-treatment with capsaicin produced a significant inhibition of BV-induced SPFR (F (1, 17) = 8.436, p < 0.05, ) and of increased PV of the injected side (2 h: F (1, 17) = 8.295, p < 0.05; 4 h: F (1, 17) = 7.985, p < 0.05; 8 h: F (1, 17) = 7.994, p < 0.05, ), compared with the BV + Vehicle (Control) group. These anti-nociceptive and anti-inflammatory effects of capsaicin were still obvious after acute restraint stress, compared to the group that received the drug without the restraint stress (BV + CAP); antinociception: (BV + CAP versus BV + CAP + Stress: (F (1, 15) = 40.327, p < 0.001, ); anti-inflammation: (2 h: F (1, 15) = 7.747, p < 0.05; 4 h: F (1, 15) = 6.442, p < 0.05; 8 h: F (1, 15) = 6.741, p < 0.05, ).
The effect of 6-OHDA treatment on the inhibition of BV-induced PFR and on enhancement of inflammation under produced by acute restraint stress
Figure 3. Effect of local treatment of the sciatic nerve with capsaicin (CAP) on BV-induced PSN and inflammation under acute restraint stress. Panel A shows the effect on the inhibition of BV-induced SPFR produced by acute stress; Panel B shows the effect on the enhancement of BV-induced increase in PV produced by acute stress. Rats in Control groups were those treated with BV + Vehicle. One way ANOVA analysis shows that local CAP had no effect on acute stress-induced anti-nociceptive and pro-inflammatory effects. *p < 0.05, **p < 0.01, ***p < 0.001, compared with Control group; &p < 0.05, &&&p < 0.001, compared with BV + CAP group. Values are mean ± SEM. One-way ANOVA with Tukey’s post-hoc tests, n = 10 for Control group; n = 8 for BV + CAP group; n = 10 for BV + Stress group; n = 8 for BV + Stress + CAP group.
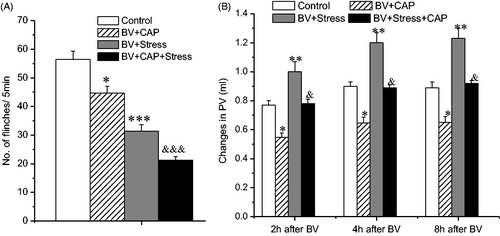
In agreement with a previous study (Chen et al., Citation2010b), chemical (6-OHDA) sympathectomy had no effect on BV-induced SPFR (F (1, 17) = 1.038, p > 0.05; ), but produced a significant inhibition of increase in PV throughout the observation period (2 h: F (1, 17) = 10.247, p < 0.01; 4 h: F (1, 17) = 11.789, p < 0.01; 8 h: F (1, 17) = 11.561, p < 0.01, ). Chemical sympathectomy had no effect on the inhibition of BV-induced SPFR induced by acute restraint stress (BV + 6-OHDA versus BV + 6-OHDA + Stress: F (1, 17) = 36.451, p < 0.001; ), but significantly blocked the enhancement of BV-induced paw swelling incited by acute restraint stress during the observation period (BV + 6OHDA versus BV + 6OHDA + Stress: 2 h: F (1, 17) = 4.031, p > 0.05; 4 h: F (1, 17) = 3.585, p > 0.05; 8 h: F (1, 17) = 2.354, p > 0.05, ). To confirm the validity of 6-OHDA sympathectomy, the noradrenaline level of hindpaw skin in 3–4 rats was measured, and the results showed that the treatment produced a significant reduction of noradrenaline content, compared with the control group (control versus 6-OHDA: 68.2 ± 5.89 versus 8.9 ± 0.71 ng/g wet tissue weight, p < 0.001), in agreement with our previous reports (Chen et al., Citation2010b).
The effect of naloxone treatment on the inhibition of BV-induced SPFR and on enhancement of inflammation produced by acute restraint stress
Figure 4. Effect of systemic treatment of 6-OHDA on BV-induced PSN and inflammation under acute restraint stress. Panel A shows the effect on the inhibition of BV-induced SPFR produced by acute stress; Panel B shows the effect on the enhancement of BV-induced increase in PV produced by acute stress. Rats in Control groups were those treated with BV + Vehicle. One way ANOVA analysis shows that systemic 6-OHDA had no effect on acute stress-induced anti-nociceptive effect, but reversed partially pro-inflammatory effects. *p < 0.05, **p < 0.01, ***p < 0.001, compared with Control group. Values are mean ± SEM. One-way ANOVA with Tukey’s post-hoc tests, n = 10 for Control group; n = 8 for BV + 6-OHDA group; n = 10 for BV + Stress group; n = 9 for BV + Stress + 6-OHDA group.
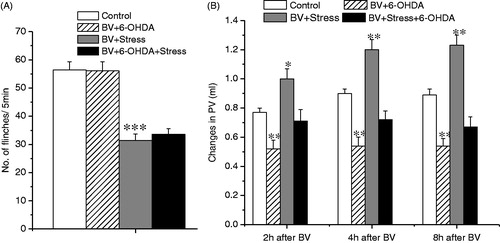
Compared to the BV + Vehicle (Control) group, pretreatment with naloxone (5 mg/kg, IP) had no effect on BV-induced SPFR (F (1, 17) = 2.038, p > 0.05; ), or on BV-induced enhancement of PV throughout the 2–8 h of observation ((2 h: F (1, 17) = 2.234, p > 0.05; 4 h: F (1, 17) = 1.639, p > 0.05; 8 h:F (1, 17) = 1.764, p > 0.05; ). However, when the administration of naloxone was followed by acute restraint stress, there was a complete blockage of the inhibition of BV-induced SPFR produced by stress (F(1, 19) = 3.125, p > 0.05, ), but naloxone had no effect on the enhancement of BV-induced increase in PV incited by acute restraint stress (2 h: F (1, 19) = 5.839, p < 0.05; 4 h: F (1, 19) = 5.025, p < 0.05; 8 h: F (1, 19) = 5.795, p < 0.05, ).
Figure 5. Effect of systemic naloxone (NAX) on BV-induced PSN and inflammation under acute restraint stress. Panel A shows the effect on the inhibition of BV-induced SPFR produced by acute stress; Panel B shows the effect on the enhancement of BV-induced decrease in PV produced by acute stress. Rats in Control groups were those treated with BV + Vehicle. One way ANOVA analysis shows that systemic NAX completely reversed the acute stress-induced anti-nociceptive effect, but had no effect on pro-inflammatory effects. *p < 0.05, **p < 0.01, ***p < 0.001 compared with Control group; ###p < 0.001 compared with BV + Stress group; &p < 0.05 compared with BV + NAX group. Values are mean ± SEM. One-way ANOVA with Tukey’s post-hoc tests, n = 10 for Control group; n = 8 for BV + NAX group; n = 10 for BV + Stress group; n = 10 for BV + Stress + NAX group.
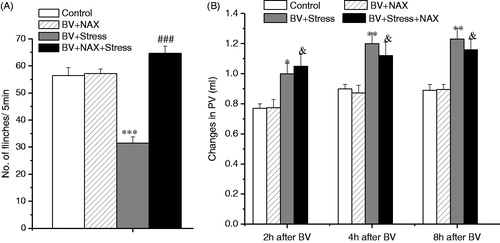
Discussion
The results of the present study clearly showed that acute restraint stress produced a significant inhibition of BV-induced PSN, and a significant enhancement of BV-induced inflammatory edema, but had no effect on mechanical hyperalgesia. Furthermore, the endogenous opioid and sympathetic nervous systems evidently contributed to the antinociceptive and proinflammatory effects, respectively. Capsaicin pretreatment had an additive antinociceptive effect with stress and alone reduced inflammation, and it reduced the stress-mediated pro-inflammatory response.
Previous studies primarily used the hot plate test to investigate the mechanisms of SIA (Amit & Galina, Citation1986; Butler & Finn, Citation2009), although a hot plate is not a pathological condition. The formalin test is a pathological model and is also often used in SIA studies (Amit & Galina, Citation1986; Butler & Finn, Citation2009). Our study provides further behavioral evidence for SIA utilizing BV in a pathological experimental pain and inflammation model.
In the present study, acute restraint stress produced a significant inhibition of BV-induced PSN, but had no effect on BV-induced mechanical hyperalgesia. The results are in agreement with previous research demonstrating the significant inhibition of formalin-induced PSN by acute restraint stress (Aloisi et al., Citation1998; Lafrance et al., Citation2010; Seo et al., Citation2006), and thus provide further evidence of SIA. It is of interest that acute restraint stress had no effect on BV-induced mechanical hyperalgesia in the present study. To the best of our knowledge, this appears to be the first study to investigate and report behavioral evidence for the effect of acute stress on mechanical hyperalgesia in a pathological condition. These interesting results beg the question as to whether there is no effect of stress on hyperalgesia in other animal pain models, and this needs to be investigated in future experiments.
The current study also showed that acute restraint stress aggravated BV-induced inflammatory swelling. Many studies have argued for the contribution of stress to the increased inflammatory response (Cikler et al., Citation2005; Peters et al., Citation2005; Theoharides & Cochrane, Citation2004). However, the aggravated inflammatory response seems contradictory to the antinociceptive effect, because it seems plausible that BV-induced inflammation may exacerbate the pain, rather than inhibit PSN. The proposal also gets support from a previous study showing the BV-induced PSN is highly related to inflammation (Lariviere et al., Citation2005). However, our recent studies do not favor the proposal. For example, sympathectomy significantly attenuated BV-induced inflammatory edema, but failed to affect PSN (Chen et al., Citation2010b), and pharmacological interventions produced differential effects on BV-induced PSN and inflammation (Chen et al., Citation2008, Citation2010a). Taken together, the inflammatory swelling and PSN may share differential mechanisms in the BV model. Another possible explanation is that perhaps acute stress has two effects: one is a worsening of peripheral inflammation hence more pain; another is a central analgesic influence. In this case, the two components are acting in opposition to each other to achieve a complex variable outcome.
Compelling evidence shows that CSPAs are essentially involved in development of pain in different models (Christoph et al., Citation2006; Simone et al., Citation1998). In our previous studies, we demonstrated that CSPA fibers contributed to BV-induced PSN, mechanical hyperalgesia and inflammatory swelling (Chen & Chen, Citation2001; Chen et al., Citation2006, Citation2007). However, to date, it is not clear whether CSPAs play a role in the effects of stress on pain and inflammation. The present results obtained in the BV model show that the inhibition of BV-induced spontaneous flinching and inflammatory edema following destruction of CSPA fibers by CAP pretreatment remained evident under the conditions of acute restraint stress. Namely, the destruction of CSPA fibers had no effects on the antinociception and proinflammation produced by acute restraint stress. To the best of our knowledge, this is the first behavioral evidence that CSPAs have no effects on stress-induced antinociception and proinflammation. Of course, the negative results may be due to the attenuated effect of capsaicin on PSN and inflammation. Further experiments need to be done in future to investigate the issue by pretreatment with a vanilloid receptor (VR1) antagonist.
In our previous studies, we demonstrated that sympathetic postganglionic neurons contributed to BV-induced PSN and mechanical hyperalgesia (Chen et al., Citation2010b). However, in the present study, we found that chemical sympathectomy had no effect on the antinociception induced by acute restraint stress, but partially blocked the proinflammatory effect, indicating the possible contribution of sympathetic postganglionic neurons to the proinflammatory effect, but not the antinociceptive effect induced by acute stress in the BV model. It is well known that the sympathetic nervous system can modulate the processing and transmission of nociceptive signals to the spinal cord, thereby modulating the development of inflammation through neurogenic mechanisms such as the axon and dorsal root reflexes (Jänig et al., Citation1996; Lin et al., Citation2003). Furthermore, we argue that degranulation of mast cells in inflamed tissue may contribute to the proinflammatory effect (Cikler et al., Citation2005; Theoharides & Cochrane, Citation2004).
In the present study, we found that the endogenous opioid system contributes to SIA, but not to the stress-induced proinflammation in the BV pain model. This was shown by the finding that pretreatment with naloxone completely blocked the antinociceptive effect induced by acute restraint stress, but had no effect on the proinflammatory effect. The results are consistent with previous studies demonstrating the role of opioid mechanisms from the descending pain control system (Butler & Finn, Citation2009; Porro & Carli, Citation1988). Given that, the drug has a short half-life, the lack of the effect of naloxone on inflammation may be due to the early administration time (prior to stress). When inflammation was measured, there may have been no or less effect of naloxone remaining. Thus, additional experiments need to be done in future to investigate the issue. It is acknowledged that peripheral opioids also play a role in SIA, especially in inflammatory conditions (Busch-Dienstfertig & Stein, Citation2010; Machelska, Citation2007). Thus, the role of peripheral opioids in SIA in the BV model warrants further investigation.
It is interesting to note that acute restraint stress produced a differential effect on BV-induced PSN and mechanical hyperalgesia, which is indicative of different underlying mechanisms, and these results agree with those of previous studies. For instance, pharmacological studies have found that different receptors or intracellular signal pathways at the peripheral and spinal levels contribute to BV-induced PSN and mechanical hyperalgesia (Chen et al., Citation2010a, Citationb; Li & Chen Citation2003; Li et al., Citation2000). In addition, the pain facilitating system from the rostral medial medulla oblongata is known to contribute to BV-induced PSN, but not mechanical hyperalgesia (Chen et al., Citation2003). A previous study showed that BV-induced PSN correlated well with increasing paw edema whereas mechanical hyperalgesia was already maximal at modest increases in PV (Lariviere et al., Citation2005). Lower doses of indomethacin reversed the BV-induced flinching reflex than mechanical hyperalgesia (Lariviere et al., Citation2005). This finding could mean that the two measures are not equally easy to reverse. It is unclear whether the same conditions apply to SIA in the BV model, and deserve to be investigated.
In conclusion, on one hand, the present results further confirm our previous finding that CSPA fibers contribute to BV-induced PSN, mechanical hyperalgesia, and the inflammatory response, while sympathetic postganglionic neurons contribute to BV-induced PSN and mechanical hyperalgesia. On the other hand, the present results clearly show that, in the rat BV pain model, acute restraint stress produces an antinociceptive effect on the persistent spontaneous flinching reflex and facilitates inflammatory swelling, but has no effect on mechanical hyperalgesia. Furthermore, the endogenous opioid system may contribute to the antinociceptive effect, while sympathetic postganglionic neurons contribute to a proinflammatory effect. CSPA fibers do not appear to play any significant role in either an antinociceptive or proinflammatory effect.
Declaration of interest
There are no conflicts of interest.
The work was supported by grants from the National Natural Science Foundation of the P.R. of China (30772089, 81271240) to Dr Hui-Sheng Chen and grant from Science and Technology Project Plan of Liao Ning Province (2012225010) to Prof. Bao-Jun Liu.
References
- Aloisi AM, Ceccarelli I, Lupo C. Behavioural and hormonal effects of restraint stress and formalin test in male and female rats. Brain Res Bull 1998;1:57–62
- Amir S, Amit Z. Endogenous opioid ligands may mediate stress-induced changes in the affective properties of pain related behavior in rats. Life Sci 1978;23:1143–52
- Amit Z, Galina ZH. Stress-induced analgesia: adaptive pain suppression. Physiol Rev 1986;66:1091–120
- Bomholt SF, Harbuz MS, Blackburn-Munro G, Blackburn-Munro RE. Involvement and role of the hypothalamo-pituitary-adrenal (HPA) stress axis in animal models of chronic pain and inflammation. Stress 2004;7:1–14
- Busch-Dienstfertig M, Stein C. Opioid receptors and opioid peptide-producing leukocytes in inflammatory pain – basic and therapeutic aspects. Brain Behav Immun 2010;24:683–94
- Butler RK, Finn DP. Stress-induced analgesia. Prog Neurobiol 2009;88:184–202
- Chen J, Chen HS. Pivotal role of capsaicin-sensitive primary afferents in development of both heat and mechanical hyperalgesia induced by intraplantar bee venom injection. Pain 2001;91:367–76
- Chen HS, He X, Wang Y, Wen WW, You HJ, Arendt-Nielsen L. Roles of capsaicin-sensitive primary afferents in differential rat models of inflammatory pain: a systematic comparative study in conscious rats. Experimental Neurology 2007;204:244–51
- Chen J, Lariviere WR. The nociceptive and anti-nociceptive effects of bee venom injection and therapy: a double-edged sword. Prog Neurobiol 2010;92:151–83
- Chen HS, Lei J, He X, Qu F, Wang Y, Wen WW, You HJ, Arendt-Nielsen L. Peripheral involvement of PKA and PKC in subcutaneous bee venom-induced persistent nociception, mechanical hyperalgesia, and inflammation in rats. Pain 2008;135:31–6
- Chen HS, Lei J, He X, Wang Y, Wen WW, Wei XZ, Graven-Nielsen T, et al. Pivotal involvement of neurogenic mechanism in subcutaneous bee venom induced inflammation and allodynia in unanesthetized conscious rats. Experimental Neurology 2006;200:386–91
- Chen HS, Li MM, Shi J, Chen J. Supraspinal contribution to development of both tonic nociception and referred mirror hyperalgesia: a comparative study between formalin test and bee venom test in the rat. Anesthesiology 2003;98:1231–6
- Chen HS, Qu F, He X, Kang SM, Liao D, Lu SJ. Differential roles of peripheral metabotropic glutamate receptors in bee venom-induced nociception and inflammation in conscious rats. J Pain 2010a;11:321–9
- Chen HS, Qu F, He X, Wang Y, Wen WW. Chemical or surgical sympathectomy prevents mechanical hyperalgesia induced by intraplantar injection of bee venom in rats. Brain Research 2010b;1353:86–93
- Christoph T, Grünweller A, Mika J, Schäfer MK, Wade EJ, Weihe E, Erdmann VA, et al. Silencing of vanilloid receptor TRPV1 by RNAi reduces neuropathic and visceral pain in vivo. Biochem Biophys Res Commun 2006;350:238–43
- Cikler E, Ercan F, Cetinel S, Contuk G, Sener, G. The protective effects of melatonin against water avoidance stress-induced mast cell degranulation in dermis. Acta Histochem 2005;106:467–75
- Fitzgerald M. Capsaicin and sensory neurones: a review. Pain 1983;15:109–30
- Houston AJ, Wong JC, Ebenezer IS. A study on the involvement of GABAB receptor ligands in stress-induced antinociception in male mice. Meth Find Exp Clin Pharmacol 1997;19:167–71
- Jänig W, Levine JD, Michaelis M. Interactions of sympathetic and primary afferent neurons following nerve injury and tissue trauma. Prog Brain Res 1996;113:161–84
- Lafrance M, Roussy G, Belleville K, Maeno H, Beaudet N, Wada K, Sarret P. Involvement of NTS2 receptors in stress-induced analgesia. Neuroscience 2010;166:639–52
- Lariviere WR, Chesler EJ, Li Z, Shang GW, Chen YN, Yu YQ, Lu ZM, et al. Correlations between edema and the immediate and prolonged painful consequences of inflammation: therapeutic implications? Sheng Li Xue Bao 2005;57:278–88
- Lariviere WR, Melzack R. The bee venom test: a new tonic-pain test. Pain 1996;66:271–7
- Lariviere WR, Melzack R. The bee venom test: comparisons with the formalin test with injection of different venoms. Pain 2000;84:111–12
- Le Roy C, Laboureyras E, Gavello-Baudy S, Chateauraynaud J, Laulin JP, Simonnet G. Endogenous opioids released during non-nociceptive environmental stress induce latent pain sensitization via a NMDA-dependent process. J Pain 2011;12:1069–79
- Li KC, Chen J. Differential roles of spinal protein kinases C and A in development of primary heat and mechanical hypersensitivity induced by subcutaneous bee venom chemical injury in the rat. Neurosignals 2003;12:292–301
- Li KC, Zheng JH, Chen J. Involvement of spinal protein kinase C in induction and maintenance of both persistent spontaneous flinching reflex and contralateral heat hyperalgesia induced by subcutaneous bee venom in the conscious rat. Neurosci Lett 2000;285:103–6
- Lin Q, Zou X, Fang L, Willis WD. Sympathetic modulation of acute cutaneous flare induced by intradermal injection of capsaicin in anesthetized rats. J Neurophysiol 2003;89:853–61
- Machelska H. Targeting of opioid-producing leukocytes for pain control. Neuropeptides 2007;41:355–63
- Peters EM, Kuhlmei A, Tobin DJ, Müller-Röver S, Klapp BF, Arck PC. Stress exposure modulates peptidergic innervation and degranulates mast cells in murine skin. Brain Behav Immun 2005;19:252–62
- Porro CA, Carli G. Immobilization and restraint effects on pain reactions in animals. Pain 1988;32:289–307
- Seo YJ, Kwon M-S, Shim E-J, Park S-H, Choi O-S, Suh H-W. Changes in pain behavior induced by formalin, substance P, glutamate and proinflammatory cytokines in immobilization-induced stress mouse model. Brain Res Bull 2006;71:279–86
- Simone DA, Nolano M, Johnson T, Wendelschafer-Crabb G, Kennedy WR. Intradermal injection of capsaicin in humans produces degeneration and subsequent reinnervation of epidermal nerve fibers: correlation with sensory function. J Neurosci 1998;18:8947–59
- Theoharides TC, Cochraned DE. Critical role of mast cells in inflammatory diseases and the effect of acute stress. J Neuroimmunol 2004;146:1–12
- Wei H, Jyväsjärvi E, Niissalo S, Hukkanen M, Waris E, Konttinen YT, Pertovaara A. The influence of chemical sympathectomy on pain responsivity and alpha 2-adrenergic antinociception in neuropathic animals. Neuroscience 2002;114:655–68
- Zimmermann M. Ethical guidelines for investigations of experimental pain in conscious animals. Pain 1983;16:109–10