Abstract
In mice, previously, we showed that restraint stress reduces the number of embryo implantation sites in the endometrium. Here, we hypothesized that the uterine microenvironment is altered by restraint stress and consequently is suboptimal for embryo implantation. On embryonic day 1 (E1), 60 of 154 pregnant CD1 mice underwent restraint stress (4 h), repeated daily to E3, E5 or E7 (n = 10 mice per group). Restraint stress decreased food intake and suppressed body weight gain on E3, E5 and E7. Restraint stress decreased the actual and relative weight (percent body weight) of uterus and ovary on E5 (by 14.9%, p = 0.03; 16.1%, p = 0.004) and E7 (by 16.8%, p = 0.03; 20.0%, p = 0.01). Morphologically, restraint stress decreased relative endometrial area (by 8.94–18.8%, p = 0.003–0.021) and uterine gland area (by 30.6%, p < 0.01 on E3 and 44.5%, p < 0.01 on E5). Immunohistochemistry showed that restraint stress decreased microvessel density (by 12.9–70.5%, p < 0.01) and vascular endothelial growth factor expression (by 14.6–45.9%, p = 0.007–0.02). Restraint stress decreased by 32.4–39.8% (p = 0.002–0.01) the mean optical density ratio for proliferating cell nuclear antigen/terminal deoxynucleotidyl transferase dUTP nick end labeling. Methyl thiazolyl tetrazolium assay showed a dose-dependent decrease in proliferative activity of endometrial stromal cells (from 52 of 154 pregnant E5 control mice) incubated with H2O2 (100–1000 μM) in vitro. These findings supported the hypothesis that restraint stress negatively influences endometrial adaptive remodeling via an oxidative stress pathway, which resulted in fewer implantation sites.
Introduction
Acute stress actually improves health, whereas chronic stress causes a variety of human health issues, including anxiety, depression, cardiac disease, and obesity (Ubaldi et al., Citation2015). Maternal psychological stress has a relationship with miscarriage (Tsartsara & Johnson, Citation2006), preterm delivery (Shapiro et al., Citation2013), intrauterine growth restriction (Torche, Citation2011), and stillbirth in women (Silver & Ruiz, Citation2013). In rodents, restraint stress has been used an experimental model of prenatal stress, which triggers psychological stress and impairs pregnancy, including by implantation failure, recurrent spontaneous abortion, spontaneous preterm birth, and developmental abnormalities of fetuses (Golub et al., Citation2004; Lee et al., Citation2008). In mice, gestation lasts for 20 d when counted from the day of vaginal plug formation (embryonic (E) 1), indicating insemination, and implantation occurs on E4.5 (Lim & Wang, Citation2010). A prerequisite for implantation is synchronization between the development of blastocysts and endometrial receptivity. The uterine stromal proliferation and uterine vasculature are important in the maintenance of endometrial receptivity (Tang et al., Citation2015).
It has been demonstrated in mice that the local uterine blood flow at implantation sites is more abundant than at interimplantation sites between E4.5–E5.5 (Nakamura et al., Citation2014). The vascular endothelial growth factor (VEGF)/VEGF receptor 2 pathway plays a key role in both angiogenesis and the regulation of vasculogenesis, and it participates in the development of the early embryo as a mediator of the fetal–maternal dialog (Douglas et al., Citation2009). CD34 is a transmembrane protein expressed in hematopoietic progenitor cells, vascular endothelial cells, and embryonic fibroblasts (Wood et al., Citation1997). Restraint stress decreases the density of uterine natural killer (uNK) cells in the endometrium (Liu et al., Citation2014), and uNK cells directly or indirectly contribute to the critical process of maternal vascular remodeling during pregnancy (Matson & Caron, Citation2014). Hence, this study investigated whether the negative effect of restraint stress is exerted via impaired development of endometrial blood vessel during embryo implantation.
In our previous study, it was demonstrated that restraint stress-induced oxidative stress, with the activity of oxidative enzyme decreased and the activity of malonydialdehyde increased in the pregnant uterus (Liu et al., Citation2014). H2O2 is an oxidative product and induces cell apoptosis. Indeed, the balance between cell proliferation and death is crucial for successful embryo implantation and the maintenance of pregnancy (Correia-da-Silva et al., Citation2004). Endometrial stromal cells (ESCs) are relevant in the regulation of lymphocyte survival at the maternal–fetal interface (Blanco et al., Citation2009). Apoptosis and proliferation of ESCs are associated with the pathogenic mechanism responsible for adenomyosis (Yang et al., Citation2007).
Restraint stress reduced the number of implantation sites by 15.3% on E5 and by 26.1% on E7 in our preliminary research (Liu et al., Citation2014). Thus, the present study hypothesized that the uterine microenvironment induced by restraint stress is suboptimal for embryo implantation and development. From the perspective of endometrial adaptive remodeling, it aimed to examine the effect of restraint stress on the proliferation and apoptosis of endometrial cells, and on the development of uterine vasculature during embryo implantation in mice.
Methods
Animals
A total of 180 female CD1 mice (aged 8 weeks; Vital River Laboratory Animal Technology Co. Ltd., Beijing, China) were housed and fed ad libitum in a quiet room that was maintained at 22–24 °C with a regular 14-h light:10-h dark cycle (lights on at 07:00 h). The mice were housed in the laboratory for 1 week before the procedure to acclimate them to their surroundings with five mice per cage. Estrous mice were identified by vaginal smears and then mated with a sexually experienced male at 17:00 h. Next morning, vaginal smears were obtained from the females with vaginal plugs, and the presence of sperm confirmed microscopically. The 154 pregnant mice were divided into the two treatment groups (control and restraint stressed group) and three gestational stages (E3, E5 and E7). Sixty of the 154 pregnant mice were used for hematoxylin and eosin (H&E) staining and immunohistochemistry (n = 10 mice per treatment group, 6 treatment groups); 52 of 154 pregnant mice from the E5 control group were used for the collection of uterine stromal cells (n = 8–9 mice per in vitro H2O2-treated group, 6 treatment groups).
Restraint stress
The pregnant mice were individually placed in transparent plastic 50 mL centrifuge tubes with ventilation holes that allowed the mice to breathe freely. After each 4 h restraint stress, the mice were returned to their cages, with 3–5 mice per cage. Because the stressed mice had no access to food and water during the restraint stress period, food and water were also unavailable to the control mice for 4 h (08:00 h–12:00 h). At the end of the restraint stress period, the mice were able to take food and water ad libitum. As shown in , restraint stress began on E1 and continued daily until the mice were killed on E3, E5 or E7.
Figure 1. A timeline diagram of experiments in the present study. E1: Embryonic day 1; E3: Embryonic day 3; E5: Embryonic day 5; E7: Embryonic day 7. At the beginning, there were 154 pregnant mice: for histological and immunocytochemical evaluation of the uterus, 3 groups of 10 mice were exposed each day to restraint stress, and one group of 10 was killed on day E3, E5 and E7; similarly, a group of 10 control mice, not exposed to the stressor was killed on each of these days (60 mice in total); the remaining 52 mice were not exposed to stress and were killed on E5 to collect uteri for in vitro studies, in groups of 8–9 across 6 H2O2 doses.
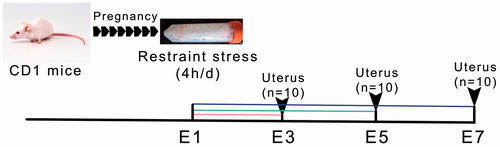
Ethical approval
The present study conformed to the Guide for the Care and Use of Laboratory Animals published by the Animal Welfare Committee of the Agricultural Research Organization, China Agricultural University (Beijing, China).
Tissue preparation
Pregnant females were anesthetized with 2% pentobarbital (4 mL/kg body weight, i.p.) and were killed by cervical dislocation on E3, E5 or E7 immediately following the restraint stress, or control period. The amount of food consumed, and the initial and final body weight were recorded. The consumed food per cage was measured (n = 8 cages per group, but E5 control group was 15 cages), and the average food intake per mouse was obtained by dividing this value by the number of mice (g/mouse/cage/day). Ovaries and uteri were weighed, immediately fixed in 4% paraformaldehyde for 48 h, and then embedded in paraffin wax for histological analysis.
Ovarian and uterine weight
Relative ovarian and uterine weight was expressed as an organ-to-body mass ratio (×100%), which indirectly reflects the development of embryos in the uterus of pregnant mice. At E3, the embryos are at the 8-cell stage and still in the oviducts near the uterus. On E5, embryos were present in the uterus, and implantation is completed.
Histology and immunohistochemistry
The uteri with implantation sites (2–3 mm in length) were routinely processed into paraffin sections for histology, immunohistochemistry, and in situ apoptosis detection. Five-micron-thick nonconsecutive transverse sections (the section interval was 25 μm) were mounted onto 10% polylysine-coated slides. To display the structure and morphology of the uterus, H&E staining was performed. The present study used the endometrial area and uterine gland cross-sectional area relative to the uterine area as an indicator of the development of implantation sites in the uterus. The data were derived from counts from five random fields from eight cross sections of the five uterine specimens from each group on E3, E5 and E7.
Sections were immunohistochemically stained for CD34 (a cell surface glycoprotein, marking vascular progenitor cells) (Wood et al., Citation1997) and VEGF to identify angiogenesis and new microvessel formation, and staining with proliferating cell nuclear antigen (PCNA) was used to visualize uterine proliferation. Briefly, all sections (5 μm) were routinely dewaxed in xylene and rehydrated through graded concentrations of ethanol. All slides were subjected to antigen retrieval by means of a 0.01 M sodium citrate–hydrochloric acid buffer. Tissue sections were washed 3 times with phosphate-buffered saline (PBS, pH7.0) for 5 min each. Endogenous peroxidase activity was blocked with 3% H2O2 for 30 min. Nonspecific staining was blocked with 5% normal goat serum for 30 min (for CD34 and VEGF) or 5% normal donkey serum for 30 min (for PCNA). The sections were incubated at 4 °C with 1:300 rabbit antimouse CD34 polyclonal antibody (Boster Biological Technology, Ltd., Wuhan, China), or with 1:300 rabbit antimouse VEGF polyclonal antibody (Boster Biological Technology, Ltd., Wuhan, China), or with 1:4000 mouse antihuman PCNA monoclonal antibody (Sigma-Aldrich, St. Louis., MO) overnight. The next morning, the slides were washed with PBS and incubated sequentially with 1:400 biotin-conjugated goat antirabbit IgG (Beijing ComWin Biotech Co., Ltd, Beijing, China) (CD34 and VEGF), or with 1:400 biotin-conjugated donkey antimouse IgG (Beijing ComWin Biotech Co., Ltd, Beijing, China) (for PCNA) for 2 h. The slides were washed with PBS and incubated sequentially with 1:400 horseradish peroxidase (HRP)-streptavidin (Beijing ComWin Biotech Co., Ltd, Beijing, China) for 2 h. The immunoreactivity was revealed by diaminobenzidine (DAB) treatment, and nuclei were counterstained with hematoxylin for 30 s. The sections incubated with PBS instead of primary antibody served as negative controls. Images were acquired using an upright DP72 microscope (Olympus, Tokyo, Japan). The mean optical density (MOD, integrated optical density per area (mm2)) was measured using an image analysis system (Image Pro Plus 6.0) and was derived from counts from five random fields from eight cross sections of the five uterine specimens from each group on E3, E5 and E7.
Uterine cell apoptosis detection by TUNEL assay
Paraffin-embedded uterine tissues were also evaluated for apoptosis via the terminal deoxynucleotidyl transferase dUTP nick end labeling (TUNEL) method using an in situ apoptosis detection kit (Roche Company, Germany) according to the manufacturer's directions. Briefly, the sections were deparaffinized and rehydrated. Endogenous peroxidase activity was blocked with 3% H2O2 for 30 min, and sections were then washed with PBS. The sections were permeated with Proteinase K at 37 °C for 30 min and then rinsed with PBS. Fifty microliters of TUNEL reaction mixture was added to the slides, which were then incubated for 1 h at 37 °C and washed with PBS. Finally, the slides were incubated with Converter-POD for 30 min at 37 °C. Visualization of the apoptotic cells was achieved using DAB, and nuclei were counterstained with hematoxylin for 30 s. The MOD of TUNEL-positive endometrial cells was counted microscopically under 400× magnification.
Primary culture of uterine stromal cells
Primary cultures of mouse uterine stromal cells were obtained from E5 pregnant mouse uteri from which blastocysts had been flushed. Stromal cells were isolated, and purity was assessed according to the previously described method, with minor modifications (Bany et al., Citation2000). Briefly, uterine tissues from E5 mice were removed from the fat tissue, split longitudinally, and washed with Hanks’ balanced salt solution (HBSS; containing 100 U/mL penicillin and 100 μg/mL streptomycin). Tissues were cut into small pieces (2–3 mm) and then digested in HBSS (containing antibiotics and 0.25% trypsin-0.04% EDTA) for 8 min at 37 °C. The tube was shaken gently to remove the endometrial epithelial cells, and the supernatant was discarded. The digested pieces were washed twice in HBSS and incubated in HBSS containing 0.1% collagenase for 40 min at 37 °C, followed by shaking until supernatants became turbid. At the end of digestion, the reactions were terminated with Dulbecco’s modified Eagle’s medium-F12 medium (DMEM-F12, HyClone, Logan, UT) containing 10% heat-inactivated fetal bovine serum (FBS) and were centrifuged at 450 × g for 10 min. The pellet was passed through a nylon tissue sieve (200 nylon mesh per 2.5 cm) and washed twice with serum-free DMEM-F12 medium before the initiation of the primary culture.
The pellet was suspended in DMEM-F12 medium containing 10% heat-inactivated FBS. The cells were plated in 24-well culture plates at a concentration of 2 × 105 cells/well. After an initial culture of 1 h in a humidified 5% CO2/95% O2 incubator at 37 °C to allow stromal cell attachment, the free-floating cells were removed, and fresh medium was added to the wells. The ESCs were cultured in DMEM-F12 complete medium for 24 h and were then identified by immunocytochemistry using a vimentin polyclonal antibody (1:200, 10366-1-AP, Proteintech Group, Chicago, IL) and a cytokeratin polyclonal antibody (1:200, 10830-1-AP, Proteintech Group, Chicago, IL). The purity of the stromal cells, mean ± SEM, was 85.9 ± 0.04%.
To study the effect of oxidative stress on the proliferative ability of the cells, the ESCs were then exposed to 0, 100, 200, 400, 800 and 1000 μM hydrogen peroxide (H2O2) for 24 h in a humidified 5% CO2/95% O2 incubator at 37 °C. The proliferation index was determined using a methyl thiazolyl tetrazolium (MTT) assay. Control cells were incubated with DMEM-F12 alone. After the addition of 10 µL MTT per well, the 96-well plate (2 mL/well) was incubated for 4 h, and 90 µL of 10 mg/mL sodium dodecyl sulfate was then pipetted into each well. After incubating at 37 °C for 4 h, the microplate was read using a Bio-Rad microplate reader at a wavelength of 570 nm. The proliferative activity of the ESCs was expressed as the stimulation index (SI) as follows (Lei et al., Citation2012):
Statistical analysis
Data were expressed as means ± SEM and analyzed using SPSS 18.0 software (SPSS Sciences, Chicago, IL). To evaluate the experimental data, a two-way (restraint stress × gestational age) analysis of variance (ANOVA) was performed, followed by a Duncan post hoc test. The significant difference between control and stressed mice at a given gestational age was evaluated using independent samples t- test (Ortolani et al., Citation2014). In the data for food intake, n was the number of cages per group, while n was the number of mice per group for the other data collected. To analyze the effect of H2O2 on the proliferative activity of ESCs, one-way ANOVA with a LSD post hoc test was performed. Different uppercase letters represent significant differences among E3, E5 and E7 in the control group (p < 0.05), and different lowercase letters represent significant differences among E3, E5 and E7 in the stressed group (p < 0.05). *p < 0.05 and #p < 0.01 were used to denote significance compared to the corresponding control groups.
Results
Food intake and body weight during mouse embryo implantation
In the present study, restraint stress decreased food intake (, F(1, 49) = 16.35, p < 0.01). The initial body weight of pregnant mice at the three gestational ages was not influenced by restraint stress (, F(1, 75) = 0.74, p = 0.39) or gestational age (F(2, 75) = 3.05, p = 0.053). There was no difference in final body weight among the three gestational ages in the control group (F(2, 42) = 1.30, p = 0.28). However, restraint stress significantly decreased the final body weight of pregnant mice on E3, E5 and E7 (independent t-test: t = 2.92, p = 0.009; t = 4.25, p < 0.01; t = 3.84, p = 0.001). In addition, weight gain was affected by restraint stress (F(1, 75) = 75.95, p < 0.01) and gestational age (F(2, 75) = 10.34, p < 0.01). The weight increase was slower in the stressed group than in the corresponding control group on E3, E5 and E7 (independent t-test: t = 4.40, p < 0.01; t = 6.51, p < 0.01; t = 4.81, p < 0.01).
Table 1. Effect of restraint stress on food intake and body weight of pregnant mice during embryo implantation.
Ovarian and uterine weight during mouse embryo implantation
Restraint stress resulted in a significant decrease in the actual and relative weights of the uterus and ovary (). The actual and relative weight of uterus and ovary were influenced by restraint stress (F(1, 106) = 13.00, p < 0.01; F(1, 139) = 15.10, p < 0.01) and gestational age (F(2,106) = 48.82, p < 0.01; F(2, 139) = 49.50, p < 0.01). However, there was no interaction effect of the two factors (stress and age) on the actual and relative weight of uterus and ovary. Restraint stress significantly decreased the actual weight of uterus and ovary on E5 and E7 (independent t-test: t = 2.26, p = 0.03; t = 2.29, p = 0.03), and the relative weight of uterus and ovary on E5 and E7 (independent t-test: t = 3.08, p = 0.004; t = 2.53, p = 0.02).
Figure 2. Effects of restraint stress on the actual and relative weight (percent body weight) of uterus and ovary during mouse embryo implantation. Data are expressed as mean ± SEM (n = 10 mice per group). Different uppercase letters represent significant differences among E3, E5 and E7 in the control group (p < 0.05), and different lowercase letters represent significant differences among E3, E5 and E7 in the stressed group (p < 0.05) (two-way ANOVA followed by Duncan post hoc test). *p < 0.05 and #p < 0.01 denote significance compared to the corresponding control group (independent samples t-test). The relative weight of uterus and ovary was calculated as organ-to-body weight ratio (×100%). E3: Embryonic day 3; E5: Embryonic day 5; E7: Embryonic day 7.

Dynamic uterine structure during mouse embryo implantation
Before implantation, the uterine lumen was enlarged on E3 (). The glandular epithelium was highly branched, and the lumen of endometrial glands was full of a pink-stained secretion that provides nutrition for endometrial adaptive remodeling (, E3 control group). The endometrium displayed signs of hyperemia and edema, which indicates an optimal environment for blastocyst implantation in E5 control mice (). The morphology of the uterus appeared loose at the periphery, with many microvessels, and compact at the center, which was composed of primary decidual cells (, E5 control group). As the embryos grew, the number of endometrial glands decreased and they were predominantly present in the basal layer of the endometrium (, E7 control group). There was residual uterine lumen on the mesometrial side, and the embryos were located on the antimesometrial side of the uterus (). After restraint stress, the endometrium appeared loose and infiltrated by many inflammatory cells. The lumens of the uterine glands were obliterated (, E7-stressed group), with an irregular epithelial cell arrangement and vacuolation (, E3- and E5-stressed group). Although the endometrium displayed signs of hyperemia and edema, the decidual area around the implanting blastocyst was not obvious with the uterine lumen incompletely closed (, E5-stressed group).
Figure 3. The dynamic structure of the uterus during mouse embryo implantation. Under an optical microscope with 40× objective, the uterine transverse section is oval-shaped and consists of three outside-in histological elements: the perimetrium, the myometrium and the endometrium. The myometrium contains an inner circular layer and an outer longitudinal layer of smooth muscle. In addition, a vascular layer is located between the muscle layers. On E7, the embryo and the decidual area are shown in the uterine section. DB: Decidua basalis; E: Embryo; End: Endometrium; E3: Embryonic day 3; E5: Embryonic day 5; E7: Embryonic day 7; M: Mesometrium; UL: Uterine lumen. Bar = 500 μm.
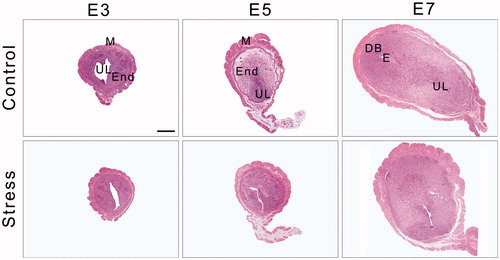
Figure 4. Effects of restraint stress on the morphology of the endometrium and uterine glands during mouse embryo implantation. Data are mean ± SEM (n = 10 mice per group). Different uppercase letters represent significant differences among E3, E5 and E7 in the control group (p < 0.05), and different lowercase letters represent significant differences among E3, E5 and E7 in the stressed group (p < 0.05) (two-way ANOVA followed by Duncan post hoc test). *p < 0.05 and #p < 0.01 denote significance compared to the corresponding control group (independent samples t-test). Long arrows indicate obliteration of uterine glands and arrowheads indicate vacuolation of a glandular epithelial cell. E3: Embryonic day 3; E5: Embryonic day 5; E7: Embryonic day 7. Bar = 20 μm.
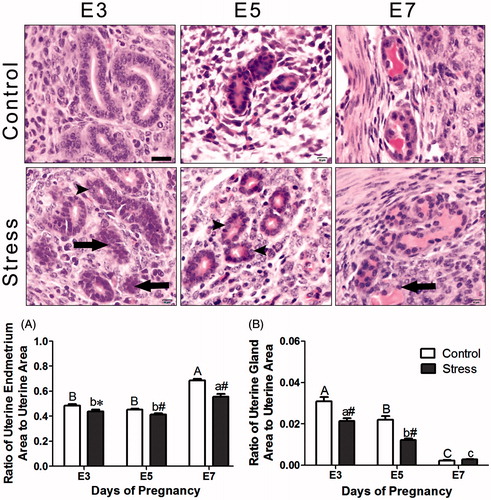
Statistically, the endometrium became enlarged as the embryos grew (, F(2, 130) = 49.95, p < 0.01). The relative endometrial area in the stressed mice was reduced relative to that of the control mice (, F(1, 130) = 127.18, p < 0.01 for restraint stress effects). There was an interaction effect of the two factors (stress and age) on the relative endometrial area (F(2, 130) = 7.37, p = 0.001). However, the uterine gland area decreased with embryo growth (, F(2, 101) = 109.56, p < 0.01). After restraint stress, the relative uterine gland area was decreased (, F(1, 101) = 23.12, p < 0.01). There was some interaction effect of the two factors (stress and age) on the relative uterine gland area (F(2, 101) = 6.53, p = 0.002). On E7, and there was no significant difference in the relative uterine gland area between the control and stressed mice (independent t-test: t = −1.36, p = 0.19).
Changes in uterine blood vessels during mouse embryo implantation
As shown in , vascular endothelial cells expressing CD34 appeared predominantly throughout the endometrium as streaked shapes or single cells. On E7, intense staining for CD34 was observed in the uterine mesometrial stromal cells. The MOD of CD34 immunostaining was affected by restraint stress (, F(1, 44) = 243.63, p < 0.01) and gestational age (, F(2, 44) = 193.53, p < 0.01). In comparison with the respective control mice, the MOD of CD34 immunostaining in the stressed mice was decreased on E5 and E7 (independent t-test: t = 8.50, p < 0.01 on E5 and t = 13.08, p < 0.01 on E7). There was some interaction effect of the two factors (stress and age) on the expression of CD34 immunostaining (F(2, 44) = 111.05, p < 0.01).
Figure 5. Immunohistochemical staining for CD34 and VEGF was used to identify angiogenesis and new microvessel formation. Long arrows indicate a CD34-positive cell and arrowheads indicate a VEGF-positive cell. E3: Embryonic day 3; E5: Embryonic day 5; E7: Embryonic day 7; VEGF: Vascular endothelial growth factor. Bar = 50 μm.
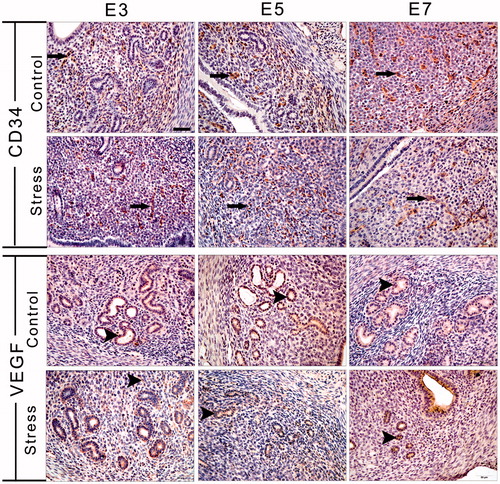
Figure 6. Effects of restraint stress on the vascular biomarkers VEGF and CD34 during mouse embryo implantation. Data are mean ± SEM (n = 10 mice per group). Different uppercase letters represent significant differences among E3, E5 and E7 in the control group (p < 0.05), and different lowercase letters represent significant differences among E3, E5 and E7 in the stressed group (p < 0.05) (two-way ANOVA followed by Duncan post hoc test). *p < 0.05 and #p < 0.01 denote significance compared to the corresponding control group (independent samples t-test). E3: Embryonic day 3; E5: Embryonic day 5; E7: Embryonic day 7. VEGF: Vascular endothelial growth factor.
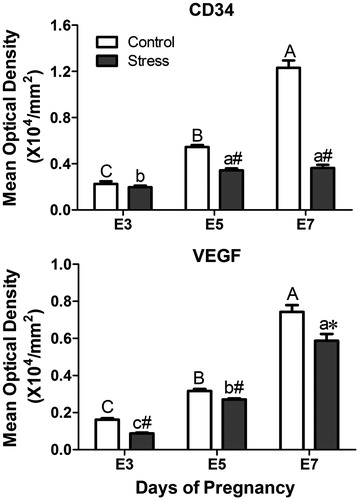
As shown in , the number of VEGF-positive cells was generally low in the endometrium of the pregnant uterus, with the most intense VEGF-positive cell staining present in the glandular epithelium. The MOD of VEGF immunostaining was influenced by restraint stress (, F(1, 25) = 25.92, p < 0.01) and gestational age (, F(2, 25) = 299.57, p < 0.01). Compared with the corresponding control group, restraint stress significantly decreased the MOD of VEGF immunostaining at the three gestational stages (independent t-test: t = 9.50, p < 0.01 on E3, t = 3.41, p = 0.007 on E5 and t = 2.97, p = 0.02 on E7). There was some interaction effect of the two factors (stress and age) on the expression of VEGF-positive cells (F(2,25) = 3.46, p = 0.047). Thus, restraint stress had an inhibitory action on blood vessel development during embryo implantation.
Proliferation and apoptosis in the uterus during mouse embryo implantation
In the present study, PCNA-positive cells were predominantly scattered in the endometrium and among glandular epithelial cells in E3 control mice (). After restraint stress, PCNA-positive cells were rarely expressed in the uterine glands. On E5, PCNA-positive cells were scattered in the endometrium, but not in the uterine glands, and there was no difference in the expression sites between the control and stressed groups. On E7, except for expression in the endometrium, little expression could be observed in the uterine glands. The MOD of PCNA immunostaining was affected by restraint stress (, F(1, 51) = 6.74, p = 0.012) and gestational age (, F(2, 51) = 5.16, p = 0.009). There was no interaction effect of the two factors on the expression of PCNA-positive cells. Restraint stress significantly decreased the expression of PCNA-positive cells on E5 (independent t-test: t = 2.37, p = 0.03).
Figure 7. Immunohistochemical staining with PCNA was used to visualize uterine cell proliferation, and the TUNEL method detected apoptosis of endometrial cells. Long arrows indicate a PCNA-positive cell and arrowheads indicate a TUNEL-positive cell. E3: Embryonic day 3; E5: Embryonic day 5; E7: Embryonic day 7; PCNA: Proliferating cell nuclear antigen; TUNEL: Terminal deoxynucleotidyl transferase dUTP nick end labeling. Bar = 50 μm.
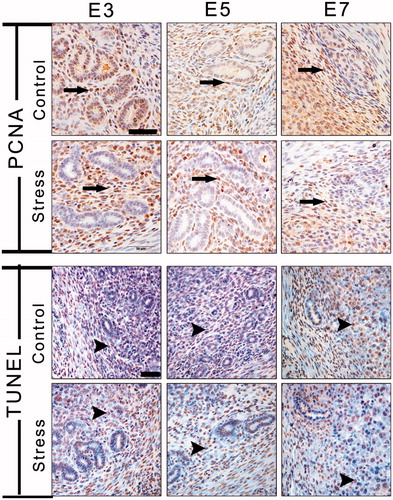
Figure 8. Effects of restraint stress on proliferation and apoptosis in the uterus during mouse embryo implantation. Data are mean ± SEM (n = 10 mice per group). Different uppercase letters represent significant differences among E3, E5 and E7 in the control group (p < 0.05), and different lowercase letters represent significant differences among E3, E5 and E7 in the stressed group (p < 0.05) (two-way ANOVA followed by Duncan post hoc test). *p < 0.05 and #p < 0.01 denote significance compared to the corresponding control group (independent samples t-test). E3: Embryonic day 3; E5: Embryonic day 5; E7: Embryonic day 7. MOD: Mean optical density.
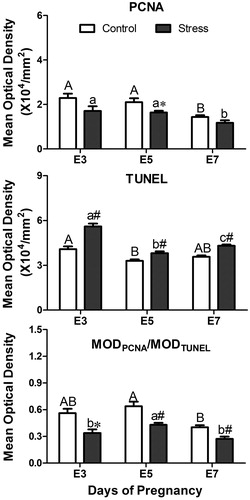
TUNEL-positive cells (apoptotic cells) were intensely scattered in the endometrium and mesometrium and were less distributed in the uterine glands. During embryo implantation, there was no difference in TUNEL expression between the control and stressed groups (). Statistically, the MOD of TUNEL immunostaining was influenced by restraint stress (, F(1, 348) = 61.47, p < 0.01) and gestational age (, F(2, 348) = 36.92, p < 0.01). There was some interaction effect of the two factors (stress and age) on the expression of TUNEL-positive cells (F(2, 348) = 6.24, p = 0.002). Compared with the corresponding control group, restraint stress significantly increased the expression of TUNEL-positive cells (independent t-test: t = −5.18, p < 0.01 on E3; t = −3.06, p = 0.003 on E5; t = −6.04, p < 0.01 on E7).
The ratio of MODPCNA/MODTUNEL was used to reflect the balance of cell proliferation and apoptosis. A two-way ANOVA revealed the significant effects that restraint stress (, F(1, 51) = 18.85, p < 0.01) and gestational age (, F(2, 51) = 6.54, p = 0.003) had the ratio of MODPCNA/MODTUNEL. There was no interaction effect of the two factors on the expression of PCNA-positive cells. Compared with the corresponding control group, restraint stress significantly decreased the ratio of MODPCNA/MODTUNEL (independent t-test: t = 2.78, p = 0.011 on E3; t = 3.56, p = 0.002 on E5; t = 3.55, p = 0.006 on E7). Hence, the endometrial cell resistance to proliferation and increased sensitivity to apoptosis suggested a possible pathological change in the endometrium induced by restraint stress.
Effect of oxidation on endometrial stromal cell proliferation
The cultured cells were vimentin-positive () and cytokeratin-negative (); these findings indicate that these cells are ESCs, and the cell purity of the isolated ESCs (mean ± SEM) was 85.9 ± 0.04%. In addition, H2O2 significantly reduced the proliferative capacity of ESCs in a dose-dependent manner, and ESCs stimulated with concentrations of H2O2 at 100–1000 µM exhibited 22.7–61.4% of the proliferative capacity of control ESCs in vitro (, one-way ANOVA with LSD post hoc test, p < 0.01).
Figure 9. Immunohistochemical staining with vimentin and cytokeratin was used to identify endometrial stromal cell (ESC) purity. (A) vimentin (+); (B) cytokeratin (−); (C) negative control; (D) effect of oxidant (H2O2) on ESC proliferation in vitro. To confirm that H2O2 induces apoptosis in endometrium cells, ESCs were treated with H2O2 over a 24-h time course and the proliferative activity of ESCs was measured by methyl thiazolyl tetrazolium (MTT) assay. Data are expressed as mean ± SEM (n = 9, number of pregnant control mice used to provide ESCs per H2O2 concentration) and analyzed by one-way ANOVA with a LSD post hoc test. #p < 0.01 denotes significance compared to the control group (zero H2O2). E3: Embryonic day 3; E5: Embryonic day 5; E7: Embryonic day 7. Bar = 50 μm.
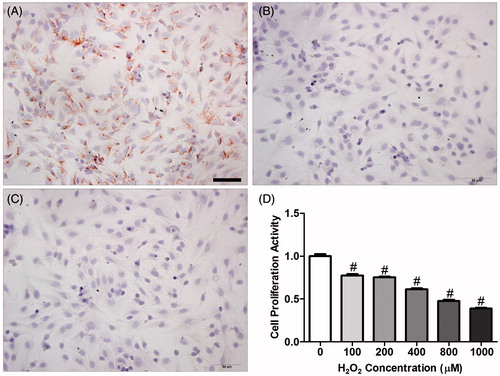
Discussion
The present study demonstrated that repeated restraint stress delayed endometrial regeneration during implantation, caused abnormal development in the endometrial glands, stroma and uterine vasculature, and induced apoptosis in endometrial cells via the oxidative stress pathway. Thus, these findings support the hypothesis that restraint stress has negative influence on the endometrial adaptive remodeling via an oxidative stress pathway, which resulted in the decrease in number of implantation sites.
Restraint stress decreased body and organ weight
Our previous study had demonstrated that the restraint stress model was valid because of an obviously increased concentration of plasma corticosterone (E3–E7, by 69–88%, when compared with the corresponding control mice) (Liu et al., Citation2014). Stress-induced increase in corticosterone secretion is implicated in stress-induced reduction in food intake, inducing anorexia in mice (Bazhan et al., Citation2007; Bazhan & Zelena, Citation2013). Restraint stress during late gestation decreases body weight and food intake of the dams and produces long-term metabolic changes (Mairesse et al., Citation2007). Moreover, restraint stress not only decreases food consumption (Ortolani et al., Citation2014), but also increases appetite for sweet food in rodents (Ely et al., Citation1997). Generally, maternal weight increases with increase in gestational age. Restraint stress during embryo implantation caused a conspicuous reduction in food intake and suppressed maternal body weight gain in the present study. It is speculated that restraint stress would also cause growth retardation of the fetuses. Whether the changes in food intake and body weight induced by restraint stress found here are associated with the endometrial adaptive remodeling needs to be studied.
On E3, embryos are at the 8-cell stage and are in the oviduct near the uterus. On E5, implantation is completed following arrival of the embryos in the uterus. Hence, the present study used the relative weight of uterus and ovary as an indicator of the development of embryos in the uterus of pregnant mice. Interestingly, restraint stress resulted in a significant decrease in the ovarian and uterine weight of E5 and E7 pregnant mice, and there was no influence on the ovarian and uterine weight of E3 pregnant mice in the present study. These findings confirm the harmful influence of restraint stress on embryo implantation (Liu et al., Citation2014).
Restraint stress interfered with establishment of the implantation platform
Acute stress can have a positive influence on the hypothalamic–pituitary–gonadal (HPG) axis to induce the luteinizing hormone (LH) surge, which triggers ovulation in estrogen-treated ovariectomized rats and perimenopausal or menopausal women (Tarín et al., Citation2010). However, chronic stress generally impairs reproductive process including ovulation, implantation and sexual behavior (Einarsson et al., Citation2008). Endometrial adaptive remodeling is essential for accepting the fertilized oocyte implanted during pregnancy. In the present study, restraint stress interfered with this development in the endometrium. This included obliteration of endometrial glands (with irregular epithelial cell arrangement and vacuolation), a looser endometrial structure comprising stromal cells with vascular spaces and mononuclear inflammatory cell infiltrations, and a delay in the decidual response. Moreover, restraint stress significantly decreased the relative endometrial area and the uterine gland area on E3 and E5. Endometrial adaptive remodeling takes place via endometrial proliferation and differentiation (Furuya et al., Citation2008). To further investigate the mechanism of establishing the implantation platform, this present study mainly focused on uterine vascular remodeling and endometrial proliferation.
Uterine vascular remodeling
Angiogenesis is crucial for the development of the endometrium and for embryo implantation and pregnancy establishment (Nakamura et al., Citation2014). VEGF withdrawal results in the regression of vasculature (Ferrara, Citation2001), and aberrant endometrial angiogenesis may result in failed implantation and first-trimester miscarriage (Nardo, Citation2005). In addition, CD34 is a transmembrane protein expressed in vascular endothelial cells (Wood et al., Citation1997) and it is highly expressed in vessels formed by vasculogenesis and angiogenesis. During implantation, it is necessary to meet the high metabolic demand of an increase in blood flow with abundant nutrients and oxygen, which is dependent on endometrial angiogenesis and vascular permeability. In the present study, CD34 was predominantly present throughout the endometrium and was visualized as either a streak or in single cells, and VEGF-positive cells generally had low expression in the endometrium of the pregnant uterus, with the most intense VEGF-positive expression in the glandular epithelium. In addition, restraint stress significantly decreased the expression of VEGF and CD34 from E3 to E7. Thus, restraint stress had an inhibitory effect on blood vessel development during embryo implantation.
Endometrial proliferation
Studies with mutant and progesterone-induced uterine gland knockout mice found that uterine glands and their secretions are required for the establishment of uterine receptivity and blastocyst implantation and stromal cell decidualization (Spencer, Citation2014). In the present study, PCNA-positive (i.e., proliferating) cells were predominantly scattered in the endometrium and among glandular epithelial cells, and TUNEL-positive (i.e., apoptotic) cells were scattered in the endometrium and mesometrium and were less distributed in the uterine glands. The balance between cell proliferation and cell death is crucial for successful embryo implantation and the maintenance of pregnancy (Correia-da-Silva et al., Citation2004). Apoptosis and proliferation of ESCs is associated with the pathogenic mechanism responsible for adenomyosis (Yang et al., Citation2007). Cas-3/PCNA balance in the luminal and glandular epithelium shifts death inducers after E3, and blastocyst implantation induces uterine luminal epithelial cell death and stromal cell proliferation around the embryo in the uterus (Oner et al., Citation2010). This present study considered the ratio of MODPCNA/MODTUNEL as indicative of the state of endometrial cell development. This demonstrated that restraint stress decreased the ratio of MODPCNA/MODTUNEL, indicating that stress delayed the proliferation of and induced apoptosis in endometrial cells. Hence, endometrial cell resistance to proliferation and increased sensitivity to apoptosis indicated a possible pathogenic aspect of the endometrium induced by restraint stress. However, unclear is the mechanism by which restraint stress induces such changes in the endometrium and blood vessels.
Oxidative stress induced by restraint stress inhibited the proliferation of ESCs
H2O2 is an apoptosis-inducing agent that injures various types of cells by both apoptosis and necrosis. The present study found that 400 µM H2O2 can inhibit the proliferation of and can cause apoptosis in ESCs in vitro, further confirming that H2O2 induces apoptosis in endometrial cells. These findings are consistent with our previous in vivo results showing that restraint stress threatened the intracellular equilibrium of oxidants and antioxidants and led to oxidative stress with decline in glutathione peroxidase (GSH-PX), superoxide dismutase (SOD) and total antioxidant capacity (T-AOC) activities and increased malondialdehyde (MDA) content during implantation (E5) and postimplantation (Liu et al., Citation2014). The oxidative stress induced by restraint stress has been detected in other organs, such as rat testis and epididymis (Dhanabalan et al., Citation2011). However, Appiah et al. found that exogenous H2O2 causes a dose-dependent decrease in the contractions of isolated rat uteri, which have higher levels of GSH-Px and MnSOD activity, while a suppression of elevated CuZnSOD activity occurred during incubations (Appiah et al., Citation2009). A recent study also reported that the mitochondria-dependent and JNK-p38 kinase pathways were involved in H2O2-induced oxidative damage of human trophoblast-like JEG-3 cells (Tang et al., Citation2014). However, the signaling molecule involved by which oxidative stress disturbs endometrial adaptive remodeling requires further research.
Conclusion
It is concluded that restraint stress-altered adaptive remodeling of the uterine endometrium during implantation in mice. The balance of proliferation and apoptosis in endometrial cells and the development of uterine vasculature were disturbed during restraint stress, reducing successful embryo implantation. Furthermore, it is suggested that the mechanism of apoptosis caused by restraint stress might be oxidative stress in endometrial cells.
Declaration of interest
The authors disclose that there is no conflict of financial interest that influenced this study.
This study was supported by the National High-tech Research and Development Projects (863) (No. 2013AA102306) and the National Natural Science Foundation of China (No. 31172277, 31272483, 31272516, 31372332, 31472157).
References
- Appiah I, Milovanovic S, Radojicic R, Nikolic-Kokic A, Orescanin-Dusic Z, Slavic M, Trbojevic S, et al. (2009). Hydrogen peroxide affects contractile activity and anti-oxidant enzymes in rat uterus. Br J Pharmacol 158:1932–41
- Bany BM, Harvey MB, Schultz GA. (2000). Expression of matrix metalloproteinases 2 and 9 in the mouse uterus during implantation and oil-induced decidualization. J Reprod Fertil 120:125–34
- Bazhan NV, Makarova EN, Shevchenko AIu, Iakovleva TV. (2007). Repeating of emotional stress prevents development of melanocortin obesity and type 2 diabetes in the mice with the Agouti yellow mutation. Ross Fiziol Zh Im I M Sechenova 93(11):1237–44
- Bazhan N, Zelena D. (2013). Food-intake regulation during stress by the hypothalamo-pituitary-adrenal axis. Brain Res Bull 95:46–53
- Blanco O, Leno-Durán E, Morales JC, Olivares EG, Ruiz-Ruiz C. (2009). Human decidual stromal cells protect lymphocytes from apoptosis. Placenta 30:677–85
- Correia-da-Silva G, Bell SC, Pringle JH, Teixeira NA. (2004). Patterns of uterine cellular proliferation and apoptosis in the implantation site of the rat during pregnancy. Placenta 25:538–47
- Dhanabalan S, Jubendradass R, Latha P, Mathur PP. (2011). Effect of restraint stress on 2,3,7,8 tetrachloro dibenzo-p-dioxin induced testicular and epididymal toxicity in rats. Hum Exp Toxicol 30:567–78
- Douglas NC, Tang H, Gomez R, Pytowski B, Hicklin DJ, Sauer CM, Kitajewski J, et al. (2009). Vascular endothelial growth factor receptor 2 (VEGFR-2) functions to promote uterine decidual angiogenesis during early pregnancy in the mouse. Endocrinology 150:3845–54
- Einarsson S, Brandt Y, Lundeheim N, Madej A. (2008). Stress and its influence on reproduction in pigs: a review. Acta Vet Scand 50:48
- Ely DR, Dapper V, Marasca J, Corrêa JB, Gamaro GD, Xavier MH, Michalowski MB, et al. (1997). Effect of restraint stress on feeding behavior of rats. Physiol Behav 61(3):395–98
- Ferrara N. (2001). Role of vascular endothelial growth factor in regulation of physiological angiogenesis. Am J Physiol Cell Physiol 280:C1358–66
- Furuya M, Ishida J, Aoki I, Fukamizu A. (2008). Pathophysiology of placentation abnormalities in pregnancy-induced hypertension. Vasc Health Risk Manag 4:1301–13
- Golub MS, Campbell MA, Kaufman FL, Iyer P, Li LH, Donald JM, Morgan JE. (2004). Effects of restraint stress in gestation: implications for rodent developmental toxicology studies. Birth Defects Res B Dev Reprod Toxicol 71:26–36
- Lee YE, Byun SK, Shin S, Jang JY, Choi BI, Park D, Jeon JH, et al. (2008). Effects of maternal restraint stress on fetal development of ICR mice. Exp Anim 57:19–25
- Lei W, Feng XH, Deng WB, Ni H, Zhang ZR, Jia B, Yang XL, et al. (2012). Progesterone and DNA damage encourage uterine cell proliferation and decidualization through up-regulating ribonucleotide reductase 2 expression during early pregnancy in mice. J Biol Chem 287:15174–92
- Lim HJ, Wang H. (2010). Uterine disorders and pregnancy complications: insights from mouse models. J Clin Invest 120:1004–15
- Liu G, Dong Y, Wang Z, Cao J, Chen Y. (2014). Restraint stress alters immune parameters and induces oxidative stress in the mouse uterus during embryo implantation. Stress 17:494–503
- Mairesse J, Lesage J, Breton C, Bréant B, Hahn T, Darnaudéry M, Dickson SL, et al. (2007). Maternal stress alters endocrine function of the feto-placental unit in rats. Am J Physiol Endocrinol Metab 292(6):E1526–33
- Matson BC, Caron KM. (2014). Uterine natural killer cells as modulators of the maternal-fetal vasculature. Int J Dev Biol 58:199–204
- Nakamura H, Hosono T, Minato K, Hamasaki T, Kumasawa K, Kimura T. (2014). Importance of optimal local uterine blood flow for implantation. J Obstet Gynaecol Res 40:1668–73
- Nardo LG. (2005). Vascular endothelial growth factor expression in the endometrium during the menstrual cycle, implantation window and early pregnancy. Curr Opin Obstet Gynecol 17:419–23
- Oner H, Oner J, Demir R. (2010). Distributions of PCNA and Cas-3 in rat uterus during early pregnancy. Folia Histochem Cytobiol 48:71–7
- Ortolani D, Garcia MC, Melo-Thomas L, Spadari-Bratfisch RC. (2014). Stress-induced endocrine response and anxiety: the effects of comfort food in rats. Stress 17:211–18
- Shapiro GD, Fraser WD, Frasch MG, Séguin JR. (2013). Psychosocial stress in pregnancy and preterm birth: associations and mechanisms. J Perinat Med 41(6):631–45
- Silver RM, Ruiz RJ. (2013). Invited commentary: maternal stress and stillbirth: another piece of the puzzle. Am J Epidemiol 177(3):228–29
- Spencer TE. (2014). Biological roles of uterine glands in pregnancy. Semin Reprod Med 32:346–57
- Tang C, Liang J, Qian J, Jin L, Du M, Li M, Li D. (2014). Opposing role of JNK-p38 kinase and ERK1/2 in hydrogen peroxide-induced oxidative damage of human trophoblast-like JEG-3 cells. Int J Clin Exp Pathol 15(7):959–68
- Tang X, Chen Y, Ran H, Jiang Y, He B, Wang B, Kong S, et al. (2015). Systemic morphine treatment derails normal uterine receptivity, leading to embryo implantation failure in mice. Biol Reprod 92(5):118
- Tarín JJ, Hamatani T, Cano A. (2010). Acute stress may induce ovulation in women. Reprod Biol Endocrinol 26(8):53. doi: 10.1186/1477-7827-8-53
- Torche F. (2011). The effect of maternal stress on birth outcomes: exploiting a natural experiment. Demography 48(4):1473–91
- Tsartsara E, Johnson MP. (2006). The impact of miscarriage on women's pregnancy-specific anxiety and feelings of prenatal maternal-fetal attachment during the course of a subsequent pregnancy: an exploratory follow-up study. J Psychosom Obstet Gynaecol 27(3):173–82
- Ubaldi M, Ricciardelli E, Pasqualini L, Sannino G, Soverchia L, Ruggeri B, Falcinelli S, et al. (2015). Biomarkers of hippocampal gene expression in a mouse restraint chronic stress model. Pharmacogenomics 16(5):471–82
- Wood HB, May G, Healy L, Enver T, Morriss-Kay GM. (1997). CD34 expression patterns during early mouse development are related to modes of blood vessel formation and reveal additional sites of hematopoiesis. Blood 90:2300–11
- Yang JH, Wu MY, Chen CD, Chen MJ, Yang YS, Ho HN. (2007). Altered apoptosis and proliferation in endometrial stromal cells of women with adenomyosis. Hum Reprod 22:945–52