Abstract
Stressful life events elicit hypothalamic–pituitary–adrenal (HPA) axis activation, which may alter psychological states or behavioral routines. Therefore, the current study focused on the HPA axis response to better understand such manifestations in female prairie voles (Microtus ochrogaster). In Experiment 1, females were stressed for 1 h via one of the four stressors: exposure to a novel environment, immobilization (“plastic mesh”), brief social defeat, or prolonged social defeat. Following a 30-min recovery, the females received a 5-min elevated plus maze (EPM) test and, subsequently, blood was collected to measure plasma corticosterone concentrations. Only immobilization stress induced an anxiety-like behavioral response in the EPM test and elevated plasma corticosterone levels compared to the control groups. Corticosterone concentrations were also significantly elevated following exposure to prolonged social defeat compared to the control conditions, but not after novel environment stress or short social defeat. In Experiment 2, females were exposed to immobilization stress over 1, 3, or 7 days in a daily (predictable; pIMO) or irregular (unpredictable; uIMO) schedule. The biobehavioral stress response in females exposed to pIMO for 3 or 7 days did not differ significantly from controls, suggesting these females habituated. By comparison, females exposed to uIMO over 3 or 7 days did not habituate behaviorally or physiologically, even producing augmented corticosterone levels. In both experiments, positive correlations were found between corticosterone levels and anxiety-like behaviors in the EPM test. Together, our data suggest that the stress response by female prairie voles is dependent on stress intensity, source, previous experience, and predictability. Furthermore, the HPA axis response, as evident by corticosterone levels, is associated with the impact that these factors have on behavioral routine.
Introduction
Stressful events are common aspects of life and can originate from a number of environmental sources, including psychological, social, and physical. Stress-induced activation of the hypothalamic–pituitary–adrenal (HPA) axis involves a cascade of physiological changes that have been associated with the stress-induced effects on emotional processing (Erickson et al., Citation2003), normal behavioral routines (Blanchard et al., Citation2001; DeVries, Citation2002), and mental health (Smith & Wang, Citation2012; Young, Citation2004). The risk for such disruptions to normal homeostatic function may be associated with the context or nature of the stressor as well as how such experiences affect the HPA response to subsequent stressors (Armario, Citation2006).
Hennessy et al. (Citation1979) noted that plasma corticosterone levels rise in response to exposure to a novel environment in rats, and this corticosterone response depended on the duration of exposure as well as the degree of environmental unfamiliarity. In succeeding studies, the stress intensity and previous stress experience have also been implicated to modulate the HPA axis stress response in humans and rats (Armario et al., Citation1996; García et al., Citation2000). Thus, characteristics associated with a stressor can dictate the responsiveness of the HPA axis, particularly the rise in plasma glucocorticoids. In addition, the stress-induced glucocorticoid response can lead to increased psychological distress, influencing the psychological state in humans and behavioral routines in animals. For example, the stress-induced cortisol response in humans is associated with an increased perception of anxiety in individuals exposed to psychosocial stress in the laboratory (e.g. Trier Social Stress Test) and in life (e.g. temporary separation from a marital partner; Diamond et al., Citation2008; Ditzen et al., Citation2007; Robles, Citation2007). Calvo & Volosin (Citation2001) noted that administration of a glucocorticoid synthesis inhibitor (e.g. metyrapone) or adrenalectomy can eliminate the anxiogenic effects of restraint stress on anxiety-like behavior in rats. In the same study, an injection of corticosterone, a mineralocorticoid receptor agonist (e.g. deoxycorticosterone), or a glucocorticoid agonist (e.g. dexamethasone) restored the anxiogenic effects of stress. Thus, the action of glucocorticoids and their receptors are sufficient and necessary to cause some the behavioral and physiological effects that are associated with acute stress. However, such physiological activation seems dependent on the context of the stressful event. These data highlight the importance of understanding stress-related factors that modulate the behavioral and physiological response toward a primary stressor as well as the adaptability of the stress system toward subsequent stressors.
Prairie voles (Microtus ochrogaster) live in a fundamentally social environment, including male-female pair-bonds, biparental caregiving, and extended families, but are also highly territorial (Carter et al., Citation1995; Getz et al., Citation1981; Keverne & Curley, Citation2004). Vole behavior and physiology is acutely attuned to alterations to the social environment or other environmental cues. In fact, a number of recent studies have denoted that the absence of social contact in prairie voles can promote a disruption to normal HPA axis activity and behavioral routines that mimic symptomatology of depression and anxiety disorders in humans (Grippo et al., Citation2007a,Citationb,Citationc, Citation2008, Citation2009; Lieberwirth et al., Citation2012; Pournajafi-Nazarloo et al., Citation2009; Stowe et al., Citation2005). In addition, prairie vole pair bonding facilitates a significant reduction in basal HPA axis activity (Carter et al., Citation1997; DeVries et al., Citation1995, Citation1997b), while separation from a bonded partner can increase plasma corticosterone concentrations (Bosch et al., Citation2009). Furthermore, despite being glucocorticoid resistant (Hastings et al., Citation1999; Taymans et al., Citation1997), the prairie vole HPA axis is still responsive to various stressors (e.g. Bosch et al., Citation2009; DeVries et al., Citation1996; Grippo et al., Citation2008; Liu et al., Citation2001; Taymans et al., Citation1997), social cues (reviewed in Smith & Wang Citation2012), and circadian cues (Taymans et al., Citation1997). However, little research has been done to determine whether the behavioral and physiological response in prairie voles varies as a function of the nature of the stressor. This is important as determining the responsivity of prairie voles to various stressors will lead to better models of stress in this socially and physiologically unique species.
Thus, the current study examined the characteristics of stress, including source and intensity as well as context of previous stress experience to a homotypic stressor, on the response of the HPA axis and subsequent behavioral manifestations during a secondary stressor (the elevated plus maze, EPM, test). Socially housed female prairie voles (M. ochrogaster) were exposed to various acute psychological and social stressors (Experiment 1) or were exposed to a homotypic stressor (immobilization) for 1, 3, or 7 days in a predictable daily schedule or an unpredictable varying schedule (Experiment 2). In Experiment 1, we utilized two psychological stressors that have been demonstrated to vary by intensity while still provoking a biobehavioral response in rodents (i.e. mild stress: environmental novelty; severe stress: immobilization stress). In addition, we exposed female prairie voles to a resident-intruder paradigm in which they entered the home cage of an aggressive same-sex conspecific to induce social defeat. We utilized pair-bonded female prairie voles as the aggressive conspecifics as cohabitation with a male will facilitate territorial behavior in female prairie voles (Getz et al., Citation1981), and they will display aggressive behavior toward an intruder in a resident-intruder confrontation. We utilized two separate social defeat paradigms that varied in the length of physical confrontation with the aggressive conspecific and ability of the defeated intruder to withdrawal from continued confrontation. We predicted that the behavioral and physiological response to each of these acute stressors will depend on the source and intensity of the stressor. In Experiment 2, we focused on whether the stress response in female prairie voles was affected by the predictability of a repeated homotypic stressor. While repeated exposures to immobilization stress can lead to habituation to this stressor when it is predictable (Gagliano et al., Citation2008; Girotti et al., Citation2006; Martí & Armario, Citation1997; Rabasa et al., Citation2011), it has been demonstrated that an irregular or unpredictable schedule of immobilization stress does not desensitize, and can even augment, the stress response in male rats (Quirce et al., Citation1981). Therefore, we predicted that female prairie voles would adapt to repeated exposures of immobilization stress as a function of predictability, with predictable immobilization stress producing a habituating effect and unpredictable immobilization stress augmenting the biobehavioral stress response.
Materials and method
Subjects
Subjects were captive-bred female prairie voles (M. ochrogaster) descended from populations in southern Illinois. Subjects were weaned at 21 days of age and then housed in same-sex age-matched pairs in plexiglass cages (29 L × 18 W × 13 H cm) containing cedar chip bedding. Food and water were provided ad libitum. Colony rooms were maintained at 21 ± 1 °C with a 14L:10D photoperiod (lights on at 0700 h). Before the start of this study, all female subjects were sexually naïve and of adult age (between 90 and 120 days of age). Two weeks prior to the first stress exposure, female subjects were housed with an unfamiliar, unrelated, vasectomized adult male. Experiments were conducted in accordance with the guidelines of the Institutional Animal Care and Use Committee at Florida State University.
Stress paradigms
Numerous environmental stimuli can be defined as stressors. The current study utilized four stress paradigms that vary in the nature of the source, predominantly psychological or social stress, and the intensity or severity, low or high. The stressors employed in this study included environmental novelty (low intensity, psychological stress), immobilization (high intensity, psychological stress), and social defeat with a brief (15 min; low intensity, social stress) or prolonged (30 min; high intensity, social stress) physical confrontation. In the novel environment paradigm, subjects were placed into an open field arena (56 L × 56 W × 20 H cm) for 60 min. In the immobilization (plastic mesh) paradigm, subjects were exposed to 60 min of immobilization in restraint tubes constructed from PVC pipes (10.5 L × 1.75 radius cm) with air vent holes in front for animal respiration and an opening in the back for animal placement – a design similar to other restraint tubes for prairie voles (DeVries et al., Citation1997a). Prior to placement into these restraint tubes, immobilized females were bound (excluding the head) in plastic mesh, leading to complete physical immobilization.
Subjects exposed to social defeat experienced one of two separate paradigms to vary the intensity of the defeat. In the brief social defeat paradigm, subjects were placed into the resident cage (45 L × 22 W × 20 H cm) of an unfamiliar, unrelated, pair-bonded female prairie vole after its male partner was removed. As pair-bonded female prairie voles are territorial (Getz et al., Citation1981), they displayed aggressive behavior toward the intruding female subject. Subjects were exposed to physical interaction with the aggressive resident for 15 min (behavioral video confirmed this interaction). Thereafter, a plexiglass divider with air holes was placed in the center of the cage to prevent physical aggression, though nonphysical aggression was still possible, and allow the subject to create spatial distance from the aggressor, up to 22.5 cm. The social defeat exposure continued for an additional 45 min with the divider in place. In the prolonged social defeat paradigm, the physical contact period lasted for 30 min, then the subject was placed in a small wire-mesh container (5 cm3) in the center of the aggressor’s cage for an additional 30 min. The small wire-mesh container was used to (1) prevent physical contact, (2) allow the resident to continue to display aggressive posturing and other forms of nonphysical aggression, and (3) prevent the subject from creating spatial separation between the resident and itself. Following each of the stress treatments, subjects were put into a clean, empty cage and remained alone for 30 min before they were tested on an EPM test.
Finally, a handled-control group (HAN) was created. HAN subjects were picked up and briefly handled at the same time as the four stress groups but then returned to their home cage with their partner. However, as the HAN females did not experience 30 min social isolation as the females experienced following their stress exposure, a second control group (social control, SC) was created for Experiment 1. SC females were briefly handled, stayed with their partner for 60 min, and then were transferred to a clean, empty cage for 30 min before the EPM test.
EPM test
The EPM test was conducted for 5 min using an established method (Pan et al., Citation2009; Stowe et al., Citation2005). Briefly, the EPM (Columbus Instruments, Columbus, OH) is comprised of two open arms (35 L x 6.5 W cm) and two closed arms (35 L × 5 W × 15 H cm) that cross in the middle, and is elevated 45 cm off the ground. Subjects were placed in the center facing an open arm and recorded with a video/computer system. Several behaviors were quantified by a trained observer blind to the treatment using J-Watcher V1.0 (Macquarie University and UCLA; http://www.jwatcher.ucla.edu/) for anxiety-like responses (latency to enter the open arm, percentage of time spent on the open arms versus total arm time, and percentage of open arm entries versus total arm entries) and locomotor activity (total arm entries).
Blood collection and preparation
Immediately after the EPM test, trunk blood (∼400 µl) was collected following rapid decapitation into microcentrifuge vials containing 20 µl EDTA. The vials were inverted and immediately placed in ice. The entire blood collection procedure until chilling did not exceed 2 min. Blood was centrifuged at 6000 rpm for 15 min at 4 °C, then plasma was aspirated and centrifuged at 6000 rpm for 10 min at 4 °C. Plasma was aliquoted into microcentrifuge vials and stored at −80 °C until processed via a corticosterone radioimmunoassay.
Corticosterone radioimmunoassay
Plasma corticosterone (1:1000) was measured (in duplicates) in 10 μl plasma samples using commercially available kits (Diagnostic Products Corp., Los Angeles, CA) that have been used and validated in previous studies in prairie voles (Bosch et al., Citation2009; Grippo et al., Citation2007a; Stowe et al., Citation2005; Taymans et al., Citation1997). Other than the dilution factor, which was optimized for vole physiology, the assays were conducted according to the manufacturer’s directions. The detecting limit of the radioimmunoassay kit was 7.7 ng/ml, and the intra-assay and inter-assay coefficient of variation (CV) was 2.85% and 2.11%, respectively.
Experimental design
Experiment 1 was designed to test the influence of the source of a stressful event on the post-stress recovery of the HPA axis and anxiety-like behavior (). Subjects were pair-housed with an unrelated, vasectomized male for 2 weeks, a period that reliably leads to vole pair bonding (Aragona & Wang, Citation2004). Thereafter, subjects were removed from their home cage between 1100 and 1200 h and randomly assigned into one of the four stress paradigms, environmental novelty (n = 6), immobilization (n = 6), brief social defeat (n = 8), or prolonged social defeat (n = 7), or the control groups (HAN: n = 6; SC: n = 6). Following the 60 min of stress, subjects were placed in an empty, clean cage with food and water ad libitum for a 30-min recovery period. Thereafter, subjects were tested for their anxiety-like behaviors in the EPM test. Immediately after the EPM test, subjects were sacrificed via rapid decapitation, and trunk blood was taken and stored at −80 °C until processed via a corticosterone radioimmunoassay.
Experiment 2 was designed to evaluate the effect of previous experience with a homotypic stressor in a daily predictable (fixed) or unpredictable (irregular) schedule on the post-stress HPA axis and behavioral recovery (). As the immobilization stress was effective in inducing both hormonal and behavioral stress responses in Experiment 1, we focused on the immobilization paradigm. Subjects were pair-housed with an unrelated, vasectomized male for 2 weeks. In the predictable immobilization paradigm (pIMO), females were exposed to 60 min of immobilization (between 1100 and 1200 h) every day for 3 (n = 8) or 7 (n = 6) consecutive days (). In the unpredictable IMO paradigm (uIMO), the immobilization schedule was established to minimize predictability of the presentation of the stress. Several studies have utilized immobilization stress with an unpredictable schedule varying stress-rest days, immobilization duration, and time of day (Bryant et al., Citation1988; Martí & Armario, Citation1997; Quirce et al., Citation1981; Rockman et al., Citation1987). In the 3-day uIMO schedule (n = 7), the schedule included immobilization on days 1 and 3 and no immobilization stress on day 2 (). In the 7-day uIMO schedule (n = 6), subjects were exposed to immobilization stress on days 1, 3, 4, and 7 and rested on days 2, 5, and 6 (). Thirty minutes after the last immobilization, subjects were tested for their anxiety-like behaviors in the EPM test, and then sacrificed via rapid decapitation. Trunk blood was collected, stored at −80 °C, and processed via a corticosterone radioimmunoassay. In addition, a HAN control group (n = 11) and an acute immobilized female group (n = 6) were established as in Experiment 1 to compare how pIMO and uIMO affect the biobehavioral stress response.
Data analysis
Data were analyzed using IBM SPSS Statistics 19 (SPSS, Inc., an IBM Company) and were expressed as mean ± SEM. EPM behavior and plasma corticosterone concentrations were analyzed with a one-way ANOVA with stress condition as the single factor. Significant group differences (p < 0.05) were further assessed with a Gabriel's post-hoc test, as this test explicitly allows for unequal sample sizes. In addition, corticosterone levels were correlated to anxiety-like behavior in the EPM test for Experiments 1 and 2 by Pearson’s correlations. All alpha levels were set at p < 0.05.
Results
Various psychological and social stressors on behavioral and physiological stress response
In Experiment 1, anxiety-like behaviors in the EPM test and corticosterone levels were significantly different among groups, an effect that seemed to depend on the source and intensity of the stressor as indicated by post-hoc analysis. Particularly, there were group differences in the latency to enter the open arm, F(5,31) = 3.08, p < 0.005 and the percentage of time spent in the open arm, F(5,33) = 3.23, p < 0.05 in the EPM test. However, post-hoc analyses indicated that only immobilized females delayed entrance into the open arm and decreased time spent in the open arm compared to the HAN females (). No group differences were observed in the percentage of entries into the open arm, F(5,33) = 2.13, p = 0.09; , or in the number of total arm entries, F(5,33) = 1.77, p = 0.15; , indicating that differences between immobilized and HAN females in their anxiety-like behaviors were not due to altered locomotor activity. In addition, corticosterone levels were significantly elevated following the EPM in females exposed to immobilization and prolonged social defeat stress compared to HAN females, but not females exposed to environmental novelty or brief social defeat stress (F(5,33) = 4.27, p < 0.005; . It is worth noting that the effects of stress on the behavioral and physiological responses seem to be a function of the stressor rather than the brief separation from the partner during the 30 min recovery period as HAN and SC females did not differ in any of the measurements. In addition, there was a negative correlation between female corticosterone concentrations and percentage of time spent in the open arm in the EPM test [r = −0.42, p = 0.01], such that females with high corticosterone concentrations spent significantly less time in the open arm than females with low corticosterone concentrations (). There was no correlation between the corticosterone concentrations and latency to enter the open arm [r = 0.08, p = 0.64] or percentage of entries into the open arm [r = −0.13, p = 0.44; data not shown].
Figure 3. The source and intensity of a stressor affected the biobehavioral stress response. (a–b), Only immobilization (IMO) stress led to a (a) delay in the latency of females to enter the open arm and (b) decreased percentage of time that females spent in the open arms during the EPM test. (c) None of the stressors influenced locomotor behavior (i.e. total arm entries) during the EPM test. (d) Corticosterone remained elevated 30 min post-stress in response to IMO and prolonged social defeat (SD), but not environmental novelty (Novel) or brief SD, in comparison to handled controls (HAN). (a–d) No differences were observed between HAN controls or social controls (SC). (e) Female plasma corticosterone concentrations were negatively associated with the percentage of time females spent in the open arm in the EPM test. Bars labeled with different letters differ significantly by Gabriel’s post hoc test in which a significant main effect was detected in the ANOVA (p < 0.05). (a–d) Data are expressed as mean ± SEM.
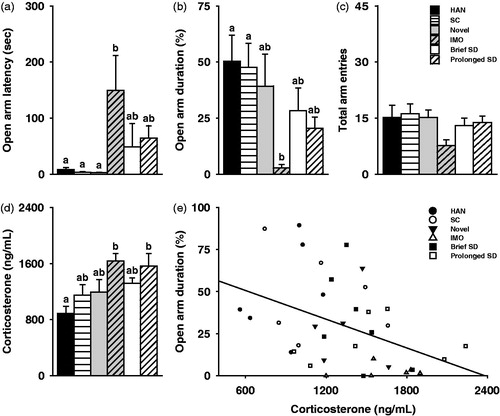
Table 1. Effect of stressors on the frequency of arm entries in the elevated plus maze.
Predictable versus unpredictable immobilization on behavioral and physiological stress response
The behavioral and physiological response seemed to vary as a function of the predictability and number of exposures to repeated immobilization in female prairie voles. There were group differences in the anxiety-like behaviors in the EPM test, including latency to enter the open arm, F(5,37) = 5.14, p < 0.001, percentage of entries into the open arm, F(5,38) = 3.23, p < 0.05, and percentage of time spent in the open arm, F(5,38) = 5.78, p < 0.001. When post-hoc analyses were conducted no group differences were observed in the percentage of entries into the open arm () and only females exposed once to immobilization significantly delayed entry into the open arm compared to HAN controls (). However, females exposed to uIMO for 3 or 7 days, like females exposed to a single immobilization, significantly decreased the percentage of time spent in the open arm compared to HAN controls, while females exposed to pIMO for 3 or 7 days were similar to HAN controls (). These effects on anxiety-like behavior were not the result of altered locomotor activity as there were no group differences in total arm entries (F(5,38) = 1.19, p = 0.33; .
Figure 4. The predictability of immobilization (IMO) stress influenced the biobehavioral stress response. (a) Females exposed to 1 day IMO delayed entry into the open arm in the EPM test compared to handled control females (HAN) and females exposed to 3 or 7 day predictable IMO (pIMO). (b) The percentage of time that females spent in the open arms during the EPM test was lower after exposure to 1 day IMO, 3-day uIMO, and 7 day unpredictable IMO (uIMO) compared to HAN controls. No differences were observed between HAN control females or females exposed to 3- or 7-day pIMO. (c) None of the stressors influenced locomotor behavior (i.e. total arm entries) during the EPM test. (d) Corticosterone remained elevated 30 min post-stress in response to exposure to 1 day IMO, 3-day pIMO, 3-day uIMO, and 7-day uIMO compared to HAN controls. However, no differences were observed between HAN controls and 7-day pIMO. Females exposed to 7-day uIMO had significantly higher corticosterone levels than 1 day IMO and 7-day pIMO. (e) Female plasma corticosterone concentrations were negatively associated with the percentage of time females spent in the open arm in the EPM test. Bars labeled with different letters differ significantly by Gabriel’s post-hoc test in which a significant main effect was detected in the ANOVA (p < 0.05). (a–d) Data are expressed as mean ± SEM.
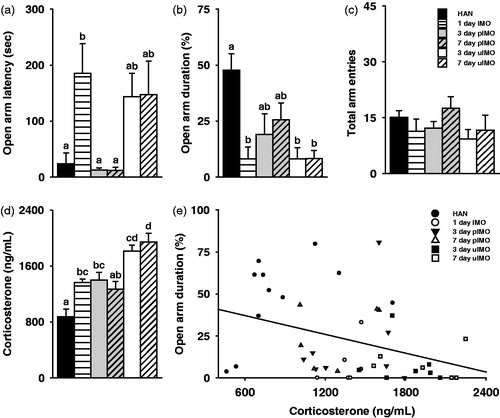
In addition, there was a significant group difference in the plasma corticosterone concentrations (F(5,38) = 14.69, p < 0.001; . Females exposed to pIMO for 3 consecutive days had corticosterone levels significantly higher than HAN controls. However, females exposed to pIMO for 7 consecutive days had corticosterone levels similar to HAN controls, indicating 7 days, rather than 3 days, of pIMO is sufficient to induce habituation. In addition, females exposed to uIMO for 3 or 7 days had corticosterone levels significantly elevated to HAN controls, like females exposed to a single day of immobilization. Furthermore, while females exposed to uIMO for 3 days had corticosterone levels similar to females exposed to immobilization for 1 day, females exposed to uIMO for 7 days had significantly higher corticosterone levels compared to females exposed to immobilization for 1 day, indicating a time course for augmentation. Females exposed to uIMO for 7 days also had significantly higher corticosterone levels than females exposed to pIMO for 7 days, indicating the predictability of immobilization affected corticosterone levels. Finally, corticosterone levels were associated with the latency to enter the open arm [r = 0.37, p < 0.05; data not shown] and percentage of time spent in the open arm [r = −0.36, p < 0.05; ], but not the percentage of entries into the open arm [r = −0.21, p = 0.17; data not shown]. Females with high corticosterone levels delayed entry into the open arm more and spent less percentage of time in the open arm compared to females with low corticosterone levels.
Discussion
Stressful life events are common and can be rather disruptive to normal physiological function and behavioral routines, depending on the nature of the stressor. In the current study, the stress response by female prairie voles was dependent on stress intensity, source, and predictability as well as previous experience with the stressor. Female prairie voles exposed to immobilization (“plastic mesh”) or prolonged social defeat displayed increased corticosterone levels after an EPM test, but only immobilized females displayed behavioral disruption (i.e. increased anxiety-like behaviors in the EPM test). This suggests that the disturbance to normal physiological function, particularly HPA axis function, induced by immobilization is persistent and may lead to aberrant behavioral manifestations in subsequent stressful conditions. Thus, we further evaluated the effects of repeated immobilization, modulating predictability of the exposure. Females exposed to pIMO displayed physiological (7 days repeated) and behavioral (3 and 7 days repeated) habituation, while females exposed to uIMO did not display habituation. In fact, females exposed to uIMO for 7 days had augmented corticosterone levels. It seems that the behavioral and physiological response to the EPM test following repeated immobilization depended on the predictability of the stress as well as the number of days of repeated immobilization. Furthermore, the HPA axis response, as evident by corticosterone levels, was associated with the impact that these factors had on behavioral routines.
The stress response can be non-specific, as described by Hans Selye (Citation1936), but there are components of the stress response that are adaptive to specific environmental cues, creating a stressor-specific response (reviewed in Armario, Citation2006). Regarding the HPA axis, circulating corticosterone concentrations during stress are related to the intensity of stress in response to low to intermediate intensity stressors, but not high intensity stressors (Armario et al., Citation1986a,Citationb; Hennessy et al., Citation1979; Hennessy & Levine Citation1978; Natelson et al., Citation1981). This is partially due to the fact that maximal adrenal steroidogenesis can be produced from an intermediate ACTH release, disassociating ACTH and corticosterone release during high intensity stressors (Keller-Wood et al., Citation1981). However, when post-stress corticosterone levels are considered, high intensity stressors provide distinctive recovery rates (García et al., Citation2000; Marquez et al., Citation2002). In the current study, corticosterone levels were significantly elevated following an EPM test in female prairie voles recovering from immobilization but not environmental novelty. Furthermore, corticosterone levels were elevated following the EPM test in females exposed to prolonged social defeat paradigm, which included longer physical confrontation and lack of control/no escape during non-physical confrontation, but not in females exposed to the brief social defeat paradigm. Therefore, the intensity of social defeat may vary as a function of duration of the physical confrontation or control during the confrontation – control referring to the capability of the vole to avoid the aggressive conspecific by making an appropriate motor response (i.e. moving to the maximal distance away from the aggressive conspecific), a definition adapted from Levine (Citation1985). Together, these data suggest that environmental novelty induces mild psychological stress while immobilization and social defeat reflects more severe stress in prairie voles, similar to other rodent species (Armario, Citation2006; Korte & De Boer, Citation2003). In addition, our data demonstrate that prairie voles may be utilized as an ethologically-relevant rodent model of female social defeat. This is valuable as animal models of female social defeat are lacking. For example, female-female aggression is not seen in most rodent species, with the exception of maternal aggression (Björkqvist, Citation2001; Bosch et al., Citation2004; Huhman, Citation2006).
Furthermore, we evaluated the behavioral response of stressed females to a secondary stressor, the EPM test. While immobilization and prolonged social defeat led to a rise in plasma corticosterone levels following an EPM test, only immobilization induced anxiety-like behavior on the EPM test. A simple interpretation would be that immobilization is a more intense stressor than social defeat and therefore led to the behavioral manifestations. However, it would be worth noting that the EPM test evaluates a non-social anxiety-like response, and therefore, social stress, like social defeat, may not induce anxiety-like behavior in this context. For example, Barsy et al. (Citation2010) noted that restraint stress evokes a generalized anxiety response in rats. Specifically, restrained rats displayed social and non-social anxiety-like behaviors as inferred from social avoidance in a social interaction test (SIT) and avoidance of the open arms in an EPM test, respectively. By comparison, social defeat induced social avoidance in the SIT in rats but had no effect on EPM behavior. Thus, social stress may selectively induce social anxiety, or anxiety-like behavior within a social context, while psychological stress induces a general anxiety-like state. In fact, several studies have observed that different types of stress can lead to different behavioral consequences (e.g. psychological versus social stress: Doremus-Fitzwater et al., Citation2009; van Erp et al., Citation1994; psychological versus physical stress: Daviu et al., Citation2012; physical versus social stress: Gasparotto et al., Citation2005; McBlane & Handley, Citation1994; environmental versus psychological stress: Muñoz-Abellán et al., Citation2008, Citation2011).
For several decades, it has been known that the magnitude of the HPA axis response to a stressor, even the more intense stressors like immobilization, declines with repeated exposures. For example, repeated restraint or immobilization stress can facilitate a reduction in the secretion of peripheral HPA axis hormones (i.e. ACTH and corticosterone) and depress the stress-induced neuronal activation (e.g. c-fos and CRH gene expression) in the PVN and other brain regions that regulate the stress-induced CRH action in the PVN (e.g. the hippocampus and amygdala) (Armario et al., Citation2004; García et al., Citation2000; Melia et al., Citation1994; Pinnock & Herbert, Citation2001). This depressed response toward a homotypic stressor has been referred to as habituation (Thompson & Spencer, Citation1966). As predicted, female prairie voles exposed to repeated pIMO (three sessions: 3-day pIMO; seven sessions: 7-day pIMO) displayed a habituated behavioral and physiological response. However, voles exposed to uIMO (two sessions: 3-day uIMO; four sessions: 7-day uIMO) displayed an augmented corticosterone response and lack of behavioral habituation. Under the pIMO and uIMO paradigms, female prairie voles were exposed to the same intense immobilization stress for the same duration (1 h). Thus, the difference in the adaptation to pIMO compared to uIMO seems to be independent of these characteristics. While increasing the number of exposures may have influenced the habituation of females exposed to pIMO, it did not facilitate habituation in females exposed to uIMO. Moreover, females exposed to three immobilization sessions during the 3-day pIMO schedule displayed a reduced anxiety-like behavioral response, while the females exposed to four immobilization sessions during the 7-day uIMO schedule did not habituate behaviorally, and even displayed an augmented corticosterone response. It seems likely that the longer and more irregular intervals between immobilization exposures observed in uIMO compared to the shorter and consistent intervals in the pIMO schedule facilitate the differences in adaptations. In order to further understand the influence that stress predictability has on the underlying neuroendocrine mechanism that governs stress habituation or augmentation in voles, it will be worth evaluating the influence of repeated pIMO and uIMO on various markers of stress-induced neuronal activation, including c-fos and CRH gene expression in the PVN and other brain regions that regulate the stress-induced CRH action in the PVN. As well-documented in other rodent species (Armario et al., Citation1988; Grissom & Bhatnagar, Citation2009; Martí & Armario, Citation1998), repeated stress may have influenced adrenal sensitivity in female prairie voles; thus, additional research needs to be conducted to determine the influence of pIMO and uIMO on the ACTH response and changes to adrenal weight.
Prairie voles are not commonly used in stress research. Nonetheless, features of vole physiology and their social system make them a promising rodent model of stress. The fact that we measured plasma corticosterone concentrations 30 min after a primary stressor and immediately following a secondary stressor (EPM test) makes interpretation of the dynamics of the corticosterone response more challenging. Nonetheless, our current results suggest that vole physiology is responsive to the stress intensity, source, and predictability as well as previous experience with a stressor. Prairie voles exhibit high basal plasma corticosterone levels, 5 to 10 times higher than rats and mice (Taymans et al., Citation1997). However, voles do not display common consequences associated with chronic hypercortisolism, potentially due to a suppressed corticosterone signal (Hastings et al., Citation1999; Taymans et al., Citation1997). Therefore, prairie voles could provide a valuable rodent model of glucocorticoid resistance, and understanding which characteristics of a stressor modulate this response is necessary for such research. Furthermore, the prairie vole social system has many similarities to human society that are not reflected in the social systems of more traditional laboratory rodents, including male–female pair-bonds, male and female territoriality, biparental care, and extended families (Carter et al., Citation1995; Getz et al., Citation1981; Keverne & Curley, Citation2004). Moreover, the social environment has a major influence on the stress system. Therefore, as more emphasis is given to understand how different facets of the vole social system influences the stress response, knowledge about how these same factors regulate human stress may be gleaned.
Declaration of interest
This work was supported by the National Science Foundation Graduate Research Fellowship and National Institutes of Health grant NIMHF31-095464 to A. S. and the NIH grant NIMHR01-058616 to Z. W.
Acknowledgements
Special thanks to L. Linton and L. Rouser for assistance in data collection and behavioral analyses. We also thank Dr. Y. Liu and K. Lei for their critical reading of an early version of this article.
References
- Aragona BJ, Wang Z. (2004). The prairie vole (Microtus ochrogaster): an animal model for behavioral neuroendocrine research on pair bonding. ILAR J 45:35–45
- Armario A. (2006). The hypothalamic-pituitary-adrenal axis: what can it tell us about stressors? CNS Neurol Disord Drug Targets 5:485–501
- Armario A, Hidalgo J, Giralt M. (1988). Evidence that the pituitary-adrenal axis does not cross-adapt to stressors: comparison to other physiological variables. Neuroendocrinology 47:263–7
- Armario A, Lopez-Calderon A, Jolin T, Castellanos JM. (1986a). Sensitivity of anterior pituitary hormones to graded levels of psychological stress. Life Sci 39:471–5
- Armario A, Martí O, Molina T, de Pablo J, Valdes M. (1996). Acute stress markers in humans: response of plasma glucose, cortisol and prolactin to two examinations differing in the anxiety they provoke. Psychoneuroendocrinology 21:17–24
- Armario A, Montero JL, Balasch J. (1986b). Sensitivity of corticosterone and some metabolic variables to graded levels of low intensity stresses in adult male rats. Physiol Behav 37:559–61
- Armario A, Valles A, Dal-Zotto S, Marquez C, Belda X. (2004). A single exposure to severe stressors causes long-term desensitisation of the physiological response to the homotypic stressor. Stress 7:157–72
- Barsy B, Leveleki C, Zelena D, Haller J. (2010). The context specificity of anxiety responses induced by chronic psychosocial stress in rats: a shift from anxiety to social phobia? Stress 13:230–7
- Björkqvist K. (2001). Social defeat as a stressor in humans. Physiol Behav 73:435–42
- Blanchard RJ, McKittrick CR, Blanchard DC. (2001). Animal models of social stress: effects on behavior and brain neurochemical systems. Physiol Behav 73:261–71
- Bosch OJ, Krömer SA, Brunton PJ, Neumann ID. (2004). Release of oxytocin in the hypothalamic paraventricular nucleus, but not central amygdala or lateral septum in lactating residents and virgin intruders during maternal defence. Neuroscience 124:439–48
- Bosch OJ, Nair HP, Ahern TH, Neumann ID, Young LJ. (2009). The CRF system mediates increased passive stress-coping behavior following the loss of a bonded partner in a monogamous rodent. Neuroendocrinology 34:1406–15
- Bryant HU, Story JA, Yim GK. (1988). Assessment of endogenous opioid mediation in stress-induced hypercholesterolemia in the rat. Psychosom Med 50:576–85
- Calvo N, Volosin M. (2001). Glucocorticoid and mineralocorticoid receptors are involved in the facilitation of anxiety-like response induced by restraint. Neuroendocrinology 73:261–71
- Carter CS, DeVries AC, Getz LL. (1995). Physiological substrates of monogamy: the prairie vole model. Neurosci Biobehav R 19:303–14
- Carter CS, DeVries AC, Taymans SE, Roberts RL, Williams JR, Getz LL. (1997). Peptides, steroids, and pair bonding. Ann N Y Acad Sci 807:260–72
- Daviu N, Delgado-Morales R, Nadal R, Armario A. (2012). Not all stressors are equal: behavioral and endocrine evidence for development of contextual fear conditioning after a single session of footshocks but not of immobilization. Front Behav Neurosci 6:69
- DeVries AC. (2002). Interaction among social environment, the hypothalamic-pituitary-adrenal axis, and behavior. Horm Behav 41:405–13
- DeVries AC, DeVries MB, Taymans SE, Carter CS. (1995). Modulation of pair bonding in female prairie voles (Microtus ochrogaster) by corticosterone. Proc Natl Acad Sci USA 92:7744–48
- DeVries AC, DeVries MB, Taymans SE, Carter CS. (1996). The effects of stress on social preferences are sexually dimorphic in prairie voles. Proc Natl Acad Sci USA 93:11980–4
- DeVries AC, Gerber JM, Richardson HN, Moffatt CA, Demas GE, Taymans SE, Nelson RJ. (1997a). Stress affects corticosteroid and immunoglobulin concentrations in male house mice (Mus musculus) and prairie voles (Microtus ochrogaster). Comp Biochem Phys A 118:655–63
- DeVries AC, Taymans SE, Carter CS. (1997b). Social modulation of corticosteroid responses in male prairie voles. Ann N Y Acad Sci 807:494–7
- Diamond LM, Hicks AM, Otter-Henderson KD. (2008). Every time you go away: changes in affect, behavior, and physiology associated with travel-related separations from romantic partners. J Pers Soc Psychol 95:385–403
- Ditzen B, Neumann ID, Bodenmann G, von Dawans B, Turner RA, Ehlert U, Heinrichs M. (2007). Effects of different kinds of couple interaction on cortisol and heart rate responses to stress in women. Psychoneuroendocrinology 32:565–74
- Doremus-Fitzwater TL, Varlinskaya EI, Spear LP. (2009). Social and non-social anxiety in adolescent and adult rats after repeated restraint. Physiol Behav 97:484–94
- Erickson K, Drevets W, Schulkin J. (2003). Glucocorticoid regulation of diverse cognitive functions in normal and pathological emotional states. Neurosci Biobehav 27:233–46
- Gagliano H, Fuentes S, Nadal R, Armario A. (2008). Previous exposure to immobilisation and repeated exposure to a novel environment demonstrate a marked dissociation between behavioral and pituitary-adrenal responses. Behav Brain Res 187:239–45
- García A, Martí O, Vallès A, Dal-Zotto S, Armario A. (2000). Recovery of the hypothalamic-pituitary-adrenal response to stress: effect of stress intensity, stress duration and previous stress exposure. Neuroendocrinology 72:114–25
- Gasparotto OC, Lopes DM, Carobrez SG. (2005). Pair housing affects anxiety-like behaviors induced by a social but not by a physiological stressor in male Swiss mice. Physiol Behav 85:603–12
- Getz LL, Carter CS, Gavish L. (1981). The mating system of prairie voles, Microtus ochrogaster: field and laboratory evidence for pair-bonding. Beh Ecol Sociobiol 8:189–94
- Girotti M, Pace TWW, Gaylord RI, Rubin BA, Herman JP, Spencer RL. (2006). Habituation to repeated restraint stress is associated with lack of stress-induced c-fos expression in primary sensory processing areas of the rat brain. Neuroscience 138:1067–81
- Grippo AJ, Cushing BS, Carter CS. (2007a). Depression-like behavior and stressor-induced neuroendocrine activation in female prairie voles exposed to chronic social isolation. Psychosom Med 69:149–57
- Grippo AJ, Gerena D, Huang J, Kumar N, Shah M, Ughreja R, Sue Carter C. (2007b). Social isolation induces behavioral and neuroendocrine disturbances relevant to depression in female and male prairie voles. Psychoneuroendocrinology 32:966–80
- Grippo AJ, Lamb DG, Carter CS, Porges SW. (2007c). Social isolation disrupts autonomic regulation of the heart and influences negative affective behaviors. Biol Psychiatr 62:1162–70
- Grippo AJ, Trahanas DM, Zimmerman Ii RR, Porges SW, Carter CS. (2009). Oxytocin protects against negative behavioral and autonomic consequences of long-term social isolation. Psychoneuroendocrinology 34:1542–53
- Grippo AJ, Wu KD, Hassan I, Carter CS. (2008). Social isolation in prairie voles induces behaviors relevant to negative affect: toward the development of a rodent model focused on co-occurring depression and anxiety. Depress Anxiety 25:E17–26
- Grissom N, Bhatnagar S. (2009). Habituation to repeated stress: get used to it. Neurobiol Learn Mem 92:215–24
- Hastings NB, Orchinik M, Aubourg MV, McEwen BS. (1999). Pharmacological characterization of central and peripheral type I and type II adrenal steroid receptors in the prairie vole, a glucocorticoid-resistant rodent. Endocrinology 140:4459–69
- Hennessy MB, Heybach JP, Vernikos J, Levine S. (1979). Plasma corticosterone concentrations sensitively reflect levels of stimulus intensity in the rat. Physiol Behav 22:821–5
- Hennessy MB, Levine S. (1978). Sensitive pituitary-adrenal responsiveness to varying intensities of psychological stimulation. Physiol Behav 21:295–7
- Huhman KL. (2006). Social conflict models: can they inform us about human psychopathology? Horm Behav 50:640–6
- Keller-Wood ME, Shinsako J, Keil LC, Dallman MF. (1981). Insulin-induced hypoglycemia in conscious dogs. I. Dose-related pituitary and adrenal responses. Endocrinology 109:818–24
- Keverne EB, Curley JP. (2004). Vasopressin, oxytocin and social behaviour. Curr Opin Neurobiol 14:777–83
- Korte SM, De Boer SF. (2003). A robust animal model of state anxiety: fear-potentiated behaviour in the elevated plus-maze. Eur J Pharmacol 463:163–75
- Levine S. (1985). A definition of stress? In: Moberg GP, editor. Animal stress. Bethesda, MD: American Physiological Society. p 51–70
- Lieberwirth C, Liu Y, Jia X, Wang Z. (2012). Social isolation impairs adult neurogenesis in the limbic system and alters behaviors in female prairie voles. Horm Behav 62:357–66
- Liu Y, Curtis JT, Fowler CD, Spencer C, Houpt T, Wang Z. (2001). Differential expression of vasopressin, oxytocin and corticotrophin-releasing hormone messenger RNA in the paraventricular nucleus of the prairie vole brain following stress. J Neuroendocrinol 13:1059–65
- Marquez C, Belda X, Armario A. (2002). Post-stress recovery of pituitary-adrenal hormones and glucose, but not the response during exposure to the stressor, is a marker of stress intensity in highly stressful situations. Brain Res 926:181–5
- Martí O, Armario A. (1997). Influence of regularity of exposure to chronic stress on the pattern of habituation of pituitary-adrenal hormones, prolactin and clucose. Stress (3):179–89
- Martí O, Armario A. (1998). Anterior pituitary response to stress: time-related changes and adaptation. Int J Develop Neurosci 16:241–60
- McBlane JW, Handley SL. (1994). Effects of two stressors on behaviour in the elevated X-maze: preliminary investigation of their interaction with 8-OH-DPAT. Psychopharmacology 116:173–82
- Melia KR, Ryabinin AE, Schroeder R, Bloom FE, Wilson MC. (1994). Induction and habituation of immediate early gene expression in rat brain by acute and repeated restraint stress. J Neurosci 14:5929–38
- Muñoz-Abellán C, Andero R, Nadal R, Armario A. (2008). Marked dissociation between hypothalamic-pituitary-adrenal activation and long-term behavioral effects in rats exposed to immobilization or cat odor. Psychoneuroendocrinology 33:1139–50
- Muñoz-Abellán C, Rabasa C, Daviu N, Nadal R, Armario A. (2011). Behavioral and endocrine consequences of simultaneous exposure to two different stressors in rats: interaction or independence? PLoS ONE 6:e21426
- Natelson BH, Tapp WN, Adamus JE, Mittler JC, Levin BE. (1981). Humoral indices of stress in rats. Physiol Behav 26:1049–54
- Pan Y, Liu Y, Young KA, Zhang Z, Wang Z. (2009). Post-weaning social isolation alters anxiety-related behavior and neurochemical gene expression in the brain of male prairie voles. Neurosci Lett 454:67–71
- Pinnock SB, Herbert J. (2001). Corticosterone differentially modulates expression of corticotropin releasing factor and arginine vasopressin mRNA in the hypothalamic paraventricular nucleus following either acute or repeated restraint stress. Eur J Neurosci 13:576–84
- Pournajafi-Nazarloo H, Partoo L, Sanzenbacher L, Paredes J, Hashimoto K, Azizi F, Sue Carter C. (2009). Stress differentially modulates mRNA expression for corticotrophin-releasing hormone receptors in hypothalamus, hippocampus and pituitary of prairie voles. Neuropeptides 43:113–23
- Quirce CM, Odio M, Solano JM. (1981). The effects of predictable and unpredictable schedules of physical restraint upon rats. Life Sci 28:1897–902
- Rabasa C, Muñoz-Abellán C, Daviu N, Nadal R, Armario A. (2011). Repeated exposure to immobilization or two different footshock intensities reveals differential adaptation of the hypothalamic-pituitary-adrenal axis. Physiol Behav 103:125–33
- Robles TF. (2007). Stress, social support, and delayed skin barrier recovery. Psychosom Med 69:807–15
- Rockman GE, Hall A, Hong J, Glavin GB. (1987). Unpredictable cold-immobilization stress effects on voluntary ethanol consumption in rats. Life Sci 40:1245–51
- Selye H. (1936). A syndrome produced by diverse nocuous agents. Nature 138:32
- Smith AS, Wang Z. (2012). Salubrious effects of oxytocin on social stress-induced deficits. Horm Behav 61:320–30
- Stowe JR, Liu Y, Curtis JT, Freeman ME, Wang Z. (2005). Species differences in anxiety-related responses in male prairie and meadow voles: the effects of social isolation. Physiol Behav 86:369–78
- Taymans SE, DeVries AC, DeVries MB, Nelson RJ, Friedman TC, Castro M, Detera-Wadleigh S, et al. (1997). The hypothalamic-pituitary-adrenal axis of prairie voles (Microtus ochrogaster): evidence for target tissue glucocorticoid resistance. Gen Comp Endocr 106:48–61
- Thompson RF, Spencer WA. (1966). Habituation: a model phenomenon for the study of neuronal substrates of behavior. Psychol Rev 73:16–43
- van Erp AM, Kruk MR, Meelis W, Willekens-Bramer DC. (1994). Effect of environmental stressors on time course, variability and form of self-grooming in the rat: handling, social contact, defeat, novelty, restraint and fur moistening. Behav Brain Res 65:47–55
- Young AH. (2004). Cortisol in mood disorders. Stress 7:205–8