Abstract
Birth asphyxia is a cause of neonatal death or adverse neurological sequelae. Biomarkers can be useful to clinicians in order to optimize intensive care management and communication of prognosis to parents. During perinatal adverse events, increased cortisol secretion is due to hypothalamo–pituitary–adrenal axis activation. We aimed to investigate if cortisol variations during therapeutic hypothermia are associated with neurodevelopmental outcome. We compared 18 cases (neonates with birth asphyxia) with 18 controls (healthy term newborns) and confirmed increased serum cortisol concentrations following the peri-partum adverse event. Among cases, we stratified patients according to neurological outcome at 18 months (group A – good; group B – adverse) and found that after 24 h of therapeutic hypothermia serum cortisol concentration was significantly lower in group A vs group B (28.7 ng/mL vs 344 ng/mL, *p = 0.01). In group B serum, cortisol concentration decreased more gradually during therapeutic hypothermia. We conclude that monitoring serum cortisol concentration during neonatal therapeutic hypothermia can add information to clinical evaluation of neonates with birth asphyxia; cortisol values after the first 24 h of hypothermia can be a biomarker associated with neurodevelopmental outcome at 18 months of age.
Introduction
In countries with a high level of perinatal care, the incidence of asphyxia responsible for moderate or severe hypoxic ischemic encephalopathy (HIE) is still 2–3 per 1000 term newborns, accounting for a large burden of medical and social costs (Bhutta & Black, Citation2013). Moderate hypothermia, started within 6 h after birth and continued for 72 h, can significantly improve survival and reduce neurologic impairment in neonates with HIE, so that it is now adopted as the standard therapy (Jacobs et al., Citation2013).
It is well known from animal models that when a severe hypoxic event occurs, the fetus is able to maintain adequate blood flow to brain, myocardium and adrenal glands for a few minutes, due to adaptive flow redistribution (Jensen et al., Citation1999). The critical time for inducing a significant reduction in adrenal blood flow after a total cord occlusion in the lamb fetus was reported to be 5 min (Hernandez-Andrade et al., Citation2005). The adrenal definitive zone (i.e. the smaller outer zone of the primordial adrenal cortex in the fetus, which develops into the zona fasciculata and zona glomerulosa) is stimulated to evoke a stress response due to this early compensatory vascular mechanism, so leading to elevated umbilical cord serum level of cortisol (Kohno et al., Citation1984; Procianoy et al., Citation1988). In the lamb fetus, moderate asphyxia induces a transient adrenocorticotropin increase (lasting 24 h) and a prolonged cortisol increase (for 72 h) (Gardner et al., Citation2001). Maternal cortisol is known to not strongly affect measurement of fetal cortisol secretion, since the mother and fetus/newborn respond independently to the stress of delivery complications (Kohno et al., Citation1984). Furthermore, in physiological situations, a diurnal cortisol rhythm is absent in healthy neonates during the first post-natal weeks, so that random cortisol levels in neonates are considered to be adequate to assess adrenocortical function (Gröschl et al., Citation2003).
A study on newborn piglets, exposed to a reduced inspired oxygen percentage and reduced respiratory rate to induce hypoxia/hypercapnia, found that there was a high degree of variability in changes in cardiovascular function (i.e. mean arterial blood pressure and heart rate) and in serum cortisol concentrations that the piglets showed in response to the hypoxia (Harris et al., Citation2009). More effective maintenance of cardiovascular function and higher serum cortisol concentrations were associated with a better neurological outcome, evaluated by means of electro-encephalography (EEG) and post-mortem immunohistochemistry (Harris et al., Citation2009). High cortisol levels were measured also in lamb neonates exposed to severe intrauterine asphyxia induced by total umbilical cord occlusion (Hernandez-Andrade et al., Citation2005).
As for a relationship between therapeutic hypothermia and cortisol levels, in the piglet model, the depth of hypothermia (35 °C, 33.5 °C or 30 °C) seemed not to influence cortisol levels, although deep hypothermia (i.e. 30 °C) had intolerable side effects (Kerenyi et al., Citation2012).
In order to seek early markers of the later consequences of perinatal asphyxia, we investigated whether serum cortisol variations during 72 h of induced neonatal hypothermia could be associated with neurodevelopmental outcome. Our working hypothesis was that serum cortisol concentrations, likely consequent on exposure to the perinatal stressor of asphyxia, could be an early marker of neurodevelopmental outcome at 18 months if measured during the first 72 h after birth.
Methods
Participants
We performed an observational case–control study. Cases (n = 18; 10 males and 8 females) were patients admitted with perinatal asphyxia to the neonatal intensive care unit (NICU) of Pisa Hospital (Italy) from April 2009 to January 2012.
The enrolment criteria for patients were the same as reported in larger previous international studies on neonatal hypothermia. Inclusion criteria were as follows: (i) gestational age (GA) ≥36 weeks and birthweight ≥1800 g, admitted to NICU within 6 h after birth; (ii) metabolic criteria, i.e. Apgar score ≤5 at 10 min or persisting need for resuscitation, including endotracheal intubation or mask ventilation for more than 10 min after birth, or acidosis (pH ≤7.0 and/or base deficit ≥−16 mmol/L in umbilical cord blood or arterial, venous or capillary blood) within 60 min from birth; (iii) neurological criteria modified from Sarnat & Sarnat (Citation1976): moderate to severe encephalopathy consisting of altered state of consciousness (irritability, lethargy, stupor or coma) and one or more of the following signs: hypotonia or abnormal reflexes including oculomotor or pupil abnormalities, or absent or weak sucking, or clinical seizures; (iv) moderately to severely abnormal amplitude-integrated EEG (aEEG) pattern or seizures; and (v) written informed consent from parents (at least the father).
Exclusion criteria were as follows: (i) GA<36 weeks or birth weight <1800 g or admitted to the NICU after 6 h after birth; (ii) major congenital abnormalities or other syndromes, including brain malformations, congenital viral infections or evidence of encephalopathy other than hypoxic–ischemic encephalopathy (HIE); and (iii) informed consent refused.
All patients received moderate (33.5 °C) whole-body hypothermia for 72 h, using a cooling servo controlled blanket with esophageal and rectal temperature probes (Blanketrol III® Hyper-Hypothermia System, Cincinnati Sub-Zero, Cincinnati, OH) and subsequently were gradually re-warmed up to 36.5–37 °C over the following 6–12 h (0.5 °C/h). aEEG was continuously monitored during therapy.
Controls (n = 18) were healthy full-term newborns with no maternal, gestational or perinatal history of pathologies or risk factors, matched for sex (10 males and 8 females) and birthweight. Neither cases nor controls had antenatal exposure to exogenous corticosteroid.
The Local Ethics Committee approved our study. Parents of the asphyxiated newborns received full verbal and written information regarding hypothermic treatment and the possible risks involved and regarding collection of serial blood samples for hormonal measurements. Written informed consent was obtained prior to study entry. Parents of the control infants signed written informed consent as healthy volunteers. Helsinki Declaration statements were followed for the whole study conception.
Evaluations
We measured serum cortisol concentration (ng/mL) at T0, corresponding to time of admission to the NICU for cases (i.e. within 6 h after birth) or admission to the Nursery for controls (i.e. immediately after birth). Subsequently, we repeated blood sampling in cases at 24 (T1), 48 (T2), 72 (T3) and 96 (T4) hours after birth, to evaluate changes in cortisol concentrations.
For the cortisol assay, we collected a 1 ml serum sample for each determination from each neonate (median or cephalic or basilic vein blood sample). For the quantitative determination of serum cortisol concentrations, our clinical service laboratory performed the Access Cortisol assay (Access Immunoassay Systems, Beckman-Coulter®, Milano, Italy), which is a competitive binding immunoenzymatic assay. The manufacturer declares that this test has a total imprecision lower than 12% at cortisol concentration of 5 mcg/dL (138 nmol/L) and lower than 10% for higher cortisol concentrations; bilirubin up to 10 mg/dL, hemoglobin up to 500 mg/dL (due to hemolysis), triglycerides up to 1800 mg/dL or total protein up to 9 g/dL do not interfere with the assay. The minimum detectable level of cortisol distinguishable from zero with 95% confidence interval (analytical sensitivity) is 0.4 mcg/dL (11 nmol/L).
Patients were evaluated at 6, 12 and 18 months of age by administering the Bayley Scales of Infant Development, BSID III (Bayley, Citation2006). The cognitive, receptive and expressive language and fine and gross motor subscale scoring were recorded. The test was administered on an individual basis, and assessors were unaware of the hormonal assay data. An adverse neurodevelopmental outcome was defined as death or severe disability (i.e. scoring less than 10 ° percentile rank on each subscale of the BSID III).
Statistical analysis
Serum cortisol concentration data are reported as median and interquartile range (IQR), as a non-Gaussian distribution was found (Kolmogorov–Smirnov test). Therefore, we performed non-parametric statistical tests to compare groups, i.e. Mann–Whitney test for independent groups (comparison between different subgroups) and Wilcoxon test for paired data (comparison between different time-points in the same subgroup). In order to compare multiple samples (more than two) in paired groups, we performed the Friedman test. Correlations between the variables were explored by means of Spearman’s coefficient.
Results
At T0, the median measured serum cortisol concentration was 615 ng/mL (IQR: 615–615 ng/mL) in cases, and 15.8 ng/mL (IQR: 6.1–28.3 ng/mL) in controls (*p < 0.001, Mann–Whitney test).
In cases, after 24 h of hypothermic treatment (T1), cortisol values were significantly decreased vs. the T0 value, median cortisol concentration at T1 was 310.5 ng/mL (p = 0.003, Wilcoxon test), but with a large intra-group variation, IQR was 34–479.5 ng/mL.
When the cases were stratified according to their neurological outcome at 18 months of age, we obtained two similarly sized subgroups: (A) good outcome (i.e. minimal or no neurological impairment, as detailed in Methods), n = 8 (five males and three females) and (B) adverse outcome (i.e. death, which occurred in four patients, or moderate to severe neurological impairment, as detailed in Methods), n = 10 (five males and five females).
Comparisons between subgroups for cortisol concentration values at each time-point are listed in . Subgroups showed almost equal cortisol concentrations at T0. Thereafter, subgroup A showed significantly lower cortisol concentration vs subgroup B at T1 (p = 0.008, Mann–Whitney U test, n = 8, 10). At the other time-points, subgroup A median values were only slightly lower than those in subgroup B (). We found statistically significant differences among cortisol concentrations along the timecourse in subgroup A (Friedman test, *p < 0.001), but not in subgroup B (p = 0.252). In subgroup A, the decrease in serum cortisol concentrations from T0 to T1 approached statistical significance (p = 0.06; Wilcoxon Test), as well as from T0 to all other time-points. In contrast, in subgroup B, there was no significant change in cortisol concentration from T0 to T1 (p = 0.125, Wilcoxon test), while statistical significance was approached for the cortisol decrease from T0 to T2 (p = 0.06; Wilcoxon test) and subsequent time-points.
Figure 1. Serum cortisol concentrations within each subgroup at different time-points. T0: admission, <6 h after birth; T1: +24 h, T2: +48 h, T3: +72 h; T4: +96 h during induced hypothermia. All comparisons are within sub-group between T0 data and data at all other time-points (Wilcoxon test). n = 8, 10 in sub-groups A (good outcome at 6–18 months) and B (poor outcome), respectively. Hypothermia started immediately after blood sample collection at T0 and ended at T3. Error bars represent 95% CI for median. #p = 0.06, vs T0.
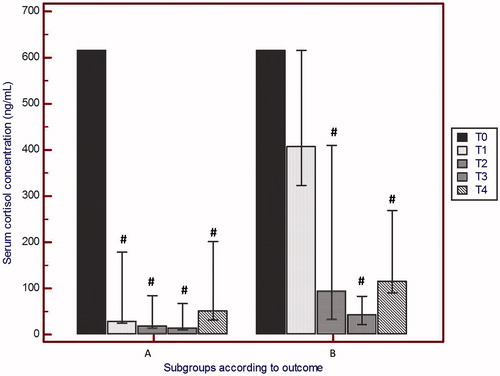
Table 1. Statistical comparisons between subgroups for serum cortisol concentration values at each time-point (T0: admission, <6 h after birth; T1: +24 h, T2: +48 h, T3: +72 h; T4: +96 h) during induced hypothermia (started after blood sample collection at T0 and ended at T3; Mann–Whitney test).
At T4, i.e. 24 h after rewarming, serum cortisol concentration was slightly, but not significantly, increased in both groups. No correlation (Spearman’s coefficient) was found between cortisol concentrations and the time of starting hypothermia.
Discussion
We interpret our findings of increased serum cortisol concentrations, compared with healthy controls, in asphyxiated neonates before hypothermic treatment began (at T0, within 6 h after birth), as the physiological response to the perinatal stress of asphyxia that they had experienced, so confirming previous data in humans (Procianoy et al., Citation1988). The studied cases had no identified conditions other than perinatal asphyxia that could be causes of hypercortisolemia. After 24 h of hypothermic treatment, the adrenocortical hormonal response was decreased in the group of cases overall. This could be partially due to a direct effect of the hypothermia on adrenal metabolism, or an indication of reduced stimulation of the hypothalamo–pituitary–adrenal axis as a result of the therapeutic effect of the hypothermia. Indeed, a statistically significant earlier reduction in serum cortisol concentrations (at T1, after hypothermia for 24 h) was observed in the infants with good neurological outcome (subgroup A), supporting this second interpretation. These infants with a good neurodevelopmental outcome had serum cortisol concentrations at least three-fold lower than birth-levels after treatment with hypothermia for 24 h.
In contrast, the neonates with an adverse outcome (subgroup B) showed a slower recovery of cortisol levels during hypothermic treatment. In particular, serum cortisol concentration in this subgroup did not show a rapid recovery of cortisol concentration at 24 h after the start of hypothermia. At the end of hypothermic treatment, in all cases, serum cortisol concentrations were similar between the subgroups and in the normal range (i.e. 10–240 ng/mL) (Mendicini, Citation2003).
To the best of our knowledge, similar results concerning cortisol in humans or in animals in relation to outcome from perinatal asphyxia and hypothermic treatment have not been previously reported. Others have focused recently on the inflammatory response that follows perinatal asphyxia and its contribution to cerebral injury. Therapeutic hypothermia has been reported to be able to rapidly suppress (i.e. within 6–12 h) production of pro-inflammatory cytokines, such as interleukin (IL)-6 and IL-4 (Róka et al., Citation2013). In contrast to these findings, cortisol levels did not significantly differ between normothermic neonates and those in hypothermia, gradually decreasing in both groups (to <414 nmol/L (150 ng/mL) at 24 h post-natal), as did the anti-inflammatory cytokine IL-10 (Róka et al., Citation2013). A minor variation in cortisol levels was revealed in the hypothermia group, and at 6 and 24 h after birth, a positive correlation was observed between cortisol and IL-10 levels; the size of the study population was similar to that in this study (Róka et al., Citation2013).
Okazaki et al. (Citation2006) reported that serum concentrations of IL-6, IL-8 and IL-10 were higher in asphyxiated than in healthy neonates, especially in the asphyxiated infants who died or had poor neurological outcome. In another study, serum cytokines were measured every 12 h for 4 d in 28 hypothermic and 22 normothermic neonates with HIE and the trend in relationship with neurodevelopmental outcome at 12 months was analyzed (Jenkins et al., Citation2012). In hypothermic neonates with better outcome, uniform down modulation of IL-6, IL-8 and IL-10 from their peak levels at 24 h to their nadir at 36 h was observed; these authors concluded that inflammatory cytokines may mediate HIE and offer insights into the severity of injury and the timing of recovery (Jenkins et al., Citation2012). Hence, modulation of cytokine production or actions may be another approach for improved outcome in neonates treated by hypothermia.
Although only cortisol was evaluated in this study, our data can be considered as consistent with accumulating evidence for an important role for inflammation in HIE. Based on differences in the pattern of changes in serum cortisol concentrations in our patients, we speculate that hypothermia may not only reduce the so-called secondary or delayed neuronal injury (due to glutamate-induced excito-toxicity and calcium overload) but may also, through early reduction of cortisol secretion, reduce the inflammation cascade and shorten the time to initiate repair in neonates recovering from HIE. Further and larger studies on biomarkers of injury and recovery are required to predict outcome and so modulate therapies.
Conclusions
In sum, our results show that serum cortisol concentration at 24 h after inducing hypothermia is associated with neurological outcome of newborns undergoing this therapy for perinatal asphyxia. Such an early biomarker may be useful to clinicians, in order to optimize intensive care management and to aid prognosis. Future studies are needed on a larger population to test interactions between cortisol and inflammatory mechanisms.
Declaration of interest
Authors have no conflicts of interest to disclose.
References
- Bayley N. (2006). Bayley scales of infant and toddler development. 3rd ed. San Antonio, TX: Harcourt Assessment Inc
- Bhutta ZA, Black RE. (2013). Global maternal, newborn and child health – so near and yet so far. N Engl J Med 369:2226–35
- Gardner DS, Fletcher AJ, Fowden AL, Giussani DA. (2001). Plasma adrenocorticotropin and cortisol concentrations during acute hypoxemia after a reversible period of adverse intrauterine conditions in the ovine fetus during late gestation. Endocrinology 142(2):589–98
- Gröschl M, Rauh M, Dörr HG. (2003). Circadian rhythm of salivary cortisol, 17-α-hydroxyprogesterone, and progesterone in healthy children. Clin Chem 49:1688–91
- Harris TA, Healy GN, Colditz PB, Lingwood BE. (2009). Associations between serum cortisol, cardiovascular function and neurological outcome following acute global hypoxia in the newborn piglet. Stress 12(4):294–304
- Hernandez-Andrade E, Hellström-Westas L, Thorngren-Jerneck K, Jansson T, Liuba K, Lingman G, Marsál K, et al. (2005). Perinatal adaptive response of the adrenal and carotid blood flow in sheep foetuses subjected to total cord occlusion. J Matern Fetal Neonatal Med 17(2):101–9
- Jacobs SE, Berg M, Hunt R, Tarnow-Mordi WO, Inder TE, Davis PG. (2013). Cooling for newborns with hypoxic ischaemic encephalopathy. Cochrane Database Syst Rev 1:CD003311
- Jenkins DD, Rollins LG, Perkel JK, Wagner CL, Katikaneni LP, Bass WT, Kaufman DA, et al. (2012). Serum cytokines in a clinical trial of hypothermia for neonatal hypoxic-ischemic encephalopathy. J Cerebr Blood Flow Metab 32:1888–96
- Jensen A, Garnier Y, Berger R. (1999). Dynamics of fetal circulatory responses to hypoxia and asphyxia. Eur J Obstet Gynecol Reprod Biol 84(2):155–72
- Kerenyi A, Kelen D, Faulkner SD, Bainbridge A, Chandrasekaran M, Cady EB, Golay X, Robertson NJ. (2012). Systemic effects of whole-body cooling to 35 °C, 33.5 °C, and 30 °C in a piglet model of perinatal asphyxia: implications for therapeutic hypothermia. Ped Res 71(5):573–82
- Kohno H, Furuhashi N, Fukaya T, Shinkawa O, Tachibana Y, Suzuki M. (1984). Serum cortisol levels in maternal venous, umbilical arterial and umbilical venous blood at delivery in the cases of fetal distress. Tohoku J Exp Med 144(3):299–304
- Mendicini M. (2003). Appendice II in Neonatologia. Rome, Italy: Verduci Editore
- Okazaki K, Nishida A, Kato M, Kozawa K, Uga N, Kimura H. (2006). Elevation of cytokine concentrations in asphyxiated neonates. Biol Neonate 89(3):183–9
- Procianoy RS, Giacomini CB, Oliveira ML. (1988). Fetal and neonatal cortical adrenal function in birth asphyxia. Acta Paediatr Scand 77(5):671–4
- Róka A, Bekö G, Halász J, Toldi G, Lakatos P, Azzopardi D, Tulussay T, Szabó M. (2013). Changes in serum cytokines and cortisol levels in normothermic and hypothermic term neonates after perinatal asphyxia. Inflamm Res 62(1):81–7
- Sarnat HB, Sarnat MS. (1976). Neonatal encephalopathy following fetal distress. A clinical and electroencephalographic study. Arch Neurol 33(10):696–705