Abstract
Understanding potential sex differences in repeated stress-induced hypothalamic–pituitary–adrenocortical (HPA) axis habituation could provide insight into the sex-biased prevalence of certain affective disorders such as anxiety and depression. Therefore in these studies, male and female rats were exposed to 30 min of either audiogenic or restraint stress daily for 10 days in order to determine whether sex regulates the extent to which HPA axis hormone release is attenuated upon repeated homotypic stressor presentation. In response to the initial exposure, both stressors robustly increased plasma concentrations of both adrenocorticotropic hormone (ACTH) and corticosterone (CORT) in both sexes. Acutely, females displayed higher ACTH and CORT concentrations following restraint stress, whereas males exhibited higher hormone concentrations following loud noise stress. HPA axis hormone responses to both stressors decreased incrementally over successive days of exposure to each respective stressor. Despite the differential effect of sex on acute hormone responses, the extent to which HPA axis hormone response was attenuated did not differ between male and female animals following either stressor. Furthermore, ACTH and CORT responses to a novel environment were not affected by prior exposure to stress of either modality in either male or female rats. These experiments demonstrate that despite the acute stress response, male and female rats exhibit similar habituation of HPA axis hormones upon repeated homotypic stressor presentations, and that exposure to repeated stress does not produce exaggerated HPA axis hormone responses to a novel environment in either female or male rats.
Introduction
Habituation of hypothalamic–pituitary–adrenocortical (HPA) axis responses is typically defined as a reduction in stress-induced circulating hormone concentrations in response to repeated exposure to the same (homotypic) stress stimulus (Grissom & Bhatnagar, Citation2009; Martí & Armario, Citation1998). This is thought to be an adaptive and/or protective process, since repeated and/or sustained stress responses have been associated with the etiology of both somatic and even psychological disorders such as major depression in humans (Chrousos & Gold, Citation1998; Claes, Citation2004; Ehlert et al., Citation2001; McEwen, Citation2004; Reagan et al., Citation2008; Vanltallie, Citation2002). Several stress-influenced psychiatric illnesses are approximately twice as prevalent in women as in men, including depression (Kessler et al., Citation2005; Linzer et al., Citation1996; Van de Velde et al., Citation2010) and several anxiety disorders (Bekker & van Mens-Verhulst, Citation2007; Christiansen & Elklit, Citation2008; Ditlevsen & Elklit, Citation2010; Holbrook et al., Citation2002; Olff et al., Citation2007; Stein et al., Citation2000; Tolin & Foa, Citation2006; Vesga-López et al., Citation2008). Furthermore, both hypo- and hyper-activity of the HPA axis have been associated with these disorders (Ehlert et al., Citation2001; Jones & Moller, Citation2011). HPA axis function can also be modulated by sex steroids (Altemus et al., Citation1997, Citation2001; Kirschbaum et al., Citation1999). Therefore, differential effects of repeated or prolonged stress on HPA axis function in women compared to men could contribute to the etiology of sex-biased stress-related psychopathology.
Both loud noise and restraint presented acutely can robustly increase the plasma concentrations of the two main hormones of the HPA axis in male and female rodents: adrenocorticotropic hormone (ACTH) and corticosterone (CORT), and have long been used to study repeated stress-induced HPA axis habituation in male rats (Armario et al., Citation1984; Bhatnagar et al., Citation2002; Girotti et al., Citation2006; Grissom et al., Citation2007; Martí & Armario, Citation1997, Citation1998; Natelson et al., Citation1988; Nyhuis et al., Citation2010a,Citationb; Pace et al., Citation2001; Sasse et al., Citation2008; Weinberg et al., Citation2010). Furthermore, we have utilized repeated presentations of loud noise extensively to investigate the neural circuitry involved in habituation of HPA axis responses in male rats (Campeau & Watson, Citation1997; Campeau et al., Citation2002; Day et al., Citation2009; Masini et al., Citation2008, Citation2012). Acutely, female rats typically display robustly higher stress-induced HPA axis hormone release following a variety of stressors compared to males (Aloisi et al., Citation1994, Citation1998; Babb et al., Citation2013a; Drossopoulou et al., Citation2004; Handa et al., Citation1994; Heinsbroek et al., Citation1991; Larkin et al., Citation2010; Le Mevel et al., Citation1978; Livezey et al., Citation1985; Ogilvie & Rivier, Citation1997; Seale et al., Citation2004; Viau et al., Citation2005; Weinstock et al., Citation1998). However, we have recently demonstrated that the effect of sex on acute stress-induced HPA axis hormones appears to be stressor-specific. Specifically, while female rats display higher HPA axis hormone release to acute restraint stress, the same effect is not observed after acute loud noise stress (Babb et al., Citation2013b). Prior studies suggest that female rats display slower or less HPA axis habituation to repeated stress than male rats (Bhatnagar et al., Citation2005; Doremus-Fitzwater et al., Citation2009; Galea et al., Citation1997; Haleem et al., Citation1988; Lunga & Herbert, Citation2004). The majority of these reports, however, utilized restraint as the homotypic stressor, where a robust sex difference in acute HPA axis hormone release is typically observed. To our knowledge, no studies have compared adaptation of HPA axis hormone release in response to repeated loud noise (audiogenic) stress in male and female rats simultaneously. Utilizing loud noise as a repeated homotypic stressor provides a unique opportunity to evaluate the impact of sex on HPA axis hormone habituation since a robust and consistent effect of sex on HPA axis responses to this stressor has not been observed acutely (Babb et al., Citation2013b).
Therefore the experiments presented here compare HPA axis responses to repeated presentations of loud noise or restraint stress in male and female rats. We hypothesized that female rats, only when exhibiting significantly higher acute stress-induced HPA axis hormone release (i.e. in response to restraint, but not loud noise), would also display slower attenuation of HPA axis of hormone release over successive days of stressor exposure compared to male rats. In addition, exaggerated or facilitated HPA axis responses to a heterotypic (novel) stressor in male rats have been observed following repeated homotypic stress exposures compared to rats exposed to the same novel stressor, but not exposed to prior stress (Andrés et al., Citation1999; Bhatnagar & Dallman, Citation1998; Bhatnagar et al., Citation2003; Martí & Armario, Citation1998; Martí et al., Citation1994). It is unknown whether female rats also display facilitated HPA axis responses to a subsequent heterotypic stressor after repeated homotypic stress exposures. Thus, we also exposed male and female rats to a mild heterotypic stressor (novel environment) following the last day of repeated stressor presentation. We hypothesized that female rats exposed to either prior stressor would also exhibit facilitated HPA axis hormone responses to this mild heterotypic stressor compared to female rats not exposed to prior stress, similar to male rats.
Methods
Subjects
One hundred male and female Sprague–Dawley rats weighing 200–250 g and ∼2 months old at the time of arrival were obtained from Harlan Laboratories (Indianapolis, IN), and were initially housed in clear polycarbonate cages (48 × 27 by 20 cm) in same-sex groups of three or four with standard wood shavings bedding and ad libitum access to food (standard rat chow) and water. The colony room was maintained on a controlled 12:12 light: dark cycle (lights on at 7 am) under constant temperature and humidity. All animals were allowed to acclimate to the animal facility for 1 week prior to any experimental manipulation. To coincide with the nadir of the diurnal rhythms of both ACTH and CORT, all experimental procedures were performed between 9:00 am and 12:00 pm. All procedures were reviewed and approved by the Institutional Animal Care and Use Committee of the University of Colorado and conformed to the National Research Council’s (2011) Guide for the Care and Use of Laboratory Animals, 8th Edition.
Estrous cycle verification
After a 1-week acclimation period to the colony, experimental female rats from both studies were monitored daily for estrous cyclicity via vaginal lavage. All females used in these experiments had displayed at least two full normal 4- to 5-day estrous cycles prior to the start of the experiment. To be consistent for both sexes, experimental males in both studies were handled daily in a manner similar to vaginal lavage sampling in females: each male was suspended above the home cage by the base of the tail for ∼10–15 s. On each day of stress presentation, the predicted estrous cycle stage was verified immediately following the stressor and males were also handled in a similar manner (held by the base of the tail for 10–15 s). Cyclicity of the estrous cycle in stressed female rats was not significantly disrupted in either experiment (data not shown). For the repeated noise experiment, unstressed females had an average estrous cycle length of 4.58 ± 0.16 days, and stressed females had an average cycle length of 4.71 ± 0.36 days, and these did not differ significantly (t14 = 0.31, p = 0.76). For the restraint experiment, unstressed females had an average estrous cycle length of 4.5 ± 0.13 days and stressed females had an average estrous cycle length of 4.75 ± 0.21 days, and these did not differ significantly (t14 = −1.0, p = 0.33).
Procedure
In separate experiments, male and female rats were exposed to 10 days of daily 30-min exposures to loud noise (100 dB) or restraint stress (Stress; n = 8 rats/sex/stressor). In each experiment, other male and female rats were exposed to the same handling blood sampling procedures, but did not receive stress (No Stress Controls; n = 8 rats/sex/stressor). Prior to the first day of stressor exposure, all Stress and No Stress Control animals were individually housed in smaller clear polycarbonate cages (43 cm × 22 cm × 20 cm), and were acclimated to being moved from the colony room for five consecutive days. Racks of animal cages were wheeled down the hall from the colony room to the testing suite, and for the noise stress experiment, the home cage of each singly housed rat was placed into the same noise chamber for 30 min/day. For the restraint experiment, the home cages of singly housed rats were left on racks in an adjacent room to where restraint would take place for 30 min/day. After this 5-day acclimation period, the same procedure was followed, except half of the male and half of the female animals were exposed to stress. For both experiments, blood samples were obtained via a lateral tail vein nick in all of these groups on days 1, 3, 6 and 10 of stressor exposure, immediately after the 30-min stressor exposure. One male from the No Stress Control group in the repeated noise experiment died during the experiment, and data from this animal were excluded from analysis. On the 11th day, Stress and No Stress control animals were exposed to a novel environment for 30 min in the colony room. All animals were sacrificed immediately after the 30-min novel environment exposure, trunk blood was collected for hormone concentration determination, and thymus and adrenal glands were extracted and weighed postmortem. In each experiment, a third group of male and female rats remained untouched in the colony room over the entire 10-day stress procedure except for body weight measurements (Naïve animals; n = 9 rats/sex/stressor). Group housed naïve male and female rats were also sacrificed on day 11 and blood and tissues were also collected in these animals, but they did not receive novel environment exposure like the Stress and No Stress Control male and female rats from both experiments. These animals served as further controls for the handling/housing/blood sampling procedures to which the No Stress Controls were subjected.
Noise stress apparatus
The sound-attenuating acoustic chambers consisted of ventilated double wooden (plywood board) chambers, with the outer chamber lined internally with 2.54 cm insulation (Celotex, Suffolk, England). The internal dimensions of the inner box were 60 cm (w) × 38 cm (d) × 38 cm (h), within which a rat home cage could be placed. The middle of the ceiling of each inner chamber was fitted with a single 6 × 9 in Optimus speaker (Radio Shack, #12-1769-120 W RMS). Light within the inner chamber was provided by a florescent bulb (15 W) covered with red tissue paper in the upper left corner. Two fans (upper left and lower right corners) allowed fresh air to flow into the inner chamber. Noise was produced by a General Radio (#1381, Concord, MA) solid-state random noise generator with the bandwidth set at 2 Hz–50 kHz. The output of the noise generator was amplified (Pyramid Studio Pro #PA-600 x, Brooklyn, NY) and delivered to each speaker. The speaker characteristics allowed sound delivery between 20 and 27 000 Hz, rolling off quickly (20 dB/octave) at both ends. Noise intensity was measured directly under the speaker on the bottom of the inner chamber with a sound level meter (A scale, #33-2050, Radio Shack). The ambient noise level inside the chamber was ∼60 dB (background noise level), which was ∼5 dB above the ambient noise level in the colony rooms due to the noise from the air circulating fans within each chamber. The entire home cage of each animal (singly housed with food and water) was placed into the same noise chamber each day.
Restraint stress apparatus
Restraint was conducted in clear ventilated, cylindrical Plexiglas tubes of adjustable length. The size of the tube (23.5 cm in length and 7 cm in diameter for the males; 15.5 cm long and 6.3 cm diameter for the females) restricted movement in all directions with the rat’s tail protruding from the cylinder, but did not interfere with breathing.
Novel environment exposure
Novel environment exposure consisted of placing rats, individually, into a white circular five gallon household plastic bucket (base diameter 25 cm, height 36 cm). This method has been utilized previously as a low-intensity stressor in male rats (Campeau et al., Citation2010; Masini et al., Citation2011).
Blood collection
For tail blood collection, animals were gently held immobile using a clean towel, and a small incision was made in a lateral tail vein with the corner of a razor blade. Blood was collected using heparinized hematocrit capillary tubes deposited into ice-chilled 0.5 ml microcentrifuge tubes (for CORT assays), and also using uncoated hematocrit capillary tubes deposited into similar tubes containing EDTA (20 mg/ml; for ACTH assays). This procedure lasted <4 min to prevent detection of elevated hormone levels due to the blood collection procedure itself. These blood samples were centrifuged at 14,000 rpm at room temperature for 1 min. On the last day of the experiment, rats were decapitated and trunk blood was collected into large EDTA-coated tubes (Vacutainers). These tubes were then centrifuged at 2000 rpm for 10 min at 4 °C. For all samples, plasma was then pipetted into 0.5 ml Eppendorf microcentrifuge tubes and stored at −80 °C until assayed for hormone concentrations.
ACTH IRMA
Plasma ACTH concentrations were assayed using a commercially available immunoradiometric (IRMA) assay kit (Cat # 27130; DiaSorin, Stillwater, MN), which has a sensitivity of 1.5 pg/ml, and intra-/inter-assay variabilities of ∼4.18% and ∼4.6%, respectively. This kit recognizes 100% of whole molecule ACTH (detects all 39 amino acids), and as such is very specific, with undetectable cross-reactivity for α- and β-MSH, as well as β-Endorphin. The assay was performed according to the kit directions. Briefly, 200 µl of plasma or plasma diluted with zero standard (provided with the kit) was incubated overnight with a 125I-labelled monoclonal antibody specific for ACTH amino acids 1–17, a goat polyclonal antibody specific for ACTH amino acids 26–39, and a polystyrene bead coated with a mouse anti-goat IgG. Only ACTH in the sample with all 39 amino acids bound both antibodies to form an antibody complex on the polystyrene bead. The following day, tubes containing the radioactive beads were washed to remove any unbound reagents and the amount of radioactivity still left on each bead was determined with a gamma counter. The concentration of ACTH for each sample was calculated by fitting values for each sample into a standard curve of known ACTH dilutions processed concurrently.
CORT ELISA
Plasma CORT concentrations were analyzed using a commercially available kit (Cat. # 901-097; Assay Designs, Ann Arbor, MI), which has 100% cross-reactivity with CORT, 28.6% with deoxycorticosterone and 1.7% with progesterone. This kit has a sensitivity of 26.99 pg/ml, and intra-/inter-assay variabilities of ∼7.5% and ∼9.7%, respectively. The kit directions were followed with the exception of a modification in making the assay buffer to use a smaller volume of plasma. Specifically, the steroid displacement reagent (SDR; 0.5 µl/ml; provided with the kit) was first added to the assay buffer. Ten microliter of plasma was then diluted 1:50 with the assay buffer/SDR solution. These diluted plasma samples were then processed according to the kit directions. We previously verified that this modification from the kit directions results in assayed CORT levels equivalent to the method in the kit directions (data not shown). Then according to the kit directions, plasma samples and diluted CORT standards were incubated in a 96-well plate coated with anti-sheep antibody raised in donkey, together with CORT with a covalently attached alkaline phosphatase molecule, and a sheep polyclonal antibody for CORT. After a 2-h incubation period the plates were washed to remove all unbound reagents and a substrate was added. After 1 h, the color reaction was stopped and the intensity of color in each well was analyzed at 405 nm using a microplate reader (Biotek EL808; Winooski, VT). The concentration of CORT for each sample was calculated by fitting unknown values into a standard curve of the known CORT dilutions processed concurrently.
Statistical analysis
All repeatedly sampled data (body weight, ACTH and CORT concentrations) were analyzed first with repeated measures ANOVAs with sex and group (stress condition) also included as variables. Initial repeated measures ANOVAs on hormone concentrations were explored further with repeated measures ANOVAs for each sex separately, again including stress group as a between-subjects factor. Body weight at arrival, and change in body weight from arrival to day 10 within each experiment were also analyzed separately using two-way ANOVA with sex and stress condition as between-subjects factors. Repeated measures and two-way ANOVAs on body weight data (containing more than two stress conditions: Naïve, No Stress and Stress groups) utilized Tukey’s HSD as a post hoc test for analysis of group (stress condition) effects. ACTH and CORT concentrations were not sampled in the Naïve animals until the final day, so post hoc analyses were not necessary for those data.
Hormone responses to the novel environment were analyzed with two different two-way ANOVAs with sex and prior stress condition (No prior Stress and Prior Stress, excluding Naïve animals), and with sex and current stress condition (Exposure to novel environment (NE; combining prior stress histories) or no NE exposure) as between-subjects factors. Raw organ weights (adrenals and thymi) and body weight to organ weight ratios (percentages) were first analyzed with two-way ANOVAs with sex and stress condition (Naïve, No stress and Stress) as between-subjects factors, and Tukey’s HSD was again utilized for post hoc analysis of stress condition effects. Due to the large sex difference observed on these endpoints, effects of stress condition (Naïve, No Stress and Stress) were further explored within each sex separately with one-way ANOVAs again using Tukey’s HSD for post hoc tests. SPSS Statistics (version 18 for Windows, SPSS Inc, Chicago, IL) was used for all statistical analyses. Significance was set at p = 0.05 for all statistical tests.
Results
HPA axis hormone responses to repeated loud noise
Noise stress significantly increased both ACTH (F1,27 = 14.88, p = 0.001; ) and CORT (F1,27 = 29.22, p < 0.001; ) concentrations (main effect of stress condition). In addition, both ACTH (F3,81 = 26.38, p < 0.001) and CORT (F3,81 = 16.68, p < 0.001) concentrations decreased significantly with repeated exposure to noise (main effect of time). There was no main effect of sex on either ACTH (F1,27 = 0.08, p = 0.78) or CORT (F1,27 = 0.41, p = 0.53) concentrations.
Figure 1. Mean (±1 SEM) ACTH (A) concentrations and CORT (B) concentrations in response to 10 days of repeated noise stress in male and female rats. Significant main effects of stress condition, time and significant two-way interactions between stress condition and time, and sex and stress condition were observed for both hormones. Collapsed across time, noise stress significantly increased ACTH concentrations in males (p = 0.001) but not females (p = 0.20), and significantly higher CORT concentrations were found in females compared to males only in the control (p < 0.001) but not stressed (p = 0.15) groups. *Stressed animals significant higher than control animals, regardless of sex.
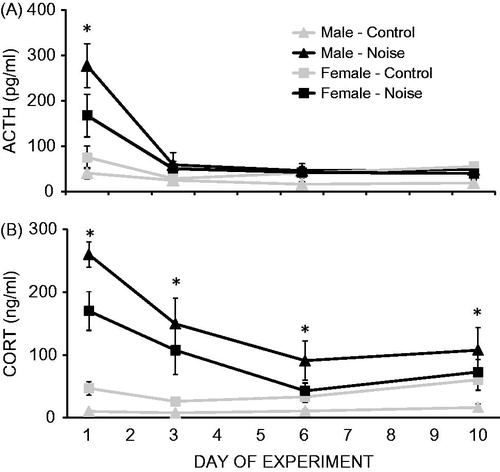
The interaction between stress condition and time was significant for both ACTH (F3,81 = 14.21, p < 0.001) and CORT (F3,81 = 16.74, p < 0.001) concentrations. Post hoc analyses collapsed across sex indicated that ACTH concentrations were significantly higher in the stressed compared to control animals only on day 1 (p = 0.0003). CORT concentrations were significantly higher in the stressed compared to control animals on every experimental day (p’s < 0.05), but the effect of stress on CORT concentrations was still significantly attenuated over the experiment (significant linear contrast; p < 0.001). There was also a significant interaction between sex and stress condition for both ACTH (F1,27 = 4.25, p = 0.049) and CORT (F1,27 = 5.31, p = 0.03) concentrations. Post hoc analyses, collapsed across experimental days, indicated that noise stress significantly increased ACTH concentrations in males (p = 0.001) but not females (p = 0.20), and that significantly higher CORT concentrations were found in females compared to males only in the control (p < 0.001) but not stressed (p = 0.15) groups. There were no significant interactions between sex and time on either ACTH or CORT concentrations (three-way interactions also n.s.).
Physiological changes to repeated loud noise
and collectively display the effects of sex and stress condition on physiological parameters measured across the repeated noise experiment. All animals significantly gained weight over the course of the repeated noise experiment (F4,72 = 277.57, p < 0.001; main effect of time; ). In addition, males weighed significantly more than females (F1,43 = 631.13, p < 0.001; main effect of sex), and gained weight significantly faster over time than females (F4,172 = 131.53, p < 0.001; significant two-way interaction). There was no significant interaction between sex and stress condition on body weight (the three-way interaction was also n.s.). Stress condition significantly affected body weight (F2,43 = 3.97, p < 0.05; main effect of stress condition), and also affected the rate of body weight gain over time (F8,172 = 4.77, p < 0.001; significant two-way interaction). Post hoc analyses of average body weight collapsed across sex revealed that Naïve animals weighed significantly more than No Stress controls (p = 0.006), but not Stressed animals (p = 0.09). Stressed animals also did not differ significantly in weight from No Stress control animals (p = 0.52). The effect of stress condition on body weight gain over time was then explored further. At arrival, animals in each stress condition did not differ significantly in body weight (F2,49 = 0.13, p = 0.88). However, stress condition significantly affected the change in body weight from arrival to day 10 (F2,48 = 11.3, p < 0.001; ), and post hoc analyses revealed that Naïve animals gained significantly more weight from arrival to day 10 than Stressed and No Stress control animals (p’s < 0.002). Stressed animals and No Stress control animals gained a similar amount of weight (p = 0.83).
Figure 2. Body weight gain (means ± 1 SEM) in male and female rats over 10 days of daily repeated noise stress exposure. Naïve rats of both sexes weighed significantly more, and gained weight significantly faster, than both No Stress and Stressed groups, which did not differ from each other. *Males weighed significantly more, and gained weight faster, than females (p < 0.05).
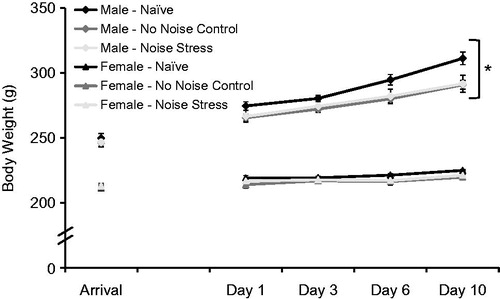
Table 1. Physiological parameters across repeated noise experiment.
also displays changes in absolute (raw) and ratio of body weight to both adrenal and thymus weight (expressed as % body weight) across the repeated noise experiment. Females had significantly higher absolute (F1,48 = 73.31, p < 0.001), and ratio of body weight to (F1,48 = 297.73, p < 0.001), adrenal weight and significantly lower absolute (F1,48 = 124.49, p < 0.001), and ratio of body weight to (F1,48 = 30.54, p < 0.001), thymus weight compared to males. There was no significant effect of stress condition on thymus weight measures (p’s > 0.05), and these effects did not vary based on sex (p’s > 0.05). Stress condition affected both absolute (F2,48 = 4.28, p = 0.03) adrenal weight and ratio of adrenal weight to body weight (F2,48 = 6.63, p = 0.003), and post hoc analyses revealed that stressed animals had significantly larger adrenals than No Stress controls (p = 0.03), but not Naïve animals (p = 0.09), and these control groups did not differ significantly (p = 0.85). The effect of sex on absolute (F2,48 = 4.78, p = 0.01) and ratio of body weight to (F2,48 = 4.17, p = 0.02) adrenal weight also varied significantly based on stress condition. Within females only, stress condition significantly affected both absolute adrenal weight (F2,24 = 7.87, p = 0.003) and ratio of adrenal to body weight (F2,24 = 8.8, p = 0.002), and post hoc analyses revealed the same trend for both measures; Stressed female adrenals were significantly larger than both Naïve and No Stress female adrenals (p’s < 0.05), but Naïve and No Stress female adrenals were similar in size (p’s > 0.05). In contrast, the male absolute adrenal size (F2,23 = 0.76, p = 0.48) and the male ratio of adrenal to body weight (F2,23 = 0.19, p = 0.83) did not differ across stress conditions.
HPA axis responses to repeated restraint
Restraint stress significantly increased both ACTH (F1,28 = 19.41, p < 0.001; ) and CORT (F1,28 = 42.35, p < 0.001; ) concentrations (main effect of stress condition). In addition, both ACTH (F3,84 = 31.18, p < 0.001) and CORT (F3,84 = 27.18, p < 0.001) concentrations decreased significantly over the experiment (main effect of experimental day). There was no main effect of sex on ACTH concentrations (F1,28 = 3.88, p = 0.06), however females had significantly higher CORT concentrations compared to males (F1,28 = 23.90, p < 0.001).
Figure 3. Mean (±1 SEM) ACTH (A) and CORT (B) concentrations over 10 days of daily restraint stress (30 min/day) in male and female rats. Significant main effects of stress condition, time, and a two-way interaction between stress condition and time were observed on concentrations of both hormones. A significant main effect of sex on CORT, but not ACTH, concentrations was also observed. *Stressed animals significantly higher than control animals, regardless of sex.
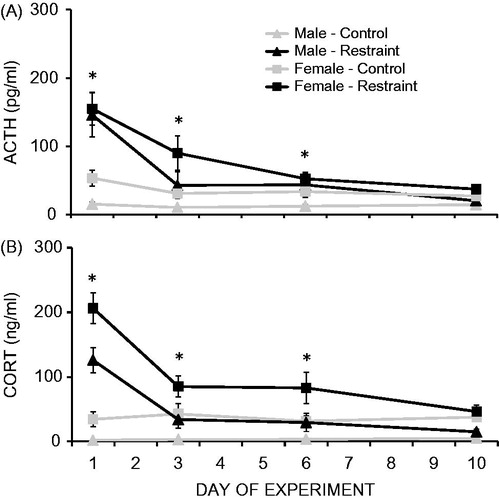
There was a significant two-way interaction between stress condition and time on both ACTH (F3,84 = 19.69, p < 0.001) and CORT (F3,84 = 29.48, p < 0.001) concentrations, and post hoc analyses collapsed across sex revealed that both ACTH and CORT concentrations were significantly elevated in stressed compared to control animals on days 1, 3 and 6 (p’s < 0.05), but did not differ from controls by day 10 (p > 0.05). There were no significant two-way interactions between sex and time or between sex and stress condition on either ACTH or CORT concentrations (three-way interaction also n.s.).
Physiological changes to repeated restraint
and collectively display the effects of sex and stress condition on physiological parameters measured in the repeated restraint experiment. For the repeated restraint experiment, body weights of naïve animals were only recorded at arrival and on day 10 (). Therefore, Naïve animal body weights were only analyzed on the day of arrival, and as a function of change over the course of the experiment from arrival to day 10. Comparing just Stress and No Stress animals across the repeated restraint experiment, all animals gained weight (F4,112 = 544.33, p < 0.001; ). Males not only weighed significantly more than females (F1,28 = 336.68, p < 0.001), they also gained weight significantly faster than females over the experiment (F4,112 = 182.04, p < 0.001). In addition, the effect of sex on body weight did not differ based on stress condition (F1,28 = 0.24, p = 0.63). Stress and No Stress control animals of both sexes had similar body weight (F1,28 = 1.76, p = 0.19), and also gained weight at a similar rate (F4,112 = 1.5, p = 0.21). There was not a significant three-way interaction. Comparing Naïve, No stress and Stress animals at arrival, there was no difference in body weight across stress condition (F2,44 = 0.39, p = 0.68). Again, males weighed significantly more than females at arrival (F1,44 = 129.75, p < 0.001), and this did not depend on stress condition (F2,44 = 0.75, p = 0.48). Although there was also no effect of stress condition on the change in body weight across the repeated restraint experiment (F2,44 = 2.64, p = 0.08; ), the effect of stress condition on body weight change did depend on sex (F2,44 = 4.62, p = 0.015). Further analysis revealed that stress condition significantly affected body weight change from arrival to day 10 in males (F2,24 = 4.17, p = 0.03) but not in females (F2,24 = 2.5, p = 0.11). Post hoc analyses in males showed that Naïve males gained significantly more weight over the experiment than Stressed males (p = 0.04), but not No Stress control males (p = 0.07). No Stress and Stressed males gained a similar amount of weight (p = 0.98).
Figure 4. Body weight gain (means ± 1 SEM) in male and female rats over the course of 10 days of daily exposure to restraint stress. Naïve males gained significantly more weight over the experiment than stressed males (p = 0.04), but not no stress control males (p = 0.07). No stress and stressed males gained a similar amount of weight. *Males weighed significantly more, and gained weight faster, than females (p < 0.05).
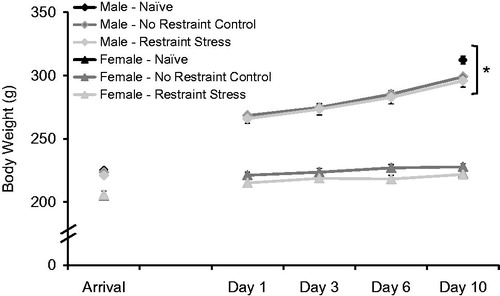
Table 2. Physiological parameters after repeated restraint experiment.
also displays changes in absolute (raw) and ratio of body weight to (percent), both adrenal and thymus weight across the repeated noise experiment. Females in the repeated restraint experiment also had significantly larger absolute (F1,49 = 114.75, p < 0.001), and ratio of body weight to adrenal weight (F1,49 = 509.79, p < 0.001), and significantly smaller absolute (F1,49 = 262.99, p < 0.001) and ratio of body weight to thymus weight (F1,49 = 71.56, p < 0.001) compared to males. There was no significant main effect of stress condition on thymus weight measures (p’s > 0.05), and these effects did not vary based on sex (p’s > 0.05). Stress condition however, affected both absolute (F2,49 = 9.05, p = 0.001) adrenal weight and ratio of adrenal weight to body weight (F2,49 = 6.72, p = 0.003), and post hoc analyses revealed that Naive animals had significantly larger adrenals than both No Stress controls (p = 0.002) and Stressed animals (p = 0.003). No Stress control adrenal weight did not differ significantly from Stressed animal adrenal weight (p = 0.98). The effect of sex on absolute and ratio of body weight to adrenal weight did not vary significantly based on stress condition (p’s > 0.05).
HPA axis responses to a novel environment following repeated stress
displays the effects of sex, novel environment exposure, and 10 days of prior stress on HPA axis responses to a novel environment exposure. For both experiments, data were analyzed in two ways. To investigate the effect of novel environment exposure alone, data were collapsed across prior stress history and compared to Naïve HPA axis hormone concentrations. Then, data from No Stress controls of both sexes were compared to data from Stressed animals of both sexes to investigate the effect of prior stress of HPA axis responses to novel environment. Following repeated noise, animals of both sexes that received novel environment exposure (collapsing across prior stress history) had significantly higher ACTH (F1,48 = 15.08, p < 0.001) and CORT (F1,48 = 20.60, p < 0.001) concentrations than Naïve animals of both sexes. However, there was no main effect of sex on either ACTH (F1,48 = 2.50, p = 0.12) or CORT (F1,48 = 1.31, p = 0.26) concentrations, and this did not vary based on novel environment exposure (p’s > 0.05). Comparing just animals that received novel environment (excluding Naïve animals), animals of both sexes with no prior stress exposure had similar ACTH (F1,30 = 0.68, p = 0.42) and CORT (F1,30 = 0.16, p = 0.70) concentrations in response to novel environment compared to animals of both sexes that did have prior stress exposure (), and this did not vary within each sex (p’s > 0.05). In addition, females had similar ACTH (F1,30 = 0.63, p = 0.43), and CORT (F1,30 = 0.28, p = 0.60) concentrations compared to males in response to novel environment exposure. However, further direct comparison of naïve animals only revealed that females had significantly higher basal ACTH (t16 = 3.19, p = 0.006) and CORT (t16 = 2.80, p = 0.01) concentrations compared to males.
Figure 5. Mean ACTH (A and B) and CORT (C and D) concentrations (±1 SEM) in response to a novel environment in animals exposed to prior repeated noise (A and C) or restraint (B and D). Novel environment exposure (NE) significantly increased ACTH and CORT concentrations in all animals in both experiments (#p’s < 0.05 compared to naïve animals). Prior stress (noise or restraint) did not affect HPA axis responses to NE (p’s > 0.05). *p < 0.05 compared to male rats in the same stress condition.
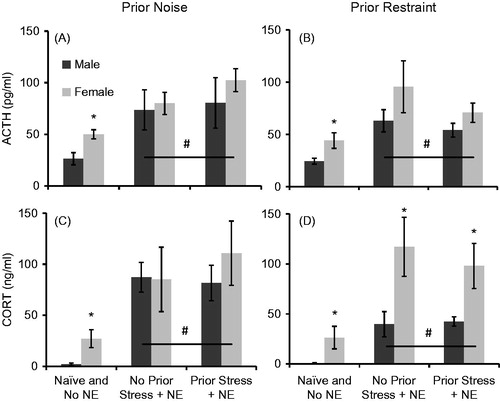
Within the repeated restraint experiment, animals of both sexes that received novel environment exposure (collapsing across prior restraint stress history) had significantly higher ACTH (F1,49 = 12.83, p = 0.001) and CORT (F1,49 = 19.86, p < 0.001) concentrations than Naïve animals of both sexes. Unlike in the repeated noise experiment, females had significantly higher ACTH (F1,49 = 4.72, p = 0.04) and CORT (F1,49 = 11.41, p = 0.001) concentrations, but this effect of sex did not vary based on novel environment exposure (p’s > 0.05). Comparing just animals that received novel environment (excluding Naïve animals), animals of both sexes without prior restraint stress exposure had similar ACTH (F1,31 = 1.33, p = 0.26) and CORT (F1,31 = 0.17, p = 0.68) concentrations in response to novel environment compared to animals of both sexes that did have prior stress exposure (), and this did not vary within each sex (p’s > 0.05). However, females had similar ACTH (F1,31 = 2.84, p = 0.10), but significantly higher CORT (F1,31 = 11.31, p = 0.002) concentrations compared to males in response to novel environment exposure. Further direct comparison of naïve animals revealed that females also had significantly higher basal ACTH (t16 = 2.47, p = 0.025) and CORT (t16 = 2.26, p = 0.04) concentrations compared to males.
Discussion
The experiments presented here investigate the effect of sex on the extent of HPA axis hormone habituation to repeated homotypic stress exposure. We previously demonstrated that female rats exhibit robustly higher HPA axis hormone concentrations in response to 30 min of acute restraint stress (Babb et al., Citation2013a), but the same effect of sex is not present in response to 30 min of acute noise stress (Babb et al., Citation2013b). Here, we replicated our previous results, demonstrating that the female HPA axis appears to be more responsive than the male HPA axis to acute restraint stress, but not acute noise stress. The results from this study suggest that males may be more sensitive to noise stress than females, since stressed compared to control males had larger increases in HPA axis responses following noise than in stressed compared to control females. We have previously attempted to explain the differential effect of sex on HPA axis responses to acute presentations of these stressors (Babb et al., Citation2013b), and have ruled out several possibilities including differences in social context between the two stressor presentations, and in pain or auditory processing between sexes as the underlying causes for this effect. These data suggest that, regardless of the acute effect of sex on HPA axis responses to a stressor, male and female rats exhibit a similar extent of stress-induced habituation of circulating ACTH or CORT concentrations in response to repeated presentations of stress.
Our hypothesis that females exposed to repeated restraint stress would exhibit slower or less habituation compared to males exposed to the same repeated stressor was not supported by our data. By and large, the rates and extents of ACTH and CORT habituation observed in male and female rates were comparable, at least at the 30-min time point that we chose to assess. We chose a 30-min time point due to the observation of peak CORT, and near peak ACTH concentrations in response to loud noise stress at this time point in males, and due to our previous research using this time point to study HPA axis habituation in males (Campeau & Watson, Citation1997; Campeau et al., Citation2002; Day et al., Citation2009; Masini et al., Citation2008, Citation2012). It is possible that if we had included earlier or later time points in our analysis, a larger effect of sex may have been observed, and therefore this is a major limitation of this study. For example, Bhatnagar et al. (Citation2005) observed habituated CORT responses in adult female rats at the 15-min time point on the 8th day of 30 min of restraint stress, but evidence of habituation was gone by the 30-min time point, and the effect was even reversed at the 60-min time point. In contrast, Doremus-Fitzwater et al. (Citation2009) found evidence of habituated CORT responses in adult females on the 5th exposure to 90 min of restraint stress, but only at the 60 and 120 min time points, and no difference in CORT responses at the 15 and 30 min time points. But unlike these prior studies, we observed robust evidence of habituated HPA axis responses in females at a 30-min time point by the 3rd day of repeated stress, and these habituated responses were similar to male habituated responses at the same time point. It is also possible that even with similarly habituated HPA axis responses to the same repeated stressor, the male and female rats in this study may have displayed robustly different behavioral or neural adaptations to repeated stress as previous research has indicated (Bowman et al., Citation2003; Khurana & Devaud, Citation2007; Lunga & Herbert, Citation2004; McCormick et al., Citation2007). Finally, although our group sizes did not allow for analysis of female responses on specific days of the estrous cycle, female HPA axis hormone concentrations in general had similar variability compared to male HPA axis hormone concentrations, and this suggests that circulating female sex steroids did not mask the potential of sex differences being observed.
Previous studies performed in male rats have demonstrated either normal or facilitated HPA axis responses to a novel stressor after repeated exposures to stress compared to rats without any prior stress exposure (Akana & Dallman, Citation1997; Bhatnagar & Dallman, Citation1998; Bhatnagar et al., Citation1995, Citation2003; Grissom et al., Citation2008; Martí & Armario, Citation1998). We have previously used the same novel environment used in this study to investigate the effect of voluntary exercise on HPA axis responses to low intensity stressors (Campeau et al., Citation2010; Masini et al., Citation2011), and have also observed facilitated HPA axis responses in male rats to the same novel environment 24 h after 3 days of 10, 1 s long, 1 mA footshocks each day (Day and Campeau, unpublished observations). In the present studies, no facilitated HPA axis responses to novel environment presentation were observed in animals of either sex previously exposed to either noise or restraint stress compared to control animals that received the same handling and blood sampling procedure but no prior stress. That is, previously habituated female rats did not exhibit greater novel stress-induced HPA axis hormone responses compared to female rats that had not been previously habituated to either stressor. However, male rats with prior stress history also did not display facilitated HPA axis responses to the novel environment as we had previously observed following footshock stress in males. This could be due to the greater intensity of footshock stress compared to either loud noise or restraint stress, i.e. perhaps neither noise nor restraint are severe enough stressors to observe facilitation of HPA axis hormones to a subsequent heterotypic stressor. Likewise, it is possible that facilitated HPA axis responses were not observed because the novel environment itself does not robustly increase HPA axis hormone concentrations; perhaps a more severe stressor is needed for this effect. However, all animals that were exposed to the novel environment did display significantly higher HPA axis hormone concentrations than naïve control animals, indicating that this stressor did significantly activate the HPA axis, however not to the same magnitude as acute presentations of either noise () or restraint (). Female rats displayed higher CORT, although similar ACTH, concentrations to novel environment exposure compared to male rats, regardless of prior stress history in the restraint experiment. This unexpected main effect of sex may reflect sex differences in basal HPA axis hormone concentrations rather than stress-induced levels, since the same effect of sex on CORT concentrations in the repeated noise stress experiment was not observed. It is unlikely that this effect was observed due to restraint inherently being a more intense stressor than noise, since acute HPA axis hormone concentrations were nominally higher in response to noise compared to restraint stress, and because the effect of sex on novel environment responses was present in the restraint experiment regardless of prior stress history.
Although both male and female naïve animals displayed faster body weight gain than the other stress conditions in general for both experiments, No Stress and Stress groups within each sex for each experiment gained weight at a similar rate, suggesting that both of these groups were affected by experimental procedures (see and ). Others have described how handling alone can affect HPA axis activity in rats (Dobrakovova et al., Citation1993), and our results support the idea that handling alone in the No Stress control groups of both sexes affected body weight gain. However, in addition to being handled daily, rats in the No Stress control group were also singly housed, and multiple blood samples were also taken from these animals, which likely contributed to the attenuated body weight gain observed in these animals compared to Naïve control animals. In addition, adrenal hypertrophy and thymic involution, two physiological hallmarks of severe stress experience, were not observed in animals exposed to repeated restraint stress, suggesting that this stressor did not by itself produced large physiological changes, and that habituation of HPA axis hormones in response to repeated stress was adaptive in both sexes. Females exposed to repeated noise stress did have larger adrenals compared to both female control groups, but stressed females have similarly sized thymi than other female groups. In addition, the same effect was not observed in male rats exposed to repeated noise even though males displayed significantly higher HPA axis hormone responses to noise stress acutely. The most robust effect on adrenal and thymus weight was in fact the main effect of sex. Female rats, regardless of stress condition, had larger raw weight of adrenals and smaller raw weight of thymi than male rats, and the effect on adrenals was made even larger when corrected for body weight, since females weighed substantially less than males. These effects of sex on organ size and morphology have been known for many decades (Leposavic et al., Citation1996; Majchrzak & Malendowicz, Citation1983; Sencar-Cupovic & Milkovic, Citation1976), and so are not unexpected, and along with sex differences in basal hormone concentrations in the absence of any stress exposure highlight the inherent differences in HPA axis function between male and female rats.
Conclusions
Despite differential effects of sex on acute HPA axis hormone activation to stress, male and female rats displayed a similar extent of habituation of these hormones following repeated presentations of the same stressor. Furthermore, previously stressed rats of either sex did not display facilitated HPA axis hormone concentrations to a novel environment compared to animals of either sex without a prior history of stress, although a main effect of sex may exist to this mild stressor. These experiments suggest that HPA axis hormone habituation can in fact occur in the female rodent as quickly as in the male rodent, and importantly, that the female HPA axis was not more sensitive to the experience of a subsequent stressful stimulus than the male HPA axis, at least within the parameters of this study.
Declaration of interest
The authors report no conflicts of interest. The authors alone are responsible for the content and writing of the article. This work was supported by National Institute of Mental Health Grant R01MH077152 awarded to S.C.
Acknowledgements
The authors would like to thank Dr. Robert Spencer (University of Colorado at Boulder, Department of Psychology and Neuroscience) for generously providing the restraint tubes used in this experiment and for his invaluable support of this work. The authors would also like to thank Laura Hinds (University of Colorado at Boulder, Department of Psychology and Neuroscience) with technological assistance.
References
- Akana SF, Dallman MF. (1997). Chronic cold in adrenalectomized, corticosterone (B)-treated rats: facilitated corticotropin responses to acute restraint emerge as B increases. Endocrinology 138:3249–58
- Aloisi AM, Ceccarelli I, Lupo C. (1998). Behavioural and hormonal effects of restraint stress and formalin test in male and female rats. Brain Res Bull 47:1–6
- Aloisi AM, Steenbergen HL, van de Poll NE, Farabollini F. (1994). Sex-dependent effects of restraint on nociception and pituitary-adrenal hormones in the rat. Physiol Behav 55:1–5
- Altemus M, Redwine L, Leong YM, Yoshikawa T, Yehuda R, Detera-Wadleigh S, Murphy DL. (1997). Reduced sensitivity to glucocorticoid feedback and reduced glucocorticoid receptor mRNA expression in the luteal phase of the menstrual cycle. Neuropsychopharmacology 17:100–9
- Altemus M, Roca C, Galliven E, Romanos C, Deuster P. (2001). Increased vasopressin and adrenocorticotropin responses to stress in the midluteal phase of the menstrual cycle. JCEM 86:2525–30
- Andrés R, Martí O, Armario A. (1999). Direct evidence of acute stress-induced facilitation of ACTH response to subsequent stress in rats. Am J Physiol 277:R863–8
- Armario A, Castellanos JM, Balasch J. (1984). Adaptation of anterior pituitary hormones to chronic noise stress in male rats. Behav Biol 41:71–6
- Babb JA, Masini CV, Day HE, Campeau S. (2013a). Sex differences in activated corticotropin-releasing factor neurons within stress-related neurocircuitry and hypothalamic-pituitary-adrenocortical axis hormones following restraint in rats. Neuroscience 234C:40–52
- Babb JA, Masini CV, Day HEW, Campeau S. (2013b). Stressor-specific effects of sex on HPA axis hormones and activation of stress-related neurocircuitry. Stress 16(6):664–77
- Bekker MH, van Mens-Verhulst J. (2007). Anxiety disorders: sex differences in prevalence, degree, and background, but gender-neutral treatment. Gend Med 4(Suppl B):S178–93
- Bhatnagar S, Dallman M. (1998). Neuroanatomical basis for facilitation of hypothalamic-pituitary-adrenal responses to a novel stressor after chronic stress. Neuroscience 84:1025–39
- Bhatnagar S, Huber R, Lazar E, Pych L, Vining C. (2003). Chronic stress alters behavior in the conditioned defensive burying test: role of the posterior paraventricular thalamus. Pharmacol Biochem Behav 76:343–9
- Bhatnagar S, Huber R, Nowak N, Trotter P. (2002). Lesions of the posterior paraventricular thalamus block habituation of the hypothalamic-pituitary-adrenal responses to repeated restraint. J Neuroendocrinol 14:403–10
- Bhatnagar S, Lee TM, Vining C. (2005). Prenatal stress differentially affects habituation of corticosterone responses to repeated stress in adult male and female rats. Horm Behav 47:430–8
- Bhatnagar S, Mitchell JB, Betito K, Boksa P, Meaney MJ. (1995). Effects of chronic intermittent cold stress on pituitary adrenocortical and sympathetic adrenomedullary functioning. Physiol Behav 57:633–9
- Bowman RE, Beck KD, Luine VN. (2003). Chronic stress effects on memory: sex differences in performance and monoaminergic activity. Horm Behav 43:48–59
- Campeau S, Dolan D, Akil H, Watson SJ. (2002). c-fos mRNA induction in acute and chronic audiogenic stress: possible role of the orbitofrontal cortex in habituation. Stress 5:121–30
- Campeau S, Nyhuis TJ, Sasse SK, Kryskow EM, Herlihy L, Masini CV, Babb JA, et al. (2010). Hypothalamic pituitary adrenal axis responses to low-intensity stressors are reduced after voluntary wheel running in rats. J Neuroendocrinol 22:1–17
- Campeau S, Watson SJ. (1997). Neuroendocrine and behavioral responses and brain pattern of c-fos induction associated with audiogenic stress. J Neuroendocrinol 9:577–88
- Christiansen DM, Elklit A. (2008). Risk factors predict post-traumatic stress disorder differently in men and women. Ann Gen Psychiatry 7:1–12
- Chrousos GP, Gold PW. (1998). A healthy body in a healthy mind – and vice versa – the damaging power of “uncontrollable” stress. JCEM 83:1842–5
- Claes SJ. (2004). Corticotropin-releasing hormone (CRH) in psychiatry: from stress to psychopathology. Ann Med 36:50–61
- Day HE, Masini CV, Campeau S. (2009). Reversible inactivation of the auditory thalamus disrupts HPA axis habituation to repeated loud noise stress exposures. Brain Res 1276:123–30
- Ditlevsen DN, Elklit A. (2010). The combined effect of gender and age on post traumatic stress disorder: do men and women show differences in the lifespan distribution of the disorder? Ann Gen Psychiatry 9:32–44
- Dobrakovova M, Kvetnansky R, Oprasalova Z, Jezova D. (1993). Specificity of the effect of repeated handling on sympathetic-adrenomedullary and pituitary-adrenocortical activity in rats. Psychoneuroendocrinology 18:63–74
- Doremus-Fitzwater TL, Varlinskaya EI, Spear LP. (2009). Social and non-social anxiety in adolescent and adult rats after repeated restraint. Physiol Behav 97:484–94
- Drossopoulou G, Antoniou K, Kitraki E, Papathanasiou G, Papalexi E, Dalla C, Papadopoulou-Daifoti Z. (2004). Sex differences in behavioral, neurochemical and neuroendocrine effects induced by the forced swim test in rats. Neuroscience 26:849–57
- Ehlert U, Gaab J, Heinrichs M. (2001). Psychoneuroendocrinological contributions to the etiology of depression, posttraumatic stress disorder, and stress-related bodily disorders: the role of the hypothalamus-pituitary-adrenal axis. Biol Psychol 57:141–52
- Galea LA, McEwen BS, Tanapat P, Deak T, Spencer RL, Dhabhar FS. (1997). Sex differences in dendritic atrophy of CA3 pyramidal neurons in response to chronic restraint stress. Neuroscience 81:689–97
- Girotti M, Pace TWW, Gaylord RI, Rubin BA, Herman JP, Spencer RL. (2006). Habituation to repeated restraint stress is associated with lack of stress-induced c-fos expression in sensory processing areas of the rat brain. Neuroscience 138:1067–81
- Grissom N, Bhatnagar S. (2009). Habituation to repeated stress: get used to it. Neurobiol Learn Mem 92:215–24
- Grissom N, Iyer V, Vining C, Bhatnagar S. (2007). The physical context of previous stress exposure modifies hypothalamic-pituitary-adrenal responses to a subsequent homotypic stress. Horm Behav 51:95–103
- Grissom N, Kerr W, Bhatnagar S. (2008). Struggling behavior during restraint is regulated by stress experience. Behav Brain Res 191:219–26
- Haleem DJ, Kennett G, Curzon G. (1988). Adaptation of female rats to stress: shift to male pattern by inhibition of corticosterone synthesis. Brain Res 458:339–47
- Handa RJ, Burgess LH, Kerr JE, O’Keefe JA. (1994). Gonadal steroid hormone receptors and sex differences in the hypothalamo-pituitary-adrenal axis. Horm Behav 28:464–76
- Heinsbroek RP, van Haaren F, Feenstra MG, Boon P, van de Poll NE. (1991). Controllable and uncontrollable footshock and monoaminergic activity in the frontal cortex of male and female rats. Brain Res 551:247–55
- Holbrook TL, Hoyt DB, Stein MB, Sieber WJ. (2002). Gender differences in long-term posttraumatic stress disorder outcomes after major trauma: women are at higher risk of adverse outcomes than men. J Trauma 53:882–8
- Jones T, Moller MD. (2011). Implications of hypothalamic-pituitary-adrenal axis functioning in posttraumatic stress disorder. J Am Psychiatr Nurses Assoc 17:393–403
- Kessler RC, Berglund P, Demler O, Jin R, Merikangas KR, Walters EE. (2005). Lifetime prevalence and age-of-onset distributions of DSM-IV disorders in the National Comorbidity Survey Replication. Arch Gen Psychiatry 62:593–602
- Khurana RC, Devaud LL. (2007). Sex differences in neurotransmission parameters in response to repeated mild restraint stress exposures in intact male, female, and ovariectomised female rats. J Neuroendocrinol 19:511–20
- Kirschbaum C, Kudielka BM, Gaab J, Schommer NC, Hellhammer DH. (1999). Impact of gender, menstrual cycle phase, and oral contraceptives on the activity of the hypothalamus-pituitary-adrenal axis. Psychosomatic Med 61:154–62
- Larkin JW, Binks SL, Li Y, Selvage D. (2010). The role of oestradiol in sexually dimorphic hypothalamic-pituitary-adrenal axis responses to intracerebroventricular ethanol administration in the rat. J Neuroendocrinol 22:24–32
- Le Mevel JC, Abitbol S, Beraud G, Maniey J. (1978). Dynamic changes in plasma adrenocorticotrophin after neurotropic stress in male and female rats. J Endocrinol 76:359–60
- Leposavic G, Karapetrovic B, Obradovic S, Dankovic BV, Kosec D. (1996). Differential effects of gonadectomy on thymocyte phenotypic profile in male and female rats. Pharmacol Biochem Behav 54:269–76
- Linzer M, Spitzer R, Kroenke K, Williams JB, Hahn S, Brody D, deGruy F. (1996). Gender, quality of life, and mental disorders in primary care: results from the PRIME-MD 1000 study. Am J Med 101:526–33
- Livezey GT, Miller JM, Vogel WH. (1985). Plasma norepinephrine, epinephrine and corticosterone stress responses to restraint in individual male and female rats, and their correlations. Neurosci Lett 62:51–6
- Lunga P, Herbert J. (2004). 17Beta-oestradiol modulates glucocorticoid, neural, and behavioral adaptations to repeated restraint stress in female rats. J Neuroendocrinol 16:776–85
- Majchrzak M, Malendowicz LK. (1983). Sex differences in adrenocortical structure and function. XII. Stereologic studies of rat adrenal cortex in the course of maturation. Cell Tissue Res 232:457–69
- Martí O, Armario A. (1997). Influence of regularity of exposure to chronic stress on the pattern of habituation of pituitary-adrenal hormones, prolactin and glucose. Stress 1:179–89
- Martí O, Armario A. (1998). Anterior pituitary response to stress: time-related changes and adaptation. Int J Dev Neurosci 6:241–60
- Martí O, Gavaldà A, Gómez F, Armario A. (1994). Direct evidence for chronic stress-induced facilitation of the adrenocorticotropin response to a novel acute stressor. Neuroendocrinology 60:1–7
- Masini CV, Babb JA, Nyhuis TJ, Day HE, Campeau S. (2012). Auditory cortex lesions do not disrupt habituation of HPA axis responses to repeated noise stress. Brain Res 1443:1–9
- Masini CV, Day HE, Campeau S. (2008). Long-term habituation to repeated loud noise is impaired by relatively short interstressor intervals in rats. Behav Neurosci 22:210–23
- Masini CV, Nyhuis TJ, Sasse SK, Day HE, Campeau S. (2011). Effects of voluntary wheel running on heart rate, body temperature, and locomotor activity in response to acute and repeated stressor exposures in rats. Stress 14:324–34
- McCormick CM, Merrick A, Secen J, Helmreich DL. (2007). Social instability in adolescence alters the central and peripheral hypothalamic-pituitary-adrenal responses to a repeated homotypic stressor in male and female rats. J Neuroendocrinol 19:116–26
- McEwen BS. (2004). Protection and damage from acute and chronic stress: allostasis and allostatic overload and relevance to the pathophysiology of psychiatric disorders. Ann NY Acad Sci 1032:1–7
- Natelson BH, Ottenweller JE, Cook JA, Pitman D, McCarty R, Tapp WN. (1988). Effect of stressor intensity on habituation of the adrenocortical stress response. Physiol Behav 43:41–6
- Nyhuis TJ, Masini CV, Sasse SK, Day HE, Campeau S. (2010a). Physical activity, but not environmental complexity, facilitates HPA axis response habituation to repeated audiogenic stress despite neurotrophin mRNA regulation in both conditions. Brain Res 1362:68–77
- Nyhuis TJ, Sasse SK, Masini CV, Day HEW, Campeau S. (2010b). Lack of contextual modulation of habituated neuroendocrine responses to repeated audiogenic stress. Behav Neurosci 124:810–20
- Ogilvie KM, Rivier C. (1997). Gender difference in hypothalamic-pituitary-adrenal axis response to alcohol in the rat: activational role of gonadal steroids. Brain Res 766:19–28
- Olff M, Langeland W, Draijer N, Gersons BP. (2007). Gender differences in posttraumatic stress disorder. Psychol Bull 133:83–204
- Pace TW, Cole MA, Ward G, Kalman BA, Spencer RL. (2001). Acute exposure to a novel stressor further reduces the habituated corticosterone response to restraint in rats. Stress 4:319–31
- Reagan LP, Grillo CA, Piroli GG. (2008). The As and Ds of stress: metabolic, morphological and behavioral consequences. Eur J Pharmacol 585:64–75
- Sasse SK, Greenwood BN, Masini CV, Nyhuis TJ, Fleshner M, Day HE, Campeau S. (2008). Chronic voluntary wheel running facilitates corticosterone response habituation to repeated audiogenic stress exposure in male rats. Stress 11:425–37
- Seale JV, Wood SA, Atkinson HC, Bate E, Lightman SL, Ingram CD, Jessop DS, Harbuz MS. (2004). Gonadectomy reverses the sexually diergic patterns of circadian and stress-induced hypothalamic-pituitary-adrenal axis activity in male and female rats. J Neuroendocrinol 16:1–9
- Sencar-Cupovic I, Milkovic S. (1976). The development of sex differences in the adrenal morphology and responsiveness in stress of rats from birth to the end of life. Mechs Ageing Dev 5:1–9
- Stein MB, Walker JR, Forde DR. (2000). Gender differences in susceptibility to posttraumatic stress disorder. Behav Res Ther 38:619–28
- Tolin DF, Foa EB. (2006). Sex differences in trauma and posttraumatic stress disorder: a quantitative review of 25 years of research. Psychol Bull 132:959–92
- Van de Velde D, Bracke P, Levecque K. (2010). Gender differences in depression in 23 European countries. Cross-national variation in the gender gap in depression. Social Sci Med 71:305–13
- Vanltallie T. (2002). Stress: a risk factor for serious illness. Metabolism 51:40–5
- Vesga-López O, Schneier FR, Wang S, Heimberg RG, Liu S, Hasin DS, Blanco C. (2008). Gender differences in generalized anxiety disorder: results from the National Epidemiologic Survey on Alcohol and Related Conditions (NESARC). J Clin Psychiatry 69:1606–16
- Viau V, Bingham B, Davis J, Lee P, Wong M. (2005). Gender and puberty interact on the stress-induced activation of parvocellular neurosecretory neurons and corticotropin-releasing hormone messenger ribonucleic acid expression in the rat. Endocrinology 146:137–46
- Weinberg MS, Johnson DC, Bhatt AP, Spencer RL. (2010). Medial prefrontal cortex activity can disrupt the expression of stress response habituation. Neuroscience 168:744–56
- Weinstock M, Razin M, Schorer-Apelbaum D, Men D, McCarty R. (1998). Gender differences in sympathoadrenal activity in rats at rest and in response to footshock stress. Int J Dev Neurosci 16:289–95