Abstract
Although stress is usually associated with disease, the physiological and behavioral responses to stressors are critical mechanisms of resilience for healthy organisms. A recent workshop comprised of researchers who study healthy humans and both free-living and captive non-human animals identified a number of key roadblocks that are impeding progress in understanding how stress responses integrate into the normal physiology of an animal. These include the lack of: (1) an unambiguous definition of a stress phenotype; (2) a robust biomarker, or suite of biomarkers, to indicate that phenotype; (3) theoretical and quantitative models to predict how humans and other animals will react to stressors; (4) a comprehensive understanding of how individual variability in stress responses arise and (5) an understanding of the transitions between acute and chronic stress responses. Collectively, these deficiencies impair our ability to both assess the physiological status of individuals and develop procedures and techniques to reverse the effects elicited by chronic stress before they become pathological. Workshop participants also identified a number of potential approaches to facilitate progress on these problems. They include: (1) increased use of mathematical models to provide quantitative predictions; (2) use of network theory to expose emergent properties not predicted from traditional approaches; (3) development and deployment of improved sensor technology that will allow long-term, dynamic, non-invasive, multi-factor measurements of suites of stress mediators and (4) the recruitment of scientists with diverse skill sets, such as engineers, bioinformaticians, etc.; and (5) the training of young scientists in the multidisciplinary study of stress. Incorporating these approaches in new research should reinvigorate the study of stress and stimulate progress in understanding both how healthy humans cope with stressors and how other animals, including free-living animals, cope with stressors in a rapidly changing environment.
Introduction
Evolution of life on Earth has been shaped by stress and stress is something that we as humans live with and hear about on a daily basis. However, despite years of research on its impact on all living things, including human health and performance, stress remains a problematic concept in biology – problematic not only because many things of both abiotic and biotic origin can act as stressors, but also because stress responses are multifaceted and multidimensional (Fischer & Agnew, Citation1955). In the simplest terms, these problems can be seen in the fact that acute, short-term responses to a stressor are generally, but are not always, beneficial, increasing survival and health. Conversely, long-term chronic stress is frequently, but not always, associated with significant detrimental effects. Further complicating a clear understanding of stress is that stress responses are context-dependent and may reflect differences in the environment, timing (e.g. time of day, season, life-history stage), history of previous stressors, social setting and huge among-individual variation (Crespi et al., Citation2013). Any of these variables may play a critical, though not yet understood, role in why a major stressor may have little or no long-term consequences for one individual while in another it has profound deleterious effects.
Research programs world-wide are studying various aspects of stress biology, reflecting the many scales of biological response to stress: molecular, cellular and neural regulation; physiological and hormonal controls; as well as differences at the social-, community-, population-and ecosystem-levels. Although, these and thousands of other studies have provided useful theoretical and mechanistic constructs that continue to drive discovery (McEwen & Wingfield, Citation2003, Citation2010; Romero et al., Citation2009), what is missing in the overall field of stress biology is an integrated theory and associated models to enable quantitative and predictive research connecting the multiple mechanisms and outcomes. One of the major paradigms of scientific research has been reductionism, i.e. reducing and controlling variability. However, there is a need to integrate and incorporate complexity, building up and creating new holistic perspectives, as an alternative and perhaps essential approach to understand the causality, expression, and in some cases eventual treatment, of stress-related disease. This essential paradigm shift also facilitates the creation and testing of predictive models applicable to how individuals, groups, populations and species respond to both short- and long-term stressors.
This paper reflects the results of a workshop held in December 2013 that had three primary goals: (1) to clarify why progress has been slow in understanding how to integrate the physiology of stress responses across biological scales (i.e. from molecules to populations); (2) to identify the roadblocks to progress in this integration and (3) to propose potential fruitful avenues of research to overcome these roadblocks. The workshop brought together a broad assemblage of researchers interested in various facets of the stress responses in healthy individuals, including both human and non-human vertebrates. The focus was not to consider stress as a cause of disease or as a result of other underlying diseases. Many of the current major foci for stress research in biomedicine, including depression, obesity and drug addiction, have limited relevance for healthy animals. Rather, the focus was to consider stress responses as adaptive, integrated and normal physiological responses essential for the health and survival of the animal.
The problem
A major roadblock to developing a successful integrative understanding of stress is that most such research is carried out at a single biological scale (); whether and how such phenomena intersect with processes at other scales is rarely studied. For example, many researchers preferentially focus on physiological levels of control, such as homeostasis and allostasis (the concept of maintaining homeostasis through changes in the underlying regulatory systems (McEwen & Wingfield, Citation2003; Sterling & Eyer, Citation1988)), including allostatic load (how hard the individual must work to maintain homeostasis) and overload (when allostatic load is so great that homeostasis starts to break down). Studies of homeostasis tend to focus on error–correction by regulatory feedback from the organ and cellular levels, whereas investigations of allostasis focus on the neuroendocrine and glucocorticoid responses, suggesting anticipation of regulatory needs (Sterling, Citation2012). The emphasis on anticipation provides a broader framework for considering differential responses to perturbations (predictable versus unpredictable, or chronic versus acute), including variation in frequency and intensity of the responses and the dynamic role of feedback from target tissues to the brain/endocrine system.
Figure 1. Different scales at which stress research is conducted, with the areas of research that focus on those scales.
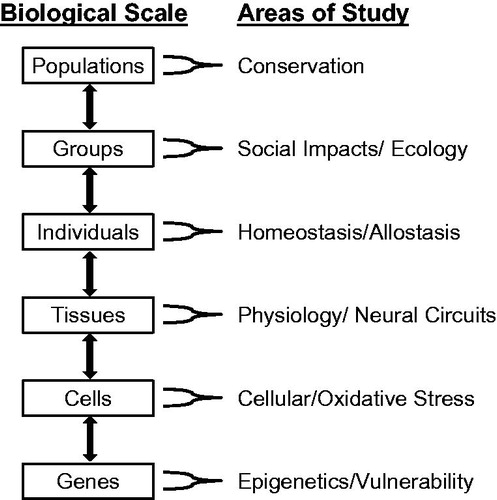
Understanding such physiological effects in the context of underlying cellular responses is often difficult, because few studies measure both physiological and cellular outputs, such as stress-associated proteins or oxidative stress mediators (e.g. Currie et al., Citation2010; Du et al., Citation2009; Tomiyama et al., Citation2012). Furthermore, to understand the actual expression or conceptualization (and even evolution) of the stress response in human beings and most other animals, it needs to be studied within the context of relevant natural stressors. Social stressors are prime examples – they require another level of analysis with indirect and/or population effects on individual stress responses (Creel et al., Citation2013). A further level of analysis includes measuring macrosystems-level and biogeographic-scale outcomes whereby individual responses can lead to species-level responses to global change and thus impact their ability to adapt or be driven to extinction (Jessop et al., Citation2013). Unfortunately, no model of stress response currently combines or comes close to addressing all of these levels of analysis.
Furthermore, there has been an over-reliance upon the measurement of corticosteroids for diagnosing stress. Other aspects of the stress response, such as the catecholamine regulation of the fight-or-flight response (Cannon, Citation1932), are known but understudied. It is unclear why the corticosteroids became the biomarker of most interest, although development of radioimmunoassays allowing their ready measurement from blood plasma is clearly part of the reason. In addition, for decades there have been three major assumptions guiding studies of the corticosteroid aspects of stress in an ecological context. First, variation in corticosteroid titers among unstressed individuals is presumed to correlate with Darwinian fitness. Unfortunately, the evidence for this is mixed at best (Bonier et al., Citation2009). Some studies indicate that higher titers correlate with fitness measures, some studies indicate the reverse, and other studies show no correlation. Second, acute corticosteroid release is presumed to increase fitness (i.e. a corticosteroid response helps survival). The evidence for this assumption is also very weak – different studies show divergent correlations (Breuner et al., Citation2008). Third, chronic stress is presumed to result in sustained elevated corticosteroid titers that decrease fitness. However, a recent review failed to find current support for this assumption (Dickens & Romero, Citation2013). Once again, the literature indicates that chronic stress can result in increased, decreased or no change in corticosteroid titers, with little indication of how to predict which response an individual will produce. The tenuous state of these three long-held assumptions has cast doubt on much of our understanding of the physiological and ecological roles of corticosteroid-mediated stress responses.
Although, considerable progress is being made in understanding how stress impacts healthy animals, including healthy humans, the above problems are impeding further progress. The workshop participants identified a number of unsolved questions and problems that, if addressed, will make major contributions to the advancement of the field of stress biology. These questions and problems are both theoretical and technical in nature.
Where progress is needed
(1) Perhaps the most difficult unresolved issue facing researchers is rigorously defining the stress phenotype (). This problem has been recognized for many years, even though it has been a major target of enquiry (Levine, Citation2005; Levine & Ursin, Citation1991). A universal and quantitative definition of the stress phenotype that is applicable across diverse taxa is a prerequisite for in depth and comparative studies. Part of creating a new definition will require rethinking the concepts of acute and chronic stress, both of which are poorly defined, thereby hindering understanding. What is chronic stress (i.e. the chronic stress phenotype)? Where is the transition between acute and chronic stress? How do we determine the point at which the transition occurs, or put in a different context, how do we know whether an animal has crossed into allostatic overload? What are the interactions between multiple, simultaneous stressors (Fischer & Agnew, Citation1955)? Furthermore, there is a bias in much of the stress literature to define specific stress responses as “maladaptive” or “disease-like” phenotypes, even though many such responses may be highly adaptive under specific experimental conditions and thus not reflect a pathological state. Creating an inclusive definition of a stress phenotype may be the most pressing problem in the field.
Figure 2. Key components of a stress response (in squares) with the major unresolved questions concerning those components (circles). See text for further details.
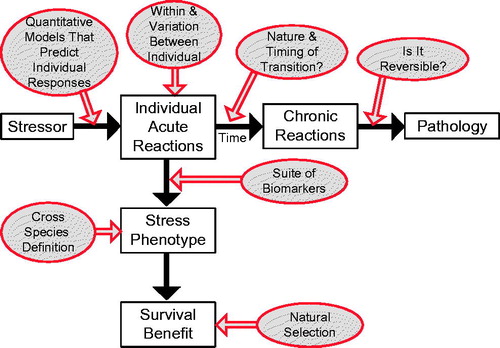
As discussed earlier, previous work on wildlife species relies heavily on using glucocorticoids to define the stress phenotype. However, it is becoming clear that relying on glucocorticoids to define stress is incomplete, and there is no current consensus that glucocorticoids should serve as the primary biomarker for defining the stress phenotype. Glucocorticoids clearly can be a valuable indicator of an organism under stress, but they are only one physiological biomarker that needs to be integrated with multiple other such markers in developing a holistic characterization of the stress phenotype. Major future progress will be unlikely until a new consensus is created for a better way to define the stress phenotype. One important aspect of any new definition would be the characterization of a single, or more likely a multifactorial, cross-species biomarker that can identify a change (or risk of change) in allostatic load (). As with corticosteroids, it is unlikely that any one variable will be satisfactory in informing or predicting a transition to allostatic overload (i.e. the onset of stress-related disease) for a given species at a given point in their life history. Thus, quite likely a constellation of variables reflecting multiple physiological systems will likely be required. Unfortunately, it is currently unknown which variables are most sensitive, informative and robust. Moreover, this constellation will need to be measured dynamically because it is likely the pattern, or rate of change, of a group of biomarkers that will provide the best indicator of an organism approaching or entering allostatic overload. These suites of biomarkers could perhaps act as predictors of when an animal is about to shift into overload.
A potential alternative approach for better defining a stress phenotype is to dispense with the concept of a stress phenotype altogether. An overarching definition might be too complex or too simplified and broad to be useful. Instead, perhaps splitting the concept of “stress” into a suite of terms that are specific to conditions or situations would provide better clarity for future paths of research.
(2) Once we agree on a definition of a stress phenotype, or whether such a concept has merit, other important questions can then be addressed. These questions include: What is the temporal component of the stress phenotype and susceptibility to stress (e.g. does the stress phenotype change across life-history stages, across the day or over the course of the lifespan, and if so, why)? Why are some phenotypes more vulnerable to the same stressor than others (i.e. Why are some individuals more likely to progress to stress-related disease than other individuals?), and is this difference among individuals predictable (i.e. what underlies the individual variation in the consequences of stress responses)? How long must the suite of measures be at a certain level before we define the animal as exhibiting a stress phenotype? How does natural selection act on the stress phenotype? These are important questions for understanding how the stress response functions in healthy animals, but they have proven very difficult to address and most proposed answers remain unsatisfying. For example, many free-living species vary corticosteroid concentrations seasonally, but current theory does not provide guidance on which of the three proposed hypothesis is likely to be strongest (Romero, Citation2002). We anticipate that a more-robust definition of a stress phenotype will facilitate great progress in addressing these questions.
(3) Another difficult unresolved question is how to integrate stress research across multiple biological scales (). Currently, stress research tends to be Balkanized. For example, studies on topics, such as oxidative stress (Vitale et al., Citation2013), neural circuitry (Herman, Citation2013) and stress ecology (Wingfield, Citation2013), rarely address stress at other levels of biological complexity. Integration across different scales, coupled with focused investigations of the healthy animal, will facilitate development of new conceptual models of stress that encompass and integrate conditional and individual responses. Such a model could result in more quantitative, predictive research linking cellular and physiological stress responses with individual-, population- and species-level outcomes. Most research currently uses one of four major conceptual models to guide research and interpret results. The first model is derived from several decades of biomedical research and emphasizes the controllability and predictability of stressors (Koolhaas et al., Citation2011). The primary emphasis of this traditional model is on the nature of stressors and the responses to those stressors. The second model derives from a synthesis of developmental psychology, traditional biomedical studies and evolutionary theory (Del Giudice et al., Citation2011; Ellis et al., Citation2011). This model emphasizes the match and/or mismatch between early childhood and adult environmental conditions to explain individual variation in stress responses and the ability to cope with stressors. The third model is a synthesis of biomedical and ecological studies emphasizing allostasis, allostatic load and allostatic overload (McEwen & Wingfield, Citation2003). The primary emphasis of this model is on the cumulative impact of stressors on the individual. A key metric is the energy requirements needed to cope with stressors, within the context of the individual’s overall energy budget. The fourth model is a modification of the concept of allostasis that emphasizes the reactive scope of an individual’s mediators of stress (Romero et al., Citation2009). This model emphasizes the capacity of the underlying physiological mechanisms to adequately cope with stressors. Work is desperately needed to test these models, as well as creating new, perhaps better, conceptual models. For example, the concept of allostatic load has become increasingly useful, but we still lack a firm understanding of how to measure it. Progress on this front has been made in humans by measuring multiple factors, such as cortisol, epinephrine, waist-hip ratio and blood pressure (Seeman et al., Citation1997). This suite of factors appears to provide a useful index of the cumulative impact of multiple stressors over time (i.e. allostatic load), but it is not always clear how to extrapolate these measures to non-human animals. For example, what is the equivalent of a waist–hip ratio of a snake? Resolving these problems will provide considerable progress to creating a unified conceptual model of stress.
(4) A third major problem facing stress researchers is a lack of understanding of the role of individual variation in reactions to stress (). Notwithstanding the discovery three decades ago of the profound impact of maternal effects on an offspring’s glucocorticoid responses to stress (Liu et al., Citation1997; Meaney, Citation2001), we still have only a rudimentary idea of how natural individual variation is created and maintained. For example, are epigenetic mechanisms for regulating transgenerational effects of glucocorticoids (Weaver et al., Citation2004) conserved across taxa? Are there similar maternal effects on other stress response systems, and if so, are they regulated via similar mechanisms? How do individual differences in our microbial symbiont community influence stress responses? At a fundamental level, we still lack a firm understanding of either the relative roles of genetics and environmental experience in shaping the individual variation in stress responses, or whether natural selection shapes the stress response by acting on specific regulatory elements of gene expression, the whole regulatory axis, function-physiology links, such as hormone titers, or all the above. Gaining an understanding of these fundamental questions is critical in order for us to predict when the stress response will be activated and its consequences, and so that we can make sense of the relationship between stress responses and fitness.
The intergenerational effects discussed above affect both basal and stress-induced responses of many physiological systems. It is the accumulation and/or constant activation of stress-induced responses that is thought to result in chronic stress, but it remains unclear whether individual variation in response to acute stressors has any correlation to variation in response to a chronic stressor. Even more fundamental, we still lack an understanding of the source of variability in how an individual copes with acute and chronic stress, or whether there is variability in how healthy animals habituate to stressors. The concept of habituation itself is plagued by conflicting definitions (Cyr & Romero, Citation2009) and, despite substantial effort, we still lack a clear mechanistic understanding of how stress is perceived by the brain and how animals become unresponsive to a previously perceived stressor. Addressing these questions is of increasing practical importance in a number of contexts. For example, it would be very useful to be able to screen healthy, human astronaut candidates for resistance to acute stressors if that resistance was predictive of their resistance to the chronic stressors encountered during space flight. Similarly, conservation biology would benefit from being able to predict how free-living animals will respond to long-term anthropogenic stressors by how they respond to acute stressors. Unfortunately, we still lack the ability to make these predictions.
(5) Finally, although there has been considerable work in understanding how chronic stress can alter the long-term health of animals, very little research has tried to determine whether these changes due to stress are reversible (). The lack of work is perhaps not surprising. This type of research has been greatly hindered by a lack of a well-defined stress phenotype and a robust theoretical context, both of which would be required to judge whether stress-induced changes had returned to “normal”. However, once sufficient progress has been made on the other four major problems discussed above, perhaps the field will be ripe for finally addressing this question. Successful techniques to reverse stress-induced changes will clearly have profound effects on our treatment of stress-related disease, but will also give us insight into how stress is impacting healthy individuals as well.
Proposed approaches
The workshop participants identified a number of technological and theoretical approaches that might lead to breakthroughs in addressing the above problems ().
Table 1. Major approaches likely to foster progress in solving unresolved questions from .
(1) The first approach to increase our understanding of stress in the healthy animal is the adoption of mathematical modeling. There have been few attempts to use mathematical modeling to understand the physiology of stress responses (some examples include mathematical analysis of heart rate variability to predict stress impacts on cardiac function (Acharya et al., Citation2006) and use of individual variation in allostatic load to predict changes in population numbers using an individual-based modeling approach (Fefferman & Romero, Citation2013)) even though these types of models have been very useful in other fields such as ecology. However, the current era of “big data” suggests that using mathematical models to integrate data from increasing numbers of physiological systems, and especially to integrate data across different levels of biological complexity, will be very fruitful. Bottom-up models can use algorithms to link interactions among many different physiological/gene expression factors to characterize healthy networks and to predict the risk of allostatic overload. Top-down models can convert the concepts and relationships of allostatic load or reactive scope to predict changes in population- or organismal-level functions in response to environmental change. For example, integrating responses to stress across the life history in matrix demographic models can potentially: (1) predict impacts on population size; (2) identify sensitive life-history stages in which stress effects on survivorship may be more important; (3) be used to redirect the study of stress in species of conservation concern and (4) be used to model effects of climate change on condition, survival or fecundity (see Crespi et al., Citation2013). Furthermore, using mathematical approaches may be necessary for both understanding the variance and shape of the distribution of stress phenotypes and for deriving a new multifactorial index that incorporates a suite of stress-associated biomarkers.
(2) A related approach would be to increase focus on network structure. Stress may be an emergent property, making reductionism a poor approach. A potential solution would be to focus on these emergent properties by studying, for example, neural networks, community ecology, gene network theory or social networks related to stress. Network analysis has the potential to identify important links between different physiological systems (Cohen et al., Citation2012; Martin et al., Citation2011). For example, exploiting new genomic approaches would likely find numerous commonalities and differences of origin in the downstream effects that are commonly measured (e.g. hormone secretion, cardiac function, overt changes in morphology, etc.). Finding connections among many different responses to a stressor, rather than focusing on a single or a few responses as is done in most studies, might be far more productive for generating an integrated understanding of a holistic stress response.
(3) One critical approach to addressing many of the challenges described above is development and use of better physiological sensors. In some ways it is astounding that most research on stress still relies upon collecting a blood sample. The problematic nature of studying stress responses while inducing a stress response via venipuncture has been recognized for many years. In addition, single blood samples are inherently problematic because they only provide a snapshot of a dynamically changing system. There have been some attempts to collect data in non-invasive ways, such as using transmitters to study sympathetic responses (Cyr et al., Citation2009) and collecting fecal and hair samples to ascertain glucocorticoid responses (Sheriff et al., Citation2011), but because each of these techniques only indirectly reflects changes associated with stress, their utility and interpretation can be limited. Furthermore, these techniques still collect data from only one, or at most a few, physiological systems. What is needed are technological advances to allow collection of data from many physiological systems in a systematic, repeatable (ideally continuous), and minimally-invasive, manner. We can already monitor some physiological variables over these time frames that may be useful, such as body temperature, heart rate and sleep, but continuous long-term measurement of many variables has not been attempted. The workshop participants envisioned an implantable chip that could continuously sample a suite of physiological variables, such as epinephrine and glucocorticoid titers, receptor numbers and/or occupancy, various metabolites including circadian regulators, blood glucose and pH, etc. over days, weeks or longer. The specific suite of monitored variables would likely depend upon progress in metabolomics and proteomics. Furthermore, to make such a device useful for field studies, progress will need to be made in transmitting the collected information over extended distances while keeping the transmitter small. Such a device does not currently exist, but the technology to build one may. Once developed, such a device would have the potential to revolutionize the study of stress, and perhaps the study of physiology in general.
In addition, a battery of individual laboratory tests that could be used along with the new sensing technology would allow us to characterize inter- and intra-individual variation. A series of physical, mental, thermal, circadian and other ethical and social stressors could form the foundation for an algorithm to determine allostatic load. This series could then be used to track allostatic load over the life span, through different life-history transitions, and potentially as an aid to modulate allostatic load to improve health. It seems likely, however, that such a series of tests will first require solving the puzzle of determining the stress phenotype discussed above.
(4) Perhaps the most important need identified by the workshop participants was the collaboration of scientists beyond physiologists interested in stress. The approaches proposed above will only be possible through extensive collaboration with engineers, mathematicians, computer scientists, bioinformatics specialists, etc. For example, we will need engineers to create the next generation of sensing devices and mathematicians and bioinformaticists to create the mathematical models discussed above and to analyze and integrate the resulting data. As with many other areas of biology, progress is becoming more and more reliant on a breadth of expertise that is impossible for single investigators. The study of stress has likely reached this point as well. What is lacking, however, is a research network and funding that can sustain this level of collaboration and intellectual manpower. A major problem is that there is currently little compelling reason for those engineers, mathematicians and other researchers to forgo discipline-specific research interests to join in a collaboration to understand stress. What is likely needed is a center, institute or web-based community dedicated to research on stress in the healthy animal that will provide the financial and intellectual incentives to bring the necessary expertise together to focus on solving the problems identified above. Hopefully, engineers would be both intrigued and enticed to tackle the challenge of development of brand new sensor technology, low power-consumption and rugged constructs with affordable and non-toxic materials. Similarly, one would hope that mathematicians would be drawn to challenging problems that will require mathematical innovation to integrate very different types of physiological data generated from different levels of biological complexity. Sharing data across these disciplines should help in developing a better theoretical conceptualization of stress and stress phenotypes.
(5) Related to the need for diverse expertise in solving problems in stress research is the training of the next generation of scientists. To accelerate progress, future training and education should emphasize the integration of different fields and the cross-fertilization of different approaches in a single program. Mathematical training and a focus on nonlinear thinking should be of special emphasis. Again, a center, institute, or web-based community would provide an ideal focus for such training.
The future
Progress in addressing the roadblocks and approaches discussed above will have a number of broader impacts. The multi-scale integration of research approaches and analyses will better inform our conceptualization and understanding of biological principles, and lead to novel insights. One reason that progress on stress research is needed is that the rapid pace of discovery and creation of engineered devices will greatly change functionality in humans. For example, brain-controlled prosthetics and artificial pancreases have the potential to change our very definition of disease. Understanding stress in the healthy animal will be critical in providing guidance on what kinds of functionality is desired. The stress response is an adaptive system for coping with the environment. Considering the stress response as only resulting in disease will greatly hinder progress in defining what is a healthy and well-adjusted animal (human or non-human). For example, one of the areas of human stress research that focuses on healthy individuals is spaceflight. The spaceflight environment provides unique opportunities to study the psychological, cognitive and biological responses to stress from the molecular level up to the level of a small community. Some stressors are unique to spaceflight (e.g. weightlessness, radiation) while others are close to extremes seen terrestrially (e.g. atmospheric composition; isolation and confinement). A poor understanding of the biological responses to stressors in healthy humans makes mitigating ill effects in long duration space exploration missions problematic.
One of the corollary benefits for producing a better conceptual model of stress is that we can start to define what makes a healthy individual. Until recently, a healthy individual was usually defined as a lack of something – in humans and domesticated animals an absence of overt disease, physical or mental; in free-living animals, mere survival coupled with a lack of infection. More recently, there has been recognition that individuals can be vulnerable to disease, even if sickness is not manifested. For example, individuals could be healthy and robust, or healthy yet frail. Many “sub-clinical” markers of future disease have been identified in humans. The current focus on “prediabetes” or “metabolic syndrome” illustrates this point. If this vulnerability and prediction of responses to future stressors could be incorporated into conceptual models of stress, a better concept of health and disease might arise. An ability to predict vulnerability will be very useful to fields as diverse as human health and conservation biology.
Conclusion
Stress is an enormously important concept in modern biology and medicine. The focus on stress as a disease, however, has hindered progress in understanding how stress responses fit into the normal physiology of a healthy individual. The workshop participants identified that if we can (1) better define stress phenotype using multiple indicators of stress, (2) utilize a new sensor that measure changes in such indicators continuously and invasively and (3) turn those data to mathematical model describing the relationship among them that can be used to predict vulnerability of the species to climate change, then we should greatly accelerate progress in understanding the normal and adaptive physiology of stress.
Acknowledgements
We thank all of the attendees at the workshop.
Declaration of interest
The workshop was funded by NSF IOS-1403615 to LMR and funding from NASA, but neither organization had any role in the writing or the decision to submit the article for publication. The authors report no conflicts of interest.
References
- Acharya UR, Joseph KP, Kannathal N, Lim CM, Suri JS. (2006). Heart rate variability: a review. Med Biol Eng Comp 44:1031–51
- Bonier F, Martin PR, Moore IT, Wingfield JC. (2009). Do baseline glucocorticoids predict fitness? Trends Ecol Evol 24:634–42
- Breuner CW, Patterson SH, Hahn TP. (2008). In search of relationships between the acute adrenocortical response and fitness. Gen Comp Endocrinol 157:288–95
- Cannon WB. (1932). The wisdom of the body. New York: W.W. Norton & Company, Inc
- Cohen AA, Martin LB, Wingfield JC, McWilliams SR, Dunne JA. (2012). Physiological regulatory networks: ecological roles and evolutionary constraints. Trends Ecol Evol 27:428–35
- Creel S, Dantzer B, Goymann W, Rubenstein DR. (2013). The ecology of stress: effects of the social environment. Func Ecol 27:66–80
- Crespi EJ, Williams TD, Jessop TS, Delehanty B, Boonstra R. (2013). Life history and the ecology of stress: how do glucocorticoid hormones influence life-history variation in animals? Func Ecol 27:93–106
- Currie S, LeBlanc S, Watters MA, Gilmour KM. (2010). Agonistic encounters and cellular angst: social interactions induce heat shock proteins in juvenile salmonid fish. Proc R Soc Lond B 277:905–13
- Cyr NE, Dickens MJ, Romero LM. (2009). Heart rate and heart rate variability responses to acute and chronic stress in a wild-caught passerine bird. Physiol Biochem Zool 82:332–44
- Cyr NE, Romero LM. (2009). Identifying hormonal habituation in field studies of stress. Gen Comp Endocrinol 161:295–303
- Del Giudice M, Ellis BJ, Shirtcliff EA. (2011). The Adaptive Calibration Model of stress responsivity. Neurosci Biobehav Rev 35:1562–92
- Dickens MJ, Romero LM. (2013). A consensus endocrine profile for chronically stressed wild animals does not exist. Gen Comp Endocrinol 191:177–89
- Du J, Wang Y, Hunter R, Wei YL, Blumenthal R, Falke C, Khairova R, et al. (2009). Dynamic regulation of mitochondrial function by glucocorticoids. Proc Natl Acad Sci USA 106:3543–8
- Ellis BJ, Boyce WT, Belsky J, Bakermans-Kranenburg MJ, van Ijzendoorn MH. (2011). Differential susceptibility to the environment: an evolutionary-neurodevelopmental theory. Develop Psychopathol 23:7–28
- Fefferman NH, Romero LM. (2013). Can physiological stress alter population persistence? A model with conservation implications. Conserv Physiol 1:cot012
- Fischer R, Agnew N. (1955). A hierarchy of stressors. J Mental Sci 101:383–6
- Herman JP. (2013). Neural control of chronic stress adaptation. Front Behav Neurosci 7:61
- Jessop TS, Woodford R, Symonds MRE. (2013). Macrostress: do large-scale ecological patterns exist in the glucocorticoid stress response of vertebrates? Func Ecol 27:120–30
- Koolhaas JM, Bartolomucci A, Buwalda B, de Boer SF, Flugge G, Korte SM, Meerlo P, et al. (2011). Stress revisited: a critical evaluation of the stress concept. Neurosci Biobehav Rev 35:1291–301
- Levine S. (2005). Stress: an historical perspective. In: Steckler T, Kalin N, Reul JMHM, editors. Handbook of stress and the brain. Amsterdam, The Netherlands: Elsevier. p 3–23
- Levine S, Ursin H. (1991). What is stress? In: Brown MR, Koob GF, Rivier C, editors. Stress: neurobiology and neuroendocrinology. New York: Marcel Dekker, Inc. p 3–21
- Liu D, Diorio J, Tannenbaum B, Caldji C, Francis D, Freedman A, Sharma S, et al. (1997). Maternal care, hippocampal glucocorticoid receptors, and hypothalamic–pituitary–adrenal responses to stress. Science 277:1659–62
- Martin LB, Liebl AL, Trotter JH, Richards CL, McCoy K, McCoy MW. (2011). Integrator networks: illuminating the black box linking genotype and phenotype. Integ Comp Biol 51:514–27
- McEwen BS, Wingfield JC. (2003). The concept of allostasis in biology and biomedicine. Horm Behav 43:2–15
- McEwen BS, Wingfield JC. (2010). What is in a name? Integrating homeostasis, allostasis and stress. Horm Behav 57:105–11
- Meaney MJ. (2001). Maternal care, gene expression, and the transmission of individual differences in stress reactivity across generations. Ann Rev Neurosci 24:1161–92
- Romero LM. (2002). Seasonal changes in plasma glucocorticoid concentrations in free-living vertebrates. Gen Comp Endocrinol 128:1–24
- Romero LM, Dickens MJ, Cyr NE. (2009). The reactive scope model – a new model integrating homeostasis, allostasis, and stress. Horm Behav 55:375–89
- Seeman TE, Singer BH, Rowe JW, Horwitz RI, McEwen BS. (1997). Price of adaptation-allostatic load and its health consequences. MacArthur studies of successful aging. Arch Internal Med 157:2259–68
- Sheriff MJ, Dantzer B, Delehanty B, Palme R, Boonstra R. (2011). Measuring stress in wildlife: techniques for quantifying glucocorticoids. Oecologia 166:869–87
- Sterling P. (2012). Allostasis: a model of predictive regulation. Physiol Behav 106:5–15
- Sterling P, Eyer J. (1988). Allostasis a new paradigm to explain arousal pathology. In: Fisher S, Reason J, editors. Handbook of life stress cognition and health. New York: John Wiley and Sons, Inc. p 629–50
- Tomiyama AJ, O'Donovan A, Lin J, Puterman E, Lazaro A, Chan J, Dhabhar FS, et al. (2012). Does cellular aging relate to patterns of allostasis? An examination of basal and stress reactive HPA axis activity and telomere length. Physiol Behav 106:40–5
- Vitale G, Salvioli S, Franceschi C. (2013). Oxidative stress and the ageing endocrine system. Nature Rev Endocrinol 9:228–40
- Weaver ICG, Cervoni N, Champagne FA, D'Alessio AC, Sharma S, Seckl JR, Dymov S, et al. (2004). Epigenetic programming by maternal behavior. Nature Neurosci 7:847–54
- Wingfield JC. (2013). Ecological processes and the ecology of stress: the impacts of abiotic environmental factors. Func Ecol 27:37–44