Abstract
Even though there are indications that stress influences body temperature in humans, no study has systematically investigated the effects of stress on core and peripheral body temperature. The present study therefore aimed to investigate the effects of acute psychosocial stress on body temperature using different readout measurements. In two independent studies, male and female participants were exposed to a standardized laboratory stress task (the Trier Social Stress Test, TSST) or a non-stressful control task. Core temperature (intestinal and temporal artery) and peripheral temperature (facial and body skin temperature) were measured. Compared to the control condition, stress exposure decreased intestinal temperature but did not affect temporal artery temperature. Stress exposure resulted in changes in skin temperature that followed a gradient-like pattern, with decreases at distal skin locations such as the fingertip and finger base and unchanged skin temperature at proximal regions such as the infra-clavicular area. Stress-induced effects on facial temperature displayed a sex-specific pattern, with decreased nasal skin temperature in females and increased cheek temperature in males. In conclusion, the amplitude and direction of stress-induced temperature changes depend on the site of temperature measurement in humans. This precludes a direct translation of the preclinical stress-induced hyperthermia paradigm, in which core temperature uniformly rises in response to stress to the human situation. Nevertheless, the effects of stress result in consistent temperature changes. Therefore, the present study supports the inclusion of body temperature as a physiological readout parameter of stress in future studies.
Introduction
Exposure to stress results in an endocrine, autonomic and behavioral response that enable an organism to adapt to a changing environment (Korte et al., Citation2005). Of these parameters, there is evidence that human body temperature changes in response to stress (Briese, Citation1995; Kleitman & Jackson, Citation1950; Marazziti et al., Citation1992; Oka & Oka, Citation2007; Vinkers et al., Citation2010). Already in 1930, a case report described the presence of psychogenic fever (Falcon-Lesses & Proger, Citation1930). Later studies have focused on axillary and oral temperature changes in students or residents following exam-related stress (Briese, Citation1995; Gotsev & Ivanov, Citation1950, Citation1954; Marazziti et al., Citation1992). Also, male participants watching or participating in a boxing contest were found to display increased oral temperatures compared to a non-stressful situation (Renbourn, Citation1960). In support of stress-induced changes in temperature, decreased finger temperature is used as a marker of stress in autonomic biofeedback techniques (Ahmed et al., Citation2010) or in relaxation approaches associated with an increase in finger skin temperature (Yang et al., Citation2011). In animals, body temperature responses to stress are present in a variety of mammalian species including rodents, pigs, squirrels, baboons and chimpanzees (Bouwknecht et al., Citation2007; Nakayama et al., Citation2005; Parr & Hopkins, Citation2000b; Vinkers et al., Citation2008). Stress may also acutely or chronically affect body temperature in psychiatric disorders, including schizophrenia (Shiloh et al., Citation2005, Citation2009), depressive disorders (Daimon et al., Citation1992), and insomnia (Lack et al., Citation2008). Nevertheless, human thermoregulation is a complex process under the control of the central nervous system, and core and peripheral temperature in humans may respond differently to stress exposure. To our knowledge, no study has systematically investigated the effects of acute stress on core and peripheral body temperature in humans. Therefore, the present study aimed to investigate the effects of acute psychosocial stress on human body temperature using different core and peripheral readout parameters.
Methods
General
To gather preliminary data on stress-induced changes in body temperature, we exposed healthy male and female individuals to the Trier Social Stress Test (study 1, n = 24). Because no control condition was included in study 1, we added a control condition in a follow up study using a within-subject design and expanded the number of core and peripheral body temperature parameters (study 2, n = 22).
Participants
Healthy individuals (age 18–26 years) were recruited in both studies. In study 1, 12 male and 12 female individuals were included, whereas in study 2, 10 male and 12 female individuals were recruited. All female participants used oral contraceptives. All studies were approved by the Utrecht University Hospital ethics review board and performed according to the International Conference of Harmonisation guidelines for Good Clinical Practice and the Declaration of Helsinki (along with its latest amendments). All participants gave their written informed consent prior to inclusion in the study. Participants were not eligible to participate in case of current drug use, use of psychoactive medication, physical or mental illness, smoking, any previous intestinal surgery, any chronic intestinal condition, any speech, language or swallowing disorder, not being fluent in Dutch or having a magnetic resonance imaging (MRI) appointment shortly after participation. Exclusion criteria on the day of participation were feeling sick, having fever, consuming any caffeine, carrying out heavy exercise, or having consumed a heavy meal. Additionally, participants with any recent intake of psychoactive substances were excluded with a urine multi-drug screening device (InstantView) which tested for amphetamines (including MDMA), barbiturates, cannabinoids, benzodiazepines, cocaine and opiates.
Experimental protocol
Study 1
All participants were exposed to the Trier Social Stress Test as described (see Trier Social Stress Test (TSST)). Throughout the session, heart rate and respiration rate (Suunto T6, Vantaa, Finland), intestinal temperature (see Core body temperature) and skin temperature (see Peripheral temperature) on the upper arm were continuously measured. Also, at distinct time points, blood pressure was measured (Microlife BPA 100, Widnau, Switzerland) and saliva samples were obtained for cortisol analysis. For one participant, temperature measurements were not obtained due to technical difficulties. For two additional participants, heart rate and respiration rate levels were not obtained due to technical difficulties. Saliva cortisol levels could not be determined for two participants due insufficient saliva quantity.
Study 2
Participants completed both the control protocol (see Control condition) and the stress protocol (see Trier Social Stress Test (TSST)) on two separate consecutive days. Because of this within-subject design, the stress condition was always carried out on the first day to prevent anticipatory stress due to knowledge of the stress condition that can be gathered from the control condition. Both experimental days were started at the same time for all participants. All rooms were under controlled temperature (20 ± 2 °C). Participants were asked to stand up 10 min prior to the TSST to exclude the acute interference of change of position (orthostatic reaction) in response to the TSST. After completion of the TSST or the control condition, participants completed the same protocol in a sedentary position while reading available reading material.
Trier Social Stress Test (TSST)
The TSST was carried out in studies 1 and 2 as described earlier (Kirschbaum et al., Citation1993). Briefly, a participant was led to a room where he/she was instructed to stand behind a microphone in front of a committee, consisting of one man and one woman. Participants were then instructed to prepare (3 min) and deliver (5 min) a speech for a job interview, followed by a mental arithmetic task (5 min). Before starting the speech, the participant was informed that the whole session would be videotaped and voice recorded and that the committee was trained in behavioral observation.
Control condition
In study 2, a control condition of the TSST was carried out as described previously (Het et al., Citation2009). In short, this procedure contained a free speech (8 min) and a simple mental arithmetic task (5 min) without a committee being present. The duration of the control condition was identical to that of the TSST (around 13 min). Participants were informed of the control aspect of the task. Therefore, the stress-inducing factors of uncontrollability and being recorded and socially evaluated were minimized.
Measures
Core body temperature
In studies 1 and 2, the intestinal core temperature was continuously measured at 1 min intervals using an ingestible radiotelemetric capsule (Respironics, Bend, OR). The measured temperature from the capsule constitutes a validated representation of core temperature (Byrne & Lim, Citation2007). The capsule was ingested approximately 1 h prior to the experimental condition. In study 2, temporal artery temperature was non-invasively measured at 10 time points throughout both experimental conditions (at −60 min, −10 min, −2 min, +15 min, +25 min, +35 min, +45 min, +60 min, +90 min and +120 min) using an infrared temporal thermometer (type TAT5000, Exergen, MA). The temporal thermometer records core temperature using an infrared reading of superficial temporal artery blood flow (Fetzer & Lawrence, Citation2008).
Peripheral body temperature
In study 1, skin temperature on the upper arm was measured using a radiotelemetric skin patch (Respironics, Bend, OR). In study 2, skin temperature was assessed at multiple sites using wireless iButtons (type DS1922L, Maxim/Dallas, TX) that were set to acquire skin temperature every 2 min with a resolution of 0.0625 °C. This method of skin temperature monitoring has been validated and described in detail elsewhere (van Marken Lichtenbelt et al., Citation2006). Specifically, skin temperature in study 2 was measured at seven different locations. Distal skin temperature was obtained from the palmar side of the finger tip, the dorsal side of the base of the middle finger and the hand palm. Also, skin temperature was measured at the dorsal side of the wrist which may represent both proximal and distal skin temperature (Romeijn & Van Someren, Citation2011). Proximal skin temperature was assessed on the upper arm, the infra-clavicular area and the stomach area. The iButtons were affixed with adhesive tape (Fixomull stretch, BSN medical GmbH). Due to technical problems, stomach skin temperature of one female participant and upper arm temperature of one male participant were discarded from analysis.
Skin temperature of the visible face was continuously measured in study 2. Thermography was performed with a calibrated IR thermal camera (FLIR ThermoCam SC640, Seattle, WA), uncooled micro-bolometer with a 640 × 480 pixel array. The sensor has a 14-bit dynamic range and a spectral sensitivity from 7.5 to 13.5 μm. The data were recorded and analyzed with the ThermaCamTMResearcher 2007 Pro 2.9 (FLIR systems AB, Sweden) software coupled to an IEEE-1394 FireWire interface with a laptop PC. The data were collected with a temperature resolution of 0.1 degree Kelvin and the thermal images were acquired with a 1 s interval. Data was reduced to twenty-six 20-s intervals. Skin temperature was determined on predefined locations on the face: the forehead, nose, cheek, the medial skin of the eye and the neck (see and ). Participants with facial parts that were not continuously visible (e.g. due to hair style and glasses) were excluded from facial part analysis. Due to technical difficulties, infrared facial temperature data of two participants were incomplete and discarded from analysis.
Table 1. Size and area of facial regions of interest for infrared temperature measurement Calculations in study 2. Regions are also depicted in .
Perceived stressfulness
In study 2, before and after both experimental conditions, participants rated their state anxiety using the state questionnaire of the State and Trait Anxiety Inventory (STAI) (Spielberger, Citation1989). In addition to pre- and post-experimental STAI assessments, perceived stress, anxiety, insecurity and warmth levels were recorded during both experimental conditions on visual analog scales (VAS, 100 mm scale).
Calculated body skin temperature changes
In addition to analysis on the raw temperature data over time, stress-induced changes in body skin temperature were calculated in study 2 to more clearly visualize the effects of stress on body skin temperature. Temperature changes were calculated by subtracting the value at the end of the experimental condition (t = +15 min) from the baseline value (the average temperature from t = –3 min until t = 0 min).
Cortisol levels
To ascertain a hormonal stress response in response to the TSST, saliva samples were collected in study 1 (Salivette, Sarstedt, Nümbrecht, Germany). In total, eight saliva samples were collected at baseline, during the stress condition, and at various time points afterwards. Samples were stored at −20 °C until analysis. Cortisol in saliva was measured without extraction using an in house competitive radioimmunoassay employing a polyclonal anti-cortisol-antibody (K7348). [1,2-3H(N)]-Hydrocortisone (PerkinElmer NET396250UC) was used as a tracer. The lower limit of detection was 1.0 nmol/l and inter-assay variation was <6% at 4–29 nmol/l (n = 33). Intra-assay variation was <4% (n = 10). Samples with levels >100 nmol/L were diluted 10× with assay buffer.
Statistics
All statistics were carried out using SPSS version 17 (SPSS Inc., Chicago, IL). Raw temperature data was analyzed using repeated-measures ANOVAs. Since all parameters were repeatedly measured, time was included as a within-subject factor in the analyses. Sex was included as a between-subject factor. In study 2, since participants completed both the stress and control condition, the within-subject factor stress was added with the two levels stress and control over the two experimental days. A significant stress effect indicates a main effect of experimental day (stress or control condition), whereas a significant time effect indicates changes over time independent of the experimental condition. Simple contrast tests were used to compare time points for blood pressure and cortisol levels in study 1 whenever a significant main effect or a significant parameter × time interaction effect was observed.
The period of 60 min following the initiation of the experimental condition was used for analyses of intestinal and skin temperature in studies 1 and 2. The period of 30 min before the initiation of the TSST until 30 min afterwards was used for analyses of heart rate and respiration data in study 1. For facial skin temperature, the period during the experimental condition was included using twenty-six 20 s time points. Temporal artery temperature was obtained during and after both experimental conditions at ten discrete time points. Calculated body skin temperatures changes were analyzed using repeated measures ANOVA with stress or control task as within-subject factor and sex as between-subject factor (study 2). The significance level was set at p < 0.05. All results shown are the mean ± standard error of mean (SEM).
Results
Study 1
Intestinal temperature
Changes in intestinal temperature in response to stress were dependent on sex (time × sex interaction F59,1239 = 1.82, p < 0.001) (). Separate analysis of both sexes revealed that female intestinal temperature did not change in response to stress (time effect F59,649 = 0.93, p = 0.63), whereas male intestinal temperature decreased in response to stress (time effect F59,590 = 8.23, p < 0.001).
Figure 1. Effects of the Trier Social Stress Test in on intestinal temperature (A), upper arm skin temperature (B), heart rate (C), respiration rate (D), saliva cortisol (E) and blood pressure (F) in healthy male and female individuals (study 1). *Time effect (*p < 0.05; ***p < 0.001). In panel A--B, symbols indicate significance from t = 0 until +60 min, whereas in panel C--D, symbols indicate significance from −30 until +30 min.
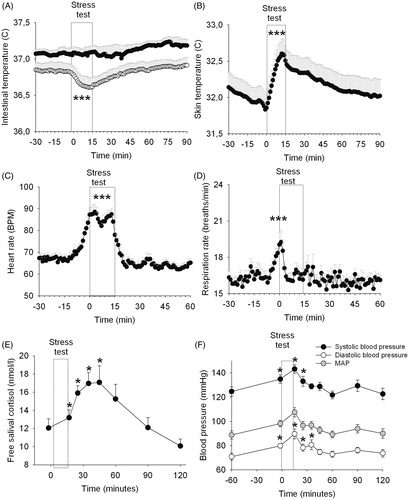
Skin temperature
Skin temperature on the upper arm increased in response to stress (time effect, F59,1239 = 9.94, p < 0.001) (). This effect was not dependent on sex (time × sex interaction F59,1239 = 1.01, p = 0.46; sex effect F1,21 = 1.08, p = 0.31).
Heart rate
The heart rate changed over time (time effect F59,1121 = 25.46, p < 0.001), which was independent of sex (time × sex interaction F59,1121 = 0.49, p = 0.81) (). The average maximum was 23 ± 2 beats per minute (bpm) (male: 21 ± 3 bpm; female: 24 ± 3 bpm).
Respiration rate
The average respiration rate increased after stress exposure (time effect F59,1121 = 2.59, p < 0.001) which was independent of sex (time × sex interaction F59,1121 = 0.81, p = 0.85) (). Average respiration rate levels increased with 5 ± 1 bpm (male: 4 ± 1 bpm; female: 6 ± 1 bpm)
Salivary cortisol
Saliva cortisol levels increased after stress exposure (time effect F7,140 = 8.41, p < 0.001) independent of sex (cortisol × sex interaction F7,140 = 1.44, p = 0.20; sex effect F1,20 = 0.33, p = 0.57) (). Simple contrasts revealed that cortisol levels increased at t = 15, t = 25, t = 35 and t = 45 min compared to the baseline value (t = −2 min), (p < 0.05).
Blood pressure
Both systolic and diastolic blood pressure increased in response to stress (diastolic: F8,176 = 14.07, p < 0.001; systolic: F8,176 = 15.92, p < 0.001) (). Simple contrasts revealed that compared to the first measurement at t = −60 min, diastolic and systolic blood pressure were elevated at t = −2 min, t = 15 min and t = 25 min, whereas only diastolic blood pressure was still increased at t = 35 min (p < 0.05). No sex effects were present in either the response of diastolic or systolic blood pressure (diastolic: sex effect F1,22 = 0.46, p = 0.50; sex × time interaction F8,176 = 1.32, p = 0.24; systolic: sex effect F1,22 = 3.17, p = 0.09; sex × time interaction F8,176 = 0.89, p = 0.53)
Study 2
Core temperature
Intestinal temperature
Stress decreased intestinal temperature over time (stress × time interaction F59,1180 = 3.48, p < 0.001; stress effect p > 0.05). Analysis of the temperature data over time showed that female participants displayed an overall significantly higher intestinal temperature (sex effect F1,20 = 19.22, p < 0.0001). However, sex did not influence the stress-induced decrease in intestinal temperature (stress × sex interaction p > 0.05; stress × time × sex interaction p > 0.05). In the light of the overall sex effect on intestinal temperature, sexes were subsequently separately analyzed. Supporting a sex-independent decrease in response to stress, stress time-dependently decreased intestinal temperature in male participants (stress × time interaction F59,531 = 2.01, p < 0.01, ) and female participants (stress × time interaction F59,649 = 2.06, p < 0.01, ).
Temporal artery temperature
Temporal artery temperature was not affected by stress (p > 0.05), even though temperature changed significantly over time (time effect F9,180 = 2.58, p < 0.01) and overall, male participants possessed a significantly higher arterial temperature (sex effect F1,20 = 3.72, p < 0.05) ().
Peripheral temperature
Skin temperature
Finger tip: On the finger tip, stress significantly decreased skin temperature over time to a larger extent compared to the control condition (stress × time interaction F30,600 = 5.23, p < 0.001), in accordance with a significantly greater calculated decline in skin temperature (stress effect F1,20 = 14.20, p < 0.001) ().
Figure 4. Effects of stress on skin temperature (study 2). *Significant effect of stress compared to the control condition from t = 0 min until t = 60 min. The white box from t = 10 to t = 15 indicates the experimental condition (stress/control). The dashed line indicates change from sitting to standing position 10 min prior to the experimental condition. The inset displays the calculated temperature change in the stress and control condition. *p < 0.05; **p < 0.01; ***p < 0.001. In all panels, symbols indicate significance from t = 0 until + 60 min.
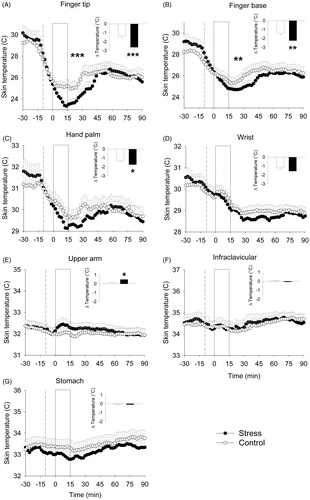
Finger base: On the finger base, a significant stress-induced decline in skin temperature was present (stress x time interaction F30,600 = 1.69, p < 0.01), confirmed by a significantly greater calculated decline in temperature after stress (stress effect F1,20 = 8.38, p < 0.01) ().
Hand palm and wrist: Stress did not significantly affect skin temperature over time both on the palm of the hand (stress effect F1,20 = 1.20, p = 0.29; stress × time interaction F30,600 = 0.54, p = 0.71) or on the wrist (stress effect F1,20 = 22.09, p = 0.32; stress × time interaction F30,600 = 0.18, p = 0.88). However, the calculated average temperature alteration showed that hand palm temperature appeared to be significantly decreased in response to stress (stress effect F1,20 = 9.13, p < 0.05), whereas for the wrist, this effect did not reach statistical significance (stress effect F1,20 = 3.91, p = 0.07) ().
Upper arm: On the upper arm, stress did not significantly increase upper arm temperature over time (stress × time interaction F30,570 = 1.44, p = 0.08), even though the calculated change in upper arm temperature did significantly increase (stress effect: F1,19 = 5.66, p < 0.05). An additional analysis of the upper arm temperature during the first 30 min after stress exposure showed a significant stress-induced temperature increased compared to the control condition (stress × time interaction F15,285 = 1.92, p < 0.05) ().
Infra-clavicular and stomach area: There were no significant effects of stress on skin temperature in the infra-clavicular area (stress effect F1,20 = 42.91, p = 0.46; stress × time interaction F30,600 = 5.68, p = 0.48) nor in the stomach area (stress effect F1,20 = 1.69, p = 0.22; Stress × time interaction F30,600 = 0.13, p = 0.89) (). Also, there was no significant change in calculated temperature in both areas (infra-clavicular: stress effect F1,20 = 2.05, p = 0.17; stomach: stress effect F1,19 = 0.01, p = 0.94; , inset).
Skin facial temperature
Nasal temperature: Stress significantly affected nasal temperature over time (stress × time interaction F25,425 = 9.22, p < 0.001) (n = 19, ). The stress effect over time was sex-dependent (stress × time × sex interaction F25,425 = 5.56, p < 0.001). Stratified analysis of the sexes revealed that this effect was due to stress effects in female participants (stress × time interaction F25,250 = 12.90, p < 0.001) but not in male participants (stress effect F1,7 = 0.08, p = 0.79, stress × time interaction F25,175 = 0.94, p = 0.55)
Figure 5. Effects of stress on facial skin temperature (study 2). The regions of interest which have been used are displayed in (see also ). *Stress effect compared to the control test over all time points (*p < 0.05; **p < 0.01; ***p < 0.001).
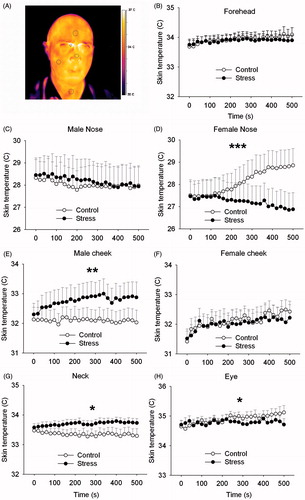
Cheek temperature: Cheek temperature was significantly influenced by stress in a sex-specific manner (stress × time × sex interaction F25,425 = 1.52, p = 0.05) (n = 19, ). No main stress effect (F1,17 = 0.97, p = 0.34) nor a time-dependent stress effect (F25,425 = 0.84, p = 0.69) was present. Separate analysis of both sexes showed that in male participants, cheek temperature significantly increased over time after stress induction (stress × time interaction F25,175 = 1.99, p < 0.01), but that this effect was absent in female participants (stress effect F1,10 = 0.05, p = 0.84, stress × time interaction F25,250 = 0.96, p = 0.52).
Neck temperature: Stress significantly increased overall neck temperature in all participants (stress effect F1,14 = 5.11, p < 0.05) which did not depend on sex (stress × sex interaction F1,14 = 0.42, p = 0.53) nor on time (stress × time interaction F25,350 = 0.82, p = 0.72) (n = 16, ).
Eye temperature: Stress significantly decreased skin temperature on the nose side of the right eye which developed over time (stress × time interaction F25,275 = 1.63, p < 0.05) but was not dependent on sex (stress × sex interaction F1,11 = 0.09, p = 0.77) (n = 13, ). No significant stress effect was present (stress effect F1,11 = 0.40, p = 0.54).
Forehead temperature: No significant effect of stress was found on the forehead temperature (stress effect F1,15 = 0.15, p = 0.70; stress × time interaction F25,375 = 0.69, p = 0.87) (n = 17, ).
Subjective stress levels
Compared to the control condition, state anxiety levels as measured by the STAI significantly increased after stress exposure compared to baseline levels (stress × test interaction F1,20 = 17.80, p < 0.001) (). This effect was not sex-dependent (stress × time × sex interaction F1,20 = 0.037, p = 0.85), although female participants reported overall higher anxiety scores (stress × sex interaction F1,20 = 4.69, p < 0.05). Stratified analysis of the stress condition revealed that the TSST increased anxiety levels (F1,20 = 15.86, p < 0.001). Visual analog scales taken during the experimental condition indicated that stress significantly increased perceived stress levels (F1,20 = 79.79, p < 0.001), anxiety levels (F1,20 = 27.28, p < 0.001), insecurity levels (F1,20 = 80.84, p < 0.001), and feelings of increased warmth (F1,20 = 24.87, p < 0.001) (). Perceived stress levels with VAS were independent of sex (sex effect F1,20 = 0.33, p = 0.58; sex × test interaction F1,20 = 0.003, p = 0.96). None of the stress-induced changes in VAS or STAI significantly correlated with computed changes in stress-induced skin temperature.
Table 2. Effects of stress on perceived subjective state levels in study 2 as measured by the Spielberger State and Trait Anxiety Inventory (STAI) scores and visual analog scales (VAS).
Discussion
The present study investigated the effects of stress on human body temperature using a standardized laboratory stress task. First, we report that intestinal core temperature significantly decreases in response to stress ( and ). Second, stress-induced changes in skin temperature were observed on the body and face. The magnitude and direction of these skin temperature changes largely depended on sex and measurement location. Third, stress exposure resulted in skin temperature changes which followed a distinct gradient-like pattern with temperature decreases at distal skin locations such as the fingertip and finger base while proximal regions such as the infraclavicular and upper arm sites remain unchanged.
The finding that intestinal core temperature decreased in response to stress is surprising, as rodent core temperature generally increases in response to stress, at least when the body temperature is measured using either rectal temperature probes or telemetric equipment located in the abdominal cavity (Bouwknecht et al., Citation2007; Vinkers et al., Citation2008). In chimpanzees, tympanic membrane temperature increased after stress exposure (Parr & Hopkins, Citation2000a). The fact that we measured core body temperature inside the lumen of the small intestine may at least partially explain this discrepancy. Thus, it may be hypothesized that vasoconstriction in the area of the small intestine decreases apparent intestinal temperature, whereas a stress-induced vasodilatation could be present in the colon and/or peritoneal cavity. In support, superior mesenteric blood flow has been found to be reduced during a arithmetic stress task (Kuipers et al., Citation2008). Our findings are also compatible with the concept of decreased gastric and small intestinal activity in response to stress when a fight-or-flight response is initiated (Martinez et al., Citation2004). This way, preclinical stress-induced increases in temperature in the abdominal cavity may then be the result of increased metabolic activity of abdominal organs including the liver, whereas stress-induced activation of the colon (see Tache et al., Citation2005) results in increased colon evacuation and increases rectal temperature. Even though it may be speculated that abdominal organs including the liver actively produce heat in response to stress, studies addressing this specific topic are lacking.
In addition to stress-induced activation of the autonomic nervous system, various studies have proposed that stress-induced changes in body temperature represent a psychogenic fever accompanied by an increased temperature set point (Briese & Cabanac, Citation1991; Hiramoto et al., Citation2009; Oka & Oka, Citation2007). However, there is convincing evidence that stress-induced temperature changes and infection-induced fever are two distinct processes (Vinkers et al., Citation2009). In the present study we did not investigate the mechanisms underlying the stress-induced temperature changes. Therefore, the neuronal pathways ultimately responsible for the changes in core temperature remain to be elucidated, and future studies could measure immune and (para)sympathetic factors that accompany stress-induced temperature changes. Also, we cannot exclude the possibility that the late drop in core temperature represents an after-effect from a transient fever state, even though we did not find indications for such a fever state in our measurements. In support, stress exposure did not influence temporal artery temperature (), even though this core temperature parameter is non-invasively measured and could be subject to measurement or calculation errors. Infrared thermometry automatically measures the temperature of the skin surface over the temporal artery and the ambient temperature. By repeatedly sampling, the highest temperature (peak) is obtained as the arterial temperature. In support, the difference between temporal and esophageal temperature was within 0.4 °C, and temporal artery thermometry was found to be more accurate compared to oral temperature measurements (Calonder et al., Citation2010). In another study, temporal artery and rectal temperature measurements yielded similar results in comparison to esophageal temperature (Al-Mukhaizeem et al., Citation2004).
Stress exposure resulted in skin temperature changes which followed a distinct gradient-like pattern with larger temperature decreases at more distal skin locations such as the fingers (). An apparent but non-significant increase in skin temperature over the deltoid muscle during stress exposure may be the result of both cutaneous vasodilatation as well as increased muscular metabolic rates. A number of studies have addressed the effects of stress on peripheral body temperature in humans. These studies differ in their setup, stress-induction method as well as site of temperature measurements. In two early studies, the axillary temperature of 1068 and 1374 students was increased before their exams (Gotsev & Ivanov, Citation1950, Citation1954). In agreement with our study, peripheral finger temperature decreased when listening to “negative” music (McFarland, Citation1985) or in affective states (Mittelmann & Wolff, Citation1939). In a more recent study, the axillary temperature was increased in medical residents before an important exam (Marazziti et al., Citation1992). Moreover, the oral temperature of 108 students was increased before a difficult exam compared to a non-stressful situation (Briese, Citation1995). Several decades ago, it was already found that male participants who watched or participated in a boxing contest displayed elevated oral temperatures (Renbourn, Citation1960). Both studies show an association between the stress-induced body temperature increase and the subsequent performance. Thus, even though we did not measure any performance-related outcomes in our study, it may speculated that the extent to which body temperature changes in response to stress may be related to task performance following stress. However, this relation is complex as we found no association between stress-induced body temperature and the psychological (subjective) response to the TSST. This is in line with known literature on the relation between the physiological (cortisol) and psychological (subjective) stress response (Campbell & Ehlert, Citation2012). Supporting a role for stress-induced vasodilation, mental stress has been found to increase forearm vascular conductance (Klein et al., Citation2010). A complicating factor in comparing stress-induced temperature changes in the present study to the literature is the heterogeneity in applied stress paradigms. This precludes a direct comparison of the different paradigms with regard to their level of stress induction. However, the TSST is an extensively validated laboratory stressor and resulted in robust increases in saliva cortisol levels in our study, indicating that a sufficiently high level of stress induction was established.
With regard to the underlying neurobiological pathways, the hypothalamus has been suggested to be involved in the drop of tail temperature after exposure to restraint stress in preclinical studies using rats and rabbits (Blessing, Citation2003; Busnardo et al., Citation2010). From our results, it is apparent that an orthostatic-medicated change in blood flow and skin temperature was apparent as standing up 10 min prior to either the TSST or the control condition decreased distal skin temperature (). This is in accordance with known results of the effect postural changes on skin temperature (Tikuisis & Ducharme, Citation1996; Van Someren, Citation2006). Such orthostatic changes in temperature have also been described for rectal temperature measurements (Tikuisis & Ducharme, Citation1996). However, in the present study, the control condition did not alter the core temperature, making a postural factor in the stress-induced decrease in intestinal temperature less likely. A relationship between behavioral activity and body temperature in our study is unlikely as participants were sedentary after both the stress and control condition.
Stress-induced effects on facial temperature which was tracked using an infrared camera followed a more sex-specific pattern (). Compared to the control condition, nasal skin temperature decreased in female participants whereas cheek temperature increased in male participants. Nevertheless, this is not necessarily contradictory as the result in both sexes was an increased temperature gradient between the cheek and nose. In male and female individuals, neck skin temperature increased and skin temperature on the medial side of the eye decreased. In support, nasal temperature decreased during and shortly after a threatening situation in rhesus monkeys (Nakayama et al., Citation2005). The current findings possess apparent face validity as stressful daily life situations are known to cause flushing of neck and cheek areas.
A strength of this study is the use of multiple core and peripheral body temperature measures. A limitation of our study is the relatively small sample size which limited the power to reveal possible stress-induced changes in body temperature, and missing endocrine and classical autonomic response measures such as cortisol, alpha-amylase and heart rate in study 2. However, exposure to stress was accompanied by increases in subjective measures of stress. Also, in study 1, stress exposure led to increases of heart rate, blood pressure, respiration rate and cortisol levels independent of sex (). These data are in agreement with previous studies on the TSST (Kirschbaum et al., Citation1993; Kudielka et al., Citation2004), and suggest that our applied protocol of the Trier Social Stress Test was able to induce an autonomic and endocrine stress response. Another important limitation of our design is the fact that the order of experimental condition in study 2 was not randomized and that the control condition was always carried out on the second testing day. The fixed order was established to prevent stress-attenuating effects or anticipation stress as the control condition may reveal important aspects of the aim and nature of the TSST. Whereas the cortisol stress response is sensitive to habituation, the autonomic stress response as measured by (nor)epinephrine levels has been found not to habituate following repeated stress exposure (Schommer et al., Citation2003). Since autonomic parameters such as heart rate and epinephrine levels have not been investigated in study 2, the role of habituation on the autonomic stress response remains to be elucidated. Female individuals in the present study used oral contraceptives which are associated with a blunted cortisol response after exposure to stress (Kudielka & Kirschbaum, Citation2005). This blunted cortisol response has been ascribed to differences in levels of corticosteroid-binding globulin (Hellhammer et al., Citation2009). Oral contraceptives can also influence circadian body temperature (Baker et al., Citation2001) and its use may therefore also have played a role in the reported sex differences. A role for gonadal hormones in thermoregulation is supported by the fact that during the transition to menopause, perimenopausal women may experience hot flushes associated with declining levels of gonadal hormones (Freeman et al., Citation2005). However, the effects of oral contraceptives on stress-induced body temperature have not been investigated, and there is currently no good explanation for the apparent sex differences in intestinal temperature and nose/cheek temperature.
In conclusion, the present study reports that stress decreases intestinal temperature in healthy human participants accompanied by skin temperature changes which distally decrease but proximally either increase or remain unchanged. Overall, we report consistent stress-induced changes in human body temperature, indicating that body temperature may be used as an autonomic readout parameter of stress. However, our results suggest that a direct translation of the preclinical stress-induced hyperthermia paradigm to a human version is not possible. The present study shows that the effect of stress on human body temperature depends on the applied method and the site of measurement and cannot directly be compared to the preclinical situation where rectal and abdominal temperature invariably increase after stress exposure (Vinkers et al., Citation2008). Nevertheless, the consistent effects of stress on body temperature in humans support the use of body temperature as a physiological readout parameter of stress.
Declaration of interest
Joris Verster has received research support from Takeda Pharmaceuticals and Red Bull GmbH and has acted as scientific advisor for Takeda, Sanofi-Aventis, Transcept, Sepracor, Red Bull GmbH, Deenox, Trimbos Institute, CBA and CBD. All other authors declare no conflict of interest.
Acknowledgements
The authors would like to thank Anne Daamen, Marieke Ebbens, Martje de Groot, Eva Hoff, Monique Mets and Emily van der Steen for their help with the stress protocol.
References
- Ahmed MU, Begum S, Funk P, Xiong N, von Scheele B. (2010). A multi-module case-based biofeedback system for stress treatment. Artif Intell Med 51(2):107–15
- Al-Mukhaizeem F, Allen U, Komar L, Naser B, Roy L, Stephens D, Read S, et al. (2004). Comparison of temporal artery, rectal and esophageal core temperatures in children: Results of a pilot study. Paediatr Child Health 9(7):461–5
- Baker FC, Waner JI, Vieira EF, Taylor SR, Driver HS, Mitchell D. (2001). Sleep and 24 hour body temperatures: a comparison in young men, naturally cycling women and women taking hormonal contraceptives. J Physiol 530(Pt 3):565–74
- Blessing WW. (2003). Lower brainstem pathways regulating sympathetically mediated changes in cutaneous blood flow. Cell Mol Neurobiol 23(4–5):527–38
- Bouwknecht JA, Olivier B, Paylor RE. (2007). The stress-induced hyperthermia paradigm as a physiological animal model for anxiety: a review of pharmacological and genetic studies in the mouse. Neurosci Biobehav Rev 31(1):41–59
- Briese E. (1995). Emotional hyperthermia and performance in humans. Physiol Behav 58(3):615–18
- Briese E, Cabanac M. (1991). Stress hyperthermia: physiological arguments that it is a fever. Physiol Behav 49(6):1153–7
- Busnardo C, Tavares RF, Resstel LB, Elias LL, Correa FM. (2010). Paraventricular nucleus modulates autonomic and neuroendocrine responses to acute restraint stress in rats. Auton Neurosci 158(1–2):51–7
- Byrne C, Lim CL. (2007). The ingestible telemetric body core temperature sensor: a review of validity and exercise applications. Br J Sports Med 41(3):126–33
- Calonder EM, Sendelbach S, Hodges JS, Gustafson C, Machemer C, Johnson D, Reiland L. (2010). Temperature measurement in patients undergoing colorectal surgery and gynecology surgery: a comparison of esophageal core, temporal artery, and oral methods. J Perianesth Nurs 25(2):71–8
- Campbell J, Ehlert U. (2012). Acute psychosocial stress: does the emotional stress response correspond with physiological responses? Psychoneuroendocrinology 37(8):1111–34
- Daimon K, Yamada N, Tsujimoto T, Takahashi S. (1992). Circadian rhythm abnormalities of deep body temperature in depressive disorders. J Affect Disord 26(3):191–8
- Falcon-Lesses M, Proger SH. (1930). Psychogenic fever. N Engl J Med 203:1034–6
- Fetzer SJ, Lawrence A. (2008). Tympanic membrane versus temporal artery temperatures of adult perianesthesia patients. J Perianesth Nurs 23(4):230–6
- Freeman EW, Sammel MD, Lin H, Gracia CR, Kapoor S, Ferdousi T. (2005). The role of anxiety and hormonal changes in menopausal hot flashes. Menopause 12(3):258–66
- Gotsev T, Ivanov A. (1954). Elevation of body temperature in students during examinations. Acta Physiol Hung 6(4):427–31
- Gotsev T, Ivanov A. (1950). Psychogenic elevation of body temperature in healthy persons. Acta Physiol Hung 1(1):53–62
- Hellhammer DH, Wust S, Kudielka BM. (2009). Salivary cortisol as a biomarker in stress research. Psychoneuroendocrinology 34(2):163–71
- Het S, Rohleder N, Schoofs D, Kirschbaum C, Wolf OT. (2009). Neuroendocrine and psychometric evaluation of a placebo version of the ‘Trier Social Stress Test’. Psychoneuroendocrinology 34(7):1075–86
- Hiramoto T, Oka T, Yoshihara K, Kubo C. (2009). Pyrogenic cytokines did not mediate a stress interview-induced hyperthermic response in a patient with psychogenic fever: a case report. Psychosom Med 71(9):932–6
- Kirschbaum C, Pirke KM, Hellhammer DH. (1993). The ‘Trier Social Stress Test’ -- a tool for investigating psychobiological stress responses in a laboratory setting. Neuropsychobiology 28(1–2):76–81
- Klein JC, Crandall CG, Brothers RM, Carter JR. (2010). Combined heat and mental stress alters neurovascular control in humans. J Appl Physiol 109(6):1880–6
- Kleitman N, Jackson DP. (1950). Body temperature and performance under different routines. J Appl Physiol 3(6):309–28
- Korte SM, Koolhaas JM, Wingfield JC, McEwen BS. (2005). The Darwinian concept of stress: benefits of allostasis and costs of allostatic load and the trade-offs in health and disease. Neurosci Biobehav Rev 29(1):3–38
- Kudielka BM, Kirschbaum C. (2005). Sex differences in HPA axis responses to stress: a review. Biol Psychol 69(1):113–32
- Kudielka BM, Schommer NC, Hellhammer DH, Kirschbaum C. (2004). Acute HPA axis responses, heart rate, and mood changes to psychosocial stress (TSST) in humans at different times of day. Psychoneuroendocrinology 29(8):983–92
- Kuipers NT, Sauder CL, Carter JR, Ray CA. (2008). Neurovascular responses to mental stress in the supine and upright postures. J Appl Physiol 104(4):1129–36
- Lack LC, Gradisar M, Van Someren EJ, Wright HR, Lushington K. (2008). The relationship between insomnia and body temperatures. Sleep Med Rev 12(4):307–17
- Marazziti D, Di Muro A, Castrogiovanni P. (1992). Psychological stress and body temperature changes in humans. Physiol Behav 52(2):393–5
- Martinez V, Wang L, Rivier J, Grigoriadis D, Tache Y. (2004). Central CRF, urocortins and stress increase colonic transit via CRF1 receptors while activation of CRF2 receptors delays gastric transit in mice. J Physiol 556(Pt 1):221–34
- McFarland R. (1985). Relationship of skin temperature changes to the emotions accompanying music. Biofeedback Self-Regulation 10(3):255–67
- Mittelmann B, Wolff H. (1939). Affective states and skin temperature: experimental study of subjects with “cold hands” and Raynaud's syndrome. Psychosom Med 1(2):271–92
- Nakayama K, Goto S, Kuraoka K, Nakamura K. (2005). Decrease in nasal temperature of rhesus monkeys (Macaca mulatta) in negative emotional state. Physiol Behav 84(5):783–90
- Oka T, Oka K. (2007). Age and gender differences of psychogenic fever: a review of the Japanese literature. Biopsychosoc Med 1:11
- Parr L, Hopkins W. (2000a). Brain temperature asymmetries and emotional perception in chimpanzees, Pan troglodytes. Physiol Behav 71:363–71
- Parr LA, Hopkins WD. (2000b). Brain temperature asymmetries and emotional perception in chimpanzees, Pan troglodytes. Physiol Behav 71(3–4):363–71
- Renbourn ET. (1960). Body temperature and pulse rate in boys and young men prior to sporting contests. A study of emotional hyperthermia: with a review of the literature. J Psychosom Res 4:149–75
- Romeijn N, Van Someren EJ. (2011). Correlated fluctuations of daytime skin temperature and vigilance. J Biol Rhythms 26(1):68–77
- Schommer NC, Hellhammer DH, Kirschbaum C. (2003). Dissociation between reactivity of the hypothalamus-pituitary-adrenal axis and the sympathetic-adrenal-medullary system to repeated psychosocial stress. Psychosom Med 65(3):450–60
- Shiloh R, Munitz H, Portuguese S, Gross-Isseroff R, Sigler M, Bodinger L, Katz N, et al. (2005). Corneal temperature in schizophrenia patients. Int J Neuropsychopharmacol 8(4):537–47
- Shiloh R, Weizman A, Stryjer R, Kahan N, Waitman DA. (2009). Altered thermoregulation in ambulatory schizophrenia patients: a naturalistic study. World J Biol Psychiatry 10(2):163–70
- Spielberger C. (1989). State-trait anxiety inventory: a comprehensive bibliography. Palo Alto: Consulting Psychologists Press
- Tache Y, Million M, Nelson AG, Lamy C, Wang L. (2005). Role of corticotropin-releasing factor pathways in stress-related alterations of colonic motor function and viscerosensibility in female rodents. Gend Med 2(3):146–54
- Tikuisis P, Ducharme MB. (1996). The effect of postural changes on body temperatures and heat balance. Eur J Appl Physiol Occup Physiol 72(5–6):451–9
- van Marken Lichtenbelt WD, Daanen HA, Wouters L, Fronczek R, Raymann RJ, Severens NM, Van Someren EJ. (2006). Evaluation of wireless determination of skin temperature using iButtons. Physiol Behav 88(4–5):489–97
- Van Someren EJ. (2006). Mechanisms and functions of coupling between sleep and temperature rhythms. Prog Brain Res 153:309–24
- Vinkers CH, Groenink L, van Bogaert MJ, Westphal KG, Kalkman CJ, van Oorschot R, Oosting RS, et al. (2009). Stress-induced hyperthermia and infection-induced fever: two of a kind? Physiol Behav 98(1–2):37–43
- Vinkers CH, Penning R, Ebbens MM, Hellhammer J, Verster JC, Kalkman CJ, Olivier B. (2010). Stress-induced hyperthermia in translational stress research. The Open Pharmacology Journal 4:30–5
- Vinkers CH, van Bogaert MJ, Klanker M, Korte SM, Oosting R, Hanania T, Hopkins SC, et al. (2008). Translational aspects of pharmacological research into anxiety disorders: the stress-induced hyperthermia (SIH) paradigm. Eur J Pharmacol 585(2–3):407–25
- Yang CY, Chen CH, Chu H, Chen WC, Lee TY, Chen SG, Chou KR. (2011). The effect of music therapy on hospitalized psychiatric patients' anxiety, finger temperature, and electroencephalography: a randomized clinical trial. Biol Res Nurs 14(2):197–206