Abstract
Women are more likely than men to develop psychopathology as a result of stress, but there is little research regarding the effects of a stressful condition and its treatment in female non-human animals, perhaps because of inherent hormonal activity. Recent studies have demonstrated that there are structural and functional differences between the dorsal and ventral hippocampus, but the effects of stress on the morphology of CA1 and CA3 neurons have been studied primarily in the dorsal hippocampus. This study assessed the effects of stress induced by restricted movement on the morphology of ventral hippocampal CA1 neurons in male and female rats. Male and female Long Evans (LE) rats were subjected to restraint stress for 6 h every day for 25 days. One group of rats was used to study the dendritic morphology of CA1 ventral hippocampal neurons using the Golgi–Cox stain. A second group of rats was used to analyze learning and memory using the Morris water maze. Stressed female rats exhibited a decrease in the density of basilar dendritic spines, an increase in the number of apical dendritic intersections and deficits in spatial memory. There were no apparent effects of stress on male rats. Our data support previous findings of a dimorphic response to chronic stress and indicate that the ventral hippocampus is not particularly susceptible to the effects of stress.
Introduction
Stress in biological systems is defined as any condition that severely disrupts the physiological and psychological homeostasis of an organism (Kim & Diamond, Citation2002; Selye, Citation1956). Various types of stressors can be categorized according to their psychogenic factors related psychological or neurological alterations. It is well known that a movement restraint paradigm, which does not involve physical pain, induces stress and results in behavioral, neurochemical and morphological changes (for reviews, refer Bowman et al., Citation2003). In male rodents, chronic restraint induces neuronal loss and morphological remodeling in the dorsal hippocampus (Akirav & Levin, Citation2002; Argumedo et al., Citation2007; Christian et al., Citation2011; Eiland et al., Citation2012; Hosseini-sharifabad & Hadinedoushan, Citation2007), as well as dendritic remodeling of neurons in the prefrontal cortex and amygdala (Cook & Wellman, Citation2004; Vyas et al., Citation2002). Chronic restraint of male rats also causes atrophy of dendritic branches and dendritic spine density and changes in the synaptic communications between these regions (Krugers et al., Citation2006; Mitra et al., Citation2005). Chronic restraint stress in female rats also produces moderate atrophy of hippocampal neurons (Galea et al., Citation1997).
Stress also causes dendritic retraction of CA3 hippocampal neurons, which is accompanied by memory deficits (Bowman et al., Citation2003; Conrad et al., Citation1996). It is possible that the stress-related alterations that are observed in CA3 neurons may affect the morphology and physiology of other neuronal types (Galea et al., Citation1997), such as CA1 neurons.
The primary stress-induced physiological changes are observed in the brain when the activation of the hypothalamic–pituitary–adrenal axis and resulting corticosterone (CORT) release by the adrenal glands are present (Bowman et al., Citation2001). Interestingly, a recent report (Morales-Medina et al., Citation2009) suggests that 3 weeks of CORT administration in rats causes dendritic remodeling and spine density reorganization in the pyramidal neurons of the CA1 subfield of the ventral hippocampus.
Most of the information about the effects of stress on the central nervous system comes from studies using male rats. Female rats have a short but prominent estrous cycle (4–5 days), and natural fluctuations in the levels of steroid hormones, specifically estrogens, may promote morphological changes that are related to the density of dendritic spines (Woolley et al., Citation1990) and to performance on learning and memory tests (Bisagno et al., Citation2003). In addition, the experimental designs of studies of different treatments in female rats consequently involve procedures such as ovariectomy and hormone replacement. However, Sutcliffe et al. (Citation2007) noted that although these procedures can change experimental outcomes dramatically, the resultant data cannot be assumed to reflect true sex differences. Moreover, Prendergast et al. (Citation2014) indicates that female mice of 40 strains are no more variable than males in terms of a broad array of biological traits, and argue that estrous cyclicity does not add measurably to experimental variability.
Women are more likely than men to develop stress-related disorders, such as anxiety disorder and post-traumatic stress disorder (PTSD; Galea et al., Citation1997; Garrett & Wellman, Citation2009). The development of these disorders is observed despite the neuroprotective activity of estrogens (Bisagno et al., Citation2003). Despite the propensity for stress disorder in females, there is little evidence on sex-related differences in the effects of stress on dendritic morphology in the ventral hippocampus. Therefore, the objective of this study was to evaluate the effect of stress that was induced by chronic restraint on the dendritic morphology of CA1 ventral hippocampal neurons (using Golgi–Cox stain) and on spatial learning and memory (as evaluated by the Morris water maze) in male and female rats.
Materials and methods
Subjects
Forty-eight adult Long Evans (LE) rats (24 females and 24 males) that were 58 postnatal days old and weighed 210 ± 20 g (females) and 250 ± 20 g (males) were obtained from the animal facility of the Claude Bernard vivarium of Universidad Autónoma de Puebla (UAP). The animals were kept on a 12:12 light:dark cycle, housed at 22 ± 1 °C and 55% relative humidity with free access to food and water. We formed two cohorts of four experimental groups each. Assignment to the groups was random with the exception of sex differences. In each cohort, there was a control group of female rats (Female-CON), a group of stressed female rats (Female-STR), a control group of male rats (Male-CON) and a group of stressed male rats (Male-STR). The first cohort (n = 6 rats per group) was assigned for morphological analysis of ventral hippocampal CA1 neurons using Golgi–Cox staining. The second cohort (n = 8 rats per group) was used to study spatial memory as measured by performance in the Morris water maze. Vaginal smears were taken from all of the females of the second cohort before subjecting them to movement restraint to determine their initial estrous status. The smear sample was collected with a sterile loop and placed on a slide. The slides were rinsed, stained with hematoxylin–eosin, rinsed again, dried and examined under light microscopy. The day of the estrous cycle was determined by the presence of leukocytes, nucleated epithelial cells and cornified epithelial cells (Bowman et al., Citation2001). The experimental protocol was then begun for each female at the same stage of the estrous cycle (diestrus). All experimental procedures were approved by the UAP Animal Care Committee and met governmental guidelines (Mexican Council for Animal Care, Norma Oficial Mexicana NOM-062-ZOO-1999) and the standards of the National Institutes of Health Guide for the Care and Use of Laboratory Animals. All efforts were made to minimize animal suffering and to reduce the number of animals that were used.
Chronic restraint
Female-STR and male-STR were placed in an acrylic restrainer that was 9 cm wide, 10 cm high and 10 cm deep for 6 h a day (10:00–16:00 h) for 25 days to produce chronic stress. As it had been found previously that the CA1 region of the hippocampus is not affected by 21 days of 6-h restraint (Conrad, Citation2006; Galea et al., Citation1997), we decided to increase stress exposure to 25 days to determine if the CA1 region is altered by stress. The rats were returned to their cages immediately after the restraint period. Control rats were always kept in home cages. Four additional days of restraint treatment was used to avoid habituation, as that mentioned by Horner et al. (Citation1991).
Golgi–Cox stain
On the day after the final day of restraint, the animals of the first cohort were deeply anesthetized with sodium pentobarbital (70 mg/kg; Juárez et al., Citation2008) and immediately perfused intracardially with 100 ml saline solution (0.9%) to remove clear excess blood from whole brain. The brain was removed and incubated in 20 ml of Golgi–Cox solution for 14 days in complete darkness. The Golgi–Cox solution was replaced by 30% sucrose at the end of this period, and the brains were kept in this sucrose solution for 2 days before being sectioned (Gibb & Kolb, Citation1998). The brains were sectioned at the level of the ventral hippocampus (; Plates 37–42, Paxinos & Watson, Citation1998) using a manual vibrotome. Sections that were 200 µm thick were obtained and mounted on gelatinized slides (2%) and kept in a humid chamber overnight. The next day, the slides were incubated for 30 min in ammonium hydroxide, rinsed and incubated for 30 min in Kodak rapid fixer (Kodak, México). The tissue was then rinsed immediately and dehydrated through ascending alcohols and xylene. The sections were fixed with synthetic resin (Entellan) and were analyzed by light microscopy 2 days later.
Figure 1. Schematic diagram of coronal sections of CA1 ventral hippocampus. Adapted from Paxinos & Watson (Citation1998). Coordinates indicate position relative to Bregma.
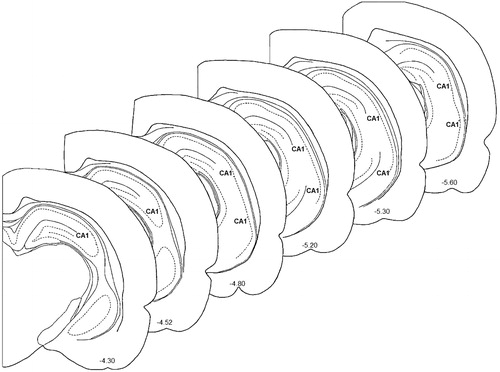
We selected 10 ventral hippocampal CA1 neurons from each brain (5 from the right hemisphere and 5 from the left Hemisphere) that had a typical form of CA1 pyramidal neuron, optimal impregnation staining, three first-order basilar dendrites and complete dendritic trees that had no apparent damage. These neurons were drawn at a magnification of 400 × using a camera lucida adapted to a DME Leica microscope. The basolateral and apical arbors were analyzed separately via Sholl analysis. Briefly, a transparent grid with equidistant concentric rings (10 µm) was placed on the image. The numbers of dendritic intersections per concentric ring were calculated to estimate the dendritic arborization and the total dendritic length (Flores et al., Citation2005; Silva-Gómez et al., Citation2003, Citation2013). In addition, third-order dendritic segments were drawn at a magnification of 1000× to calculate the dendritic spine density, which is the number of dendritic spines in relation to a dendritic length of 10 µm.
Spatial learning and memory
On the day after the final day of restraint, the rats of the second cohort were tested for spatial learning and memory using the Morris water maze, which consists of a circular pool that is 1.40 m in diameter at the top, 1.35 m in diameter at the base and 0.80 m high. The tub was divided into four imaginary sectors that were defined by the cardinal compass points. An escape platform (0.40 m high and 0.15 m in diameter) was placed in the pool in the SE sector 2 cm below the water level (Morris, Citation1984). The pool was located in a quiet room that had extra-maze cues on the wall to help the animals learn and memorize the location of the escape platform. The water was maintained at a constant temperature of 22 ± 1 °C and was stained with 100 ml of titanium dioxide (a non-toxic colorant). The animals were taken to the room 20 min before behavioral testing to allow them to acclimate. The rats were then subjected to four trials per day, with 50-min inter-trial intervals, on 6 experimental days. The first four trials (habituation phase: day 1) consisted of placing the rats in the pool at each of the cardinal points in a W, E, S and N order. The order of the starting points has been varied, and the sequences between each day are not repeated.
The rats had 120 s to locate the escape platform. If they could not or did not find the escape platform in 120 s, they were placed on it for 30 s to promote visual cue-based learning of the location of the escape platform. Escape latencies in seconds (the time taken to find and climb on the escape platform) were recorded. Days 2–5 were related with learning stage (trials 5 through 20). The memory test (experimental day 6) was conducted on the 15th day after the last day of the learning test. Escape latency was again recorded.
Statistical analysis
The mean values for each animal were considered to be a single value for data analysis. Data on total dendritic length, dendritic spine density and learning and memory were analyzed by two-way ANOVA followed by a post-hoc Bonferroni test for comparisons with chronic stress treatment and sex as independent factors. Dendritic branching was analyzed via repeated-measures two-way ANOVA with treatment and the number of concentric rings as independent factors. The differences were considered significant at p < 0.05. Graph Pad 4.0 software (GraphPad Software, Inc., La Jolla, CA) was used for all analyses.
Results
The effect of stress on the morphology of CA1 neurons of the ventral hippocampus was examined in 240 neurons (120 from female and 120 from male rats). shows representative photomicrographs of CA1 neurons and dendritic spines that had been impregnated with Golgi–Cox stain for each experimental and control group (A, C and D are of the Female-CON group; B, E and F are of the Female-STR group; G, I and J are of the Male-CON group; and H, K and L are of the Male-STR group).
Figure 2. Photomicrograph illustrating ventral hippocampus CA1 neurons of (A) Female-CON, (B) Female-STR, (G) Male-CON, (H) Male-STR rats. Dendritic spines are also shown. (C) Apical spines and (D) basilar spines in Female-CON rats; (E) basilar spines and (F) apical spines in Female-STR rats; (I) basilar spines and (J) apical spines in Male-CON rats; (K) apical spines and (L) basilar spines in Male-STR rats. These neurons were impregnated with Golgi–Cox stain.
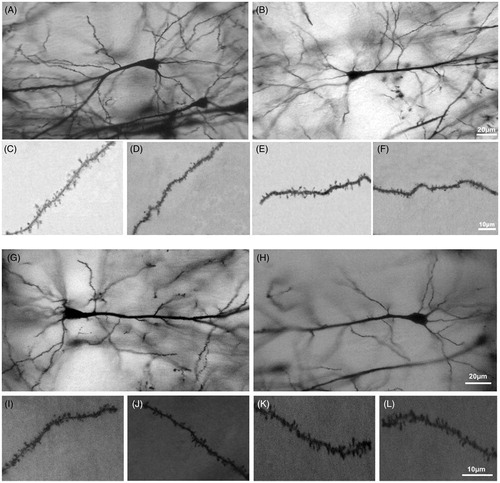
Total dendritic length and density of dendritic spines were estimated using methods that have been used in previous studies (Flores et al., Citation2005; Juárez et al., Citation2008; Martínez-Téllez et al., Citation2009; Silva-Gómez et al., Citation2013). Dendritic arborization was analyzed using the Sholl method. A two-way ANOVA for differences in basilar spine density shows that chronic restraint promotes a significant decrease in dendritic spine density in female rats (STR, F(1,20) = 6.303, p = 0.0208; Sex, F(1,20) = 1.522, p = 0.2317; ). The dendritic spine density of apical trees was not affected by stress in any of the experimental groups () and did not differ significantly between males and females.
Figure 3. Dendritic spines density. (A) Basilar and (B) apical ventral hippocampal CA1 neurons of stressed rats. The decrease in the density of basilar spines in Female-STR rats was significant (*p < 0.05).
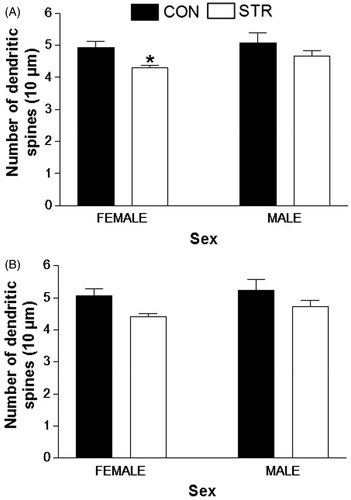
Furthermore, dendritic branching of basilar CA1 neurons in female rats () and male rats () was not affected by stress. There were no significant differences in the total length of dendritic branching trees (). However, there was significantly more dendritic apical branching in female-STR rats in the middle section of the dendritic tree (Two-way ANOVA, STR, F(1,270) = 50.24, p < 0.01; intersection per shell, F(26,270) = 67.65, p < 0.0001; interaction between STR and intersection per shell F(26,270) = 1.968, p = 0.0042; ). There were no changes in apical dendritic branching in male-STR rats (). Overall dendritic length in male-STR rats was not different from that in female-STR rats (STR, F(1,20) = 4.343, p = 0.0502; Sex, F(1,20) = 2.168, p = 0.1565; interaction between STR and sex F(1,20) = 1.734, p = 0.2028; ). No significant sex differences were observed.
Figure 4. Basilar dendritic tree analysis of ventral hippocampal CA1 neurons in female and male rats that were stressed by chronic restraint. (A and B) Dendritic arborization and (C) total dendritic length. There were no significant differences.
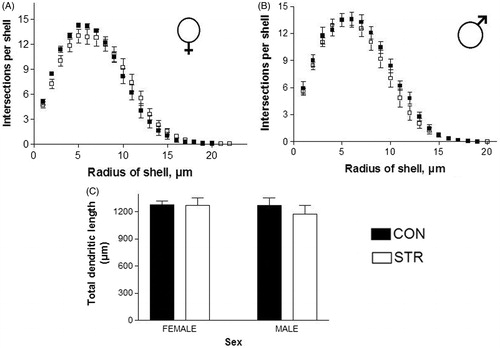
Figure 5. Apical dendritic tree analysis of ventral hippocampal CA1 neurons in female and male rats that were stressed by chronic restraint. (A) Arborization in the middle section was increased by stress in female rats. (B) There was no difference in arborization in stressed male rats. (C) Total dendritic length; there is a tendency toward increased dendritic length (p = 0.0502).
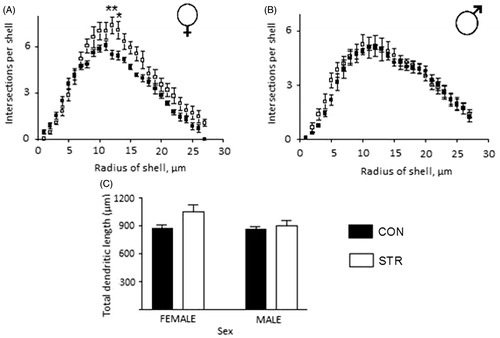
The results of spatial memory testing in the Morris water maze are shown in . Female-STR rats showed a significant increase in escape latency compared with female-CON rats (STR, F(1,336) = 7.251, p < 0.01). There was no significant difference in escape latency between the male-CON and male-STR rats (STR, F(1,336) = 2.299, p = 0.1078). We also analyzed the data for each test phase. There were no significant differences between the groups or by treatment or sex during the learning phase (). However, the female-STR rats showed an increase in escape latency during the memory phase (STR, F(1,27) = 8.152, p < 0.01; Sex, F(1,27) = 0.1034, p = 0.7503; interaction between STR and sex F(1,27) = 4.671, p = 0.0397) (). There was no significant difference in escape latency between male-STR and male-CON in the memory phase.
Figure 6. Spatial learning and memory in stressed rats. (A) Female and (B) male rats. The mean ± SE of escape latency over 24 trials is shown, **p < 0.01. (C) The learning phase: no difference was observed between groups. (D) The memory phase: escape latency was increased in the stressed female group compared with the female control group.
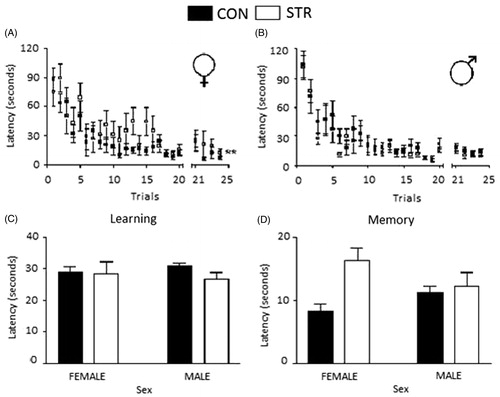
Finally, the mean length of the estrous cycle during the restraint period was 5.713 ± 0.405 days in female-STR rats and 5.738 ± 0.386 days in female-CON rats. This difference was not significant.
Discussion
This study evaluated the effects of stress due to restricted movement (restraint stress) on the morphology of ventral hippocampal CA1 neurons as well as spatial learning and memory in Long–Evans female and male rats. Our results revealed that stress caused a decrease in dendritic spine density and spatial memory deficits in female rats but did not cause these differences in male animals.
The strain of the rat is an important factor in stress research because different strains exhibit different sensitivities; thus, caution is necessary when extrapolating results between strains (McLaughlin et al., Citation2007). Interestingly, LE rats exhibit superior spatial task performance (Epp et al., Citation2011) but are rarely used to study stress phenomena, possibly because this strain of rat is susceptible to developing ulcers (Pare, Citation1990). Sprague–Dawley (SD) and Wistar rats are commonly used in studies of chronic restraint stress (Cook & Wellman, Citation2004; Conrad et al., Citation1996; Gerrits et al., Citation2003; Mitra et al., Citation2005; Mizoguchi et al., Citation2000; Luine, Citation2002; Perez-Cruz et al., Citation2007; Radley et al., Citation2006; Sandi et al., Citation2003; Vyas et al., Citation2002). However, these strains have poor visual acuity, which can contribute to differences in the performance of behavioral tasks (Prusky et al., Citation2002).
Prior studies have controlled for the short estrous cycle of female rats by ovariectomy with or without hormonal replacement (Gould et al., Citation1990; Hajszan et al., Citation2004; McLaughlin et al., Citation2005; Muñoz-Cueto et al., Citation1990; Stewart & Kolb, Citation1994; Woolley & McEwen, Citation1993). However, these manipulations in the rats do not assure differences between the sexes (Sutcliffe et al., Citation2007), and according to a meta-analysis study, the estrous cycle is not a source of variability in the parameters studied in neuroscience and biomedical research (Prendergast et al., Citation2014). In addition, there are reports of female rats weighing 200–220 g that have regular estrous cycles, as well as stressed female rats having estrous cycles that are unchanged in length during and after the period of stress, and this observation implies normal ovarian function (Bowman et al., Citation2001, Citation2003; Bisagno et al., Citation2003). The second cohort of rats was used only to measure spatial learning and memory in relation to the estrous cycle and revealed that the stressed female rats had estrous cycle durations that were similar to those of the control rats.
The reports on the effects of chronic restraint-induced stress on hippocampal neuron morphology described alterations of both dendritic trees of the dorsal CA3 neurons (Galea et al., Citation1997; Watanabe et al., Citation1992); however, more consistent findings have been related to atrophy of the apical dendritic trees (Conrad et al., Citation2006, Citation2007; Hutchinson et al., Citation2012; Magariños et al., Citation1996, Citation1997; McLaughlin et al., Citation2005), possibly because this region is the first structure to respond to chronic stress (McLaughlin et al., Citation2007).
Unlike previous reports using prenatal stress, forced-immobilization stress, social isolation stress and corticosterone or dexamethasone treatment (Martínez-Tellez et al., Citation2009; Morales-Medina et al., Citation2009; Sicilia et al. Citation2007; Silva-Gomez et al., Citation2003, Citation2013), restraint stress for 6 h a day for 25 days did not induce changes in the densities of the dendritic spines of the ventral CA1 neurons in the male rats. However, this treatment produced morphological changes in the stressed female rats. Changes in the dendritic spine densities of CA1 neurons of the dorsal hippocampus following ovariectomy and hormonal manipulation have been described in female rats (Gould et al., Citation1990; Hajszan et al., Citation2004). However, there are contradictory findings; changes in the numbers of spines with large heads have been observed in the absence of changes in overall density (Li et al., Citation2004; McLaughlin et al., Citation2005; Murakami et al., Citation2006; Wallace et al., Citation2006). These discrepant results might be due to the characteristics of the response to stress of each strain of rat, each animal species or animal sex.
Alterations in dendritic spine density may reflect changes in neuronal connectivity. Increases in the numbers of dendritic spines suggest increases in the numbers of glutamatergic synaptic connections (Horner & Arbuthnott, Citation1991). It is well known that the increase in corticosterone levels that occurs during movement restraint (Bowman et al., Citation2001; Garrett & Wellman, Citation2009) promotes significant changes in the metabolism of Ca2 + -permeable NMDA receptors that disrupt the cytoskeleton and may ultimately result in the retraction of the spines (Woolley & McEwen, Citation1994).
In our study, dendritic arborization and total dendritic lengths of ventral hippocampal CA1 neurons are minimally affected by stress. However, dendritic remodeling has been observed in the middle portion of the basilar dendritic tree of female rats following increases in corticosterone levels, supporting the notion that differences in the expression and sensitivity of NMDA receptors may affect neuronal connectivity independently of changes in dendritic spine density (Conrad et al., Citation2006). It is possible that the neuroprotective effects of estrogens (Bisagno et al., Citation2003; Hoffman et al., Citation2006) promote dendritic remodeling as a compensatory mechanism in response to the loss of synaptic input from the CA3 region resulting from stress-induced dendritic atrophy (Magariños et al., Citation1996; McKittrick et al., Citation2000; McLaughlin et al., Citation2005; Watanabe et al., Citation1992). The reduction in apical CA3 dendritic arborization may be due to the inhibition of glucose metabolism by corticosteroids, increased excitatory amino acid metabolism or increase in the activity of the calcium-dependent protease, which can damage cytoskeletal proteins (Haynes et al., Citation2004; Magariños et al., Citation1997).
Studies of the effects of stress and sexual dimorphism have reported impaired learning and spatial memory in male rats compared to female rats (Luine et al., Citation1994), which are less susceptible to stress-induced cognitive impairment (Bisagno et al., Citation2003). However, in this study, LE male rats did not perform poorly, but the female rats exhibited a deficit in spatial memory. This deficit may be temporary (Conrad et al., Citation2006) and may be related to the high activity of exploration expressed by the female rats beginning in the early stages of life (Eiland et al., Citation2012) that is exacerbated under stressful conditions.
Dendritic retraction observed in CA3 neurons after stress are thought to cause deficits in learning and memory. However, Conrad et al. (Citation2007) and McLaughlin et al. (Citation2005) suggested that these two variables are independent, as the morphologies of CA3 neurons do not always predict spatial memory performance. Specifically, it has been proposed that spatial memory deficits result from increases in corticosterone levels that occur subsequent to the activation of the hypothalamic–pituitary–adrenal axis and the generation of oxidative stress at the level of the hippocampus (Ghadrdoost et al., Citation2011).
Other parameters that are altered by chronic stress and differ between the sexes are corticosterone levels and neurotransmitter systems (Bowman et al., Citation2001). In female rats, stress produces a 2- to 3-fold increase in the concentration of corticosterone, but 7- to 10-fold increases have been observed in male rats after 1 h of restraint (Cook & Wellman Citation2004; Galea et al., Citation1997; Watanabe et al., Citation1992). In male rats, stress alters dopamine and GABA levels, while in female rats stress promotes increases in norepinephrine and serotonin (Bowman et al., Citation2003). Moreover, female rats may habituate more easily to stress than male rats (Garrett & Wellman, Citation2009).
Recently, structural and functional differences between the dorsal and ventral hippocampus have been reported. Dexamethasone treatment promotes dendritic retraction of ventral hippocampal CA1 neurons without affecting the neurons of the dorsal hippocampus in male rats (Silva-Gómez et al., Citation2013). However, Lambert et al. (Citation1998) found that multiple exposures to stress promote apical dendritic atrophy of the CA3 and CA1 neurons of the dorsal hippocampus. Together, these data support earlier observations about the structural and functional differences between the dorsal and ventral hippocampus (Fanselow & Dong, Citation2010) and the possible differences between strains.
In summary, chronic stress due to restricted movement affects dendritic parameters of the CA1 pyramidal neurons in the ventral hippocampus and spatial memory in female but not male LE rats. The expressions of hormone receptors vary between females and males, and thus it is possible that the female ventral CA1 neurons expressed greater numbers of corticosterone receptors and may be more sensitive to corticosterone fluctuations. Further specific studies of the relationship between the expressions of glucocorticoid and corticosteroid receptors and sex differences are needed.
Declaration of interest
There are no conflicts of interest among the authors. This work was supported by F-PROMEP-21/Rev 05 SEP-23-003-A and CA-PIFI 2009. Support was provided to AB Silva-Gómez. None of the funding institutions had any further role in the study design, collection of data, analyses and interpretation of data, writing of the report or decision to submit the paper for publication. Elsevier Language Editing Services was hired for editing the English language text. All authors have no conflicts of interest.
Acknowledgements
The authors wish to thank Dr. Carlos Escamilla for his help with the animal care and Dr. Daniel I. Limón for his help with the Morris water maze. AMR and DABD performed the morphologic analyses, ALM and HDLLT applied the water maze test and ABSG conducted the data analysis and wrote the paper.
References
- Akirav I, Levin GR. (2002). Mechanisms of amygdala modulation of hippocampal plasticity. J Neurosci 22:9912–21
- Argumedo SG, Martínez-Ávila MA, Peraza VA, Zavala Gonzáles MA, Rojas GL, Mejía-Barragán GM. (2007). Efecto protector del pirofosfato de tiamina en el hipocampo de ratas expuestas a estrés por inmovilización forzada. Vet Mex 38:429–38
- Bisagno V, Bowman RE, Luine VN. (2003). Functional aspects of estrogen neuroprotection. Endocrine 21:33–41
- Bowman RE, Beck KD, Luine VN. (2003). Chronic stress effects on memory: sex differences in performance and monoaminergic activity. Horm Behav 43:48–59
- Bowman RE, Zrull MC, Luine VN. (2001). Chronic restraint stress enhances radial arm maze performance in female rats. Brain Res 904:279–89
- Christian KM, Miracle AD, Wellman CL, Nakazawa K. (2011). Chronic stress-induced hippocampal dendritic retraction requires CA3 NMDA receptors. Neuroscience 174:26–36
- Conrad CD, Galea LA, Kuroda Y, McEwen BS. (1996). Chronic stress impairs rat spatial memory on the Y maze, and this effect is blocked by tianeptine pretreatment. Behav Neurosci 110:1321–34
- Conrad CD, McLaughlin KJ, Harman JS, Foltz C, Wieczorek L, Lightner E, Wright RL. (2007). Chronic glucocorticoids increase hippocampal vulnerabilitily to neurotoxicity under conditions that produce CA3 dendritic retraction but fail to impair spatial recognition memory. J Neurosci 27(31):8278–85
- Conrad CD. (2006). What is the functional significance of chronic stress-induced CA3 dendritic retraction within the hippocampus? Behav Cogn Neurosci Rev 5(1):41–60
- Cook SC, Wellman CL. (2004). Chronic stress alters dendritic morphology in rat medial prefrontal cortex. J Neurobiol 60:236–48
- Eiland L, Ramroop J, Hill MN, Manley J, McEwen BS. (2012). Chronic juvenile stress produces coritoclimbic dendritic architectural remodeling and modulates emotional behavior in male and female rats. Psychoneuroendocrinology 37:39–47
- Epp JR, Scott NA, Galea LAM. (2011). Strain differences in neurogenesis and activation of new neurons in the dentate gyrus in response to spatial learning. Neuroscience 172:342–54
- Fanselow MS, Dong H-W. (2010). Are the dorsal and ventral hippocampus functionally distinct structures? Neuron 65(1):7–19
- Flores G, Alquicer G, Silva-Gómez AB, Rivera G, Quirion R, Srivastava K. (2005). Alterations in dendritic morphology of prefrontal cortical and nucleus acumbens neurons in adult rats after neonatal excitotoxic lesions of the ventral hippocampus. Neuroscience 133(2):463–70
- Galea LA, McEwen BS, Tanapat P, Deak T, Spence RL, Dhabhar FS. (1997). Sex differences in dendritic atrophy of CA3 pyramidal neurons in response to chronic restraint stress. Neuroscience 81:689–97
- Garrett JE, Wellman CL. (2009). Chronic stress effects on dendritic morphology in medial prefrontal cortex: sex differences and estrogen dependence. Neuroscience 162:195–207
- Gerrits M, Westenbroek C, Fokkema DS, Jongsma ME, Den Boer JA, Ter Horst GJ. (2003). Increased stress vulnerability after a prefrontal cortex lesion in female rats. Brain Res Bull 61:627–35
- Ghadrdoost B, Vafaei AA, Rashidy-Pour A, Hajisoltani R, Bandegi AR, Motamedi F, Haghighi S, et al. (2011). Protective effects of saffron extract and its active constituent crocin against oxidative stress and spatial learning and memory deficits induced by chronic stress in rats. Eur J Pharmacol 667(1–3):222–9
- Gibb R, Kolb B. (1998). A method for vibratome sectioning of Golgi-Cox stained whole rat brain. J Neurosci Methods 79:1–4
- Gould E, Woolley CS, Frankfurt M, McEwen BS. (1990). Gonadal steroids regulate dendritic spine density in hippocampal pyramidal cells in adulthood. J Neurosci 10(4):1286–91
- Hajszan T, Maclusky NJ, Leranth C. (2004). Dehydroepiandrosterone increases hippocampal spine synapse density in ovariectomized female rats. Endocrinology 145(3):1042–5
- Haynes LE, Barber D, Mitchell IJ. (2004). Chronic antidepressant medication attenuates dexamethasone-induced neuronal death and sublethal neuronal damage in the hippocampus and striatum. Brain Res 1026:157–67
- Hoffman GE, Merchenthaler I, Zup SL. (2006). Neuroprotection by ovarian hormones in animal models of neurological disease. Endocrine 29(2):217–31
- Horner CH, Arbuthnott E. (1991). Methods of estimation of spine density – are spines evenly distributed throughout the dendritic field? J Anat 177:179–84
- Horner CH, O’Regan M, Arbuthnott E. (1991). Neural plasticity of the hippocampal (CA1) pyramidal cell-quantitative changes in spine density following handling and injection for drug testing. JAnat 174:229–38
- Hosseini-sharifabad M, Hadinedoushan H. (2007). Prenatal stress induces learning deficits and is associated with a decrease in granules and CA3 cell dendritic tree size in rat hippocampus. Anat Sci Int 82:211–17
- Hutchinson KM, McLaughlin KJ, Wright RL, Ortiz JB, Anouti DP, Mika A, Diamond DM, Conrad CD. (2012). Environmental enrichment protects against effects of chronic stress on cognitive and morphological measures of hippocampal integrity. Neurobiol Learn Mem 97:250–60
- Juárez I, Gratton A, Flores G. (2008). Ontogeny of altered dendritic morphology in the rat prefrontal cortex, hippocampus, and nucleus accumbens following cesarean delivery and birth anoxia. J Comp Neurol 507:1734–47
- Kim JJ, Diamond DM. (2002). The stressed hippocampus, synaptic plasticity and lost memory. Nature 3:453–62
- Krugers HJ, Goltstein PM, van der Linden S, Joëls M. (2006). Blockade of glucocorticoid receptors rapidly restores hippocampal CA1 synaptic plasticity after exposure to chronic stress. Eur J Neurosci 23:3051–5
- Lambert KG, Buckelew SK, Staffiso-Sandoz G, Gaffga S, Carpenter W, Fisher J, Kinsley CH. (1998). Activity-stress induces atrophy of apical dendrites of hippocampal pyramidal neurons in male rats. Physiol Behav 65(1):43–9
- Li C, Brake WG, Romeo RD, Dunlop JC, Gordon M, Buzescu R, Magariños AM, et al. (2004). Estrogen alters hippocampal dendritic spine shape and enhances synaptic protein immunoreactivity and spatial memory in female mice. Proc Natl Acad Sci USA 101(7):2185–90
- Luine V, Villegas M, Martinez C, McEwen BS. (1994). Repeated stress causes reversible impairments of spatial memory performance. Brain Res 639:167–70
- Luine V. (2002). Sex differences in chronic stress effects on memory in rats. Stress 5:205–16
- Magariños AM, García-Verduga JM, McEwen BS. (1997). Chronic stress alters synaptic terminal structure in hippocampus. Proc Natl Acad Sci USA 94:14002–8
- Magariños AM, McEwen BS, Flügge G, Fuchs E. (1996). Chronic psychosocial stress causes apical dendritic atrophy of hippocampal CA3 pyramidal neurons in subordinate tree shrews. J Neurosci 16(10):3534–40
- Martínez-Téllez RI, Hernández-Torres E, Gamboa C, Flores G. (2009). Prenatal stress alters spine density and dendritic length of nucleus accumbens and hippocampus neurons in rat offspring. Synapse 63:794–804
- McKittrick CR, Magariños AM, Blanchard DC, Blanchard RJ, McEwen BS, Sakaf RR. (2000). Chronic social stress reduces dendritic arbors in CA3 of hippocampus and decreases binding to serotonin transporter sites. Synapse 36:85–94
- McLaughlin KJ, Baran SE, Wright RL, Conrad CD. (2005). Chronic stress enhanced spatial memory in ovariectomized female rats despite CA3 dendritic retraction: possible involvement of CA1 neurons. Neuroscience 135:1045–54
- McLaughlin KJ, Gomez J, Baran SE, Conrad CD. (2007). The effects of chronic stress on hipocampal morphology and function: an evaluation of chronic restraint paradigms. Brain Res 1161:56–64
- Mitra R, Jadhav S, McEwen BS, Vyas A, Chattarji S. (2005). Stress duration modulates the spatiotemporal patterns of spine formation in the basilar amygdale. Proc Natl Acad Sci USA 102:9371–6
- Mizoguchi K, Yuzurihara M, Ishige A, Sasaki H, Chui DH, Tabira T. (2000). Chronic stress induces impairment of spatial working memory because of prefrontal dopaminergic dysfunction. J Neurosci 20:1568–74
- Morales-Medina JC, Sanchez F, Flores G, Dumont Y, Quirion R. (2009). Morphological reorganization after repeated corticosterone administration in the hippocampus, nucleus accumbens and amygdala in the rat. J Chem Neuroanat 38:266–72
- Morris R. (1984). Developments of a water-maze procedure for studying spatial learning in the rat. J Neurosci Methods 11:47–60
- Muñoz-Cueto JA, García-Segura LM, Ruiz-Marcos A. (1990). Developmental sex differences and effect of ovariectomy on the numbrer of cortical piramidal cell dendritic spines. Brain Res 515:64–8
- Murakami G, Tsurugizawa T, Hatanaka Y, Komatsuzaki Y, Tanabe N, Mukai H, Hojo Y, et al. (2006). Comparison between basal and apical dendritic spines in estrogen-induced rapid spinogenesis of CA1 principal neurons in the adult hippocampus. Biochem Biophys Res Commun 351(2):553–8
- Pare WP. (1990). Technique and strain comparisons in stress ulcer. Ann NY Acad Sci 597:223–30
- Paxinos G, Watson C. (1998). The rat brain in stereotaxic coordinates. 4th ed. New York: Academic Press
- Perez-Cruz C, Muller-Keuker JIH, Heilbronner U, Fuchs E, Flugge G. (2007). Morphology of pyramidal neurons in the rat prefrontal cortex: lateralized dendritic remodeling by chronic stress. Neural Plasticity 2007:1–14
- Prendergast BJ, Onishi KG, Zucker I. (2014). Female mice liberated for inclusion in neuroscience and biomedical research. Neurosci Biobehav Rev 40:1–5
- Prusky GT, Harker KT, Douglas RM, Whishaw IQ. (2002). Variation in visual acuity within pigmented, and between pigmented and albino rat strains. Behav Brain Res 136:339–48
- Radley JJ, Rocher AB, Miller M, Janssen WGM, Liston C, Hof PR, McEwen BS, Morrison HJ. (2006). Repeated stress induces dendritic spine loss in the rat medial prefrontal cortex. Cerebral Cortex 16:313–20
- Sandi C, Davies HA, Cordero MI, Rodriguez JJ, Popov VI, Stewart MG. (2003). Rapid reversal of stress induced loss of synapses in CA3 of rat hippocampus following water maze training. Eur J Neurosci 17:2447–56
- Selye HA. (1956). Syndrome produced by diverses nocuous agents. Nature 138:132
- Sicilia AG, Martínez AMA, Valenzuela PA, González Z MA, Granados RL, Barragán MMG. (2007). Efecto protector del pirofosfato de tiamina en el hipocampo de ratas expuestas a estrés por inmovilización forzada. Vet Méx 38(4):429–38
- Silva-Gómez AB, Aguilar-Salgado Y, Reyes-Hernández DO, Flores G. (2013). Dexamethasone induces different morphological changes in the dorsal and ventral hippocampus of rats. J Chem Neuroanat 47:71–8
- Silva-Gómez AB, Rojas D, Juárez I, Flores G. (2003). Decreased dendritic spine density on prefrontal cortical and hippocampal pyramidal neurons in postweaning social isolation rats. Brain Res 983:128–36
- Stewart J, Kolb B. (1994). Dendritic branching in cortical pyramidal cells in response to ovariectomy in adult female rats: suppression by neonatal exposure to testosterone. Brain Res 654:149–54
- Sutcliffe JS, Marshall KM, Neill JC. (2007). Influence of sex on working and spatial memory in the novel object recognition task in the rat. Behav Brain Res 177:117–25
- Vyas A, Mitra R, Shankaranarayana Rao BS, Chattarji S. (2002). Chronic stress induces contrasting patterns of dendritic remodeling in hippocampal and amygdaloid neurons. J Neurosci 22:6810–18
- Wallace M, Luine V, Arellanos A, Frankfurt M. (2006). Ovariectomized rats show decreased recognition memory and spine density in the hippocampus and prefrontal cortex. Brain Res 1126:176–82
- Watanabe Y, Gould E, McEwen BS. (1992). Stress induces atrophy of apical dendrites of hippocampal CA3 pyramidal neurons. Brain Res 588:341–5
- Woolley CS, Gould E, Frankfurt M, McEwen BS. (1990). Naturally occurring fluctuation in dendritic spine density on adult hippocampal pyramidal neurons. J Neurosci 10(12):4035–9
- Woolley CS, McEwen BS. (1993). Roles of estradiol and progesterone in regulation of hippocampal dendritic spine density during the estrous cycle in the rat. J Comp Neurol 336:293–306
- Woolley CS, McEwen BS. (1994). Estradiol regulates hippocampal dendritic spine density via an N-Methyl-D-Aspartate receptor-dependent mechanism. J Neurosci 14(12):7680–7