Abstract
The present study was aimed at determining the role of centrally released oxytocin in regulation of blood pressure and heart rate (HR) under resting conditions and during an acute air-jet stress in rats with a myocardial infarction and controls infarcted. Four weeks after ligation of a coronary artery or sham surgery, conscious Sprague Dawley rats were subjected to one of the following intracerebroventricular (ICV) infusions: (1) 0.9% NaCl (control), (2) oxytocin, (3) oxytocin receptor antagonist {desGly-NH2-d(CH2)5[D-Tyr2Thr4]OVT}(OXYANT). Resting arterial blood pressure and HR were not affected by any of the ICV infusions either in the infarcted or sham-operated rats. In the control experiments, the pressor and tachycardic responses to the air jet of infarcted rats were significantly greater than in the sham-operated rats. OXYANT significantly enhanced the cardiovascular responses to stress only in the sham-operated rats whereas oxytocin significantly attenuated both responses in the infarcted but not in the sham-operated rats. The results suggest that centrally released endogenous oxytocin significantly reduces the cardiovascular responses to the acute stressor in control rats. This buffering function of the brain-oxytocin system is not efficient during the post-myocardial infarction state, however it may be restored by central administration of exogenous oxytocin.
Introduction
Until recently, oxytocin, the neurohypophysial neuropeptide, has been known for its pivotal role in the progress of parturition and initiation of milk ejection (Russell et al. Citation2003; Leng et al. Citation2005). The regulatory spectrum of oxytocin is now known to be substantially wider. An increasing amount of data in the literature strongly suggests that oxytocin may exert an analgesic effect (Robinson et al. Citation2002) and participate in the control of cognitive functions, affective behaviour and stress-related reactions (Engelmann et al. Citation1999; Windle et al. Citation1997; Neumann et al. Citation2000; Nakashima et al. Citation2002; Choleris et al. Citation2007; Blume et al. Citation2008; Carter et al. Citation2008; Neumann Citation2008). In addition, some investigators have provided evidence that oxytocin may be involved in regulation of the cardiovascular system by means of direct peripheral and indirect central actions (Petersson et al. Citation1996; Braga et al. Citation2000; McCann et al. Citation2002; Costa e Sousa et al. Citation2005). Anatomical studies of oxytocin pathways in the brain have revealed extensive innervation of the brain stem structures regulating the cardiovascular, behavioural and neuroendocrine responses to stress by oxytocin fibres projecting from the paraventricular nucleus (PVN) (Sawchenko and Swanson Citation1982; Bergquist and Ludwig Citation2008). Expression of oxytocin receptors in the same regions of the brain stem has been also well documented (Sofroniew and Schrell Citation1981; Barberis and Tribollet Citation1996), and there is evidence that oxytocin acting on the neurones of the solitary vagal complex modulates reflex control of the heart rate (HR) (Higa et al. Citation2002). The studies of Braga et al. (Citation2000) and Michelini (Citation2001) suggest that during physical exercise central oxytocin decreases modulation of tachycardia by the neurones located in the nucleus of the solitary tract. Moreover, it has been found that content of oxytocin in the brain stem and the PVN is significantly altered in the spontaneously hypertensive rats (Möhring et al. Citation1983; Morris et al. Citation1985). Engagement of oxytocin in the control of neuroendocrine responses to stress and its putative contribution to the regulation of cardiovascular parameters raises the question whether or not the centrally released oxytocin may also be involved in modulation of the cardiovascular responses to stress. Sudden, alarming stress evokes rapid resetting of the autonomic control of the HR and blood pressure (Lampert et al. Citation2000; Kario et al. Citation2003). It is generally known that in each individual the same stressors may evoke markedly different cardiovascular responses under different circumstances, however, the exact mechanisms responsible for this variation are not yet fully understood. Previous studies have implicated the involvement of brain angiotensin II AT1 receptors and vasopressin V1a receptors in regulation of the cardiovascular responses to acute air-jet stress (Mayorov and Head Citation2003; Dobruch et al. Citation2005; Mayorov et al. Citation2004; Cudnoch-Je˛drzejewska et al. Citation2007; Stojicić et al. Citation2008). Moreover, it has been shown that engagement of these receptors in cardiovascular responses to stress increases remarkably during the post-infarct state. Thus far, the role of oxytocin receptors in the regulation of cardiovascular responses to stress has not been clarified. The only study devoted to this problem (Petersson and Uvnäs-Moberg Citation2007) did not provide convincing results. The present investigation was designed to determine whether centrally released endogenous oxytocin is involved in regulation of the arterial blood pressure and HR under resting conditions and during an alarming stress (air-jet), and whether its function is altered during the post-myocardial infarction state. The results provide evidence that centrally released endogenous oxytocin reduces the pressor and tachycardic responses to the acute air jet stress in the control non-infarcted rats and that this action is abolished after a myocardial infarction. Preliminary data from this study have been presented at a scientific meeting (Szczepanska-Sadowska et al. Citation2008).
Methods
Animals and surgical procedures
Adult, male Sprague Dawley rats (SPRD/Möl/Lod) weighing 250–300 g were used in the study. The rats were raised in the Department of Animal Breeding at the Medical University of Warsaw. They were housed in a room with regulated temperature (range 22–25°C) and had free access to tap water and a commercial rat diet containing 0.45% NaCl. The rats were maintained on a 12 h/12 h light/dark cycle (light on at 7.00 am). During the experimental sessions, water and food were withdrawn to avoid incidental changes in cardiovascular parameters due to feeding and drinking activity. All surgical procedures and experimental protocols were in accordance with the international/European Union guidelines and regulations on the use and care of laboratory animals. The experimental protocol was approved by the Ethical Committee on the Animal Research of the Warsaw Medical University. Before the experimental sessions all rats were subjected to the following surgical procedures: ligation of a coronary artery at 10–12 weeks of age, or a sham procedure, implantation of a guide tube into the left cerebral ventricle at 14–16 weeks of age, and insertion of an aortic catheter at 15–17 weeks of age. Each of these procedures was performed under pentobarbital anaesthesia (Pentobarbital, Biowet, Puławy; 15 mg/ml, 50 mg/kg i.p.).
Induction of myocardial infarction and sham procedure
The rats were divided at random into two groups. One group was subjected to the myocardial infarction procedure and the other group to sham surgery. The myocardial infarct was produced according to the technique described by Selye et al. (Citation1960) and modified by Dobruch et al. (Citation2005). In brief: a surgical incision was made between the fourth and the fifth intercostal space while the lungs were ventilated by means of air puffs applied from a small rubber balloon. The infarction was produced by ligation of the left coronary artery with a 6-0 prolen stich (Ethicon). In the sham-infarcted rats, the cardiac pericardium was touched with the needle but the coronary artery was not ligated. At the end of both procedures, the wound was closed with surgical sutures (Ethicon, Sommerville, NJ, USA 4.0) and spontaneous ventilation was re-established. After surgery, the rats were given analgesic (Buprenorphine sulphate, Polfa, Rzeszów, Poland; 9 μg/ml, 30 μg/kg s.c.) and antibiotic (Penicillin, Polfa, Pabianice, Poland; 30,000 U in 1 ml/rat i.m), and placed in individual cages. The rate of survival of the infarcted and the sham-operated rats was 53 and 97%, respectively.
Implantation of the intracerebroventricular guide tube
The rat's head was placed in a Kopf's stereotaxic device and the stainless steel guide tube (o.d., 0.81 mm, MIFAM S.A. Milanówek, Poland) was implanted into the brain using the following stereotaxic coordinates: 1.3 mm posterior to the bregma and 2 mm lateral from the midline, 4.5 mm below the surface of the skull. The guide tube was secured in the skull with acrylic cement (Duracryl, SPOFA-DENTAL, Jicin, Czech Republic) and closed with a stainless steel stylet (o.d., 0.46 mm). After surgery, the rats received antibiotic (Penicillin, Polfa; 30,000 U in 1 ml/rat i.m.) and were placed in their home cages.
Implantation of the arterial catheter
The intraarterial catheter consisted of an intraarterial portion (3.5–4.0 cm long; i.d., 0.12 mm; o.d., 0.25 mm) and an external portion (i.d., 0.25 mm; o.d., 0.4 mm) made from polyvinyl tubing (Scientific Commodities, Inc., Lake Havasu City, AZ, USA). The internal part was inserted into the aorta through a femoral artery, so that its end was 2 cm below the renal arteries. The external part was pushed under the skin and exteriorised dorsally on the neck. The catheter was filled with 0.9% NaCl containing 500 U of heparin/ml and plugged with a stopper. The experiments were performed 24–48 h after the surgery when the rats had fully recovered from the anaesthesia and could move freely.
Experimental protocol
Six groups of experiments were performed in order to determine the cardiovascular effects of stimulation and blockade of oxytocin receptors in the brain under resting conditions and during stress in the infarcted and the sham-operated rats. At the beginning of each experimental session, the arterial catheter was connected to the blood pressure and HR recording system (BIOPAC, MP100, Santa Barbara, CA, USA). The stylet was removed from the guide tube and the stainless steel tube (o.d., 0.46 mm) that was 0.1 mm longer than the stylet was inserted into the guide tube and connected via the polyvinyl tubing to a microsyringe placed in a Harvard 22 Infusion Pump (Harvard, Smith Natick, MA, USA). The intracerebroventricular (ICV) infusion started after a 15–30 min rest period, allowed for stabilisation of the cardiovascular parameters. Each ICV infusion was delivered at the rate of 5 μl/h (0.083 μl/min) and lasted until the end of the experiment. The rats were assigned at random into six experimental groups. The control sham-operated (n = 7) and control infarcted (n = 8) groups were infused with vehicle (0.9% NaCl). The sham-operated (n = 6) and infarcted (n = 7) groups receiving oxytocin were infused with 0.9% NaCl for the first 10 min and subsequently with oxytocin (Phoenix Pharmaceuticals, Strasbourg, France) at the rate of 100 ng/5μl/h (1.66 pmol/0.083 μl/min) during the remaining part of the experiment. A similar experimental design was applied in the sham-operated (n = 7) and infarcted (n = 6) rats receiving the OXYANT except that DesGly-NH2-d(CH2)5[D-Tyr2,Thr4]OVT was infused at the rate of 4.3 μg/5 μl/h (71.6 nmol/0.083 μl/min). The antagonist was kindly provided by Prof. Maurice Manning, Medical College of Ohio, USA). In the case of oxytocin, the rationale for the choice of the dose of oxytocin used in the present study was based on our previous investigation in which we found that an equimolar dose of vasopressin produces significant changes in baseline blood pressure and in cardiovascular responses to stress in rats after a myocardial infarction. Because oxytocin and vasopressin are frequently released together we aimed to compare their central cardiovascular effects using comparable doses. The dose of OXYANT was calculated basing on a published effective dose of DesGly-NH2-d(CH2)5[D-Tyr2,Thr4]OVT (Manning et al. Citation1995). In each group measurements of mean arterial blood pressure (MABP) and HR were continued for 50 min under resting conditions and during 10 min after application of the air jet which served as the acute stress. The air jet was applied using the technique described by Zhang et al. (Citation1999) and modified by Dobruch et al. (Citation2005). The procedure involves blowing air for 1 s onto the top of the rat's had from a tank containing compressed air (10 atm) through a funnel (i.d., 41.5 mm) held above the rat's head and connected with the tank by plastic tubing (i.d., 3.0 mm). The cardiovascular responses to the stressor were evaluated by measuring the maximum increases in MABP and HR occurring during the first 10 s after application of the stressor. MABP and HR immediately preceding application of the air jet were used as the reference level. The experiments were concluded with measurements of the end-diastolic ventricular pressure (EDVP) in the left ventricle. To this end the rats were anaesthetised (Pentobarbital, Biowet, Puławy, Poland; 15 mg/ml, 50 mg/kg i.p.) and a catheter (Dural Plastics and Engineering, Auburn, Australia; i.d., 0.5 mm; o.d., 0.8 mm) was inserted through the right carotid artery and the aortic arch into the left ventricle.
Measurements
MABP and HR were recorded continuously by means of a BIOPAC system (MP100) which determines MABP as the area under the arterial pressure curve divided by the cardiac cycle duration. The system calculates HR (beats/min) from the number of systolic pressure peaks. The BIOPAC system was also used to determine left ventricle end-diastolic pressure (LVEDP).
Post mortem examination
At the end of the experiment, the rats were killed by an overdose of 5% chloral hydrate (50 mg/ml, 500–667 mg/kg i.p.) and the heart and brain were harvested for evaluation of the size of the myocardial infarct and contact of the ICV cannula with the cerebroventricular system.
Size of the myocardial infarct
The size of the infarct was determined planimetrically (Zhang et al. Citation1999) with some modifications (Dobruch et al. Citation2005). Briefly, the heart was excised from the thorax and gently washed with saline. The wall of the left ventricle (including septum) was separated from the right ventricle along the longitudinal axis and placed flat on transparent graph paper with 1 mm squares. The circumferences of the ventricle and of the infarct scar were outlined on the internal and external surfaces. The surface area of the infarct expressed as the number of mm squares was estimated on both sites of the ventricle and averaged. The size of the infarct scar was expressed as the percentage of the total left ventricle wall surface. Previous studies have shown that estimation of the infarct size with this method corresponds well to evaluation of the infarct size during histological examination (Dobruch et al. Citation2005; Cudnoch-Je˛drzejewska et al. Citation2007, Citation2008).
Verification of the contact of the ICV cannula with the cerebroventricular system
To verify the position of the infusing cannula in the lateral cerebral ventricle 5 μl of Evans blue was injected through the guide tube via the needle used for the ICV infusions. Sagittal sections of the brain were made for visual inspection of the ventricular system for presence of the dye. The inspection showed that all rats used in the experiments had appropriate locations of the infusing cannula.
Statistical analysis
Statistica software (Version 7) was used for statistical analysis of the data. Five min averages covering the first 50 min of the ICV infusion were used in the statistical analysis to determine changes in MABP and HR under resting conditions. Three-way ANOVA on repeated measurements was used to evaluate changes of cardiovascular parameters at rest, as recommended by Ludbrook (Citation1994) and Curran-Everet and Benos (Citation2004). Two-way ANOVA was applied to determine the significance of differences between the air jet stress-induced maximum changes of the cardiovascular parameters in the different groups of experiments. The horizontal and vertical multiple pairwise comparisons (Ludbrook Citation1994; Curran-Everet and Benos Citation2004) were made using the post-hoc Tukey test. The differences were considered significant if P was < 0.05. All values presented in the text, figures and table are mean ± standard error of mean.
Results
The size of the myocardial infarct in the rats assigned to the groups receiving ICV infusions of 0.9% NaCl, oxytocin or OXYANT did not differ significantly and amounted to 33.79 ± 2.40, 32.57 ± 2.30 and 31.00 ± 1.06% of the total left ventricle wall surface. Left ventricle end-diastolic pressure was significantly higher in the infarcted (23.9 ± 1.0 mm Hg; n = 18) than in the sham-operated rats (4.2 ± 0.4 mm Hg; n = 17; [F(1,33) = 313.69; P < 0.001]. Significant differences were also found when the individual groups of the infarcted and sham-operated rats were included in the analysis [F(5,29) = 57.950; P < 0.001] (). MABP and HR at the beginning of the ICV infusions were similar in all experimental groups. As shown in and resting MABP and HR were not appreciably affected by any of the ICV infusions either in the infarcted or in the sham-operated rats. The results of the three way ANOVA on changes of MABP and HR from baseline during ICV infusions of 0.9% NaCl, oxytocin or OXYANT were as follows: ΔMABP: [F(35,245) = 0.606; P = 0.962]; ΔHR: F(35,245) = 0.659; P = 0.931].
Table I. MABP and HR before (0 min) and after 40 min of ICV infusion of 0.9% NaCl (control), oxytocin or oxytocin receptor antagonist in conscious sham-operated rats, and rats after myocardial infarction.
Figure 1 Non-significant changes of MABP and HR from baseline under resting conditions in the sham-operated (open symbols) and infarcted (filled symbols) rats receiving ICV infusions of 0.9% NaCl (triangles), OXYANT (squares) or oxytocin (OXY, circles). The arrow indicates the start of ICV infusion. Number of rats per group: sham-operated 0.9%: n = 7; sham-operated OXY: n = 6; sham-operated OXYANT: n = 7; infarcted 0.9% NaCl: n = 8; infarcted OXY: n = 6; infarcted OXYANT: n = 6.
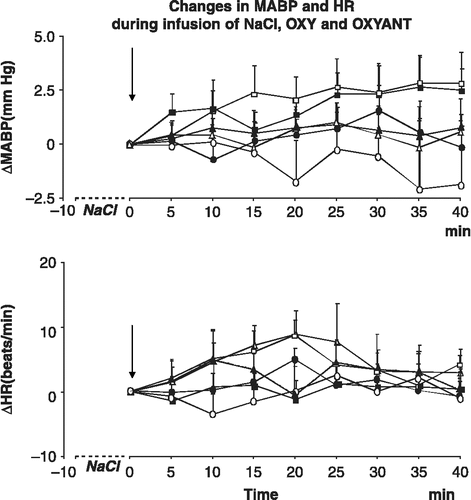
Application of the air jet elicited significant increases of MABP and HR in each experimental group. As illustrated in , changes in MABP and HR appeared immediately after application of the stressor and their duration was similar in each experimental group. The overall two-way ANOVA performed on maximum changes in MABP from baseline (immediately before application of the air jet stressor) revealed significant interaction for data obtained in the infarcted and the sham-operated rats with the type of the experiment [F(5,35) = 15.701; P < 0.001].
Figure 2 Representative illustrations of changes in MABP and HR after application of the air jet stressor (arrow) in the sham-operated and infarcted rats during ICV infusion of 0.9% NaCl, OXY or OXYANT. The Figure shows that (1) during infusion of 0.9% NaCl MABP and HR responses to stress are markedly greater in the infarcted than in the sham-operated rat, (2) ICV administration of OXYANT intensifies MABP and HR responses to stress in the sham-operated but not in the infarcted rat and (3) ICV administered OXY reduces MABP and HR responses to stress in the infarcted but not in the sham-operated rat.
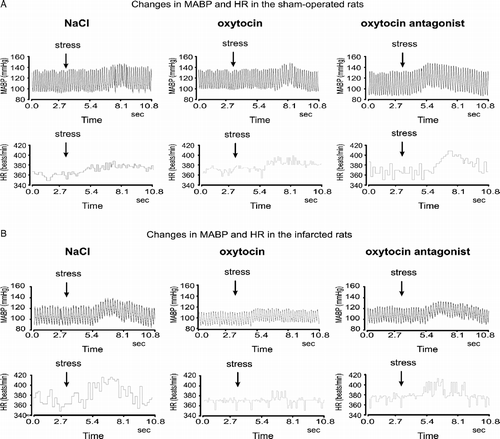
shows significant differences between the individual groups for maximum increases in MABP. In rats given ICV vehicle, the air-jet stressor increased the maximal change in MABP more in infarcted rats than in sham-operated controls (P < 0.001); ICV oxytocin reduced the maximum increase in MABP in the infarcted, but not the sham-operated rats (P < 0.001); in contrast, ICV OXYANT increased the maximum increase in MABP in the sham rats, but not in the infarcted rats (P < 0.001). The maximum increases in MABP expressed as percentage of baseline MABP in the individual groups of rats were as follows: sham-operated control: 6.8 ± 0.9%, infarcted control: 13.1 ± 0.9% (P < 0.001); sham-operated + oxytocin group: 7.4 ± 0.8%, infarcted + oxytocin group: 7.9 ± 1.0% (n.s.); sham-operated + OXYANT group: 11.4 ± 0.8%, infarcted + OXYANT group 13.8 ± 0.4% (n.s.).
Figure 3 Mean maximal changes of MABP and HR from baseline after application of air jet stress in the sham-operated (open columns) and infarcted (filled columns) rats receiving ICV infusions of 0.9% NaCl, OXY or OXYANT. Number of rats per group: sham-operated 0.9%: n = 7; sham-operated OXY: n = 6; sham-operated OXYANT: n = 7; infarcted 0.9% NaCl: n = 8; infarcted OXY: n = 6; infarcted OXYANT: n = 6. Asterisks indicate significant differences between the experimental groups *P < 0.05; **P < 0.01; ***P < 0.001.
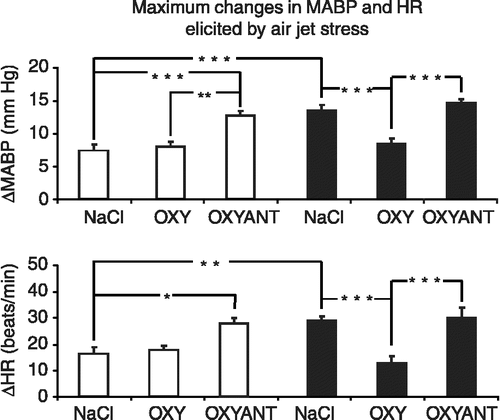
Similarly, significant differences were found in maximum changes of HR after application of the air jet stressor [F(5,35) = 9.676; P < 0.001]. Differences between the individual groups are shown in . In rats given ICV vehicle, the air-jet stressor increased the maximal change in MABP more in infarcted rats than in sham-operated controls (P < 0.01); ICV oxytocin reduced the maximum increase in MABP in the infarcted, but not the sham-operated rats (P < 0.001); in contrast, ICV OXYANT increased the maximum increase in MABP in the sham rats, but not in the infarcted rats (P < 0.05). The maximum increases in HR expressed as percentage of baseline HR in the individual groups of rats amounted to: 4.4 ± 0.7% in the sham-operated controls, 7.6 ± 0.4% in the infarcted controls (P < 0.01); 4.8 ± 0.4% in the sham operated oxytocin-treated, 3.8 ± 0.6% in the infarcted oxytocin-treated group (n.s.); 6.8 ± 0.4% in the sham-operated OXYANT group, and 7.9 ± 1.5% in the infarcted OXYANT group (n.s.).
Discussion
The present investigation provides strong evidence for a significant role of centrally released oxytocin in the control of cardiovascular responses to an acute mild stressor and for impairment of this function after a myocardial infarction. Specifically, it is demonstrated that (1) blockade of oxytocin receptors in the brain of the control rats significantly enhances blood pressure and HR elevations evoked by an unexpected stressor, (2) administration of the same dose of antagonist does not modify the cardiovascular responses to the stressor in the infarcted rats and (3) administration of exogenous oxytocin at a dose which does not modulate function of the cardiovascular system in the sham-operated rats effectively reduces the exaggerated cardiovascular responses to stress in the infarcted rats.
Buffering role of endogeneous oxytocin
Oxytocin acting centrally has been previously described as an important anxiolytic peptide (Windle et al. Citation2006; Blume et al. Citation2008). It has been also demonstrated that it reduces the hypothalamic-pituitary-adrenal axis responses to stress (Windle et al. Citation1997; Neumann et al. Citation2000). Our finding showing that oxytocin buffers the cardiovascular responses to stress uncovers another previously unknown function of this peptide. Namely, the results with central blockade of oxytocin receptors indicate that centrally released endogenous oxytocin significantly attenuates the intensity of the cardiovascular responses to acute stress in intact rats. It is also shown that the role of endogenous oxytocin in buffering the cardiovascular responses to stress is impaired in rats after myocardial infarction.
Interestingly, our results demonstrate that although the cardiovascular responses to stress are significantly enhanced in the infarcted rats they are not further elevated by blockade of oxytocin receptors. Reduction of cardiovascular responses to stress in the infarcted rats by centrally applied exogenous oxytocin strongly suggests that the lack of effects observed from oxytocin receptor blockade on the cardiovascular responses to stress during the post-infarct state is mainly caused by an inadequate release of oxytocin in the brain. The apparent impairment of the buffering role of oxytocin during the post-infarct state is obviously an unfavourable factor. Sudden stress causes significant acceleration of the HR, cardiac contractility and total peripheral resistance (Zhang et al. Citation1999). Consequently, cardiac work and oxygen consumption may markedly increase and current clinical studies in the literature frequently demonstrate a coincidence of exposure to a sudden stress with serious cardiovascular complications (Clarke et al. Citation2000; Kario et al. Citation2003).
Effects of exogenous oxytocin
In the present study, ICV infusion of oxytocin at the rate of 100 ng/h did not evoke significant changes of arterial blood pressure and HR under resting conditions. Previous studies assessing the role of centrally released oxytocin in the regulation of arterial blood pressure and HR provided controversial results. In a study performed on anaesthetised rats, Tran et al. (Citation1982) demonstrated that the intracisternal injection of oxytocin (1–10 mU/kg) elicited significant pressor responses not associated with any changes of HR. Alternatively, Feuerstein et al. (Citation1984) reported that ICV injections of 0.15, 1.0 or 10 nmol of oxytocin did not produce significant changes of blood pressure, however, higher doses elicited significant tachycardia. In contrast, some investigators provided evidence that oxytocin stimulates cardiovascular neurones responsible for hypotensive responses. For instance, Versteeg et al. (Citation1983) reported that oxytocin blunted the pressor component of the neurogenic hypertension evoked by electrical stimulation of the mesencephalic reticular formation. Oxytocin was also found to stimulate neurones of the dorsal vagal complex (Charpak et al. Citation1984), enhance baroreflex bradycardia and reduce exercise-induced cardioacceleration (Michelini Citation2001; Higa et al. Citation2002). Recently, Petersson and Uvnäs-Moberg (Citation2007) reported that repeated ICV injections of 300 ng of oxytocin for 5 days significantly reduced arterial blood pressure in Sprague Dawley rats. Thus, it seems that the final effect of oxytocin depends on several factors such as the dose, mode and site of application, presence of anaesthesia or hypertension. The rate of ICV infusion of oxytocin used in the present study (100 ng/h) may be considered to be relatively low in comparison to the doses administered by other authors. Oxytocin was infused at this rate in order to match the rate of vasopressin infusion that has been used in our previous investigations (Dobruch et al. Citation2005; Cudnoch-Je˛drzejewska et al. Citation2008). Therefore, it cannot be excluded that a higher dose of oxytocin would affect function of the cardiovascular system under baseline conditions. It is important that in the infarcted rats ICV infusion of oxytocin at the same rate (100 ng/h) effectively reduced the cardiovascular responses to the acute stressor. This finding suggests that there is some deficit of endogenous oxytocin in the brain during the post-infarct state and at the same time indicates that the pool of the cardiovascular neurones engaged in regulation of the pressor and tachycardic responses to stress might be different from that involved in regulation of arterial blood pressure and HR under resting conditions.
Possible interaction of oxytocin and vasopressin
Because vasopressin V1 receptors antagonists are not entirely specific and show some affinity to oxytocin receptors, it is sometimes claimed that the effects of blockade of V1 receptors result from the blockade of oxytocin receptors. The present study does not support such a possibility with regard to the cardiovascular responses to stress. The OXYANT used in the present study has much greater affinity to oxytocin ( pA2 = 7.37 ± 0.07) than to vasopressin V1 ( pA2 = 5.39 ± 0.04) and V2 ( pA2 < 5.5) receptors (Manning et al. Citation1995). Besides, administration of the OXYANT {DesGly-NH2-d(CH2)5[D-Tyr2,Thr4]OVT} produced opposite effects to those observed after administration of antagonist for V1 receptors in our previous studies (Dobruch et al. Citation2005; Cudnoch-Je˛drzejewska et al. Citation2007). Similarly, oxytocin produced opposite effects to vasopressin (Dobruch et al. Citation2005; Cudnoch-Je˛drzejewska et al. Citation2008; Stojicić et al. Citation2008). For instance, blockade of oxytocin receptors enhanced the pressor and tachycardic responses to stress in the sham-operated rats while being ineffective in the infarcted rats, whereas blockade of V1 receptors with d(CH2)5Tyr(Me)2,Ala-NH29]AVP was not effective in the sham-operated rats but it markedly strengthened the pressor and tachycardic responses to stress during the post-infarct state (Dobruch et al. Citation2005; Cudnoch-Je˛drzejewska et al. Citation2007). Thus, it may be concluded that the effects observed in the present study after administration of OXYANT or oxytocin were specifically caused by an interaction with oxytocin receptors. However, it cannot be excluded that oxytocin and vasopressin can mutually interact at the receptor level. Such interaction could be especially important during enhanced release of one or both of these peptides.
Summary and perspectives
Our results suggest that centrally released oxytocin protects against excessive increases of blood pressure and HR during sudden stress. The buffering function of this endogenous oxytocin appears to be significantly reduced during the post-infarct state. Previous studies demonstrated that exaggerated stimulation of the cardiovascular system by air jet stress in rat models of coronary disease is caused by enhanced stimulation of AT1 angiotensin and V1 vasopressin receptors (Zhang et al. Citation1999; Dobruch et al. Citation2005; Cudnoch-Je˛drzejewska et al. Citation2007). The present study reveals that an inadequate action of the endogenous oxytocin system in the brain may also contribute to this phenomenon. In the present study, we administered ICV infusions of only single doses of oxytocin and OXYANT. Therefore, we cannot exclude that administration of higher doses of these compounds could elicit some additional central cardiovascular effects, for instance change in the resting blood pressure or HR. Our study opens several new questions regarding engagement of oxytocin in adjustment of the cardiovascular system to other types of stress, including chronic stress, as well as possibility of use of oxytocin agonists for treatment of exaggerated cardiovascular responses to stress.
Acknowledgements
The authors wish to express their gratitude to Professor Maurice Manning from the Department of Biochemistry and Molecular Biology, Medical College of Ohio, Toledo, USA for the generous supply of oxytocin antagonist used in the present study, to Marzanna Tkaczyk for her skilful technical assistance, and to Marcin Kumosa for preparation of illustrations. This study was supported by a grant from the Medical University of Warsaw (1MAW2/2006-2008).
Declaration of interest: The authors report no conflicts of interest. The authors alone are responsible for the content and writing of the paper.
References
- Barberis C, Tribollet E. 1996. Vasopressin and oxytocin receptors in the central nervous system. Crit Rev Neurobiol. 10:119–154.
- Bergquist F, Ludwig M. 2008. Dendritic transmitter release: A comparison of two model systems. J Neuroendocrinol. 20:677–686.
- Blume A, Bosch OJ, Miklos S, Torner L, Wales L, Waldherr M, Neumannn ID. 2008. Oxytocin reduces anxiety via ERK1/2 activation: Local effect within the rat hypothalamic paraventricular nucleus. Eur J Neurosci. 27:1947–1956.
- Braga DC, Mori E, Higa KT. 2000. Central oxytocin modulates exercise-induced tachycardia. Am J Physiol Regul Integr Comp Physiol. 278:R1474–R1482.
- Carter CS, Grippo AJ, Pournajafi-Nazarioo M, Ruscio MG, Porges SW. 2008. Oxytocin, vasopressin and sociality. Progr Brain Res. 170:331–336.
- Charpak S, Armstrong WE, MŰhlethaler M, Dreifuss JJ. 1984. Stimulatory action of oxytocin on neurones of the dorsal motor nucleus of the vagus nerve. Brain Res. 300:83–89.
- Choleris E, Little SR, Mong JA, Puram SV, Langer R, Pfaff DW. 2007. Microparticle based delivery of oxytocin receptor antisense DNA in the medial amygdala blocks social recognition in female mice. Proc Natl Acad Sci USA. 104:4670–4675.
- Clarke SP, Frasure-Smith N, Lesperance F, Bourassa MG. 2000. Psychosocial factors as predictors of functional status at 1 year patients with left ventricular dysfunction. Res Nurs Health. 23:290–300.
- Costa e Sousa RH, Pereira-Junior PP, Oliveira PF, Olivares EL, Werneck de Castro JPS, Mello DB, Nascimento JHM, Campos de Carvalho AC. 2005. Cardiac effects of oxytocin: Is there a role for this peptide in cardiovascular homeostasis?. Regul Pept. 132:107–112.
- Cudnoch-Je˛drzejewska A, Dobruch J, Puchalska L, Szczepanska-Sadowska E. 2007. Interaction of AT1 receptors and V1a receptors-mediated effects in the central cardiovascular control during the post-infarct state. Regul Pept. 142:86–94.
- Cudnoch-Je˛drzejewska A, Szczepanska-Sadowska E, Dobruch J, Puchalska L, Ufnal M, Kowalewski S, Wsół A. 2008. Differential sensitisation to central effects of angiotensin II in rats with a myocardial infarct: Relevance to stress and interaction with vasopressin. Stress. 11:290–301.
- Curran-Everet D, Benos DJ. 2004. Guidelines for reporting statistics in journals published by the American Physiological Society. Physiol Genom. 18:249–251.
- Dobruch J, Cudnoch-Jędrzejewska A, Szczepańska-Sadowska E. 2005. Enhanced involvement of brain vasopressin V1 receptors in cardiovascular responses to stress in rats with myocardial infarction. Stress. 8:273–284.
- Engelmann M, Ebner K, Landgraf R, Hoisboer F, Wotjak CT. 1999. Emotional stress triggers intrahypothalamic but not peripheral release of oxytocin in male rats. Neuroendocrinol. 11:867–872.
- Feuerstein G, Zerbe RL, Faden AI. 1984. Central cardiovascular effects of vasotocin, oxytocin and vasopressin in conscious rats. J Pharmacol Exp Ther. 228:348–353.
- Higa KT, Mori E, Viana FF, Morris M, Michelini LC. 2002. Baroreflex control of heart rate by oxytocin in the solitary-vagal complex. Am J Physiol Regul Integr Comp Physiol. 282:R537–R545.
- Kario K, McEwen BS, Pickering TG. 2003. Disasters and the heart: A review of the effects of earthquake induced stress on cardiovascular disease. Hypertens Res. 26:355–367.
- Lampert R, Jain D, Burg MM, Batsford WP, McPherson CA. 2000. Destabilizing effects of mental stress on ventricular arrhythmia in patients with implantable cardioverter-defibrillators. Circulation. 101:158–164.
- Leng G, Caquineau C, Sabatier N. 2005. Regulation of oxytocin secretion. Vitam Horm. 71:27–58.
- Ludbrook J. 1994. Repeated measurements and multiple comparisons in cardiovascular research. Cardiovasc Res. 28:303–311.
- Manning M, Miteva K, Pancheva S, Stoyev S, Wo NC, Chan WY. 1995. Design and synthesis of highly selective in vitro and in vivo uterine receptors antagonists of oxytocin: Comparisons with Atosiban. Int J Peptide Protein Res. 46:244–252.
- Mayorov DN, Head GA. 2003. AT1 receptors in the RVLM mediate pressor responses to emotional stress in rabbits. Hypertension. 41:1168–1173.
- Mayorov DN, Head GA, De Matteo R. 2004. Tempol attenuates excitatory actions of angiotensin II in the rostral ventrolateral medulla during emotional stress. Hypertension. 44:101–106.
- McCann SM, Antunes-Rodrigues J, Jankowski M, Gutkowska J. 2002. Oxytocin, vasopressin and atrial natriuretic peptide control body fluid homeostasis by action on their receptors in brain, cardiovascular system and kidney. Prog Brain Res. 139:309–326.
- Michelini LC. 2001. Oxytocin in the NTS. A new modulator of cardiovascular control during exercise. Ann NY Acad Sci. 940:206–219.
- Morris M, Ross J, Sundberg DK. 1985. Catecholamine biosynthesis and vasopressin and oxytocin secretion in the spontaneously hypertensive rat: An in vitro study of localized brain regions. Peptides. 6:949–955.
- Möhring J, Schoun J, Kintz J, Robinson IC, McNeill JR. 1983. Vasopressin and oxytocin content are decreased in the brain stems of spontaneously hypertensive rats. Neuroendocrinology. 36:457–461.
- Nakashima T, Nogfuchi T, Furukawa T, Yamasaki M, Makino S, Miyata S, Kiyohara T. 2002. Brain oxytocin augments stress-induced long-lasting plasma adrenocorticotropic hormone elevation in rats. Neurosci Lett. 22:161–164.
- Neumann ID. 2008. Brain oxytocin: A key regulator of emotional and social bevavior in both females and males. J Neuroendocrinol. 20:858–865.
- Neumann JD, Krömer SA, Toschi N, Ebner K. 2000. Brain oxytocin inhibits the (re)activity of the hypothalamo-pituitary-adrenal axix in male rats: Involvement of hypothalamic and limbic brain regions. Regul Pept. 96:31–38.
- Petersson M, Alster P, Lundeburg T, Uvnäs-Moberg K. 1996. Oxytocin causes a long time decrease of blood pressure in female and male rats. Physiol Behav. 60:1311–1315.
- Petersson M, Uvnäs-Moberg K. 2007. Effects of an acute stressor on blood pressure and heart rate in rats pretreated with intracerebroventricular oxytocin injections. Psychoneuroendocrinology. 32:959–965.
- Robinson DA, Wei F, Wang GD, Li P, Kim SJ, Vogt SK, Muglia LJ, Zhuo M. 2002. Oxytocin mediates stress-induced analgesia in adult mice. J Physiol. 540:593–606.
- Russell JA, Leng G, Douglas AJ. 2003. The magnocellular oxytocin system, the fount of maternity: Adaptations in pregnancy. Front Neuroendocrinol. 24:27–61.
- Sawchenko PE, Swanson LW. 1982. Immunochistochemical identification of neurons in the paraventricular nucleus of hypothalamus that project to the medulla or to the spinal cord in the rat. J Comp Neurol. 205:260–272.
- Selye H, Bajusz E, Grasso S, Mendell P. 1960. Simple techniques for the surgical occlusion of coronary vessels in the rat. Angiology. 11:398–407.
- Sofroniew MV, Schrell U. 1981. Evidence for direct projections from oxytocin and vasopressin neurons in the hypothalamic paraventricular nucleus in the medulla oblongata: Immunohistochemical visualization of both the horseradish peroxidase transported and peptide produced by the same neurons. Neurosci Lett. 22:211–217.
- Stojicić S, Milutinović-Smiljanić S, Sarenac O, Milosavljević S, Paton JF, Murphy D, Japundić-Zigon N. 2008. Blockade of central vasopressin receptors reduces the cardiovascular responses to acute stress in freely moving rats. Neuropharmacology. 54:824–836.
- Szczepanska-Sadowska EK, Wsol A, Kowalewski S, Cudnoch-Jędrzejewska A. 2008. Oxytocin reduces pressor and tachycardic response to the alarming stress in the infarcted rats. FASEB J. 22:952.2.
- Tran LD, Montastruc JL, Montastruc P. 1982. Effects of lysine-vasopressin and oxytocin on central cardiovascular control. Br J Pharmacol. 77:69–73.
- Versteeg CA, Cransberg K, De Jong W, Bohus B. 1983. Reduction of centrally induced pressor response by neurohypophyseal peptides: The involvement of lower brainstem mechanisms. Eur J Pharmacol. 94:133–140.
- Windle RJ, Shanks N, Lightman SL, Ingram CD. 1997. Central oxytocin administration reduces stress-induced corticosterone release and anxiety behavior in rats. Endocrinology. 138:2829–2834.
- Windle RJ, Gamble LE, Kershaw YM, Wood SA, Lightman SL, Ingram CD. 2006. Gonadal steroid modulation of stress-induced hypothalamo-pituitary-adrenal activity and anxiety behavior: Role of central oxytocin. Endocrinology. 147:2423–2431.
- Zhang W, Huang BS, Leenen FH. 1999. Brain renin-angiotensin system and sympathetic hyperactivity in rats after myocardial infarction. Am J Physiol Heart Circ Physiol. 276:H1608–H1615.