Abstract
This study evaluated whether phenotypic appearance (PA) alteration during two developmental phases in laying hens, reared in two different group sizes, affects stress and immune responses. After hatching, 750 chicks were randomly assigned to 30 pens at a group size of either 10 or 40 birds. Then, the appearance of 0, 30, 50, 70 or 100% of the chicks in each pen was altered by blackdyeing their head feathers (marked); remaining chicks were unmarked. At 32 weeks, basal and postacute stress plasma corticosterone concentration, leukocyte counts, phytohemagglutinin-p lymphoproliferative and primary antibody responses were measured in six birds/pen. Analysis of variances (ANOVAs) showed no differences among treatment combinations. In a second phase, birds within initially homogeneous pens were sequentially either marked or had dye bleached to alter PA of 70% of hens in each flock (= group in a pen). Hens within initially heterogeneous pens remained unaltered as controls. The above variables were remeasured. Hens in phenotypically manipulated pens showed modified leukocyte counts compared to hens in control pens, indicating a chronic stress reaction in all penmates (whether individual PA was altered or not). Social isolation increased plasma corticosterone concentration. However, within groups of n = 40, phenotypically unaltered hens had lower responses than their altered penmate counterparts, suggesting that remaining in a stable PA group aids better coping with challenges. Although all hens in manipulated pens showed modified leukocyte counts, their antibody and lymphoproliferative responses did not differ from controls suggesting that all groupmates were able to immunologically cope with the challenges presented, within the timeframe evaluated.
Introduction
Stressors comprise a variety of conditions or forces that may be external to the body and disturb homeostasis, inducing a state of stress (Kuenzel & Jurkevich, Citation2010; Siegel, Citation1995). This state usually involves activation of the hypothalamo–pituitary–adrenal (HPA) axis, and in the final stage, the release of glucocorticoids (corticosterone) from the adrenal glands (Hazard et al., Citation2008; Kuenzel & Jurkevich, Citation2010; Siegel, Citation1995). One of the main systems affected by this stress response is the immune system (Dhabhar, Citation2009; Nazar et al., Citation2012; Shini et al., Citation2009), which provides individuals with rapid and efficient responses. These reflect a diverse repertoire of recognition and effector molecules and a certain flexibility to match the changing internal and external environment (Degen et al., Citation2005; Du Pasquier, Citation2005). Failure to accomplish this objective at different stages along rearing and production leads to various detrimental health- and welfare-related consequences in poultry (Dohms & Metz, Citation1991; Ruff, Citation1999). When stressors are repeated or their consequences are prolonged and sustained in time immune depression may ensue, and this is mainly attributed to the immunosuppressive effects of corticosterone (Shini & Kaiser, Citation2009; Shini et al., Citation2010).
Both social interactions and social environment and its instability may frequently result in an important source of physiological and behavioral stressful situations (Bilcík & Keeling, Citation1999; Dennis et al., Citation2008; Guzman et al., Citation2009). How a particular individual should interact with a conspecific in a group (or flock) relies on the capacity of the individual bird to readily access information about the bird that it is encountering (e.g., individual identity, gender, social and reproductive status, kinship, familiarity) (Gobbini & Haxby, Citation2007). Therefore, recognition, or the lack thereof, is an important factor with demonstrated relevance in the group dynamics of poultry species, including the domestic fowl (Guzman & Marin, Citation2008; Lindberg & Nicol, Citation1996; Marin et al., Citation2001). Phenotypic appearance (PA) characteristics, such as body mass, comb size and feather coloring, allow individual birds to be recognized, and the establishment of social structures is usually adjusted through different social interactions ranging from neutral to even aggressive encounters. Additionally, it is observed that birds are phenotypically different from their conspecifics, due to natural variation or artificial manipulation, are at higher risk of being pecked and possibly cannibalized (Dennis et al., Citation2008; Estevez et al., Citation2003). Moreover, the inclusion of marks on the birds was found to alter not only behavioral responses but also stress-related hormonal responses, body weight and egg production (Dennis et al., Citation2008; Hostetler & Ryabinin, Citation2013; Liste et al., Citation2015).
The developed theoretical framework emphasizes the fact that management of birds in productive systems now implies an increasing number of factors that need to be considered and controlled to maximize both poultry welfare and production. When not properly taken care of, these aspects may lead to stressful situations with detrimental behavioral and physiological consequences. In particular, factors such as PA composition, social group size (GS), previous social experience, environmental familiarity and stability of social group might play an important role in modulating adaptative responses of birds, and hence, their performance and welfare (Bilcík & Keeling, Citation1999; Estevez et al., Citation2003; Jones, Citation1996; Sossidou & Elson, Citation2009). We hypothesized that manipulation of the PA of Hy-line Brown laying hens along ontogeny may have long-lasting effects on stress- and immune-response parameters. We proposed that those effects may depend on the GS they are reared at. Specifically, in a first phase, the phenotypic composition of the groups was changed on day one and stress and immune parameters were assessed when birds had reached full maturity and peak egg production. Groups evaluated included homogeneous or heterogeneous PA compositions. In a second phase, the PA of homogeneous groups was partially and sequentially altered to determine whether changes during this ontogeny stage may impact later stress and immune responses. Heterogeneous PA groups remained stable and were used as controls.
Methods
Animals and rearing conditions
This study is part of a larger project that evaluated the effects of changes in PA and GS on behavioral and productive variables. Newly hatched one-day-old Hy-line brown female chicks were obtained from Avigán Terralta and transported to the experimental poultry facility at the Neiker-Tecnalia research center (Vitoria-Gasteiz, Spain). Immediately upon arrival, 750 chicks were randomly assigned to one of the 30 pens and housed in groups of 10 or 40 birds (GS 10 and 40, respectively; 15 pens per GS). Birds were kept at the same density (8 birds/m2), management and housing/environmental conditions described elsewhere (Marin et al., Citation2014). The dimension of the pens housing 10 birds was 0.75 × 1.78 m (1.25 m2) and 2.00 × 2.50 m (5.00 m2) for groups of 40 birds. At two days of age, all birds were individually identified with two white-laminated paper tags on each wing side (Cornetto & Estevez, Citation2001; Liste et al., Citation2015). Tags included a pen number and the individual bird number identification (Dennis et al., Citation2008). Before the laying period started, pens were also provided with nest space of 26.5 × 35 cm and 106 × 35 cm (width × depth) for GS 10 and 40, respectively. Animal care was provided in adherence with Institutional animal Care and Use Committee guidelines.
The experiment was approved by the ethical committee at Neiker-Tecnalia in compliance with the Spanish legislation regarding the use of animals for experimental and other scientific purposes (Real Decreto 1201/2005).
Experimental design phase I: same phenotypic appearance throughout
Upon arrival, the PA was either maintained unaltered (unmarked) or artificially altered (marked) by placing a black mark with a nontoxic dye on the back of the head (Dennis et al., Citation2008; Liste et al., Citation2015). Pens from each GS were assigned to one of the following five PA conditions: 0, 30, 50, 70 or 100% of the birds with marks, yielding three pens for each PA option and within each GS (; Marin et al., Citation2014). According to the birds’ PA, the following five conditions were studied in each of the two GS: homogeneous groups with 100% individuals unmarked, homogeneous groups with 100% individuals marked, heterogeneous groups with 30% individuals marked and 70% unmarked, heterogeneous groups with 50% individuals marked and 50% unmarked and heterogeneous groups with 70% individuals marked and 30% unmarked. This summarizes 10 PA condition combinations in phase I of the study. All groups (flocks) remained with the same assigned PA until 34 weeks of age ().
Table 1. Experimental design for phase I and II regarding phenotypic appearance (PA) assignment within groups (flocks) for each group size (10 or 40 birds per pen, flock).
Experimental design phase II: changing phenotypic appearance in 70% of hens in a group (flock) through time
The same birds used in phase I of the study were also evaluated during phase II. After 34 weeks of age, groups with initially homogeneous PA (100% marked or 100% unmarked) of each GS were altered by changing PA of 70% of the hens of these groups. The PA changes were accomplished by either randomly marking the birds’ head (or unmarking them by applying an H2O2 solution to the dyed feathers (Liste et al., Citation2015; Marin et al., Citation2014) (; Marin et al., Citation2014) until the following distribution was reached in the originally homogeneous groups: 30% marked and 70% unmarked, or 70% marked and 30% unmarked. The groups with initially heterogeneous PA in each GS (30% marked and 70% unmarked, and 70% marked and 30% unmarked) remained with the same originally assigned phenotype composition until the end of this study and served as controls for the phase-II PA changes. A total of four control conditions and four phenotypically altered conditions were evaluated.
Variables measured and sampling time schedule
Variables were analyzed at 29 weeks of age and at 46 weeks of age. A total of 180 birds were evaluated in phase I: six randomly chosen birds from each pen were designated for analysis of the condition of their immune system: three marked and three unmarked birds from the heterogeneous condition and six marked or six unmarked birds from each homogeneous condition. Similarly, in phase II, three marked and three unmarked birds from each condition were evaluated, totaling in this case 144 birds.
The complete sampling procedure, both in phase I and phase II, took 3 days within a period of one week. On day one, each bird was captured, and the brachial vein of the left wing was punctured in order to obtain 1 ml of ethylenediamine tetraacetic acid (EDTA)-anticoagulated blood for smears and for quantifying basal plasma corticosterone concentration. At the same time, the phytohemagglutinin-p (PHA-P) lymphoproliferative response was induced. To ensure a reliable corticosterone value, the sampling procedure took no longer than 80 s from the moment the bird was initially captured.
A blood smear was made on a slide for each sample, which was placed on ice prior to centrifugation of the samples. Immediately after, birds were intraperitoneally injected with 0.5 ml of a 10% sheep red blood cell (SRBC) suspension in order to induce a humoral immune response. One week later, the corticosterone response to an acute social isolation stressor was evaluated by placing each hen in isolation for 5 min in a dark cardboard box (Cheng et al., Citation2002; Jones, Citation1996; Richard et al., Citation2008). Therefore, the stressor combined the effects of a novel environment and isolation from conspecifics. After 5 min, blood was immediately withdrawn from a brachial vein, on the right side, for the assessment of plasma corticosterone concentration and primary antibody response against SRBC. Plasma was obtained by blood centrifugation at 2500 g during 15 min and it was immediately stored at −20 °C until further analyses.
Lymphoproliferative responses to phytohemagglutinin-p (PHA-P; inflammation)
To determine cell-mediated immunity, the responses to PHA-P (a lectin from Phaseolus vulgaris (Sigma Chemical, St. Louis, MO)), was measured in the wing web following the methods described elsewhere (Nazar & Marin, Citation2011; Roberts et al., Citation2009). Briefly, on day 1, a 0.05 ml of a solution of PHA-P in phosphate saline buffer (1 mg/ml) was injected intradermally in the wing web of each bird. The dermal swelling response was measured as the percentage increase in wing web thickness at the injection site 24-h post-PHA-P injection (day 2). Measurements were recorded to the nearest 0.01 mm using a mechanical digital micrometer.
Heterophil/lymphocyte and innate/acquired ratio
Leukocyte counts were performed on blood smears stained with the May–Grünwald–Giemsa method. Differential counts of 100 white cells per blood smear were made (Huff et al., Citation2005; Fair et al., Citation1999; Nazar & Marin, Citation2011). The INN/ACQ (used to compare the subpopulations of cells involved in the two main branches of the immune response) cell and heterophil/lymphocyte (H/L) ratios (commonly used as a hematological indicator of chronic stress) were calculated using the following formulae: INN/ACQ = (number of basophils + number of heterophils + number of monocytes) / (number of eosinophils + number of lymphocytes); H / L = (number of heterophils) / (number of lymphocytes).
Primary antibody response against sheep red blood cells (SRBC)
To evaluate the induced humoral immune response, the antibody titer was assessed with a microagglutination assay in serum (Nazar & Marin, Citation2011; Smits & Baos, Citation2005) obtained from blood samples taken one week after the intraperitoneal administration of the SRBC suspension. Briefly, 20 μl of complement-inactivated (through heating to 56°C) plasma was serially diluted in 20 μl of phosphate-buffered saline (PBS; 1:2, 1:4, 1:8, …, 1:512). Next, 20 μl of a 2% suspension of SRBC in PBS was added to all wells. Microplates were incubated at 40°C for 1 h, and hemagglutination by the test plasma samples was compared to the blanks (PBS only) and negative controls (wells with no SRBC suspension). Antibody titers were reported as the Log2 of the highest dilution yielding significant agglutination.
Corticosterone determinations
Plasma corticosterone (ng/ml) was quantified using a validated specific corticosterone enzyme-linked immunosorbent assay (ELISA) kit (ENZO Life Sciences – ADI-901-097) (Davies et al., Citation2013) and following the procedure specified by the manufacturer. The reactivity with corticosterone was 100% with a sensitivity of 27.0 pg/ml, detecting concentrations ranging from 32 to 20,000 pg/ml. The cross reactivity with other molecules was: deoxycorticosterone (21.3%), desoxycorticosterone (21.0%), progesterone (0.46%), testosterone (0.31%), tetrahydrocorticosterone (0.28%), aldosterone (0.18%), cortisol (0.046%) and <0.03%: pregnenolone, estradiol, cortisone, 11-dehydrocorticosterone acetate. Intra- and interassay variations were 3.1% and 5.8%, respectively. All samples were assessed together.
Statistical analysis
Data analyses were conducted following general procedures described by Dennis et al. (Citation2008) and Marin et al. (Citation2014). Data within each condition combination replicate were averaged before statistical analysis. Phase-I immunological data were analyzed using mixed-model ANOVAs with five PA conditions at hatch (100% marked, 100% unmarked, 30% marked and 70 % unmarked, 50% marked and 50% unmarked, 70% marked and 30% unmarked), and two GS (10 and 40 hens) as fixed effects and pen as a random effect. H/L ratio data were subjected to a square root transformation before analysis to fit the analysis assumptions. Transformations were not required for the other variables. Phase-II immunological data were analyzed using mixed-model ANOVAs with PA change (70% marked-30% unmarked and 70% unmarked-30% marked from flocks with unchanged PA during adulthood, and 70% marked-30% unmarked and 70% unmarked-30% marked from flocks with PA changed during adulthood) and GS (10 and 40 hens) as fixed effects and pen as a random effect.
For both phases I and II, corticosterone data analyses also incorporated in the model stress treatment (basal and stressed) as a within-subject factor and the three-level interactions (PA × GS × stress treatment) were also evaluated. Whenever significant effects were detected, least square means were determined and contrasts were used for means comparisons. Post hoc treatment group comparisons were conducted using the Fisher least significant difference test. A p value of <0.05 was considered to represent significant differences.
Results
Phase I: same PA throughout
Plasma corticosterone concentrations under basal conditions and after acute stress in adult hens reared from day one in homogeneous and heterogeneous PA groups are shown in . Analyses revealed a main effect (F(1,32) = 340, p < 0.001) of acute stress exposure with no effects of the PA assigned on day one, the GS in which birds were reared, or interactions among treatments (p > 0.26 in all cases) at the end of phase I (29 weeks of age).
Figure 1. Plasma corticosterone concentrations in adult hens with different artificial phenotypic appearance (PA) from day one of age. Basal = birds reared in regular husbandry conditions; Stressed = same birds submitted to 5 min acute stress consisting of individual isolation in a novel environment. Bars represent the mean ± SE (number of birds per homogeneous or heterogeneous group/phenotype condition = 9, total number of birds in the study = 180). Data were analyzed by mixed-model ANOVA; p = 0.001, stressed > basal; no significant effects of PA or GS. M = marked; UM = unmarked; 100, 30, 50 and 70 = 100, 30, 50 and 70% of the birds within a flock either marked or unmarked. Group size 10 = birds reared in groups of 10 individuals. Group size 40 = birds reared in groups of 40 individuals.
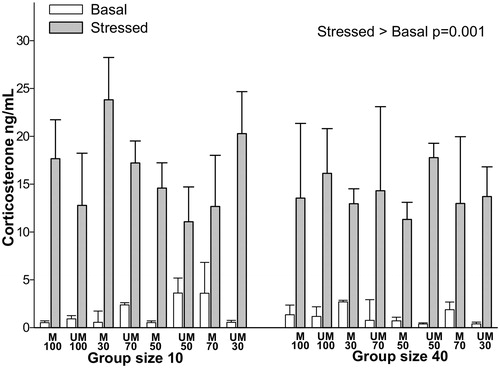
The effects of PA and GS on immune-related variables are shown in . Analyses of inflammation (PHA-P) and antibody titer responses, and H/L and INN/ACQ ratios, showed no effects of the PA assigned, GS or their interaction (p > 0.17 in all cases; for further details) on any of the cellular or humoral variables evaluated.
Table 2. Immune-related variables measured (mean ± SE) in adult laying hens with different artificial phenotypic appearance (PA) from day one of age.
Phase II: changing PA proportions after age 34 weeks
Results of the effects of PA alteration and GS during adulthood, after 34 weeks, on the basal and acute stress response corticosterone concentrations, after changing the PA of 70% hens in flocks initially homogeneous for marked or unmarked hens are shown in . Analyses revealed a significant interaction between PA, GS and acute stress response (F(7,32) = 2.34, p = 0.047). Mean group comparisons showed that basal corticosterone concentration was similar among all groups. After the acute stressor exposure, every hen showed increased corticosterone concentration. Within GS 10 hens, both groups of flockmates (with their PA changed and unchanged) showed similar corticosterone increases after the acute stress challenge. However, within the flocks of 40 hens that were submitted to a PA alteration, those hens that remained with their PA unchanged from hatch showed a minor acute stress increment in their corticosterone response compared to their flockmate counterparts with changed PA (p < 0.01 in both cases; ).
Figure 2. Plasma corticosterone concentrations after changing the phenotypic appearance (PA) of 70% of a flock during adulthood. Basal = birds reared in regular husbandry conditions; Stressed = same birds submitted to 5 min acute stress consisting of individual isolation in a novel environment. Bars represent the mean ± SE (number of birds per treatment = 9, total number of birds in the study = 144). Data were analyzed by mixed-model ANOVA. M = marked; UM = unmarked; 30 and 70 = 30% and 70% of the birds within the flock (i.e., group/pen) either marked or unmarked. Flocks with nonaltered PA: PA of all birds within the flock remained unchanged from day 1 of age. Flocks with altered PA = PA of birds was either changed during adulthood or remained unchanged from day 1 of age. Group size 10 = birds reared in groups of 10; Group size 40 = birds reared in groups of 40. a,cStressed groups with no common letters differ significantly (p < 0.05; Fisher least significant difference test).
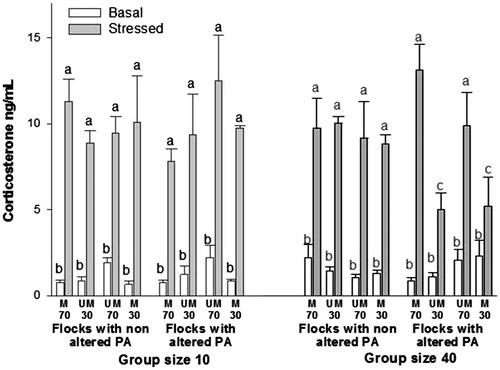
summarizes the results for the immune-related variables evaluated after the PA of 70% of the previously homogeneous flocks was changed during adulthood. Analyses revealed a main effect of the PA treatment on H/L ratio (F(3,32) = 3.22, p = 0.01) and INN/ACQ ratio (F(7,32) = 2.35, p = 0.04). No significant main effect of the GS or interaction between PA and GS were detected. Therefore, both altered and nonaltered hens in manipulated pens showed increased H/L and INN/ACQ ratios compared to hens in control pens (where all hens remained with their PA unaltered from hatch). Antibody titer and inflammation were not affected by PA, GS or their combined effects (p > 0.05 in all cases; ).
Table 3. Immune-related variables measured (mean ± SE) after changing the phenotypic appearance (PA) of 70% of a flock during adulthood.
Discussion
The present study evaluated whether a phenotypic manipulation at two stages (posthatching and adulthood) in the ontogeny of Hy-line Brown laying hens, may have long-lasting effects on stress and immune responses, and whether those effects may depend on the size of groups (flocks) in which birds are reared. Our results support the contention that diverse stress- and immune-related parameters are differentially influenced by the manipulation of PA depending on the age when the PA manipulation was applied and the GS in which the hens were reared.
The results of phase I (PA changes applied on day 1 without later alteration throughout) showed no individual no combined PA and GS effects. All hens showed similar basal plasma corticosterone concentrations and responded to an acute social isolation stressor as expected and described in the literature (Grissom et al., Citation2008; Hazard et al., Citation2008; Malisch et al., Citation2010; Marin et al., Citation2002). Regarding immune-related variables, all hens showed responses within the expected physiological range for healthy birds. Interestingly, the PA changes applied at hatching did not influence these outcomes nor appeared to interact with the size of groups (flocks) the birds were reared in. As found in complementary studies with these same birds (Liste et al., Citation2015; Marin et al., Citation2014), the described lack of differences across groups is not unexpected as the PA and GS conditions were applied soon after hatching, when early filial learning processes are established (Bolhuis & Bateson, Citation1990; Bolhuis & Honey, Citation1998). Thus, flockmate recognition despite their PA and degree of group PA heterogeneity would be established during this early-study phase, and potential negative behavioral interactions due to differences in PA would not be strong enough to induce a chronic stress state that could compromise immune responses of the birds.
After changing PA in 70% of hens (46 weeks of age) in flocks with homogeneous PA since hatching, all groups (either PA altered or not, ) showed similar basal corticosterone concentration. After exposure to acute social isolation, all groups also responded with an increased corticosterone responses as expected from this type of stressor (Hazard et al., Citation2008; Malisch et al., Citation2010; Marin et al., Citation2001). However, within altered flocks of GS 40 hens during this second phase, hens that remained with their PA unaltered showed significantly lower corticosterone responses than their PA altered group-mates. This difference in corticosterone responses indicates that in GS 40, unaltered hens within a pen with altered hens (independently of whether they belonged to the 100% marked or 100% unmarked initial groups) were able to better cope with a new social challenging situation of isolation in a novel environment. This result may be explained via previous experience in dealing with new situations, possibly learned after each phenotypical alteration was carried out with successful and detrimental outcomes from unaltered and altered PA hens, respectively. Such a phenomenon would have enhanced social plasticity in the unaltered group of hens making them, as above, better adapted to new social challenging situations. Hence, the phenotypical alteration in adulthood of the flock-mates may have initiated a process leading to better coping or habituation for the unaltered hens, and perhaps an opposite scenario for the altered ones.
The analyses of the immune parameters showed that the variables affected by the PA alteration were the H/L and the INN/ACQ ratios. The increased heterophil population (as well as the decrease in lymphocytes) showed that in all flockmates where a proportion of its members had undergone PA alteration in adulthood this change induced hematological changes consistent with an underlying chronic stress process (Gross & Siegel, Citation1985; Nazar & Marin, Citation2011; Siegel, Citation1980; Huff et al., Citation2005). This suggests that the appearance of new phenotypes in a previously homogeneous flock triggered a chronic social reaction, physiologically evidenced in all pen members whether their PA was in particular altered or unaltered. This phenomenon seems to be independent of the flock size because 10 and 40 hen groups manifested the same described response. The elevation of the INN/ACQ ratio may indicate, based on the functionality of the cells in each population, different potential responses. When encountering an actual immune challenge such as bacteria, viruses or parasites, hens in altered flocks would manifest higher innate and diminished acquired responses. Remarkably, despite the finding that all birds in pens with hens with altered adult phenotype showed modified blood cells numbers, their induced antibody (acquired) and lymphoproliferative (innate) responses did not differ from their respective control counterparts. This suggests that all groupmates were able to equally cope at the immunological level with the chronic social challenge induced, at least within the time frame evaluated.
Considering all immune-related variables together, we may infer that there were cellular parameters that seemed to manifest alterations, and effectors of immunity which were not modified by the effects of PA alteration combined with GS. In particular, H/L and INN/ACQ ratios did show effects; however, it seems that this change did not directly impair body weight and egg production (Marin et al., Citation2014) or immune effectors. Cellular populations were altered in absolute numbers but their functionality (analyzed via lymphoproliferation and antibody production) seemed not to be undergoing any modification. A plausible explanation for this phenomenon could be based on the time frame evaluated in the study and the physiology of stress responses considered in the context of prolonged effects on homeostasis. The possibility exists that a series of adjustments in immune effectors due to chronic stress would have taken place in shorter times than those analyzed in our work (Dhabhar, Citation2009; Dhabhar & McEwen, Citation1997; Dohms & Metz, Citation1991; Shini & Kaiser, Citation2009; Shini et al., Citation2010), as for parameters that were affected in instances temporarily closer to the first phenotypic alterations (Marin et al., Citation2014). In this sense, the birds in our study might have developed a physiological habituation to social challenging situations concerning their basal corticosterone response and also immune effector parameters. The only remaining manifestation of the mentioned alteration would be the different H/L and INN/ACQ ratios.
In conclusion, phenotypical appearance alterations and group size are important factors when designing poultry management schedules to optimize welfare. Repeated alterations taking place over long periods of time should be analyzed in the context of possible physiological responses to environmental challenges. This could be of importance because some phenomena may lead to habituation or to sensitization depending on the contexts.
Acknowledgements
The authors wish to thank the excellent contribution of Iurancha González, David López, the maintenance personnel at Neiker-Tecnalia and Avícola Gorrotxategui S.L. We also thank the veterinary assistance of Ignacia Beltrán de Heredia and David Lizaso, and Dario C. Arbelo for his technical advice during leukocyte determinations. We thank the editor and two anonymous reviewers for the comments received that helped improve the quality of the manuscript.
Declaration of interest
The authors report no conflict of interest. The authors alone are responsible for the content and writing of the paper. This project was funded by the Ministry of Economy and Competitiveness of Spain (Agro resources and technologies (AGL) 2010-18276, MODELAY), including the salary of G.L. This work done while R.H.M. was on a sabbatical visit to Neiker-Tecnalia, Arkaute Agrifood Campus, Department of Animal Production, P.O. Box 46, 01080 Vitoria-Gasteiz, under an IKERBASQUE (Basque Foundation for Science, Alameda Urquijo 36-5 Plaza Bizkaia, 48011 Bilbao, Spain) visiting professor support program.
R.H.M. is a career member of Consejo Nacional de Investigaciones Científicas y Técnicas (CONICET) Argentina. F.N.N. holds a post-graduate research fellowship from the latter institution.
References
- Bilcík B, Keeling LJ. (1999). Changes in feather condition in relation to feather pecking and aggressive behaviour in laying hens. Br Poult Sci 40:444–51
- Bolhuis JJ, Bateson P. (1990). The importance of being first: a primacy effect in filial imprinting. Anim Behav 40:472–83
- Bolhuis JJ, Honey RC. (1998). Imprinting, learning and development: from behaviour to brain and back. Trends Neurosci 21:306–11
- Cheng HW, Singleton P, Muir WM. (2002). Social stress in laying hens: differential dopamine and corticosterone responses after intermingling different genetic strains of chickens. Poult Sci 81:1265–72
- Cornetto T, Estevez, I. (2001). Influence of vertical panels on use of space by domestic fowl. Appl Anim Behav Sci 71:141–53
- Davies S, Rodriguez NS, Sweazea KL, Deviche P. (2013). The effect of acute stress and long-term corticosteroid administration on plasma metabolites in an urban and desert songbird. Physiol Biochem Zool 86:47–60
- Degen WGJ, Daal NV, Rothwell L, Kaiser P, Schijns VEJC. (2005). Th1/Th2 polarization by viral and helminth infection in birds. Vet Microbiol 105:163–67
- Dennis RL, Newberry RC, Cheng HW, Estevez I. (2008). Appearance matters: artificial marking alters aggression and stress. Poult Sci 87:1939–46
- Dhabhar FS. (2009). Enhancing versus suppressive effects of stress on immune function: implications for immunoprotection and immunopathology. Neuroimmunomodulation 16:300–17
- Dhabhar FS, McEwen BS. (1997). Acute stress enhances while chronic stress suppresses cell-mediated immunity in vivo: a potential role for leukocyte trafficking. Brain Behav Immun 11:286–306
- Dohms JE, Metz A. (1991). Stress-mechanisms of immunosuppression. Vet Immunol Immunopathol 30:89–109
- Estevez I, Keeling LJ, Newberry RC. (2003). Decreasing aggression with increasing group size in young domestic fowl. Appl Anim Behav Sci 84:213–18
- Fair JM, Hansen ES, Ricklefs RE. (1999). Growth, developmental stability and immune response in juvenile Japanese quails (Coturnix coturnix japonica). Proc Biol Sci 266:1735–42
- Gobbini MI, Haxby JV. (2007). Neural systems for recognition of familiar faces. Neuropsychologia 45:32–41
- Grissom N, Kerr W, Bhatnagar S. (2008). Struggling behavior during restraint is regulated by stress experience. Behav Brain Res 191:219–26
- Gross WB, Siegel PB. (1985). Selective breeding of chickens forcorticosterone response to social stress. Poult Sci 64:2230–33
- Guzman DA, Marin RH. (2008). Social reinstatement responses of meat-type chickens to familiar and unfamiliar conspecifics after exposure to an acute stressor. Appl Anim Behav Sci 110:282–93
- Guzman DA, Satterlee DG, Kembro JM, Schmidt JB, Marin RH. (2009). Effect of the density of conspecifics on runway social reinstatement behavior of male Japanese quail genetically selected for contrasting adrenocortical responsiveness to stress. Poult Sci 88:2482–90
- Hazard D, Couty M, Richard S, Guémené D. (2008). Intensity and duration of corticosterone response to stressful situations in Japanese quail divergently selected for tonic immobility. Gen Comp Endocrinol 155:288–97
- Hostetler CM, Ryabinin AE. (2013). The CRF system and social behavior: a review. Front Neurosci 7:92
- Huff GR, Huff WE, Balog JM. (2005). Stress response differences and disease susceptibility reflected by heterophil to lymphocyte ratio in turkeys selected for increased body weight. Poult Sci 84:709–17
- Jones RB. (1996). Fear and adaptability in poultry: insights, implications and imperatives. Worlds Poult Sci J 52:131–74
- Kuenzel WJ, Jurkevich A. (2010). Molecular neuroendocrine events during stress in poultry. Poult Sci 89:832–40
- Lindberg AC, Nicol CJ. (1996). Effects of social and environmental familiarity on group preferences and spacing behaviour in laying hens. Appl Anim Behav Sci 49:109–23
- Liste G, Campderrich I, de Heredia IB, Estevez I. (2015). The relevance of variations in group size and phenotypic appearance on the behaviour and movement patterns of young domestic fowl. Appl Anim Behav Sci 163:144–57
- Malisch JL, Satterlee DG, Cockrem JF, Wada H, Breuner CW. (2010). How acute is the acute stress response? Baseline corticosterone and corticosteroid-binding globulin levels change 24 h after an acute stressor in Japanese quail. Gen Comp Endocrinol 165:345–50
- Marin RH, Benavidez E, Garcia DA, Satterlee DG. (2002). Sex differences in central benzodiazepine receptor densities and circulating corticosterone release after acute stress in broiler chicks. Poult Sci 81:261–4
- Marin RH, Freytes P, Guzman D, Jones RB. (2001). Effects of an acute stressor on fear and on the social reinstatement responses of domestic chicks to cagemates and strangers. Appl Anim Behav Sci 71:57–66
- Marin RH, Liste MG, Campderrich I, Estevez I. (2014). The impact of phenotypic appearance on body weight and egg production in laying hens: a group-size- and experience-dependent phenomenon. Poult Sci 93:1623–35
- Nazar FN, Magnoli AP, Dalcero AM, Marin RH. (2012). Effect of feed contamination with aflatoxin B1 and administration of exogenous corticosterone on Japanese quail biochemical and immunological parameters. Poult Sci 91:47–54
- Nazar FN, Marin RH. (2011). Chronic stress and environmental enrichment as opposite factors affecting the immune response in Japanese quail (Coturnix coturnix japonica). Stress 14:166–73
- Du Pasquier L. (2005). Meeting the demand for innate and adaptive immunities during evolution. Scand J Immunol 62 Suppl 1:39–48
- Richard S, Wacrenier-Ceré N, Hazard D, Saint-Dizier H, Arnould C, Faure JM. (2008). Behavioural and endocrine fear responses in Japanese quail upon presentation of a novel object in the home cage. Behav Processes 77:313–19
- Roberts ML, Buchanan KL, Evans MR, Marin RH, Satterlee DG. (2009). The effects of testosterone on immune function in quail selected for divergent plasma corticosterone response. J Exp Biol 212:3125–31
- Ruff MD. (1999). Important parasites in poultry production systems. Vet Parasitol 84:337–47
- Shini S, Huff GR, Shini A, Kaiser P. (2010). Understanding stress-induced immunosuppression: exploration of cytokine and chemokine gene profiles in chicken peripheral leukocytes. Poult Sci 89:841–51
- Shini S, Kaiser P. (2009). Effects of stress, mimicked by administration of corticosterone in drinking water, on the expression of chicken cytokine and chemokine genes in lymphocytes. Stress 12:388–99
- Shini S, Shini A, Huff GR. (2009). Effects of chronic and repeated corticosterone administration in rearing chickens on physiology, the onset of lay and egg production of hens. Physiol Behav 98:73–7
- Siegel HS. (1995). Gordon memorial lecture. Stress, strains and resistance. Br Poult Sci 36:3–22
- Siegel HS. (1980). Physiological stress in birds. Bioscience 30:529–34
- Smits JEG, Baos R. (2005). Evaluation of the antibody mediated immune response in nestling American kestrels (Falco sparverius). Dev Comp Immunol 29:161–70
- Sossidou EN, Elson HA. (2009). Hens’ welfare to egg quality: a European perspective. Worlds Poult Sci J 65:709–18