Abstract
Mice subjected to restraint stress (RRS) daily for 3 days lose weight. Once stress ends they are slow to recover the weight loss and exhibit increased anxiety and hypothalamus–pituitary–adrenal (HPA) activity in response to novel stressors. We tested the effect of RRS in mice deficient in corticotropin releasing factor receptor one (CRFR1-KO) or two (CRFR2-KO). Wild type (WT) and CRFR2-KO, but not CRFR1-KO, mice lost weight during RRS. All adrenalectomised mice lost weight and CRFR2-KO controls stopped gaining weight on the days of RRS. WT RRS mice returned to the weight of their controls 8 days after restraint. CRFR2-KO mice showed high levels of anxiety in an elevated plus maze (EPM) 11 days after RRS and in a light/dark choice test 14 days after RRS. CRFR1-KO mice displayed low anxiety in both tests, but RRS decreased EPM exploration. By contrast, exploration increased in RRS ADX mice. Testing in the EPM increased serum corticosterone level in all WT and CRFR2-KO mice. Corticosterone increased in RRS CRFR1-KO mice compared with their controls. These results suggest that CRFR1 are required for stress-induced weight loss, but that hyper-reactivity of the HPA axis in RRS mice exposed to a subsequent novel stress is independent of CRFR1.
Introduction
Rats and mice exposed to restraint stress (RRS) on three consecutive days lose weight and maintain a lower body weight than their non-stressed controls for extended periods of time (Harris et al. Citation1998; Chotiwat and Harris Citation2006). In rats, the weight loss is associated with both an inhibition of food intake and an increase in energy expenditure on the days of restraint, but energy intake and expenditure rapidly return to control levels once stress ends (Harris et al. Citation2006). In addition to the chronic change in body weight, rats and mice that have been subjected to RRS demonstrate increased anxiety behavior in non-stressed conditions and increased endocrine responsiveness to novel stressors in the post-stress period (Harris et al. Citation2004; Chotiwat and Harris Citation2006). The increased sensitivity of the endocrine response to novel stress has been associated with an impaired ability of the glucocorticoids to suppress hypothalamic-pituitary–adrenal (HPA) axis activity and a lowering of the threshold for the initiation of endocrine responses to stress (Buwalda et al. Citation1999; Harris et al. Citation2004). This hyper-responsiveness is not limited to animals that have been subjected to restraint stress as others have reported increased stress responsiveness in rats that had previously been subjected to foot shock (Caggiula et al. Citation1989).
Corticotropin releasing factor (CRF) and urocortin proteins are considered to be primary initiators of stress-induced changes in endocrine, physiological, and behavioral status (Koob et al. Citation1993; Bale and Vale Citation2004). These neuropeptides activate CRF receptors that are widely distributed in both the periphery and central nervous system (Van Pett et al. Citation2000). There are two major subtypes of CRF receptors that show some specificity in the pathways that they activate. CRF receptor one (CRFR1) appears to be primarily responsible for activating the HPA axis and for inducing anxiety-type behaviors in rodents (Contarino et al. Citation1999). It also mediates an acute inhibition of food intake in stressed animals (Sekino et al. Citation2004). In contrast, CRFR2 is responsible for a delayed inhibition of food intake (Sekino et al. Citation2004) in stressed animals by decreasing both meal frequency and meal size (Fekete et al. Citation2007), a down-regulation of thermogenesis (Carlin et al. Citation2006) possibly as a result of control over sympathetic tone (Bale et al. Citation2003), and it also may have anxiolytic properties, dampening the effects of CRFR1 activation on stress-induced behaviors (Bale et al. Citation2000) and modifying serotonin transport (McEuen et al. Citation2009). The objective of the studies described here was to determine the importance of the two different CRF receptor subtypes in mediating the changes in body weight, food intake, and sensitivity to novel stress in mice that had been subjected to RRS. We used two genotypes of mice in which either CRFR1 or CRFR2 had been deleted. Previous studies with these animals show that the CRFR1-deplete (CRFR1-KO) mice display reduced levels of anxiety and a blunted hypothalamus–pituitary–adrenal (HPA) activation in response to stress (Smith et al. Citation1998; Timpl et al. Citation1998). By contrast the CRFR2-deplete (CRFR2-KO) mice, especially females (McEuen et al. Citation2009), are hyper-responsive to stress and show increased anxiety behaviors (Bale et al. Citation2000). Although studies on knockout (KO) mice may be criticized for the potential confounds of developmental compensation modifying the mouse phenotype (Inui Citation2000), they are nonetheless useful when trying to determine the importance of a specific protein in mediating a response when the specific site of activity is unknown. In this study, we anticipated that a comparison of the response of these two lines of KO mice to RRS would elucidate the importance of each receptor subtype in the different aspects of response to RRS. In previous studies with rats we have been able to modify the decrease in food intake or the weight loss of RRS rats using third ventricle infusions of antagonists to CRFR1 or CRFR2 (Chotiwat and Harris Citation2008). In these studies some aspects of the RRS phenotype, such as the change in food intake or the prolonged down-regulation of body weight, were blocked but acute changes in body weight and activation of the HPA axis by RRS were not changed, suggesting a diverse anatomical distribution of the receptors responsible for initiation of different aspects of the chronic response to RRS. The knockout mice used in the studies described here allow us to evaluate the importance of CRFR1 and CRFR2 in the RRS-induced changes in energy balance and HPA activity, although we are not able to locate the site of receptor activation.
The experimental design used here is similar to one used previously to test the effects of RRS on body weight, food intake, and anxiety-type behavior of NIH Swiss mice (Chotiwat and Harris Citation2006). That study showed that in the weeks following the end of RRS, the mice that had been exposed to RRS displayed increased anxiety in the elevated plus maze (EPM) and light dark choice test (L/D choice; Chotiwat and Harris Citation2006). These behavioral tests are frequently used as a measure of stress-responsiveness in rodents (MacNeil et al. Citation1997; Harris et al. Citation2001; Morilak et al. Citation2003). The EPM relies on a balance between the desire of a rodent to explore novel areas and a natural aversion to illuminated, open spaces (Crawley Citation1985). The reluctance of an animal to enter the open arms of an EPM is considered a fear-motivated behavior (Trullas and Skolnick Citation1993). In the L/D choice test, time spent in the dark represents avoidance of the aversive, light, chamber whereas transitions between chambers may represent attempts to escape a novel environment (Misslin et al. Citation1989). Serum corticosterone level measured after testing in the EPM also was higher in mice that had previously been restrained than in their non-stressed controls, confirming our previous observations that RRS rats have an exaggerated HPA response to mild stressors in the post-restraint period (Harris et al. Citation2004). Therefore, by using the same test conditions for mice that either do not express CRFR1 or do not express CRFR2 we aimed to determine which receptor subtype was responsible for the sustained changes in body weight, behavior, and endocrine status in RRS mice and whether each of these responses was likely to be processed through the same or different receptor-mediated pathways.
Materials and methods
The mice used in these studies were obtained from breeding colonies maintained at the University of Georgia and all animal procedures were carried out in accordance with the National Institute of Health Guide for Care and Use of Laboratory Animals and were approved by the Institutional Animal Care and Use Committee of the University of Georgia. Mice had free access to mouse chow (Mouse Chow 5012: PMI International, Brentwood, MO, USA) and water and were housed in a humidity- and temperature-controlled room on a 12 h:12 h light:dark cycle with lights on at 07.00 h. Two male and two female heterozygote CRFR1 knockout (CRFR1-KO) mice were purchased from Jackson Labs (B6;129-Crhr1tm1Klee/J: Bar Harbor MN) and two male and two female heterozygote CRFR2-KO mice were a generous gift from Dr Tracy L. Bale, Department of Animal Biology, University of Pennsylvania. These mice were initially bred with C57BL/6J mice and subsequent generations were obtained by breeding heterozygotes. Male offspring of the F5 generation were used for studies described here. No more than one wild type (WT) mouse from each litter was included in each treatment group, KO mice were evenly distributed between restrained and control groups, described below. The yield of male CRFR1-KO mice was low (18 from 45 litters) compared with that of male CRFR2-KO mice (22 from 12 litters).
Mice were genotyped by PCR. At 10 days of age unanesthetized pups were toe-clipped both for identification and to provide tissue for genotyping. Toes from CRFR1 mice were digested by the HotSHOT method (Truett et al. Citation2000). Aliquots of DNA were amplified in two separate 25 μl PCR reactions using PCR Supermix (Invitrogen, Carlsbad, CA, USA) and primers were included at a final concentration of 200 nM. The first reaction amplified a 200 bp WT sequence and the second amplified a 400 bp knockout sequence. Amplification conditions were an initial 4 min denaturation at 94°C and 40 cycles of 94°C for 30 s, 50°C for 45 s, and 72°C for 45 s. The final extension was 3 min at 72°C. Toes from CRFR2 mice were digested overnight at 55°C with Proteinase K. A single reaction was used to amplify a 500 bp WT sequence and a 400 bp knockout sequence (Gammie et al. Citation2005). Amplification conditions were an initial 4 min denaturation at 94°C and 40 cycles of 94°C for 1 min, 61°C for 1 min, and 72°C for 40 s. The final extension was 3 min at 72°C. Primer sequences for both genotyping procedures are in .
Table I. PCR Primers for genotyping.
Twenty-two CRFR2-KO male mice, 24 of their WT male littermates, 18 CRFR1-KO male mice, and 38 of their WT male littermates were included in the study. Eighteen of the WT mice from the CRFR1 colony were adrenalectomized at 5 weeks of age and subsequently had free access to water containing 0.9% NaCl and 25 mg/l corticosterone. Immediately before surgery and 24 h after surgery each mouse received a subcutaneous injection of ketophen (2 mg/kg) analgesic. Mice were anesthetized with isoflurane and adrenal glands were removed through small dorsal incisions on either side of the spine. Incisions of muscle and skin on each side were sutured closed. This group of mice controlled for the adrenal insufficiency of the CRFR1-KO mice. The lack of corticosterone response to the behavioral tests (see below) was used as confirmation of the completeness of adrenalectomy.
Starting at 7 weeks of age the mice were housed in individual cages with grid floors. Food intake, corrected for spillage, and body weight were measured daily at 08.00 h. After 7 days the mice within each treatment group (WT, ADX CRFR2-KO, and CRFR1-KO) were divided into two weight-matched subgroups. One subgroup was subjected to 2 h of RRS on each morning for three consecutive days (RRS) starting at 09.00 h. Mice were restrained in Perspex restraining tubes (9.5 × 2.5 cm, Plas-Labs, Lansing, MI, USA) in an experimental room. Control mice were placed in shoebox cages in the same experimental room and all animals were deprived of food and water during the 2 h of restraint. After restraint, the mice were returned to their home cages and given free access to chow and water. Food intakes were recorded for 8 days following RRS and body weights were recorded for 11 days (day 13 of the experimental period).
Nine days after the end of RRS (day 11) mice were tested in an EPM. The EPM consisted of brightly illuminated 45 × 10 cm arms elevated 50 cm above the ground. Two opposing arms of the maze were painted black and enclosed by black walls 40 cm high. The other two opposing arms were painted white and had no walls. Others have shown that stressed animals show more reliable increases in anxiety-like behavior on the second exposure to the EPM (File and Gonzalez Citation1996; Hogg Citation1996), therefore, each mouse was given a 10 min acclimation period in the EPM the day before behavioral testing. At the start of the test the mouse was placed at the center of the maze facing an open arm. Behavior of the mice on the EPM was video-taped and subsequently scored for 5 min for the time spent in the open arms, enclosed arms, and the middle zone and the number of entries made into open arms, closed arms, and the middle zone. Immediately before placing the mouse in the EPM and 25 min following the start of testing approximately 20μl of blood was collected from a facial vein using Goldenrod lancets (Medipoint Inc., Mineola, NY, USA). Serum was analyzed for corticosterone concentration by radioimmunoassay (Corticosterone DA kit: MP Biomedicals, Solen, OH, USA). The within assay variation for this assay is 3.3% and between assay variation is 6.1%.
Twelve days after the end of RRS (day 14) the mice were tested in a light/dark choice procedure. The apparatus consisted of a 25.4 × 25.4 cm chamber with 40 cm-high clear acrylic walls and a black insert chamber (Coulbourn Instruments, Allentown, PA, USA) occupying half of the area. The mice had access to the dark area via a small hole cut into the side of the insert. The floor of the chamber not covered by the insert was painted white and illuminated by bright, direct lighting. On the day of testing, the mice were placed in the white chamber of the box facing the opening to the dark chamber and activity was recorded for 5 min. Others have reported no effect of whether the starting chamber is light or dark on time spent within the dark chamber or number of transitions between chambers (Misslin et al. Citation1989). Behavior was scored for number of transitions made between chambers and total time in each chamber.
Statistical analysis
Initially data from CRFR2-KO and their WT controls were analyzed separately from those of CRFR1-KO mice, WT controls, and the ADX mice. Because there were no differences between the WT controls from the two different colonies all of the WT mice were incorporated into one treatment group and all four treatments (WT, ADX, CRFR1-KO, CRFR2-KO) were compared in one analysis. Body weight change, food intake, and serum corticosterone concentrations were compared using two-way repeated measure ANOVA (Statistica, Stat Soft, Tulsa, OK, USA) with treatment and RRS as dependent variables. Baseline body weight was used as a covariate when comparing the weight changes of the groups. Two-way ANOVA was used to analyze behavioral data. Differences between specific groups were determined by post hoc Duncan's multiple range test. Differences were considered significant at P < 0.05.
Results
The ADX mice weighed less than any other treatment group, but there were no significant differences in the food intake of any of the groups during the first 10 days of the experimental period when all groups of mice were compared (). When weight change of all of the groups was compared, there were significant interactions between treatment and time, and between stress and time (: F(df), P treatment: NS; stress: NS; time: 93(12), P < 0.0001, treatment × time: 2.5(36) P < 0.0001; stress × time: 6.4(12) P < 0.0001; treatment × stress × time: NS). Both RRS WT and RRS CRFR2-KO mice lost weight on the days of restraint, and control CRFR2-KO mice did not gain weight on the days of restraint, therefore weight gain was similar for RRS and control CRFR2-KO mice on all but day 5. By contrast, the RRS WT mice gained less weight than their controls up to day 10, 8 days post-restraint. Both Control and RRS ADX lost a small amount of weight on the days of restraint, but there was no difference in weight gain of the two groups during the experimental period. The RRS CRFR1-KO mice did not lose weight on the days of restraint and they tended to gain more weight than their controls in the post-stress period although the difference in weight gain was significant only on day 11.
Table II. Body weight and food intakes.
Figure 1. Change in body weight from the morning before the first restraint (day 0). Data are mean ± SEM for groups of 9 to 22 mice. The WT mice represent combined groups of WT from CRFR1-KO and CRFR2-KO colonies. Asterisks indicate days on which there was a significant difference in weight change between Control and RRS mice. ADX, adrenalectomised and corticosterone replacement; CRFR, corticotropin releasing factor receptor; EPM, elevated plus maze exposure; KO, gene deletion; L/D, light/dark choice test; RRS, daily restraint stress for 3 days; WT, wild type.
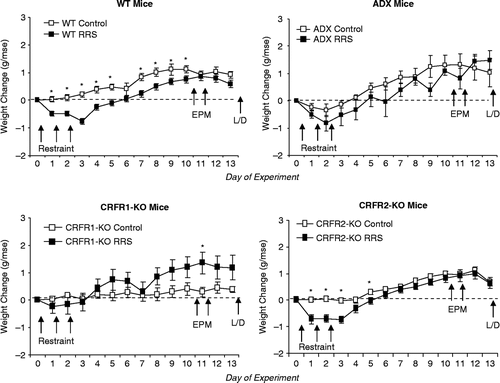
Food intakes of the mice were compared over 3 day blocks, before, during, and after RRS (). When all treatments were compared over all time blocks, there was no significant effect of treatment or of stress on intake. When different treatments were considered individually, then the food intake of RRS WT mice was inhibited on the days of restraint compared with their controls. RRS ADX mice ate a similar amount as their controls on the days of stress, but ate more in the 3 days immediately after the end of restraint. There were no differences in food intakes of Control and RRS CRFR1-KO or CRFR2-KO mice during any of the time blocks.
Figure 2. Food intake of the mice represented as total intake for 3 days before, during, and after RRS. The asterisk indicates a significant difference (P < 0.05) in intake (g/mse[mouse]) of Control versus RRS mice. Data are mean ± SEM for groups of 9–22 mice. ADX, adrenalectomised and corticosterone replacement; CRFR, corticotropin releasing factor receptor; KO, gene deletion; RRS, daily restraint stress for 3 days; WT, wild type.
![Figure 2. Food intake of the mice represented as total intake for 3 days before, during, and after RRS. The asterisk indicates a significant difference (P < 0.05) in intake (g/mse[mouse]) of Control versus RRS mice. Data are mean ± SEM for groups of 9–22 mice. ADX, adrenalectomised and corticosterone replacement; CRFR, corticotropin releasing factor receptor; KO, gene deletion; RRS, daily restraint stress for 3 days; WT, wild type.](/cms/asset/21466aef-bdb4-4085-a413-b8243e4884b0/ists_a_420925_f0002_b.gif)
There were significant effects of treatment and of previous exposure to RRS on the behavior of the mice in the EPM (). There was a significant effect of treatment (F 8, df 12, P < 0.001) on all of the parameters measured; stress had a significant effect on the number of entries into the middle zone (F 13, df 3, P < 0.03) and there was a significant interaction between treatment and stress (F 2.9, df 3, P < 0.04) for time spent in the middle zone and for the number of entries into the open arms. The CRFR2-KO mice exhibited the highest level of anxious behavior, spending almost all of the 5 min test period in the closed arms and making few entries into the middle zone or open arms. There was no significant effect of RRS on any of the measures. The CRFR1-KO mice exhibited the lowest level of anxious behavior of all of the treatment groups, spending the most time in the open arms and the least time in the closed arms. These mice also made a large number of entries into the middle zone, although most of these led to a return to the closed arm of the maze, rather than an entry into an open arm. Previous exposure to RRS significantly inhibited the exploratory behavior of these mice as they made fewer entries into the middle zone and into the open and closed arms of the maze and they spent significantly less time in the middle zone than their controls. WT mice exhibited less anxiety-type behavior than the CRFR2-KO, but more than the CRFR1-KO mice and there was no effect of previous exposure to restraint stress on their activity in the EPM. In contrast to the other groups, previous exposure to RRS tended to increase exploratory behavior of ADX mice, suggesting a reduced level of anxiety compared with control ADX mice. RRS increased the number of entries into the open arms and the time spent in the middle zone and reduced the amount of time spent in closed arms of the maze.
Figure 3. Behavior measured during 5 min in the elevated plus maze (EPM) on day 11 of the experimental period, 9 days after the end of daily RRS for 3 days (RRS). Values on any specific axis that do not share a common superscript are significantly different at P < 0.05. Data are mean ± SEM for groups of 9–22 mice. ADX, adrenalectomised and corticosterone replacement; CRFR, corticotropin releasing factor receptor; KO, gene deletion; WT, wild type.
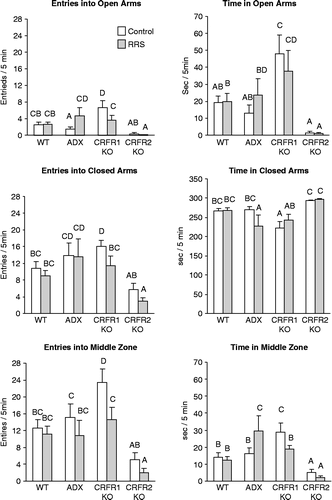
There was a significant effect of treatment (F 10, df 3, P < 0.002) on all three measures of behavior in the L/D choice test, but no effect of stress and no interaction between stress and treatment for any of the measurements (). The behavior of CRFR2-KO mice was not different from that of WT mice, but they crossed between light and dark chambers less frequently than ADX or CRFR1-KO mice, and spent more time in the dark chamber and less time in the light chamber than the ADX or CRFR1-KO mice. RRS ADX mice spent less time in the dark chamber and more time in the light chamber than WT mice, but there were no other differences in behavior of the mice.
Figure 4. Behavior measured in the light/ dark (L/D) choice test on day 14 of the experimental period, 12 days after the end of daily restraint for 3 days (RRS). Values on an axis that do not share a common superscript are significantly different at P < 0.05. Data are mean ± SEM for groups of 9–22 mice. ADX, adrenalectomised and corticosterone replacement; CRFR, corticotropin releasing factor receptor; KO, gene deletion; WT, wild type.
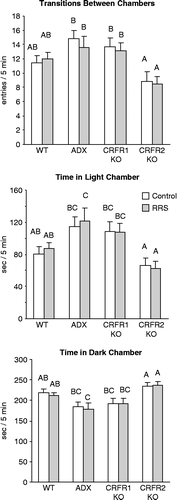
There were significant effects of treatment on both basal serum corticosterone concentration and on corticosterone measured after the mice had been tested in the EPM (: F(df) P: treatment: 8(3) P < 0.00001, RRS: NS; time: 81.5(1) P < 0.0001; treatment × time: 18.2(3) P < 0.001). Testing in the EPM did not increase corticosterone concentration in either the CRFR1-KO or ADX rats, but caused a significant stimulation of corticosterone release in both WT and CRFR2-KO mice. Basal (time 0) and stimulated corticosterone (time 25) were lowest in the CRFR1-KO mice, but were increased by previous exposure to RRS. There were no effects of previous exposure to RRS on either basal or stimulated corticosterone in any other group.
Figure 5. Serum corticosterone measured before (time 0) and 25 min (time 25) after the start of testing in the EPM on day 11 of the experimental period. Values that do not share a common superscript are significantly different at P < 0.05. ADX, adrenalectomised and corticosterone replacement; CRFR, corticotropin releasing factor receptor; CTRL, control; KO, gene deletion; RRS, daily restraint stress for 3 days; WT, wild type.
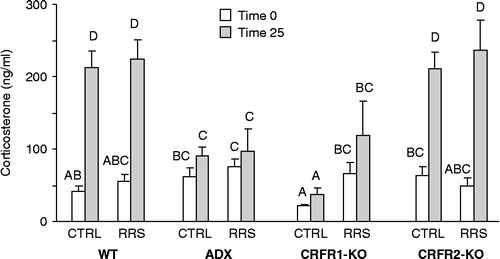
Discussion
The objective of this study was to determine whether the long-term effects of RRS on body weight, HPA activity, and anxiety-type behavior were dependent on the activation of CRFR1 or CRFR2. The results suggest that both CRFR1 and CRFR2 contribute to the long-term down-regulation of body weight in RRS mice and that stress-induced corticosterone release may also play an important role in stress-induced weight loss. The WT mice showed the expected weight loss on the days of restraint and RRS WT mice did not achieve the same weight gain as their controls until 8 days after RRS ended. These results are almost identical to those we reported previously for NIH Swiss mice subjected to RRS (Chotiwat and Harris Citation2006). Both ADX and CRFR1 KO mice had a limited HPA response to stress and CRFR1-KO mice did not lose significant amounts of weight in response to RRS, but all ADX mice lost some weight on the days of RRS. Therefore, it is unlikely that a stress-induced surge in corticosterone secretion can fully account for the acute weight loss that occurred in WT mice on the days of restraint, but it may contribute to the extended duration of reduced weight in WT RRS mice. Because CRFR1-KO mice did not lose weight initially, it is impossible to determine from this study whether CRFR1 are required for the prolonged inhibition of weight gain that is apparent in WT mice after RRS has ended. In a previous study with rats we showed that acute inhibition of CRFR1 immediately before stress did not prevent weight loss on the days of restraint, but did prevent the sustained reduction in weight of the rats (Chotiwat and Harris Citation2008). This effect of the CRFR1 antagonist antalarmin was observed both when it was administered peripherally and when it was infused into the third ventricle. One significant difference between the rat study and the experiment described here was a robust stress-induced release of corticosterone in the rats, providing further support for the requirement for a glucocorticoid surge in the mediation of the acute weight loss of stressed rodents. Thus, CRFR1 would be involved in the response to RRS at two levels, first by mediating the activation of the HPA axis which is involved in the acute loss of weight caused by RRS and second by initiating a series of events that leads to a chronic reduction in body weight once stress has ended.
In contrast to CRFR1-KO mice, the CRFR2-KO mice lost weight on the first day of restraint stress, but regained weight and weighed the same as their controls in the days immediately after RRS ended. The similarity in body weight of RRS and Control CRFR2-KO mice appears to have been due to the controls not gaining weight on the days after the restraint ended. These data confirm that CRFR1 are required for the acute weight loss experienced by stressed mice, but the exaggerated response of control CRFR2-KO mice to the procedures of being moved to a new cage without food or water during the period of restraint may have accounted for the inhibition of weight gain, making it difficult to determine whether CRFR2, either alone or in combination with CRFR1, are involved in the mechanisms that cause a sustained reduction in weight once stress has ended. We have previously reported that third ventricle infusions of the CRFR2 antagonist antisauvagine-30 in rats immediately before each restraint stress produce a change in weight gain of non-stressed controls which is identical to that of rats subjected to RRS (Chotiwat and Harris Citation2008). This was attributed to the inhibition of CRFR2 allowing increased activation of CRFR1 even in non-stressed rats (Chotiwat and Harris Citation2008). Pathways independent of CRFR1, but modulated by activation of CRFR2, such as the regulation of serotonin transport (McEuen et al. Citation2009), also may contribute to the increased stress-responsiveness of CRFR2 mice. Apart from the possible difference in studies due to species, the greatest difference between the rats and the mice in this experiment is that the mice did not express CRFR2 in any tissue and had potentially developed compensatory mechanisms for the deletion of these receptors. In the rat study CRFR2 were antagonized only immediately before the start of restraint and the inhibition of receptor activity was limited to brain regions in the vicinity of the third ventricle (Chotiwat and Harris Citation2008).
The weight loss of RRS WT mice was associated with a significant inhibition of food intake on the days of restraint and then a return of intake to control levels once stress ended. There was no effect of RRS on the food intake of CRFR2 mice, even though they lost weight on the days of restraint, suggesting a more important role for a stress-associated increase in energy expenditure in these animals. In RRS rats energy expenditure (Harris et al. Citation2006) and core temperature (Harris et al. Citation2002) are increased only during the time that they are in the restraint tubes. Others have reported that CRFR2-KO mice have high levels of brown fat thermogenesis and whole animal energy expenditure (Carlin et al. Citation2006) even in basal conditions, and the results from this study would suggest that the thermic response to stress also is exaggerated. Importantly, peripheral CRFR2 also are responsible for a stress-induced inhibition of gut motility (Stengel and Tache Citation2008), which could contribute to changes in food intake and energy balance of stressed animals. There was no effect of RRS on food intake of CRFR1-KO or ADX mice. Studies by others indicate that food intake is normal under basal conditions in both CRFR1-KO and CRFR2-KO mice (Bale et al. Citation2000; Bradbury et al. Citation2000), but experiments using receptor-specific antagonists show that CRFR1 are responsible for the immediate ( < 90 min), transient inhibition of food intake caused by stress or CRFR agonists, whereas CRFR2 mediate hypophagia starting an hour or more after administration of stress or CRFR agonists (Bradbury et al. Citation2000; Sekino et al. Citation2004). These changes in food intake are relatively short-lived compared with measures of intake recorded at 24 h intervals, as they were in this study. Thus, it is possible that there were transient changes in food intake of both CRFR1-KO and CRFR2-KO mice, but that these changes were not detectable over a 24 h period. Surprisingly, there also was no effect of RRS on food intake of ADX mice, suggesting a role for stress-induced corticosterone release in causing the hypophagia that was measurable in RRS WT mice. The effect of RRS on food intake in WT mice here was similar to that observed in rats subjected to RRS (Harris et al. Citation2006). Although food intake returns to control levels once restraint ends, there is no attempt to overeat in the post-stress period to compensate for the energy deficit experienced on the days of stress (CitationHarris et al. 2002,2006). This lack of compensation undoubtedly contributes to the sustained reduction of body weight in WT RRS animals, but also suggests that stress causes a change in a regulatory system that normally would respond to correct for a loss of body weight.
Previously we showed that rats and mice subjected to RRS show exaggerated glucocorticoid release (Harris et al. Citation2004) and anxiety-type behavior (Chotiwat and Harris Citation2006) in response to a novel mild stress in the post-restraint period. In this study the CRFR1-KO mice were the only group in which RRS influenced corticosterone release in the post-restraint period. Serum corticosterone concentrations were low in the non-restrained CRFR1-KO mice compared with the other treatment groups. Previous exposure to RRS significantly increased both basal and post-test corticosterone, but did not restore a normal increase in corticosterone release following testing in the EPM. The ADX mice in this study had free access to water that contained low concentrations of corticosterone, resulting in circulating concentrations that were similar to those found in the RRS CRFR1-KO mice, but higher than those in Control CRFR1-KO mice. The only difference in behavior of the two RRS groups of mice was that CRFR1-KO mice spent more time in the middle zone of the EPM, whereas Control ADX mice tended to have higher levels of anxiety-type and fear-related behaviors than Control CRFR1-KO mice. We previously found that RRS NIH Swiss mice subjected to the same experimental design as was used in this study had higher corticosterone after exposure to the EPM, compared with control mice that had not been subjected to RRS (Chotiwat and Harris Citation2006). It is possible that the background strain of the mice accounted for the difference between the NIH Swiss and WT C57Bl/6 mice used here as there are large strain differences in fear-motivated behavior (Paylor and Crawley Citation1997).
Consistent with reports from other investigators, the CRFR1-KO mice displayed low levels of fear-related behavior, making more entries into the middle zone and both the open and closed arms of the maze than any other treatment group, but this was partially reversed in mice that had previously been exposed to RRS. By contrast, previous exposure to RRS appeared to decrease fear and anxiety in ADX mice, increasing the number of entries into open arms and reducing the time spent in closed arms. These opposing effects of RRS on behavior of ADX and CRFR1-KO mice imply that chronic effects of RRS on activity in the EPM was determined by an interaction between adrenal function and CRFR activation at the time of restraint. It is possible that the low level of fear-related behavior in CRF1-KO mice was secondary to a simultaneously low level of stress-induced corticosterone release, but if this had been the case then the behavior in the EPM would be expected to be the same in ADX and CRFR1-KO mice. Others have attributed changes in responsiveness of the HPA axis in chronically stressed rats to activation of the central nucleus of the amygdala (CeA; Bhatnagar and Dallman Citation1998) and the anterior region of the bed nucleus of the stria terminalis (Choi et al. Citation2008), which express CRFR2. The elevated levels of corticosterone during stress downregulate activation of some areas of the brain including the paraventricular nucleus of the hypothalamus (Imaki et al. Citation1991), but at the same time increase activation of other areas, such as the CeA (Cook Citation2002). Inhibition of glucocorticoid receptors in the CeA during stress has been reported to prevent the subsequent hyper-reactivity of the HPA axis (Cook Citation2002). Based on the results of this study it seems unlikely that the same integrative pathway is responsible for increased anxiety as for hyper-reactivity of the HPA axis, otherwise one would expect the behavior of RRS ADX and RRS CRFR1-KO mice to be similar.
There was no effect of previous exposure to RRS on the behavior of any of the mice in the L/D choice test although, consistent with reports from others (Bale et al. Citation2000), CRFR2-KO mice showed significantly higher levels of anxiety-type behavior than the other treatments tested. The increase in anxiety of chronically stressed rats has been attributed to activation of the basolateral nucleus of the amygdala (Rainnie et al. Citation2004) which has high levels of expression of CRFR1 (Van Pett et al. Citation2000) and has been associated with consolidation of emotional memories (Pare Citation2003). If CRFR2 normally moderate CRFR1 activity, then chronic, unopposed activation of CRFR1 in the basolateral amygdala is one potential explanation for the increased anxiety of CRFR2-KO mice. More recent evidence for CRFR2 control of sympathetic tone (Bale et al. Citation2003) and serotonin transport (McEuen et al. Citation2009) provides alternate potential mechanisms for the increased anxiety of the KO mice.
Overall, these results suggest that a surge in corticosterone secretion, mediated by CRFR1, during restraint is required for stress-induced weight loss in mice. An increase in anxiety-type behaviors and hyper-reactivity of the HPA axis in the post-restraint period were observed in CRFR1-KO mice, which indicates that these changes are independent of the RRS-induced weight loss and also are independent of the ability of the adrenal gland to mount a significant glucocorticoid response to stress.
Acknowledgements
The authors thank Donna Walker, Rachel Doyle, and Neil Naik for their assistance with this experiment. This work was supported by an NIH Grant MH068281 awarded to R. Harris.
Declaration of interest: The authors declare no conflict of interest. The authors alone are responsible for the content and writing of the paper.
References
- Bale TL, Vale WW. 2004. CRF and CRF receptors: Role in stress responsivity and other behaviors. Annu Rev Pharmacol Toxicol. 44:525–557.
- Bale TL, Contarino A, Smith GW, Chan R, Gold LH, Sawchenko PE, Koob GF, Vale WW, Lee KF. 2000. Mice deficient for corticotropin-releasing hormone receptor-2 display anxiety-like behaviour and are hypersensitive to stress. Nat Genet. 24:410–414.
- Bale TL, Anderson KR, Roberts AJ, Lee KF, Nagy TR, Vale WW. 2003. Corticotropin-releasing factor receptor-2-deficient mice display abnormal homeostatic responses to challenges of increased dietary fat and cold. Endocrinology. 144:2580–2587.
- Bhatnagar S, Dallman M. 1998. Neuroanatomical basis for facilitation of hypothalamic-pituitary–adrenal responses to a novel stressor after chronic stress. Neuroscience. 84:1025–1039.
- Bradbury MJ, McBurnie MI, Denton DA, Lee KF, Vale WW. 2000. Modulation of urocortin-induced hypophagia and weight loss by corticotropin-releasing factor receptor 1 deficiency in mice. Endocrinology. 141:2715–2724.
- Buwalda B, de Boer SF, Schmidt ED, Felszeghy K, Nyakas C, Sgoifo A, Van der Vegt BJ, Tilders FJ, Bohus B, Koolhaas JM. 1999. Long-lasting deficient dexamethasone suppression of hypothalamic-pituitary–adrenocortical activation following peripheral CRF challenge in socially defeated rats. J Neuroendocrinol. 11:513–520.
- Caggiula AR, Antelman SM, Aul E, Knopf S, Edwards DJ. 1989. Prior stress attenuates the analgesic response but sensitizes the corticosterone and cortical dopamine responses to stress 10 days later. Psychopharmacology. 99:233–237.
- Carlin KM, Vale WW, Bale TL. 2006. Vital functions of corticotropin-releasing factor (CRF) pathways in maintenance and regulation of energy homeostasis. Proc Natl Acad Sci USA. 103:3462–3467.
- Choi DC, Evanson NK, Furay AR, Ulrich-Lai YM, Ostrander MM, Herman JP. 2008. The anteroventral bed nucleus of the stria terminalis differentially regulates HPA axis responses to acute and chronic stress. Endocrinology. 149:818–826.
- Chotiwat C, Harris RB. 2006. Increased anxiety-like behavior during the post-stress period in mice exposed to repeated restraint stress. Horm Behav. 50:489–495.
- Chotiwat C, Harris RB. 2008. Antagonism of specific corticotropin releasing factor receptor subtypes selectively modifies weight loss in restrained rats. Am J Physiol Regul Integr Comp Physiol. 295:1762–1773.
- Contarino A, Dellu F, Koob GF, Smith GW, Lee KF, Vale W, Gold LH. 1999. Reduced anxiety-like and cognitive performance in mice lacking the corticotropin-releasing factor receptor 1. Brain Res. 835:1–9.
- Cook CJ. 2002. Glucocorticoid feedback increases the sensitivity of the limbic system to stress. Physiol Behav. 75:455–464.
- Crawley JN. 1985. Exploratory behavior models of anxiety in mice. Neurosci Biobehav Rev. 9:37–44.
- Fekete EM, Inoue K, Zhao Y, Rivier JE, Vale WW, Szücs A, Koob GF, Zorrilla EP. 2007. Delayed satiety-like actions and altered feeding microstructure by a selective type 2 corticotropin-releasing factor agonist in rats: Intra-hypothalamic urocortin 3 administration reduces food intake by prolonging the post-meal interval. Neuropsychopharmacology. 32:1052–1068.
- File SE, Gonzalez LE. 1996. Anxiolytic effects in the plus-maze of 5-HT1a-receptor ligands in dorsal raphe and ventral hippocampus. Pharmacol Biochem Behav. 54:123–128.
- Gammie SC, Hasen NS, Stevenson SA, Bale TL, D'Anna KL. 2005. Elevated stress sensitivity in corticotropin-releasing factor receptor 2 deficient mice decreases maternal, but not intermale aggression. Behav Brain Res. 160:169–177.
- Harris RB, Zhou J, Youngblood BD, Rybkin II, Smagin GN, Ryan DH. 1998. Effect of repeated stress on body weight and body composition of rats fed low- and high-fat diets. Am J Physiol. 275:R1928–R1938.
- Harris RB, Zhou J, Shi M, Redmann S, Mynatt RL, Ryan DH. 2001. Overexpression of agouti protein and stress responsiveness in mice. Physiol Behav. 73:599–608.
- Harris RB, Mitchell TD, Simpson J, Redmann SMJr, Youngblood BD, Ryan DH. 2002. Weight loss in rats exposed to repeated acute restraint stress is independent of energy or leptin status. Am J Physiol Regul Integr Comp Physiol. 282:R77–R88.
- Harris RB, Gu H, Mitchell TD, Endale L, Russo M, Ryan DH. 2004. Increased glucocorticoid response to a novel stress in rats that have been restrained. Physiol Behav. 81:557–568.
- Harris RB, Palmondon J, Leshin S, Flatt WP, Richard D. 2006. Chronic disruption of body weight but not of stress peptides or receptors in rats exposed to repeated restraint stress. Horm Behav. 49:615–625.
- Hogg S. 1996. A review of the validity and variability of the elevated plus-maze as an animal model of anxiety. Pharmacol Biochem Behav. 54:21–30.
- Imaki T, Nahan JL, Rivier C, Sawchenko PE, Vale W. 1991. Differential regulation of corticotropin-releasing factor mRNA in rat brain regions by glucocorticoids and stress. J Neurosci. 11:585–599.
- Inui A. 2000. Transgenic approach to the study of body weight regulation. Pharmacol Rev. 52:35–61.
- Koob GF, Heinrichs SC, Pich EM, Menzaghi F, Baldwin H, Miczek K, Britton KT. 1993. The role of corticotropin-releasing factor in behavioural responses to stress. Ciba Found Symp. 172:277–289.
- MacNeil G, Sela Y, McIntosh J, Zacharko RM. 1997. Anxiogenic behavior in the light–dark paradigm follwoing intraventricular administration of cholecystokinin-8S, restraint stress, or uncontrollable footshock in the CD-1 mouse. Pharmacol Biochem Behav. 58:737–746.
- McEuen JG, Semsar KA, Lim MA, Bale TL. Influence of sex and CRF pathways as determinants in 5-HT sensitivity. Endocrinology. 2009 (EPub).
- Misslin R, Belzung C, Vogel E. 1989. Behavioral validation of a light/dark choice procedure. Behav Process. 18:119–132.
- Morilak DA, Cecchi M, Khoshbouei H. 2003. Interactions of norepinephrine and galanin in the central amygdala and lateral bed nucleus of the stria terminalis modulate the behavioral response to acute stress. Life Sci. 73:715–726.
- Pare D. 2003. Role of the basolateral amygdala in memory consolidation. Prog Neurobiol. 70:409–420.
- Paylor R, Crawley JN. 1997. Inbred strain differences in prepulse inhibition of the mouse startle response. Psychopharmacology. 132:169–180.
- Rainnie DG, Bergeron R, Sajdyk TJ, Patil M, Gehlert DR, Shekhar A. 2004. Corticotrophin releasing factor-induced synaptic plasticity in the amygdala translates stress into emotional disorders. J Neurosci. 24:3471–3479.
- Sekino A, Ohata H, Mano-Otagiri A, Arai K, Shibasaki T. 2004. Both corticotropin-releasing factor receptor type 1 and type 2 are involved in stress-induced inhibition of food intake in rats. Psychopharmacology. 176:30–38.
- Smith GW, Aubry JM, Dellu F, Contarino A, Bilezikjian LM, Gold LH, Chen R, Marchuk Y, Hauser C, Bentley CA, Sawchenko PE, Koob GF, Vale W, Lee KF. 1998. Corticotropin releasing factor receptor 1-deficient mice display decreased anxiety, impaired stress response, and aberrant neuroendocrine development. Neuron. 20:1093–1102.
- Stengel A, Tache Y. Neuroendocrine control of the gut during stress: Corticotropin-releasing factor signaling pathways in the spotlight. Annu Rev Physiol. 2008 (EPub).
- Timpl P, Spanagel R, Sillaber I, Kresse A, Reul JM, Stalla GK, Blanquet V, Steckler T, Holsboer F, Wurst W. 1998. Impaired stress response and reduced anxiety in mice lacking a functional corticotropin-releasing hormone receptor 1. Nat Genet. 19:162–166.
- Truett GE, Heeger P, Mynatt RL, Truett AA, Walker JA, Warman ML. 2000. Preparation of PCR-quality mouse genomic DNA with hot sodium hydroxide and Tris (hotshot). Biotechniques. 52:54.
- Trullas R, Skolnick P. 1993. Differences in fear motivated behaviors among inbred mouse strains. Psychopharmacology. 111:323–331.
- Van Pett K, Viau V, Bittencourt JC, Chan RK, Li HY, Arias C, Prins GS, Perrin M, Vale W, Sawchenko PE. 2000. Distribution of mRNAs encoding CRF receptors in brain and pituitary of rat and mouse. J Comp Neurol. 428:191–212.