Abstract
Phthalate diesters, widely used in flexible plastics and consumer products, have become prevalent contaminants in the environment. Human exposure is ubiquitous and higher phthalate metabolite concentrations documented in patients using medications with phthalate-containing slow release capsules raises concerns for potential health effects. Furthermore, animal studies suggest that phthalate exposure can modulate circulating hormone concentrations and thus may be able to adversely affect reproductive physiology and the development of estrogen sensitive target tissues. Therefore, we conducted a systematic review of the epidemiological and experimental animal literature examining the relationship between phthalate exposure and adverse female reproductive health outcomes. The epidemiological literature is sparse for most outcomes studied and plagued by small sample size, methodological weaknesses, and thus fails to support a conclusion of an adverse effect of phthalate exposure. Despite a paucity of experimental animal studies for several phthalates, we conclude that there is sufficient evidence to suggest that phthalates are reproductive toxicants. However, we note that the concentrations needed to induce adverse health effects are high compared to the concentrations measured in contemporary human biomonitoring studies. We propose that the current patchwork of studies, potential for additive effects and evidence of adverse effects of phthalate exposure in subsequent generations and at lower concentrations than in the parental generation support the need for further study.
Introduction
Phthalates are alkyl diesters of phthalic acid named based on the lengths of the alkyl chains () and are used to impart flexibility in plastic or as a matrix in cosmetic products (Wittassek et al., Citation2011). Phthalates are not covalently bound to the plastic and thus leach into the environment where they are ubiquitous contaminants (Wittassek et al., Citation2011). Environmental sources of exposure include dust and water (Wittassek et al., Citation2011). However, the main sources of human exposure are food and consumer products such as cosmetics (Wittassek et al., Citation2011). As a result, human exposure to phthalates is widespread (Blount et al., Citation2000; Calafat & McKee, Citation2006).
Figure 1. The general chemical structure of a phthalate diester (alkyl chains designated by R) in addition to the chemical structures of the more commonly researched phthalates and major metabolites of the diester.
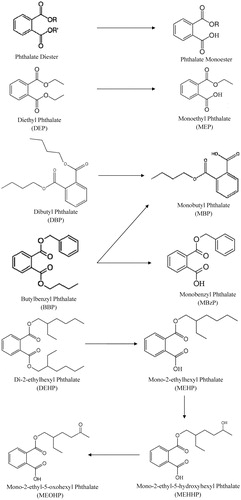
Owing to the extensive use of phthalates in many commercial products and medical devices as well as broad environmental contamination, great care needs to be exercised to avoid sample contamination and ensure accurate exposure estimates. Specifically, contamination is a potential concern if materials containing phthalates are used to collect, process or analyze study subject samples. After absorption, phthalates are initially metabolized to monoesters which can be metabolized further and are eventually excreted as glucuronides in urine (Wittassek et al., Citation2011). Phthalates are rapidly metabolized and eliminated in the urine and quantification of phthalate monoesters in the urine has become a reliable biomarker of phthalate exposure. While measuring metabolites in urine is a more valid method of quantification, some metabolites are more accurate indicators of exposure than others. For example, the utility of two di-2-ethylhexyl phthalate (DEHP) metabolites, mono-(2-ethyl-5-oxohexyl) phthalate (MEOHP) and mono-(2-ethyl-5-hydroxyhexyl) phthalate (MEHHP), were examined as potential DEHP exposure biomarkers (Barr et al., Citation2003). These metabolites are formed by oxidative metabolism of mono-2-ethylhexyl phthalate (MEHP). Both the range and the mean urinary MEOHP and MEHHP concentrations in 62 subjects were on average four-fold higher than those of MEHP; the mean of the individual ratios of MEHHP/MEOHP, MEHHP/MEHP and MEOHP/MEHP were 1.4, 8.2 and 5.9, respectively (Barr et al., Citation2003). These data suggest that MEOHP and MEHHP are more sensitive biomarkers of exposure to DEHP than is MEHP. These findings also suggest a predominant human metabolic route for DEHP hydrolysis to MEHP followed by the oxidation of MEHP; they also imply that a similar mechanism may be relevant for other high-molecular-weight phthalates, such as di-n-octyl, di-isononyl and di-isodecyl phthalates (DnOP, DiNP and DiDP). As a result, the selection of metabolites as biomarkers for the phthalate diesters with longer alkyl chains should be carefully considered in order to allow for accurate quantification of exposure.
Based on urinary biomarkers, average human exposures are estimated to be between 2.32–12 μg/kg/d for diethyl phthalate (DEP), 0.26–0.88 μg/kg/d butylbenzyl phthalate (BBP), 0.84–5.22 μg/kg/d dibutyl phthalate (DBP), 0.12–1.4 μg/kg/d di-isobutyl phthalate (DiBP), 0.71–4.6 μg/kg/d DEHP and 0.29 μg/kg/d DiNP in German and US populations (reviewed in Koch & Calafat, Citation2009). Interestingly, young women have greater exposure than males of the same age, possibly due to an increased use of cosmetics (Wittassek et al., Citation2011). Exposure may also be substantially higher in people using medications with slow release capsules in which phthalates have been employed in their manufacture (Hauser et al., Citation2004; Hernandez-Diaz et al., Citation2009). In a spot urine sample collected 3 months after a single male subject was prescribed a mesalamine preparation in which DBP is a component of the slow release capsule, the concentration of monobutyl phthalate (MBP), a DBP metabolite, was 16 868 ng/mL (6180 μg/g creatinine) (Hauser et al., Citation2004). This concentration was more than two orders of magnitude higher than the 95th percentile for males reported in the 1999–2000 National Health and Nutrition Examination Survey (NHANES) whereas the patient’s urinary concentrations of monoethyl phthalate (MEP) (443.7 ng/mL, 162.6 μg/g creatinine), MEHP (3.0 ng/mL, 1.1 μg/g creatinine) and monobenzyl phthalate (MBzP) (9.3 ng/mL, 3.4 μg/g creatinine), and thus exposure to DEP, DEHP and BBP were unremarkable (Hauser et al., Citation2004). Of the 7999 persons with information on urinary phthalate concentrations in the NHANES 1999–2004 study, six reported using mesalamine formulations, some of which may include DBP; the mean urinary concentration of MBP, the main DBP metabolite, was 50 times higher among mesalamine users compared to controls (2257 μg/L versus 46 μg/L; p < 0.0001) (Hernandez-Diaz et al., Citation2009). Similarly, DEP is present in some prescription medication preparations of didanosine, omeprazole and theophylline and mean urinary concentrations of MEP, the main DEP metabolite, were significantly higher in those taking the medications than in a reference population (Hernandez-Diaz et al., Citation2009). Hence, some medications can be a source of high exposure to some phthalates and thus raise concern about potential human health risks resulting from these exposures. In view of widespread human exposure and potential greater exposure through some medications, the possibility that phthalates could affect health is concerning. Therefore, we conducted a systematic review of the literature to evaluate the strength of the evidence concerning the potential reproductive and developmental health effects of phthalates. For the purposes of this review, we defined reproductive and developmental toxicity as the ability of phthalates to adversely affect reproductive health in women or the reproductive capacity of experimental animals at any stage throughout the life-span. Potential adverse effects of phthalates on the risk of breast cancer or mammary gland development were included in this review owing to the role of estrogens and other hormones in mammary gland development and pathophysiology of breast cancer.
Approach to systematic review
A Pubmed search was carried out to identify studies relevant to the effect of phthalates on the female reproductive system. Topics of interest included puberty, fertility, endometriosis, polycystic ovarian syndrome (PCOS), pre-eclampsia or eclampsia, spontaneous abortion and pregnancy outcomes. The search terms used and the number of articles retrieved were: phthalic acid or phthalates AND abortion spontaneous OR miscarriage, 30 articles; phthalic acid AND endometriosis, 13 articles, phthalic acid OR phthalates AND fertility OR infertility, 142 articles; phthalic acid AND obstetric labor, premature OR infant, premature OR fetal development OR preterm delivery OR birth weight, 154 articles; and phthalic acid OR phthalates AND puberty OR puberty, delayed OR puberty, precocious, 49 articles. Searches for articles on phthalic acid OR phthalates AND polycystic ovarian syndrome, phthalic acid OR phthalates AND pre-eclampsia, and phthalic acid OR phthalates AND eclampsia each returned one article which was not relevant to the focus of this review. In total, 388 articles were initially retrieved. Of these, duplicate papers (n = 35), reviews (n = 17), and unrelated (n = 265) or inaccessible (n = 5) articles were excluded. The abstracts of the remaining articles were read and studies describing the effect of phthalates on the female reproductive system in humans or animals were retained for further assessment. Studies examining phthalates in combination with other compounds or examining outcomes in areas other than the reproductive system were excluded. Relevant studies were also identified through a concurrent search on the effects of phthalates in the male reproductive system as some studies provided information about effects on the pregnant animals despite focusing on males. Additional searches were carried out as new topics such as breast cancer were identified. Non-systematic searches, for example on follicle health, were also conducted to explore the areas of interest. Finally, bibliographies of all retained articles were searched for additional relevant citations.
Epidemiological literature
Puberty
Seven studies were identified in which the effect of phthalate exposure on the onset of puberty in girls was reported. In one study of six girls, no effect of phthalate exposure during infancy on puberty was reported (Rais-Bahrami et al., Citation2004). However, as exposure was remote to the time of puberty assessment and animal studies have shown that the effects of phthalates are reversible (Alam et al., Citation2010; Boekelheide et al., Citation2009; Oishi Citation1985; Saffarini et al., Citation2012), any potential effect of phthalate exposure may have been missed. Other studies of puberty in girls yielded conflicting results. Two studies reported that increased phthalate concentrations were correlated with premature thelarche (in one study, 96.5 ± 134 ng/ml monomethyl phthalate (MMP) in cases versus 26.4 ± 30 ng/ml MMP in controls p = 0.005; in the other, 68% of cases had high serum concentrations of phthalates) (Chou et al., Citation2009; Colon et al., Citation2000). However, the possibility that the samples in these studies were inadvertently contaminated by environmental sources of phthalates cannot be excluded, an issue that has been discussed previously (McKee, Citation2004). Both studies had small samples sizes with only 41 cases of premature thelarche in one study (Colon et al., Citation2000) while 30 cases of premature thelarche and 26 cases of central precocious puberty with 33 controls were described in the second study which was only obtained as an abstract (Chou et al., Citation2009). In contrast, a study with a larger sample size measured development in normal girls twice a year and found that low-molecular-weight phthalates, or phthalate diesters with short alkyl chains, were non-significantly (p = 0.087) correlated with more rapid breast development (Wolff et al., Citation2010). This study also reported an association between exposure to the high-molecular-weight phthalates and a significant decrease in the development of pubic hair (p = 0.04) (Wolff et al., Citation2010). Similarly, in another study involving 725 girls, no association between phthalate exposure, as measured in urine, with breast development was found; however, there was an association with delayed pubic hair growth (mean age 11.4 years [11.1–11.7] in the fourth quartile of exposure compared to 10.7 years [10.4–11.0] in the first) (Frederiksen et al., Citation2012). As phthalates are considered anti-androgenic compounds, delayed appearance of pubic hair is not unexpected. Similarly, while phthalates are only weakly estrogenic, they may also be able to affect breast development. However, assessment of breast development may be confounded by increased body weight and failure to distinguish between increased adiposity and breast bud development, yielding misleading conclusions about the onset of puberty (Walvoord, Citation2010).
Phthalates have also been investigated for an association with precocious puberty. One case control study, available only in English abstract form, observed higher levels of phthalates in the serum of girls with precocious puberty (27.3% and 22.7% of cases with DBP and DEHP compared to 4% and 3% of controls) (Qiao et al., Citation2007). Unfortunately, phthalate exposure was measured in serum and thus the potential for sample contamination cannot be excluded. Furthermore, no association between phthalates and precocious puberty was found in a study of 28 case/control pairs when metabolites were measured in urine (Lomenick et al., Citation2010). Additionally, one large cohort study compared the concentrations of phthalate metabolites in the urine of 725 healthy and 25 girls with precocious puberty and reported no difference between the groups (Frederiksen et al., Citation2012). Therefore, we conclude that the evidence does not support an association between phthalate exposure and precocious puberty.
Endometriosis
Endometriosis is an estrogen-dependent disease characterized by the growth of endometrial epithelial and stromal cells anywhere in the body outside the uterine cavity. The central role of estrogens in the pathophysiology of endometriosis has led several investigators to postulate a potential relationship between phthalate exposure and endometriosis; however, the results have been ambiguous. In one case-control study, with 35 cases and 24 controls, higher DEHP concentrations were found in cases compared to controls (p = 0.0047) (Cobellis et al., Citation2003). Another case-control study reported a non-significant association between DEHP and endometriosis (OR = 1.001 [1.000–1.002] p = 0.161) but found a significant weak association between MEHP and endometriosis (OR = 1.020 [1.003–1.038] p = 0.020) after analyzing 97 cases and 169 controls (Kim et al., Citation2011). In addition, higher concentrations of DBP, BBP, DEHP and DnOP (p < 0.01) were found in 49 women with endometriosis versus 38 infertile controls and 21 fertile controls and exposure to DBP, BBP, DEHP and DnOP was correlated with the severity of endometriosis (p < 0.01 for all) (Reddy et al., Citation2006a). Higher concentrations of DBP, BBP, DEHP and DnOP in cases compared to controls were also reported in a case-control study with a larger sample size (85 cases and 135 controls) (Reddy et al., Citation2006b). Taken together, the above studies suggest that there is a weak association between phthalate exposure and endometriosis. However, phthalates were measured in plasma leading to the possibility of sample contamination from plastics used to obtain or analyze the samples, and thus provide potentially misleading results. Indeed, in one study, no correlation between DEHP and MEHP concentrations was found, suggesting that higher DEHP concentrations in plasma may be the result of sample contamination (Cobellis et al., Citation2003). Methodological problems were also present in other studies. No association between urinary phthalate concentrations and endometriosis was found in another case-control study which grouped endometriosis-free and endometriosis-stage 1 women together as controls (80 women) while treating more advanced stages of endometriosis as cases (57 women) (Itoh et al., Citation2009). Other investigators, using measures of phthalate metabolites in the urine, have also been unable to demonstrate significant relationships between phthalate exposure and endometriosis (Huang et al., Citation2010; Weuve et al., Citation2010). The concentrations of the phthalate metabolites MBP and MEHP were non-significantly higher (p < 0.1) in endometriosis cases (n = 28) compared to controls in one study (Huang et al., Citation2010). Similarly, a non-significant association between MBP and endometriosis was found in another study (Weuve et al., Citation2010). Furthermore, MEHP was significantly higher in controls compared to cases (p = 0.03) in this cross-sectional study involving 1221 women including 201 cases (Weuve et al., Citation2010). However, case/control status was determined by questionnaire and past diagnosis (Weuve et al., Citation2010). Given that a definitive diagnosis of endometriosis requires an invasive procedure (surgery) and histopathological confirmation results in significant delays in diagnosis, the potential for misclassification error in these studies is high. Hence, due to the poor reliability of studies in which exposure was determined through the quantification of phthalates in plasma and methodological difficulties, we suggest that the available literature currently fails to support any association between phthalate exposure and endometriosis.
Leiomyomas
Leiomyomas or fibroids are noncancerous tumors in the uterus whose cause is unknown, but are thought to be tied to estrogen and potentially progesterone exposure. Therefore, a possible association between phthalate exposure and leiomyomas was also investigated in two studies. However, while MEHP exposure was higher in cases with leiomyomas compared to controls (OR = 2.90 [1.05–7.97]; p = 0.04), other metabolites (MEP, MBP, MBzP, MEOHP, MEHHP) were not significantly different between cases and controls in one study (Huang et al., Citation2010). In a second study, MBP was non-significantly higher in cases and MEHP was non-significantly higher in controls (OR = 1.56 [0.93–2.61] and OR = 0.63 [0.35–1.12], respectively) (Weuve et al., Citation2010). Hence, the results are equivocal and we conclude that they are insufficient to establish a relationship between phthalate exposure and leiomyomas.
Time-to-pregnancy and pregnancy loss
Only one epidemiological study was found that examined a potential association between phthalate exposure and female fecundity. In a cohort study involving 6302 women, increased time to pregnancy after probable occupational exposure to phthalates was observed (OR = 2.16 [1.02–4.57]) (Burdorf et al., Citation2011). While this study suggests that phthalate exposure can negatively impact conception, we note that exposure to phthalates was not directly quantified and thus consider this weak evidence of a potential association.
The literature examining the relationship between early and late pregnancy loss and phthalate exposure is also sparse. One study used the data collected in a previous prospective cohort study as well as stored urine samples to examine possible associations between phthalate exposure (DEP, DEHP, DBP, BBP) and subclinical early pregnancy loss as well as clinical spontaneous abortions (Toft et al., Citation2012). High MEHP exposure (third tertile) was associated with early pregnancy loss when compared to low MEHP exposure (first tertile) (OR = 2.87 [1.09–7.57]) (Toft et al., Citation2012). Conversely, increased exposure to MEHP was protective for late pregnancy loss (third to first tertile: OR = 0.25 [0.05–1.8], second to first tertile: OR = 0.17 [0.03–0.95]) (Toft et al., Citation2012). Although the authors suggested that an increased loss of susceptible early pregnancies led to the decrease in spontaneous abortions later in pregnancy, they acknowledge that their results could be due to chance (Toft et al., Citation2012). A second study, obtained only in abstract form, reported an increased risk for spontaneous abortions with increased exposure to phthalates (Tabacova et al., Citation1999). Despite the paucity of studies, we suggest that the association between phthalate exposure and early pregnancy loss merits further study.
Pregnancy outcomes
We identified 12 studies examining potential relationships between phthalate exposure during pregnancy and gestation length and birth weight. In a cohort study of 283 women, metabolites of DEHP in urine were associated with increased gestational age (MEHP: OR = 2.0 [1.1–3.5], MEOHP: OR = 2.2 [1.3–4.0], MEHHP: OR = 2.1 [1.3–3.7]) (Adibi et al., Citation2009). Accordingly, exposure to DEHP was concluded to be associated with a protective effect on preterm birth (Adibi et al., Citation2009). Similarly, an association between low-molecular-weight phthalates in urine and increased gestational age at birth (β = 0.14 week [0.01–0.27 week]) was found in a cohort of 382 pregnant women (Wolff et al., Citation2008). When specific phthalates were examined, the association was again with MEHP (β = 0.15 weeks [0.02–0.29]) (Wolff et al., Citation2008). On the other hand, the absence of MEHP in cord blood was associated with increased gestational age (OR = 1.50 [1.013–2.21]) in a cross-sectional study involving 84 infants (Latini et al., Citation2003). Likewise, MEHP in the urine was associated with decreased gestational age (5.0 d shorter [2.1–8.0 d], p = 0.001 between fourth and first quartiles) in a cohort study involving 311 maternal infant dyads (Whyatt et al., Citation2009). In this study, exposure to DEHP was quantified by measuring metabolites in urine in addition to measuring inhaled DEHP exposure using personal air sampling pumps over a 2 d period (Whyatt et al., Citation2009). Interestingly, the levels of DEHP in the air were not significantly correlated with gestational age (Whyatt et al., Citation2009), suggesting that other sources may be more important contributors to exposure. We also note that contradictory results may arise from exclusion of extremely premature infants from the study. For example, a correlation between phthalate exposure and increased gestational age was found when infants born at less than 32 weeks of gestation were excluded (Wolff et al., Citation2008) whereas the opposite was found when infants born from 27 weeks of gestation were included (Latini et al., Citation2003). Two studies included infants born at less than 37 weeks gestation but their inclusion/exclusion criteria for extremely premature infants was unclear (Adibi et al., Citation2009; Whyatt et al., Citation2009).
Other studies directly examined preterm birth as opposed to gestational age. No significant association between preterm birth and phthalate exposure was found in a cohort of 6302 women (Burdorf et al., Citation2011). However, exposure was not directly measured in this study but was estimated through estimates of occupational exposure only. On the other hand, preterm birth was correlated with increased maternal exposure to most phthalates measured as metabolites in urine (MBP, mono-isobutyl phthalate (MiBP), mono-3-carboxypropyl phthalate (MCPP), MEHP, MEHHP and MEOHP) in a case-control study with 30 cases and 30 controls (Meeker et al., Citation2009). When adjusted for dilution using creatinine, only the concentration of MBP was significantly associated with preterm birth (OR = 5.4 [1.5–19.3]) (Meeker et al., Citation2009). These results suggest that phthalate exposure may be associated with preterm birth and, therefore, that an effect on gestational age may be observed in observational studies depending on the inclusion criteria regarding premature infants.
Several studies reported a decrease in infant size after in utero phthalate exposure whereas other studies reported the opposite and still others were unable to show any relationship (Burdorf et al., Citation2011; Huang et al., Citation2009; Philippat et al., Citation2012; Suzuki et al., Citation2010; Wolff et al., Citation2008; Zhang et al., Citation2009). For example, Burdorf et al. (Citation2011) suggested that occupational exposure to phthalates was associated with low birth weight (OR = 2.42 [1.10–5.34]). Similarly, after the measurement of phthalate exposure in umbilical vein blood, maternal blood and meconium in 88 cases and 113 controls, DBP and MEHP were associated with low birth weight (DBP: OR = 3.54 [1.54–6.15], p = 0.008; MEHP: OR = 2.05 [1.17–3.70], p = 0.05) whilst DEP and DEHP were not significantly associated with birth weight (Zhang et al., Citation2009). Two other studies directly contradict these results and report an association between phthalate exposure as measured using urinary biomarkers and increased size at birth (Huang et al., Citation2009; Wolff et al., Citation2008). In a cohort study of 65 children, increased birth weight and length were associated with exposure to DBP, quantified as metabolites in urine (birth weight p = 0.031; length p = 0.018 between first and fourth quartiles) (Huang et al., Citation2009). However, premature infants were included in this study, potentially biasing results towards the null effect and thus the association may be stronger than reported. In the second study, an association between exposure to phthalates with low molecular weights and increased head circumference (β = 0.13 cm [0.01–0.24 cm] with increases in concentration) was found (Wolff et al., Citation2008). Associations between MBzP, a metabolite of BBP, and length (β = 0.20 cm [0.00–0.40]) as well as with MEP, a metabolite of DEP, and head circumference (β = 0.12 cm [0.01–0.23]) were also reported (Wolff et al., Citation2008). Still, other studies reported no association between the birth size and phthalate exposure. In a cohort of 287 women, no association was found with the birth weight, length or head circumference after measuring phthalate exposure in urine (p > 0.14) (Philippat et al., Citation2012). Additionally, no association was found between urinary concentrations of phthalate metabolites and any birth outcome measured in a cohort study of 149 women (Suzuki et al., Citation2010). In this study, the birth outcomes measured included weight, length and head circumference (Suzuki et al., Citation2010). Taken together, on a weight-of-evidence basis, studies in which exposure was assessed through urinary metabolites, extremely premature infants were excluded, and effects on birth weight were corroborated by complementary measures, suggest only a weak association between phthalate exposure and premature birth.
Breast cancer
A possible relationship between phthalate exposure and breast cancer has been investigated in several studies yielding mixed results. One large case-control study with 233 cases and 221 controls reported increased mean concentrations of MEP but decreased mean concentrations of MBP, MCPP and MEOHP in the urine of women with breast cancer compared to controls (Lopez-Carrillo et al., Citation2010). The odds ratios, after correction for age, age of menarche, parity and menopausal status, showed a protective effect of MBzP and MCPP exposure (MBzP: OR = 0.46 [0.27–0.79], p = 0.008; MCPP: OR = 0.44 [0.24–0.80], p = 0.007) (Lopez-Carrillo et al., Citation2010). On the other hand, increased urinary concentrations of MEP and mono-2-ethyl-5-carboxypentyl phthalate (MECPP), a metabolite of DEHP, were significantly associated with increased breast cancer risk (MEP: OR = 2.20 [1.33–3.63], p = 0.003]; MECPP: OR = 1.68 [1.01–2.78], p = 0.047) (Lopez-Carrillo et al., Citation2010). The odds ratio for MECPP was only borderline significant and may have been due to chance, especially as the other metabolites of DEHP were not significantly different between cases and controls (Lopez-Carrillo et al., Citation2010). A second case-control study examined occupational exposure to endocrine disrupting compounds including BBP in 261 cases and 753 controls and found no association with breast cancer (Aschengrau et al., Citation1998). However, a limitation of this study is its failure to directly quantify exposure, instead relying only on estimates from potential occupational exposure to BBP. Based on these two studies, exposure to phthalates is not thought to be associated with an increased risk of breast cancer and may even be protective.
However, the lack of association between phthalate exposure and breast cancer risk was unexpected as phthalates are thought to have estrogenic properties and thus play a role in the pathobiology of breast cancer. For example, BBP and DBP both increased transcription of an estrogen-regulated gene in breast cancer cells after treatment with a combination of estradiol and phthalate (Jobling et al., Citation1995). Furthermore, these cells proliferated in response to DBP and BBP alone, although cell growth was less than after treatment with estradiol (Jobling et al., Citation1995). These results suggest that phthalates are weakly estrogenic and may act as partial agonists (Jobling et al., Citation1995). In a second study, BBP was more potent than DEP after the estrogenic activity of the main phthalates was compared (Harris et al., Citation1997). Furthermore, BBP but not DEP increased the proliferation of human breast cells in vitro (Harris et al., Citation1997). Still, the phthalates with estrogenic properties were much less potent than estradiol (Harris et al., Citation1997). The results from these in vitro studies are difficult to reconcile with the protective effect seen by Lopez-Carrillo and colleagues. One hypothesis is that the phthalates may compete with estradiol for the estrogen receptor, decreasing the effect of estrogen on breast cancer (Jobling et al., Citation1995). However, as BBP and DBP added to the effect of estradiol in culture, this is unlikely. Moreover, BBP and other phthalates were more potent estrogenic compounds (although still weak) than DEP (Harris et al., Citation1997). In summary, we conclude that the current literature is insufficient to support a role, if any, of phthalate exposure in the development or progression of breast cancer.
Animal studies
While epidemiological studies are able to uncover potential associations between environmental contaminant exposure and adverse health outcomes (as summarized in ), they alone cannot establish causal links. Animal experiments are important because they allow for controlled exposure to known quantities of test chemicals in genetically similar or identical animals, and thus allow for mechanistic insight that cannot be achieved through epidemiological studies. Hence, animal studies are essential to understand potential causal links between phthalate exposure and adverse health outcomes and provide insight into the mode of action as well as dose–response characteristics. Therefore, the effects of phthalate exposure on the reproductive health of experimental animals were evaluated. There was a paucity of studies for many of the phthalate diesters, whereas multiple studies were found for the more common phthalates. For each phthalate the lowest observed adverse effect level (LOAEL), no observed adverse effect level (NOAEL) and main adverse outcomes reported are summarized in .
Table 1. Summary of the epidemiological literature exploring the link between phthalate exposure and adverse reproductive health outcomes in women.
Table 2. Summary of the NOAELs and LOAELs in mg/kg/d as well as the direction of change for each outcome, respectively, found in animal studies for each individual phthalate.
Dimethyl phthalate
Prior studies have established that phthalate potency is related to the length of alkyl chains with the most potent phthalates having chain lengths of 4–6 carbon atoms (Gray, Jr. et al., Citation2000; Heindel et al., Citation1989). Dimethyl phthalate (DMP) is the phthalate diester with the shortest alkyl chain length and is therefore thought to be less toxic than other phthalates. In the only study found, DMP exposure had no effect on pregnancy at doses up to 2 ml/kg when administered during early gestation to Sprague–Dawley rats through intraperitoneal (ip) injection (Peters & Cook, Citation1973). Although ip injection bypasses first pass metabolism of the DMP by the liver, the absence of any adverse effects is reassuring.
Diethyl phthalate
Three studies examined the effect of diethyl phthalate (DEP) exposure on the female reproductive system. Two of these studies focused exclusively on pregnancy outcomes whereas one administered a mixture of contaminants including DEP to mice (Hayashi et al., Citation2010). In this study, DEP made up 0.7 ppm of the mixture, two mice failed to become pregnant and delivery in the other mice was delayed (Hayashi et al., Citation2010). Unfortunately, the results of this study cannot be attributed solely to DEP, and thus were not considered further in this review.
Dietary exposure to DEP at doses of 0.25–2.5% (approximately 340–3640 mg/kg/d) had no effect on the litter size, the number of pups born and pup weight in Swiss CD-1 mice; however, the number of pups per litter was decreased in the next generation (Anonymous, 1997b), suggesting a transgenerational effect of continuous exposure. In another study, no change in estrous cycle characteristics was found in Sprague–Dawley rats administered 600–15 000 ppm DEP in diet over two generations (approximately 40–1375 mg/kg/d); however, vaginal opening was delayed at 15 000 ppm in the second generation (Fujii et al., Citation2005). Furthermore, the duration of pregnancy was shortened and the weights of the pups decreased (Fujii et al., Citation2005). Additionally, pinna detachment and eye opening in the pups was delayed at 15 000 ppm (1000–1375 mg/kg/d) even though there was no effect on the development of other reflexes (Fujii et al., Citation2005). Taken together, these studies suggest that DEP may negatively affect the female reproductive system (pregnancy and puberty after in utero exposure) at high doses administered throughout the lives of the animals. The results also indicate that the most sensitive period for exposure is during gestation and that potential transgenerational effects of DEP can occur. However, we also note that the lowest doses used in these studies are approximately 100 times greater than human exposure and effects were only noted at the highest concentrations used.
Dipropyl phthalate
Only two studies were found that examined the effects of dipropyl phthalate (DPrP) on pregnancy. In one study, adult female Swiss CD-1 mice at the highest dose (5% in diet or approximately 8600 mg/kg/d administered before and during breeding) were infertile (Heindel et al., Citation1989). Additionally, there were fewer pups per litter in the 2.5% DPrP in the diet (4100 mg/kg/d) treatment group (Heindel et al., Citation1989). However, female fertility was unaffected at 1.25% in diet (1900 mg/kg/d) (Heindel et al., Citation1989). In contrast, in utero exposure to DPrP (gestational day (GD) 6-GD20) had no effect on pregnancy in Sprague–Dawley rats at all doses administered by gavage (up to 1500 mg/kg/d) although pup weight was decreased starting in the 1000 mg/kg/d treatment group (Saillenfait et al., Citation2011b). Impaired development of the pups, supernumerary ribs, and fewer ossification centers in the paws was observed after exposure to 1000 and 1500 mg/kg/d (Saillenfait et al., Citation2011b). These studies suggest a negative effect of DPrP on the female reproductive system and a possible teratogenic role at high doses.
Dibutyl phthalate
We found 20 studies that examined the effect of dibutyl phthalate (DBP), a phthalate diester with four carbon alkyl chains, on the female reproductive system. The effect of DBP exposure on sexual maturation, fertility and pregnancy outcomes, development of the female reproductive tract, and mammary gland development and tumors has been studied.
Sexual maturation
In total, six studies examining sexual maturity, either through vaginal opening or the estrous cycle, were found. Four of these studies did not demonstrate any evidence of a DBP-induced change in the onset of sexual maturity as measured by the day of vaginal opening in Long–Evans, Wistar or Sprague–Dawley rats (Gray, Jr. et al., Citation2006; Guerra et al., Citation2010; Mylchreest et al., Citation1998, Citation2000). The highest dose used in these studies was 750 mg/kg/d (Mylchreest et al., Citation1998), while the lowest dose used was 0.5 mg/kg/d (Mylchreest et al., Citation2000). The exposure periods in these studies included postnatal day (PND) 21 to sacrifice, GD12-PND21, GD3-PND20 and GD12-21 (Gray, Jr. et al., Citation2006; Guerra et al., Citation2010; Mylchreest et al., Citation1998, Citation2000). Therefore, these studies suggest no effect of DBP on vaginal opening at either high or low doses when exposure occurs at any time (during gestation, nursing or after weaning). However, two studies reported a trend for delayed onset of vaginal opening in Sprague–Dawley rats exposed to 10 000 ppm in diet GD15-PND21 (Lee et al., Citation2004) and significantly delayed the onset of vaginal opening in Long–Evans rats exposed to 12 or 50 mg/kg/d in utero and after birth until sexual maturation, respectively (Salazar et al., Citation2004). Additionally, first estrus was delayed at 50 mg/kg/d (Salazar et al., Citation2004). However, the litter was not the statistical unit in one study (Salazar et al., Citation2004), meaning that similarities between animals from the same litter could affect the conclusions. Therefore, the results may not be an accurate indication of the effects of DBP at a low dose. The doses of DBP used in these conflicting studies covered a similar range and mainly utilized the same route of administration (gavage). While different strains of rats were used, strain differences do not appear to be responsible for the inconsistent results. Despite the two studies reporting an effect, we propose that overall, the evidence suggests that DBP does not affect sexual maturation and the onset of sexual maturity, results that are remarkably harmonious with those in the epidemiological literature (Frederiksen et al., Citation2012; Wolff et al., Citation2010).
Fertility and pregnancy loss
DBP exposure has been shown to decrease maternal weight gain, fetal weight and food consumption as well as increase the number of resorptions, and pre- and post-implantation losses in animal studies. Adverse effects on pregnancy and maternal health became apparent at doses of DBP or MBP administered by gavage during gestation from 500 mg/kg/d and up, including a reduction in maternal weight gain and in fetal weight in Wistar, Long–Evans and Sprague–Dawley rats (Ema & Miyawaki, Citation2001a,Citationb; Ema et al., Citation1995, Citation1996c, Citation2000; Gray, Jr. et al., Citation2006; Zhang et al., Citation2004). While some studies in Wistar rats reported effects as mild as changes in maternal food consumption and weight gain, or in the weight of the pups (Ema et al., Citation1996c, Citation2000), other studies reported a decrease in the number of live pups at similar doses (500 mg/kg/d by gavage or 1% in diet) in Long–Evans and Sprague–Dawley rats (Gray, Jr. et al., Citation2006; Wine et al., Citation1997). Increases in pregnancy loss, including increases in resorptions and the number of dead fetuses, at doses of 500 mg/kg/d or higher have also been reported in Wistar and Long–Evans rats (Ema et al., Citation1996c, Citation2000; Gray, Jr. et al., Citation2006). Additionally, more female Wistar rats were unable to become pregnant when exposed to 1250 or 1500 mg/kg/d compared to controls (Ema et al., Citation2000). Interestingly, a decrease in the maternal weight and an increase in pregnancy loss was reported at a dose of 50 mg/kg/d in Long–Evans rats (Salazar et al., Citation2004).
Other groups, however, have reported no effect on pregnancy in rats after doses of 500 mg/kg/d (Barlow & Foster, Citation2003; Carruthers & Foster, Citation2005; Ema et al., Citation1993a; Ema Citation2002; Fisher et al., Citation2003; Jiang et al., Citation2007; Mylchreest et al., Citation2000, Citation1998; Struve et al., Citation2009). One study examining a lower dose of DBP (100 mg/kg/d by gavage GD12-PND21) reported no effects on pregnancy outcomes including maternal weight, the number of implantation sites, serum progesterone and fertility of the female Wistar rats exposed during gestation (Guerra et al., Citation2010). Overall, the studies which examined both the health of the pregnant dam and the reproductive health of the male pups generally found that the doses needed to affect the dam were higher than the doses that affected the male pups (Barlow & Foster, Citation2003; Carruthers & Foster, Citation2005; Ema & Miyawaki, Citation2001a; Fisher et al., Citation2003; Jiang et al., Citation2007; Lee et al., Citation2004; Mylchreest et al., Citation1998, Citation2000; Salazar et al., Citation2004; Struve et al., Citation2009; Zhang et al., Citation2004).
The mode of DBP action has been poorly defined; however, decreased progesterone was observed in pregnant Wistar rats treated with 1500 mg/kg/d DBP although there was no change in estradiol (Ema et al., Citation2000). Similarly, a decrease in serum progesterone in Long–Evans rats was reported after exposure to 500 mg/kg/d (Gray, Jr. et al., Citation2006). Furthermore, in this study, DBP decreased progesterone and increased estradiol production from ovaries in vitro (Gray, Jr. et al., Citation2006). These studies suggest that the effects of DBP may occur through changes in the circulating concentration of the hormones responsible for maintaining pregnancy.
Female reproductive tract
DBP treatment has been reported to have no effect on estrous cycle characteristics in rats at doses up to 1000 mg/kg/d by gavage and 1% DBP in diet even if exposure occurred during gestation (Gray, Jr. et al., Citation2006; Guerra et al., Citation2010; Wine et al., Citation1997). However, a non-significant increase in estrous cycle irregularity after exposure to 2000 and 10 000 ppm DBP in diet GD15-PND21 has also been observed (Lee et al., Citation2004). Still, other outcomes including ovarian histology in female fetuses, anogenital distance (AGD) in female pups, number of nipples, ovary and uterine weight and histology, number of corpora lutea and follicles at different developmental stages, serum follicle stimulating hormone (FSH), luteinizing hormone (LH), progesterone concentrations and mating behavior in Wistar rats were all unaffected after in utero and lactational exposure to 100 mg/kg/d of DBP (Guerra et al., Citation2010). Thus, the absence of an adverse effect on complementary outcome measures leads us to conclude that the female reproductive tract function is relatively insensitive to potential toxic effects of DBP exposure.
Mammary gland development
Only one study that examined the histology of the mammary glands after exposure to DBP was found. In this study, hypoplasia of the mammary glands was observed in Sprague–Dawley rats after in utero exposure to doses as low as 20 ppm DBP in diet (Lee et al., Citation2004). These data suggest a potential anti-estrogenic effect of DBP exposure on mammary gland development. Further, the epidemiological studies to date do not support a role for DBP in the development of breast cancer and are consistent with the results of the present review.
The lowest NOAEL reported for female rats was 50 mg/kg/d (Zhang et al., Citation2004). Other studies in rats reported NOAELs of 250 mg/kg/d (Ema Citation2002; Ema & Miyawaki, Citation2001a,Citationb; Ema et al., Citation1995, Citation2000; Gray, Jr. et al., Citation2006; Mylchreest et al., Citation1998). On the other hand, studies have also reported LOAELs of 20 ppm in diet and 50 mg/kg/d by gavage (Lee et al., Citation2004; Salazar et al., Citation2004). Overall, DBP is able to adversely affect the health of pups exposed in utero as well as the reproductive health of female animals exposed as adults.
Di-isobutyl phthalate
The majority of the studies found no effect of di-isobutyl phthalate (DiBP) exposure on pregnancy in Wistar and Sprague–Dawley rats at doses up to 625 mg/kg/d (Boberg et al., Citation2008; Borch et al., Citation2006; Saillenfait et al., Citation2008b). However, decreased maternal and fetal weights at 500 mg/kg/d administered orally during gestation as well as increased resorptions and fetal deaths at 750 mg/kg/d or higher doses were seen in a separate study conducted in Sprague–Dawley rats (Saillenfait et al., Citation2006). Interestingly, increased AGD in female Wistar rat pups was found in two studies administering in utero doses of 600 mg/kg/d by gavage (Boberg et al., Citation2008; Borch et al., Citation2006). Additionally, the expression of aromatase in the ovaries was increased after oral exposure to 600 mg/kg/d (Boberg et al., Citation2008). Currently, a NOAEL of 250 mg/kg/d DIBP is suggested based on maternal toxicity and the weights of pups (Saillenfait et al., Citation2006).
Butylbenzyl phthalate
Like DBP, one metabolite of butylbenzyl phthalate (BBP) is MBP; however, the effects of BBP are less well documented. We found and reviewed 14 articles examining the effects of BBP on sexual maturation, fertility and pregnancy, and mammary tumors.
Sexual maturation
The effect of BBP on sexual maturation in female animals is unclear, with several studies providing divergent findings. A 2 d delay in vaginal opening was reported in Sprague–Dawley rats exposed orally to 500 mg/kg/d BBP in utero (Moral et al., Citation2011). On the other hand, a low dose of BBP (183 μg/kg), administered in drinking water, was reported to advance vaginal opening by 1 d in AP rats exposed during gestation and nursing (Ashby et al., Citation1997). It is important to note that these studies did not consider litter effects, meaning their results may be affected by similarities between animals from the same litter. Other studies reported no change in the onset of sexual maturity using oral doses from 20 to 500 mg/kg/d in Sprague–Dawley rats (Aso et al., Citation2005; Nagao et al., Citation2000). The reasons for the contradictory results in these studies are difficult to resolve. In one study, the advance in vaginal opening was the only significant effect observed and the authors suggest that it was due to the increased weights of the pups (Ashby et al., Citation1997). However, because opposing effects have been reported at high and low doses, more research on the effect of low doses of BBP on puberty is needed to rule out a potential biphasic effect.
Fertility and pregnancy
Exposure to BBP during pregnancy induced decreased body weight gain, decreased food consumption, increased resorptions, an increased number of dead fetuses, decreased weight of live fetuses and increased the incidence of external, internal and skeletal malformations in rats (Ema & Miyawaki Citation2002; Ema et al., Citation1993b, Citation1996a,Citationb, Citation1998, Citation1999, Citation2003; Piersma et al., Citation2000; Uriu-Adams et al., Citation2001). The effects of BBP as a teratogen in Wistar rats varied depending on when the exposure occurred. Internal and skeletal malformations occurred when oral exposure was provided from GD 7–9; external and skeletal malformations occurred when exposure was from GD 13–15; and relatively few malformations occurred when exposure occurred between GD 10–12 (Ema et al., Citation1993b, Citation1996a). Similarly, an increase in pre-implantation loss was found when exposure began on GD0 (Ema et al., Citation1993b; Ema et al., Citation1998). Further, decreased ovarian weights were found in 500 mg/kg/d treated Sprague–Dawley rats (Nagao et al., Citation2000). BBP exposure (200 mg/kg/d orally) also decreased uterine weights in treated Sprague–Dawley rats (Aso et al., Citation2005).
Based on decreased uterine weights and decreased levels of progesterone, it was suggested that decidualization in pregnant rats dosed with BBP was reduced due to lower serum concentrations of progesterone (Ema et al., Citation1994, Citation1998). Other hypotheses including changes in metabolism of zinc as a result of BBP exposure have been postulated to explain these observations; however, no real changes in the absorption or distribution of zinc have been documented (Uriu-Adams et al., Citation2001). On the other hand, a study examining the viability of embryos treated in vitro with MBzP found that embryo viability decreased at doses of 3 mM in rats and 5 mM in mice (Saillenfait et al., Citation2003). This evidence suggests that the adverse effects of BBP exposure on pregnancy outcomes may, in part, result from the direct effects of this metabolite on the embryo.
Mammary tumors
One study examined the effect of BBP on mammary gland morphology in Sprague–Dawley rats and reported increases in the number of terminal end buds, alveolar buds and terminal ducts after exposure to 500 mg/kg/d BBP in utero (Moral et al., Citation2011). Changes in proliferating cells were also seen and the authors suggested that these effects increase the susceptibility to breast cancer (Moral et al., Citation2011). However, these results directly contradict an epidemiological study in humans which reported a negative association between concentrations of MBzP (a metabolite of BBP) in urine and the incidence of breast cancer and a second study which found no association between occupational exposure to BBP and breast cancer (Aschengrau et al., Citation1998; Lopez-Carrillo et al., Citation2010). Thus, although the literature is limited, we suggest that there is insufficient evidence to either support or disprove a potential role of BBP exposure in the pathophysiology of breast cancer.
Based on these studies, we conclude that BBP has the ability to affect both the mature and developing female reproductive system as well as pregnancy at high doses. We note that one study examining doses of BBP relevant to human exposure found no effect except for advanced vaginal opening which was not believed to be a result of BBP. Thus, BBP is thought to be a reproductive and developmental toxicant only at high concentrations. The lowest NOAEL reported for effects on pregnancy was 250 mg/kg/d (Ema et al., Citation1998). On the other hand, 250 mg/kg/d caused a decrease in maternal weight gain and food consumption in other studies (Ema et al., Citation1996a,Citationb). In general, we suggest a NOAEL of 250 mg/kg/d, a dose that is much higher than human exposure.
Di-pentyl phthalate (DPP)
Only two studies were identified in our search of the literature that examined the effects of di-pentyl phthalate (DPP) on the female reproductive system (Hannas et al., Citation2011; Heindel et al., Citation1989). Fetal mortality was increased after oral exposure of the pregnant Sprague–Dawley rats to 1200 mg/kg/d (Hannas et al., Citation2011) and the number of litters and pups decreased in Swiss CD-1 mice exposed to 0.5% DPP in diet (760 mg/kg/d) before and during pregnancy (Heindel et al., Citation1989). Female mice were infertile after exposure to 1.25% DPP or greater in diet (2160 mg/kg/d) (Heindel et al., Citation1989). Therefore, we conclude that DPP is a reproductive toxicant at high concentrations.
Di-n-hexyl phthalate
In our search, three studies were identified that examined the effects of di-n-hexyl phthalate (DnHP) exposure in female animals. One study reported infertility in Swiss CD-1 mice after exposure to 1.2% DnHP in the diet (1670 mg/kg/d) as well as a decreased number of litters with fewer live pups per litter at 0.3% (380 mg/kg/d) when exposure occurred continuously over two generations (Anonymous, 1997a). In the other two studies, oral DnHP exposure at concentrations of 625 mg/kg/d and more during pregnancy increased post-implantation losses, resorptions, and decreased the number and weight of pups in Sprague–Dawley rats (Saillenfait et al., Citation2009a,Citationb). Based on these studies, we suggest that DnHP is a reproductive toxicant at high concentrations.
Di-cyclohexyl phthalate
While di-cyclohexyl phthalate (DcHP) is thought to be less potent than other phthalates due to the different configuration of its alkyl groups (Saillenfait et al., Citation2009a), prolonged estrous cycle length was found in Sprague–Dawley rats exposed to 6000 ppm DcHP in diet (Hoshino et al., Citation2005). Regardless, there was no effect of DcHP exposure on fertility or pregnancy in this study and pup viability, development and reflexes were also unaffected even in the pups of animals exposed in utero and throughout their lifespan (Hoshino et al., Citation2005). Similarly, a second study reported no changes in the number of implantations or viable fetuses in Sprague–Dawley rats after oral exposure GD6-20 to 750 mg/kg/d DcHP (Saillenfait et al., Citation2009a). On the other hand, decreases in pup weights were reported at 500 mg/kg/d (Saillenfait et al., Citation2009a; Yamasaki et al., Citation2009). Additionally, a decrease in the survival of pups to PND4 was seen after Sprague–Dawley rats were exposed to 500 mg/kg/d during gestation (Yamasaki et al., Citation2009). Thus, we suggest that these studies provide evidence that DcHP in high concentrations is a reproductive and developmental toxicant.
Di-(2-ethylhexyl) phthalate
Di-(2-ethylhexyl) phthalate (DEHP) has been relatively more thoroughly researched than the other phthalates as shown by the presence of 28 articles in the literature in which the impact of DEHP on female reproductive health was investigated. These studies describe the effects of DEHP on sexual maturation, fertility and pregnancy loss, and changes to female reproductive tract structure.
Sexual maturation
The effect of DEHP exposure on sexual maturation is unclear. A non-significant trend for delayed first estrus and earlier vaginal opening at oral doses from 375 to 1500 mg/kg/d has been described in Sprague–Dawley rats exposed during gestation (Moore et al., Citation2001). Both vaginal opening and the time of first estrus were delayed starting at an oral dose of 15 mg/kg/d in Wistar rats (Grande et al., Citation2006). However, in another study using Wistar–Imamichi rats, both markers of sexual maturation occurred earlier after exposure through inhalation to approximately 1 and 5 mg/kg/d from PND22 to PND84 (Ma et al., Citation2006). Interestingly, a third study reported delayed vaginal opening in C57/Bl6 mice after oral exposure to higher doses of DEHP (500 or 1000 mg/kg/d) in utero (Moyer & Hixon, Citation2012). These conflicting results cannot be explained by a difference in doses as these studies used overlapping dose ranges, and found opposite effects at relatively similar doses. Another possible explanation for the inconsistent results is the length of exposure or the method of administration, which varied between these studies. In one study, oral exposure continued until weaning (Grande et al., Citation2006), in the second, rats were exposed through inhalation after weaning (Ma et al., Citation2006), and in the third, mice were orally exposed only during gestation (Moyer & Hixon, Citation2012). In summary, these animal studies suggest that DEHP at high concentrations may be able to delay puberty. However, the effect of low concentrations of DEHP is less clear, although it may advance the onset of puberty.
Fertility and pregnancy loss
Several studies were found that revealed no effect of DEHP exposure on pregnancy based on maternal weight and food consumption, litter size and pup weights in mice and rats (Anonymous, 1997d; Grande et al., Citation2006; Gray, Jr. et al., Citation2009). In these studies, the doses used ranged from 0.05% in the diet to 405 mg/kg/d orally (Anonymous, 1997d; Grande et al., Citation2006; Gray, Jr. et al., Citation2009). Interestingly, Wistar rat pup weights were increased at doses less than 5 mg/kg/d (Grande et al., Citation2006). Also of interest, one study reported increased litter sizes after administering oral doses of 1000 mg/kg/d to female mice (Moyer & Hixon, Citation2012). Other studies using high doses of DEHP found negative effects on pregnancy. Maternal weight and food consumption, as well as pup weight, number of pups born and the rate of post-implantation loss were variably affected at oral doses from 500 to 1500 mg/kg/d as well as 10 ml/kg/d and 1% in diet in mice and rats (Anonymous, 1997c; Dalsenter et al., Citation2006; Jarfelt et al., Citation2005; Moore et al., Citation2001; Peters et al., Citation1997; Pocar et al., Citation2012; Shirota et al., Citation2005; Tomita et al., Citation1982; Tyl et al., Citation1988). One study reported a 100% pregnancy loss rate after exposure to 500 mg/kg/d DEHP in C3H/N mice (Schmidt et al., Citation2012). Decreased pup weight in CD-1 mice was also found at a relatively low dose of 5 mg/kg/d in diet, although resorptions did not increase until 500 mg/kg/d (Pocar et al., Citation2012). While this study did not correct for a possible litter effect, it suggests that adverse effects may also occur at lower doses. Interestingly, the effects of DEHP exposure on pregnancy in PPARα knockout mice as well as in wild-type mice were similar at high doses in both groups of mice (Peters et al., Citation1997). PPARα is thought to be at least partially responsible for toxicity in rodents, particularly in the liver. However, the human PPARα has lower affinity for phthalates and is expressed at a lower level (Ito & Nakajima, Citation2008; Rusyn & Corton, Citation2011). As a result, the consequences of phthalate exposure in rats may be due to mechanisms that are not applicable in humans. However, as Peters et al. show, there may be PPARα-independent pathways leading to reproductive toxicity (Ito & Nakajima, Citation2008; Peters et al., Citation1997). Therefore, the effects of DEHP in rats and mice may be biologically relevant to humans despite the lesser importance of the PPARα pathway in humans.
In Crj:CD-1 mice exposed to 0.01%, 0.03% and 0.09% DEHP in the diet, one failure to become pregnant occurred in both the 0.01% and 0.03% DEHP dose groups and pregnancy loss was observed in one dam of the 0.03% treatment group (Tanaka, Citation2002). At 0.09% DEHP in the diet, the survival of the mice and the pups was significantly reduced (Tanaka, Citation2002). In the crossover mating trials, failures to become pregnant occurred in both male-treated/female-control and female-treated/male-control groups, suggesting that both male and female fertilities are affected by DEHP exposure (Tanaka, Citation2003). Although DEHP exposure negatively affected fertility, the number of adverse events was small. Still, in a separate study, failure to become pregnant occurred in some Sprague–Dawley rats after oral exposure to 3000 mg/kg/d (Takai et al., Citation2009).
A recent study revealed that after in utero exposure to 0.05 and 5 mg/kg/d DEHP in diet, the oocytes of treated rats were less likely to mature and were less able to form a viable zygote (Pocar et al., Citation2012). Similarly, an in vitro study has demonstrated decreased mouse oocyte survival, possibly as a result of oxidative stress after treatment with 250 μM MEHP (Bonilla & Del, Citation2010). Furthermore, two studies have reported impaired maturation of MEHP-treated oocytes (bovine and mouse) in vitro (Anas et al., Citation2003; Dalman et al., Citation2008). Likewise, growth of mouse follicles treated with 2.77 μM DEHP, 0.34 μM MEHP or higher doses was decreased in vitro (Gupta et al., Citation2010). Similarly, two recent studies treated mouse follicle with DEHP and MEHP and reported decreased follicle growth after doses from 0.1 to 100 μg/ml MEHP and 1 to 100 μg/ml DEHP (Wang et al., Citation2012a,Citationb). In these studies, oxidative stress was increased as a result of treatment while expression and activity of antioxidant enzymes were inhibited. Interestingly, the enzyme affected by DEHP treatment, superoxide dismutase 1, was not the enzyme affected by MEHP, glutathione peroxidase (Wang et al., Citation2012a,Citationb). Furthermore, co-treatment with an antioxidant (N-acetyl cysteine) rescued follicle growth (Wang et al., Citation2012a,b). These results indicate an adverse effect of both MEHP and DEHP on follicle growth through reactive oxygen species. On the other hand, a second in vitro study reported no changes in the development of mouse follicles exposed to 200 μM MEHP in culture (Lenie & Smitz, Citation2009). Still, another recent study reported decreased numbers of primordial follicles as well as increases in the numbers of primary, secondary and antral follicles after oral exposure to 1000 mg/kg/d DEHP, which the authors suggest indicated increased recruitment of follicles and therefore a shorted reproductive lifespan with earlier entry into ovarian failure (Moyer & Hixon, Citation2012). Interestingly, in this study, the animals’ concentrations of estradiol were decreased compared to controls later in life, possibly supporting the authors’ idea of earlier ovarian failure (Moyer & Hixon, Citation2012).
Other in vitro studies have also investigated changes in steroidogenesis as a possible mode of action. One study examined Fischer 344 rat granulosa cells treated with 100 μM MEHP in vitro and reported decreases in progesterone as a result of decreased cAMP response to FSH (Treinen et al., Citation1990). While this study corroborates the idea that DEHP may be able to negatively affect the production of progesterone, other in vitro studies have found contradictory results. In one study, progesterone production was increased in KK-1 granulosa tumor cells treated with 100 μM MEHP, although there was no change in cAMP (Gunnarsson et al., Citation2008). In another, progesterone and testosterone production were increased in mouse follicles after treatment with 200 μM MEHP (Lenie & Smitz, Citation2009). These results are at odds with the idea that negative effects on reproduction are caused by decreases in the circulating concentrations of progesterone. Interestingly, both studies that reported increases in progesterone were conducted in mice while the study reporting a decrease was conducted in rats. Thus, the opposing results could be due to species differences. In vitro studies have also examined changes in estradiol production. In Fischer 344 rat granulosa cells, estradiol was decreased after treatment with 50 μM MEHP (Lovekamp-Swan et al., Citation2003). Decreases in the expression of aromatase as a result of PPARγ activation, and increases in 17β-HSD expression as a result of PPARα activation were thought to be responsible for the change in steroidogenesis (Lovekamp-Swan et al., Citation2003). Other studies have partially corroborated these hypotheses. One reported a relative increase in estrone production and a decrease in estradiol production from mouse follicles treated with 200 μM MEHP (Lenie & Smitz, Citation2009). Other studies have reported decreases in estradiol combined with decreased aromatase expression after exposure to 50 or 100 μM MEHP (Davis et al., Citation1994b; Lovekamp & Davis, Citation2001). Overall, these studies show that estradiol production can be affected by MEHP treatment in vitro.
Studies conducted in vivo have also examined the effects of DEHP on steroidogenesis. However, the results of these studies are mixed. One study found that serum estradiol and progesterone concentrations were unaffected by exposure to maximum oral doses of 405 and 2500 mg/kg/d DEHP in Wistar rats and marmosets, respectively (Grande et al., Citation2007; Tomonari et al., Citation2006). In contrast, circulating concentrations of estrogen were decreased, FSH was increased and surges in progesterone and LH were suppressed in adult Sprague–Dawley rats at 2000 mg/kg/d, a dose that also prevented ovulation (Davis et al., Citation1994a). Furthermore, estradiol and progesterone concentrations were decreased in immature Sprague–Dawley rats treated orally with 500 mg/kg/d DEHP, potentially the result of decreased transport of cholesterol across cell membranes (Svechnikova et al., Citation2007). Additionally, Sprague–Dawley rats treated with 1400 mg/kg DEHP twice a week had lower serum concentrations of estradiol and FSH, as well as lower levels of FSH and LH in the pituitary (Hirosawa et al., Citation2006). This study also examined protein expression in the pituitary and concluded that secretion of LH and FSH could be changed by DEHP treatment (Hirosawa et al., Citation2006). On the other hand, increased levels of estradiol and increased expression of aromatase in the ovaries after low doses administered by inhalation (around 1 mg/kg/d) have also been reported (Ma et al., Citation2006). Similarly, increases in estradiol and FSH as well as decreases in LH were reported in mice after oral exposure to 1000 mg/kg/d (Moyer & Hixon, Citation2012). Finally, one study reported increases in estradiol and testosterone production from ovaries taken from mice treated with 1500 mg/kg/d DEHP and cultured during the diestrus stage of the estrous cycle (Laskey & Berman, Citation1993). Taken together, these data suggest that DEHP can adversely affect the ovarian function and steroidogenesis although the mechanisms have yet to be defined.
Female reproductive tract
The impact of DEHP on female reproductive health in the non-pregnant animal has been studied with equivocal results. Two studies found no effect of DEHP on female reproductive health in Wistar and albino rats when administered through inhalation and intraperitoneal injections, respectively (Klimisch et al., Citation1992; Seth et al., Citation1976), whereas three studies have shown that DEHP exposure increased estrous cycle length and induced irregular estrous cycles after exposure to 25 mg/km3 through inhalation, or oral doses greater than 1000 mg/kg/d in Wistar and Sprague–Dawley rats, and in mice (Hirosawa et al., Citation2006; Ma et al., Citation2006; Moyer & Hixon, Citation2012; Takai et al., Citation2009). On the other hand, another study reported no change in estrous cyclicity at doses up to 405 mg/kg/d in Wistar rats (Grande et al., Citation2007). At higher doses, ovulation was suppressed by oral DEHP exposure (2000 mg/kg/d) in Sprague–Dawley rats (Davis et al., Citation1994a). In a similar study with the same maximum dose, no change in forced ovulation in Fischer 344 rats as a result of subcutaneous DEHP treatment for 4 d was demonstrated (Sekiguchi et al., Citation2006). Taken together, these data suggest that the adverse effects of DEHP may be at the level of the hypothalamus or pituitary.
DEHP exposure has also been shown to adversely affect the morphology of the female reproductive tract. Greater numbers of atretic follicles and vacuolization of stromal cells was found in Wistar and Sprague–Dawley rats exposed to DEHP doses between 300 and 405 mg/kg/d (Grande et al., Citation2007; Takai et al., Citation2009). Additionally, epithelial thinning, decreased corpora lutea and uterine atrophy have also been described in rats exposed to 3000 mg/kg/d (Takai et al., Citation2009). Conversely, an increase in the weight of the uterus and ovaries was found when marmosets were exposed to 500 and 2500 mg/kg/d (Tomonari et al., Citation2006). Although the results of these two studies differ, we note that different animal models were used and that marmosets are recognized to be less sensitive. Therefore we conclude that DEHP exposure adversely affects estrous cycle length and reproductive organ structure.
In summary, DEHP exposure can affect reproductive organ structure, and function as shown by changes in circulating reproductive hormone concentrations, oocyte health, ovulation, fertility and progression of pregnancy in adult mice and rats. Two studies have reported a NOAEL of 5 mg/kg/d orally for female pups exposed in utero (Grande et al., Citation2006; Pocar et al., Citation2012) whereas the NOAEL reported in female prepubescent rats was approximately 1 mg/kg/d through inhalation (Ma et al., Citation2006). In adult females, inhalation and subcutaneous DEHP exposure resulted in NOAELs that were 11–18 and 250 mg/kg/d, respectively (Klimisch et al., Citation1992; Sekiguchi et al., Citation2006). While there is a large difference between these values, the LOAELs in the studies were 230–360 and 500 mg/kg/d respectively (Klimisch et al., Citation1992; Sekiguchi et al., Citation2006). Based on these studies, we suggest a NOAEL of 1.0 mg/kg/d.
Diheptyl phthalate
In the only study found in our search, diheptyl phthalate (DHPP) exposure had no effect on body weight, number of implantations, number of live fetuses or number of resorptions in pregnant Sprague–Dawley rats exposed orally to 1000 mg/kg/d (Saillenfait et al., Citation2011a) although there was a trend for decreased pup weight (Saillenfait et al., Citation2011a). While this is only one study, the results suggest that DHPP is neither a reproductive nor developmental toxicant.
Di-isoheptyl phthalate
Only one study describing the effects of di-isoheptyl phthalate (DiHP) was found. In this study, Crl:CDBR VAF/Plus rats were treated with 100, 300 or 750 mg/kg/d by gavage during gestation, or administered DiHP in the diet at concentrations of 1000, 4500 or 8000 ppm over two generations (McKee et al., Citation2006). Decrease in weight gain during pregnancy as well as decreased fetal viability and increased malformations were found with exposure to 750 mg/kg/d DiHP (McKee et al., Citation2006). Exposure to lower doses in the diet (1000 ppm equivalent to 64–144 mg/kg/d) had no effect on body weight or fertility, and the number of offspring (McKee et al., Citation2006). However, females exposed in utero to 8000 ppm had decreased fertility and the pups of females exposed in utero to 4500 or 8000 ppm (approximately 304–716 or 532–1289 mg/kg/d) had decreased weights compared to controls (McKee et al., Citation2006). Overall, while adverse effects on the female reproductive system occurred, they did so at relatively high doses.
Di-n-octyl phthalate
We reviewed two studies which examined the effects of di-n-octyl phthalate (DnOP) on the female reproductive system. In one study, there was no effect on fertility in the first or second generation of Swiss CD-1 mice exposed to DnOP at doses up to 5% in the diet (approximately 7500 mg/kg/d) (Heindel et al., Citation1989). Additionally, there was no change in body weight or in the estrous cycle characteristics (Heindel et al., Citation1989). Furthermore, a second study reported no changes in the number of implantations, live fetuses, resorptions or the number of malformations after oral exposure of pregnant Sprague–Dawley rats to 1000 mg/kg/d from GD6-20 (Saillenfait et al., Citation2011a). Although the literature is sparse, data suggest that DnOP is neither a reproductive nor developmental toxicant.
Di-isononyl phthalate
Three studies examining the effects of di-isononyl phthalate (DiNP) on the female reproductive system were found. In one study, oral exposure to DiNP had no effect on pregnancy in Sprague–Dawley rats at doses from 250 to 750 mg/kg/d but increased male pup weights at 250 mg/kg/d (Adamsson et al., Citation2009). Similarly, another study reported no significant effects on pregnancy in Wistar rats as determined by body weights, gestation length, number of pups and post-implantation loss at oral doses from 300 to 900 mg/kg/d (Boberg et al., Citation2011). Likewise, a third study in Sprague–Dawley rats found no effect on pregnancy except for a trend for decreased pup weight at 0.4% and 0.8% in the diet; however, this effect was attributed in some cases to an increased number of pups per litter (Waterman et al., Citation2000). This study also reported decreased pup weights at doses from 0.5% in the diet, decreased maternal weight at 1% in the diet as well as decreased ovarian weight and decreased survival of pups at 1.5% in the diet (Waterman et al., Citation2000). Overall, these studies indicate that there are no important adverse effects of DiNP exposure on pregnancy.
Di-isodecyl phthalate
Only one study investigating di-isodecyl phthalate (DiDP) and female reproductive health was found. The estrous cycle and number of oocytes in Sprague–Dawley rats were unaffected after exposure to DiDP at doses from 0.02% to 0.8% in the diet over two generations (Hushka et al., Citation2001). On the other hand, the number of pups and pup survival was decreased at 0.8% in the first generation and at 0.4% and 0.8% in the second generation, and vaginal opening was delayed in the 0.4% and 0.8% exposure groups (Hushka et al., Citation2001). Despite a paucity of studies, we suggest that DiDP exposure can adversely affect pregnancy and female reproductive health.
Di-C7-C9 alkyl phthalate and di-C9-C11 alkyl phthalate
Two articles examining the effects of di-C7–C9 alkyl phthalate (D79P) and di-C9–C11 alkyl phthalate (D911P) on the female reproductive system were reviewed. No significant effect of D79P or D911P on pregnancy was reported at doses up to 1000 mg/kg/d by gavage during gestation or 1% in diet over two generations in Sprague–Dawley rats (Fulcher et al., Citation2001; Willoughby et al., Citation2000). A dose-dependent relationship with the incidence of supernumerary ribs in the pups was noted (Fulcher et al., Citation2001). However, this was the only important significant effect in this study (Fulcher et al., Citation2001). Gestation length was decreased in the first generation of animals in the other study (Willoughby et al., Citation2000); however, gestation length was unaffected in the second generation. There was no change in the estrous cycle (Willoughby et al., Citation2000). Similarly, in the second generation of animals, there was no significant difference in the onset of sexual maturity (Willoughby et al., Citation2000). Interestingly, in the second generation, ovarian weights were decreased after exposure to D79P and uterine weights were decreased after exposure to D911P (Willoughby et al., Citation2000). However, these effects are thought to be random as they are not consistent between phthalate diesters or generations.
Diallyl phthalate
Only one article regarding the effects of diallyl phthalate (DAP) on reproductive health was found and revealed decreased body weight and food consumption, and two premature births in Sprague–Dawley rats exposed to oral doses of 200 mg/kg/d DAP or higher during gestation (Saillenfait et al., Citation2008a). Fetal weights were decreased in the 250 mg/kg/d treatment group whilst there was no change in fertility, litter size or post-implantation loss in any treatment groups (Saillenfait et al., Citation2008a). Furthermore, no increases in external, internal or skeletal malformations were found (Saillenfait et al., Citation2008a). Therefore we suggest that DAP may have a negative effect on pregnancy but is not teratogenic at low doses. However, the reproductive tract was not rigorously examined, hence definitive conclusions cannot be made. Thus, we suggest that, on the basis of this single study, DAP is unlikely to be a reproductive or developmental toxicant.
Summary and conclusions
There is compelling evidence of widespread human exposure to phthalates and some phthalate-containing medications result in much higher exposures than seen in the general population. The epidemiological literature fails to reveal an association with increased risk for endometriosis, breast cancer and precocious puberty. However, these studies are plagued by inadequate sample size, methodological issues and potential misclassification errors. Future studies are needed to clarify any potential associations between phthalate exposure and these reproductive health concerns. Furthermore, we suggest that the epidemiological literature supports a weak potential relationship between phthalate exposure and subfertility, pregnancy loss, pre-term birth and decreased birth weight that merits further investigation.
A relatively robust literature was found for DBP, BBP and DEHP; however, the literature was sparse for less well-known phthalates including DiBP and DPP. Furthermore, the animal literature has shown that adverse reproductive effects occur at high doses whereas concentrations representative of human exposure do not produce reproductive or developmental effects. The relevance of current animal models is questionable. Rats are strongly affected by phthalates through peroxisome proliferating pathways which are not relevant in humans (Ito & Nakajima, Citation2008; Rusyn & Corton, Citation2011; Ward et al., Citation1998). While phthalates can affect the reproductive system through other pathways as seen in PPARα null mice (Peters et al., Citation1997; Ward et al., Citation1998), the majority of the animal studies have been conducted in wild-type mice or rats. Therefore, the effects seen in animal studies may be completely or in part the result of pathways not important in humans. As it is impossible to determine which effects are due to the PPAR pathways and which may occur in humans, all the results are of questionable relevance. A different animal model, marmosets, has different problems. When excretion of phthalates in marmosets was measured, only a small percentage was found in the urine although the metabolites were conjugated to glucuronic acid, as is seen in humans (Kurata et al., Citation2012; Rhodes et al., Citation1986). However, as the pattern of excretion is different from that seen in humans, who excrete the majority of phthalate metabolites in urine, it is possible that marmosets absorb phthalates differently and thus may be less sensitive to phthalates.
Overall, while important data gaps remain, the weight-of-evidence suggests that phthalates may not significantly affect the female reproductive system of women in the general population who are only exposed to low concentrations of phthalates. The potential for adverse effects resulting from higher exposure and through interaction with other phthalates and chemicals is unknown, and thus continues to present a concern.
Future directions
While the documented adverse effects of phthalates occur at concentrations above those representative of human exposure, we suggest that further study is needed to resolve concerns arising from exposure through medications in which phthalates are used in the manufacture of slow release capsules. We also suggest that measurement of phthalate metabolites in urine and target tissues of the treated animals could provide useful data for comparison with data from human biomonitoring studies. Results of such studies will enhance translation of results to humans and comparison of findings with those of epidemiological studies and help reduce uncertainties in risk assessment.
Studies to date have focused almost exclusively on exposure to single phthalate metabolites. Although this is understandable, evidence that phthalates may act in an additive manner suggest that studies involving exposure to mixtures of phthalates representative of human exposure are needed to better understand potential human health risks. Furthermore, the interaction of phthalate metabolites with other contaminants widely documented in human biomonitoring studies would also contribute to greater confidence in existing NOAELs and calculated reference fixed doses.
Results of transgenerational studies suggest that offspring may be more sensitive to subsequent exposure to phthalates than parental animals. Therefore, future studies should better define the effects of phthalate exposure in the offspring of animals exposed in utero and determine whether or not the LOAEL decreases in subsequent generations. These results suggest potential epigenetic mechanisms that are yet to be explored in relation to phthalate exposure. The concentration of phthalates needed to induce epigenetic changes should be defined in order to better understand potential implications to human health. Moreover, the health implication of changes in epigenetic signatures also needs to be better developed.
Declaration of interests
Funding has been provided by Ferring Pharmaceutical to WGF for the qualification of phthalate exposure in patients prescribed medications containing phthalates in slow release capsules. No external funding support was provided for the preparation of this manuscript. The manuscript was prepared during the normal course of the authors’ employment. The authors have not participated in any legal or regulatory proceedings related to the subject matter of the manuscript. The authors have sole responsibility for the preparation of the manuscript and the views and opinions expressed in the manuscript.
Acknowledgements
The authors gratefully acknowledge the administrative support of Ms. Margaret Talbot during the preparation of this manuscript.
References
- Anonymous (1997a). Reproductive toxicology: di-n-hexylphthalate. Environ Health Perspect, 105, 251–2
- Anonymous (1997b). Reproductive toxicology: Diethylphthalate. Environ Health Perspect, 105, 245–6
- Anonymous (1997c). Reproductive toxicology: diethylhexyl phthalate. Environ Health Perspect, 105, 241–2
- Anonymous (1997d). Reproductive toxicology: diethylhexyl phthalate, juvenile exposure, mice. Environ Health Perspect, 105, 243–4
- Adamsson A, Salonen V, Paranko J, Toppari J. (2009). Effects of maternal exposure to di-isononylphthalate (DINP) and 1,1-dichloro-2,2-bis(p-chlorophenyl)ethylene (p,p'-DDE) on steroidogenesis in the fetal rat testis and adrenal gland. Reprod Toxicol, 28, 66–74
- Adibi JJ, Hauser R, Williams PL, et al. (2009). Maternal urinary metabolites of Di-(2-Ethylhexyl) phthalate in relation to the timing of labor in a US multicenter pregnancy cohort study. Am J Epidemiol, 169, 1015–24
- Alam MS, Andrina BB, Tay TW, Tsunekawa N, et al. (2010). Single administration of di(n-butyl) phthalate delays spermatogenesis in prepubertal rats. Tissue Cell, 42, 129–35
- Anas MK, Suzuki C, Yoshioka K, Iwamura S. (2003). Effect of mono-(2-ethylhexyl) phthalate on bovine oocyte maturation in vitro. Reprod Toxicol, 17, 305–10
- Aschengrau A, Coogan PF, Quinn M, Cashins LJ. (1998). Occupational exposure to estrogenic chemicals and the occurrence of breast cancer: an exploratory analysis. Am J Ind Med, 34, 6–14
- Ashby J, Tinwell H, Lefevre PA, et al. (1997). Normal sexual development of rats exposed to butyl benzyl phthalate from conception to weaning. Regul Toxicol Pharmacol, 26, 102–18
- Aso S, Ehara H, Miyata K, et al. (2005). A two-generation reproductive toxicity study of butyl benzyl phthalate in rats. J Toxicol Sci, 30, 39–58
- Barlow NJ, Foster PM. (2003). Pathogenesis of male reproductive tract lesions from gestation through adulthood following in utero exposure to Di(n-butyl) phthalate. Toxicol Pathol, 31, 397–410
- Barr DB, Silva MJ, Kato K, et al. (2003). Assessing human exposure to phthalates using monoesters and their oxidized metabolites as biomarkers. Environ Health Perspect, 111, 1148–51
- Blount BC, Silva MJ, Caudill SP, et al. (2000). Levels of seven urinary phthalate metabolites in a human reference population. Environ Health Perspect, 108, 979–82
- Boberg J, Christiansen S, Axelstad M, et al. (2011). Reproductive and behavioral effects of diisononyl phthalate (DINP) in perinatally exposed rats. Reprod Toxicol, 31, 200–9
- Boberg J, Metzdorff S, Wortziger R, et al. (2008). Impact of diisobutyl phthalate and other PPAR agonists on steroidogenesis and plasma insulin and leptin levels in fetal rats. Toxicology, 250, 75–81
- Boekelheide K, Kleymenova E, Liu K, et al. (2009). Dose-dependent effects on cell proliferation, seminiferous tubules, and male germ cells in the fetal rat testis following exposure to di(n-butyl) phthalate. Microsc Res Tech, 72, 629–38
- Bonilla E, Del MJ. (2010). Deregulation of the Sod1 and Nd1 genes in mouse fetal oocytes exposed to mono-(2-ethylhexyl) phthalate (MEHP). Reprod Toxicol, 30, 387–92
- Borch J, Axelstad M, Vinggaard AM, Dalgaard, M. (2006). Diisobutyl phthalate has comparable anti-androgenic effects to di-n-butyl phthalate in fetal rat testis. Toxicol Lett, 163, 183–90
- Burdorf A, Brand T, Jaddoe VW, et al. (2011). The effects of work-related maternal risk factors on time to pregnancy, preterm birth and birth weight: the Generation R Study. Occup Environ Med, 68, 197–204
- Calafat AM, McKee RH. (2006). Integrating biomonitoring exposure data into the risk assessment process: phthalates [diethyl phthalate and di(2-ethylhexyl) phthalate] as a case study. Environ Health Perspect, 114, 1783–9
- Carruthers CM, Foster PM. (2005). Critical window of male reproductive tract development in rats following gestational exposure to di-n-butyl phthalate. Birth Defects Res B Dev Reprod Toxicol, 74, 277–85
- Chou YY, Huang PC, Lee CC, et al. (2009). Phthalate exposure in girls during early puberty. J Pediatr Endocrinol Metab, 22, 69–77
- Cobellis L, Latini G, De FC, et al. (2003). High plasma concentrations of di-(2-ethylhexyl)-phthalate in women with endometriosis. Hum Reprod, 18, 1512–15
- Colon I, Caro D, Bourdony CJ, Rosario O. (2000). Identification of phthalate esters in the serum of young Puerto Rican girls with premature breast development. Environ Health Perspect, 108, 895–900
- Dalman A, Eimani H, Sepehri H, et al. (2008). Effect of mono-(2-ethylhexyl) phthalate (MEHP) on resumption of meiosis, in vitro maturation and embryo development of immature mouse oocytes. Biofactors, 33, 149–55
- Dalsenter PR, Santana GM, Grande SW, et al. (2006). Phthalate affect the reproductive function and sexual behavior of male Wistar rats. Hum Exp Toxicol, 25, 297–303
- Davis BJ, Maronpot RR, Heindel JJ. (1994a). Di-(2-ethylhexyl) phthalate suppresses estradiol and ovulation in cycling rats. Toxicol Appl Pharmacol, 128, 216–23
- Davis BJ, Weaver R, Gaines LJ, Heindel JJ. (1994b). Mono-(2-ethylhexyl) phthalate suppresses estradiol production independent of FSH-cAMP stimulation in rat granulosa cells. Toxicol Appl Pharmacol, 128, 224–8
- Ema, M. (2002). Antiandrogenic effects of dibutyl phthalate and its metabolite, monobutyl phthalate, in rats. Congenit Anom (Kyoto), 42, 297–308
- Ema M, Amano H, Itami T, Kawasaki, H. (1993a). Teratogenic evaluation of di-n-butyl phthalate in rats. Toxicol Lett, 69, 197–203
- Ema M, Harazono A, Miyawaki E, Ogawa Y. (1996a). Characterization of developmental toxicity of mono-n-benzyl phthalate in rats. Reprod Toxicol, 10, 365–72
- Ema M, Harazono A, Miyawaki E, Ogawa Y. (1996b). Developmental toxicity of mono-n-benzyl phthalate, one of the major metabolites of the plasticizer n-butyl benzyl phthalate, in rats. Toxicol Lett, 86, 19–25
- Ema M, Itami T, Kawasaki H. (1993b). Teratogenic phase specificity of butyl benzyl phthalate in rats. Toxicology, 79, 11–19
- Ema M, Kurosaka R, Amano H, Ogawa Y. (1995). Developmental toxicity evaluation of mono-n-butyl phthalate in rats. Toxicol Lett, 78, 101–6
- Ema M, Kurosaka R, Amano H, Ogawa Y. (1994). Embryolethality of butyl benzyl phthalate during early pregnancy in rats. Reprod Toxicol, 8, 231–6
- Ema M, Kurosaka R, Harazono A, et al. (1996c). Phase specificity of developmental toxicity after oral administration of mono-n-butyl phthalate in rats. Arch Environ Contam Toxicol, 31, 170–6
- Ema M, Miyawaki E. (2001a). Adverse effects on development of the reproductive system in male offspring of rats given monobutyl phthalate, a metabolite of dibutyl phthalate, during late pregnancy. Reprod Toxicol, 15, 189–94
- Ema M, Miyawaki E. (2001b). Effects of monobutyl phthalate on reproductive function in pregnant and pseudopregnant rats. Reprod Toxicol, 15, 261–7
- Ema M, Miyawaki E. (2002). Effects on development of the reproductive system in male offspring of rats given butyl benzyl phthalate during late pregnancy. Reprod Toxicol, 16, 71–6
- Ema M, Miyawaki E, Hirose A, Kamata, E. (2003). Decreased anogenital distance and increased incidence of undescended testes in fetuses of rats given monobenzyl phthalate, a major metabolite of butyl benzyl phthalate. Reprod Toxicol, 17, 407–12
- Ema M, Miyawaki E, Kawashima K. (1998). Reproductive effects of butyl benzyl phthalate in pregnant and pseudopregnant rats. Reprod Toxicol, 12, 127–32
- Ema M, Miyawaki E, Kawashima K. (1999). Developmental effects of plasticizer butyl benzyl phthalate after a single administration in rats. J Appl Toxicol, 19, 357–65
- Ema M, Miyawaki E, Kawashima K. (2000). Effects of dibutyl phthalate on reproductive function in pregnant and pseudopregnant rats. Reprod Toxicol, 14, 13–19
- Fisher JS, Macpherson S, Marchetti N, Sharpe RM. (2003). Human 'testicular dysgenesis syndrome': a possible model using in-utero exposure of the rat to dibutyl phthalate. Hum Reprod, 18, 1383–94
- Frederiksen H, Sorensen K, Mouritsen A, et al. (2012). High urinary phthalate concentration associated with delayed pubarche in girls. Int J Androl, 35, 216–26
- Fujii S, Yabe K, Furukawa M, et al. (2005). A two-generation reproductive toxicity study of diethyl phthalate (DEP) in rats. J Toxicol Sci, 30, 97–116
- Fulcher SM, Willoughby CR, Heath JA, et al. (2001). Developmental toxicity of di-(C(7)-C(9) alkyl) phthalate and di-(C(9)-C(11) alkyl) phthalate in the rat. Reprod Toxicol, 15, 95–102
- Grande SW, Andrade AJ, Talsness CE, et al. (2006). A dose-response study following in utero and lactational exposure to di(2-ethylhexyl)phthalate: effects on female rat reproductive development. Toxicol Sci, 91, 247–54
- Grande SW, Andrade AJ, Talsness CE, et al. (2007). A dose-response study following in utero and lactational exposure to di-(2-ethylhexyl) phthalate (DEHP): reproductive effects on adult female offspring rats. Toxicology, 229, 114–22
- Gray LEJr, Barlow NJ, Howdeshell KL, et al. (2009). Transgenerational effects of di (2-ethylhexyl) phthalate in the male CRL:CD(SD) rat: added value of assessing multiple offspring per litter. Toxicol Sci, 110, 411–25
- Gray LEJr, Laskey J, Ostby J. (2006). Chronic di-n-butyl phthalate exposure in rats reduces fertility and alters ovarian function during pregnancy in female Long–Evans hooded rats. Toxicol Sci, 93, 189–95
- Gray LEJr, Ostby J, Furr J, et al. (2000). Perinatal exposure to the phthalates DEHP, BBP, and DINP, but not DEP, DMP, or DOTP, alters sexual differentiation of the male rat. Toxicol Sci, 58, 350–65
- Guerra MT, Scarano WR, de Toledo FC, et al. (2010). Reproductive development and function of female rats exposed to di-eta-butyl-phthalate (DBP) in utero and during lactation. Reprod Toxicol, 29, 99–105
- Gunnarsson D, Leffler P, Ekwurtzel E, et al. (2008). Mono-(2-ethylhexyl) phthalate stimulates basal steroidogenesis by a cAMP-independent mechanism in mouse gonadal cells of both sexes. Reproduction, 135, 693–703
- Gupta RK, Singh JM, Leslie TC, et al. (2010). Di-(2-ethylhexyl) phthalate and mono-(2-ethylhexyl) phthalate inhibit growth and reduce estradiol levels of antral follicles in vitro. Toxicol Appl Pharmacol, 242, 224–30
- Hannas BR, Furr J, Lambright CS, et al. (2011). Dipentyl phthalate dosing during sexual differentiation disrupts fetal testis function and postnatal development of the male Sprague–Dawley rat with greater relative potency than other phthalates. Toxicol Sci, 120, 184–93
- Harris CA, Henttu P, Parker MG, Sumpter JP. (1997). The estrogenic activity of phthalate esters in vitro. Environ Health Perspect, 105, 802–11
- Hauser R, Duty S, Godfrey-Bailey L, Calafat AM. (2004). Medications as a source of human exposure to phthalates. Environ Health Perspect, 112, 751–3
- Hayashi K, Nakae A, Fukushima Y, et al. (2010). Contamination of rice by etofenprox, diethyl phthalate and alkylphenols: effects on first delivery and sperm count in mice. J Toxicol Sci, 35, 49–55
- Heindel JJ, Gulati DK, Mounce RC, et al. (1989). Reproductive toxicity of three phthalic acid esters in a continuous breeding protocol. Fundam Appl Toxicol, 12, 508–18
- Hernandez-Diaz S, Mitchell AA, Kelley KE, et al. (2009). Medications as a potential source of exposure to phthalates in the U.S. population. Environ Health Perspect, 117, 185–9
- Hirosawa N, Yano K, Suzuki Y, Sakamoto Y. (2006). Endocrine disrupting effect of di-(2-ethylhexyl)phthalate on female rats and proteome analyses of their pituitaries. Proteomics, 6, 958–71
- Hoshino N, Iwai M, Okazaki Y. (2005). A two-generation reproductive toxicity study of dicyclohexyl phthalate in rats. J Toxicol Sci, 30, 79–96
- Huang PC, Kuo PL, Chou YY, et al. (2009). Association between prenatal exposure to phthalates and the health of newborns. Environ Int, 35, 14–20
- Huang PC, Tsai EM, Li WF, et al. (2010). Association between phthalate exposure and glutathione S-transferase M1 polymorphism in adenomyosis, leiomyoma and endometriosis. Hum Reprod, 25, 986–94
- Hushka LJ, Waterman SJ, Keller LH, et al. (2001). Two-generation reproduction studies in Rats fed di-isodecyl phthalate. Reprod Toxicol, 15, 153–69
- Ito Y, Nakajima T. (2008). PPARalpha- and DEHP-Induced Cancers. PPAR Res, 20, 759–66
- Itoh H, Iwasaki M, Hanaoka T, et al. (2009). Urinary phthalate monoesters and endometriosis in infertile Japanese women. Sci Total Environ, 408, 37–42
- Jarfelt K, Dalgaard M, Hass U, et al. (2005). Antiandrogenic effects in male rats perinatally exposed to a mixture of di(2-ethylhexyl) phthalate and di(2-ethylhexyl) adipate. Reprod Toxicol, 19, 505–15
- Jiang J, Ma L, Yuan L, et al. (2007). Study on developmental abnormalities in hypospadiac male rats induced by maternal exposure to di-n-butyl phthalate (DBP). Toxicology, 232, 286–93
- Jobling S, Reynolds T, White R, et al. (1995). A variety of environmentally persistent chemicals. including some phthalate plasticizers, are weakly estrogenic. Environ Health Perspect, 103, 582–7
- Kim SH, Chun S, Jang JY, et al. (2011). Increased plasma levels of phthalate esters in women with advanced-stage endometriosis: a prospective case-control study. Fertil Steril, 95, 357–9
- Klimisch HJ, Gamer AO, Hellwig J, et al. (1992). Di-(2-ethylhexyl) phthalate: a short-term repeated inhalation toxicity study including fertility assessment. Food Chem Toxicol, 30, 915–19
- Koch HM, Calafat AM. (2009). Human body burdens of chemicals used in plastic manufacture. Philos Trans R Soc Lond B Biol Sci, 364, 2063–78
- Kurata Y, Makinodan F, Shimamura N, Katoh M. (2012). Metabolism of di (2-ethylhexyl) phthalate (DEHP): comparative study in juvenile and fetal marmosets and rats. J Toxicol Sci, 37, 33–49
- Laskey JW, Berman, E. (1993). Steroidogenic assessment using ovary culture in cycling rats: effects of bis(2-diethylhexyl)phthalate on ovarian steroid production. Reprod Toxicol, 7, 25–33
- Latini G, De FC, Presta G, et al. (2003). In utero exposure to di-(2-ethylhexyl)phthalate and duration of human pregnancy. Environ Health Perspect, 111, 1783–5
- Lee KY, Shibutani M, Takagi H, et al. (2004). Diverse developmental toxicity of di-n-butyl phthalate in both sexes of rat offspring after maternal exposure during the period from late gestation through lactation. Toxicology, 203, 221–38
- Lenie, S. & Smitz, J. (2009). Steroidogenesis-disrupting compounds can be effectively studied for major fertility-related endpoints using in vitro cultured mouse follicles. Toxicol Lett, 185, 143–52
- Lomenick JP, Calafat AM, Melguizo Castro MS, et al. (2010). Phthalate exposure and precocious puberty in females. J Pediatr, 156, 221–5
- Lopez-Carrillo L, Hernandez-Ramirez RU, Calafat AM, et al. (2010). Exposure to phthalates and breast cancer risk in northern Mexico. Environ Health Perspect, 118, 539–44
- Lovekamp TN, Davis BJ. (2001). Mono-(2-ethylhexyl) phthalate suppresses aromatase transcript levels and estradiol production in cultured rat granulosa cells. Toxicol Appl Pharmacol, 172, 217–24
- Lovekamp-Swan T, Jetten AM, Davis BJ. (2003). Dual activation of PPARalpha and PPARgamma by mono-(2-ethylhexyl) phthalate in rat ovarian granulosa cells. Mol Cell Endocrinol, 201, 133–41
- Ma M, Kondo T, Ban S, et al. (2006). Exposure of prepubertal female rats to inhaled di(2-ethylhexyl)phthalate affects the onset of puberty and postpubertal reproductive functions. Toxicol Sci, 93, 164–71
- McKee RH. (2004). Phthalate exposure and early thelarche. Environ Health Perspect, 112, A541–3
- McKee RH, Pavkov KL, Trimmer GW, et al. (2006). An assessment of the potential developmental and reproductive toxicity of di-isoheptyl phthalate in rodents. Reprod Toxicol, 21, 241–52
- Meeker JD, Hu H, Cantonwine DE, et al. (2009). Urinary phthalate metabolites in relation to preterm birth in Mexico city. Environ Health Perspect, 117, 1587–92
- Moore RW, Rudy TA, Lin TM, et al. (2001). Abnormalities of sexual development in male rats with in utero and lactational exposure to the antiandrogenic plasticizer Di(2-ethylhexyl) phthalate. Environ Health Perspect, 109, 229–37
- Moral R, Santucci-Pereira J, Wang R, Russo IH, Lamartiniere CA, Russo J. (2011). In utero exposure to butyl benzyl phthalate induces modifications in the morphology and the gene expression profile of the mammary gland: an experimental study in rats. Environ Health, 10, 5 . doi: 10.1186/1476-069X-10-5
- Moyer B, Hixon ML. (2012). Reproductive effects in F1 adult females exposed in utero to moderate to high doses of mono-2-ethylhexylphthalate (MEHP). Reprod Toxicol, 34, 43–50
- Mylchreest E, Cattley RC, Foster PM. (1998). Male reproductive tract malformations in rats following gestational and lactational exposure to Di(n-butyl) phthalate: an antiandrogenic mechanism?. Toxicol Sci, 43, 47–60
- Mylchreest E, Wallace DG, Cattley RC, Foster PM. (2000). Dose-dependent alterations in androgen-regulated male reproductive development in rats exposed to di(n-butyl) phthalate during late gestation. Toxicol Sci, 55, 143–51
- Nagao T, Ohta R, Marumo H, et al. (2000). Effect of butyl benzyl phthalate in Sprague–Dawley rats after gavage administration: a two-generation reproductive study. Reprod Toxicol, 14, 513–32
- Oishi S. (1985). Reversibility of testicular atrophy induced by Di(2-ethylhexyl) phthalate in rats. Environ Res, 36, 160–9
- Peters JM, Taubeneck MW, Keen CL, Gonzalez FJ. (1997). Di(2-ethylhexyl) phthalate induces a functional zinc deficiency during pregnancy and teratogenesis that is independent of peroxisome proliferator-activated receptor-alpha. Teratology, 56, 311–16
- Peters JW, Cook RM. (1973). Effect of phthalate esters on reproduction in rats. Environ Health Perspect, 3, 91–4
- Philippat C, Mortamais M, Chevrier C, et al. (2012). Exposure to phthalates and phenols during pregnancy and offspring size at birth. Environ Health Perspect, 120, 464–70
- Piersma AH, Verhoef A, te Biesebeek JD, et al. (2000). Developmental toxicity of butyl benzyl phthalate in the rat using a multiple dose study design. Reprod Toxicol, 14, 417–25
- Pocar P, Fiandanese N, Secchi C, et al. (2012). Exposure to di(2-ethyl-hexyl) phthalate (DEHP) in utero and during lactation causes long-term pituitary-gonadal axis disruption in male and female mouse offspring. Endocrinology, 153, 937–48
- Qiao L, Zheng L, Cai D. (2007). Study on the di-n-butyl phthalate and di-2-ethylhexyl phthalate level of girl serum related with precocious puberty in Shanghai. Wei Sheng Yan Jiu, 36, 93–95
- Rais-Bahrami K, Nunez S, Revenis ME, et al. (2004). Follow-up study of adolescents exposed to di(2-ethylhexyl) phthalate (DEHP) as neonates on extracorporeal membrane oxygenation (ECMO) support. Environ Health Perspect, 112, 1339–40
- Reddy BS, Rozati R, Reddy BV, Raman NV. (2006a). Association of phthalate esters with endometriosis in Indian women. BJOG, 113, 515–20
- Reddy BS, Rozati R, Reddy S, et al. (2006b). High plasma concentrations of polychlorinated biphenyls and phthalate esters in women with endometriosis: a prospective case control study. Fertil Steril, 85, 775–9
- Rhodes C, Orton TC, Pratt IS, et al. (1986). Comparative pharmacokinetics and subacute toxicity of di(2-ethylhexyl) phthalate (DEHP) in rats and marmosets: extrapolation of effects in rodents to man. Environ Health Perspect, 65, 299–307
- Rusyn I, Corton JC. (2012). Mechanistic considerations for human relevance of cancer hazard of di(2-ethylhexyl) phthalate. Mutat Res, 750, 141--58
- Saffarini CM, Heger NE, Yamasaki H, et al. (2012). Induction and persistence of abnormal testicular germ cells following gestational exposure to di-(n-butyl) phthalate in p53-null Mice. J Androl, 33, 505–13
- Saillenfait AM, Gallissot F, Sabate JP. (2009a). Differential developmental toxicities of di-n-hexyl phthalate and dicyclohexyl phthalate administered orally to rats. J Appl Toxicol, 29, 510–21
- Saillenfait AM, Gallissot F, Sabate JP. (2008a). Evaluation of the developmental toxicity of diallyl phthalate administered orally to rats. Food Chem Toxicol, 46, 2150–6
- Saillenfait AM, Roudot AC, Gallissot F, Sabate JP. (2011a). Prenatal developmental toxicity studies on di-n-heptyl and di-n-octyl phthalates in Sprague–Dawley rats. Reprod Toxicol, 32, 268–76
- Saillenfait AM, Roudot AC, Gallissot F, et al. (2011b). Developmental toxic potential of di-n-propyl phthalate administered orally to rats. J Appl Toxicol, 31, 36–44
- Saillenfait AM, Sabate JP, Gallissot F. (2009b). Effects of in utero exposure to di-n-hexyl phthalate on the reproductive development of the male rat. Reprod Toxicol, 28, 468–76
- Saillenfait AM, Sabate JP, Gallissot F. (2008b). Diisobutyl phthalate impairs the androgen-dependent reproductive development of the male rat. Reprod Toxicol, 26, 107–15
- Saillenfait AM, Sabate JP, Gallissot F. (2006). Developmental toxic effects of diisobutyl phthalate, the methyl-branched analogue of di-n-butyl phthalate, administered by gavage to rats. Toxicol Lett, 165, 39–46
- Saillenfait AM, Sabate JP, Gallissot F. (2003). Comparative embryotoxicities of butyl benzyl phthalate, mono-n-butyl phthalate and mono-benzyl phthalate in mice and rats: in vivo and in vitro observations. Reprod Toxicol, 17, 575–83
- Salazar V, Castillo C, Ariznavarreta C, et al. (2004). Effect of oral intake of dibutyl phthalate on reproductive parameters of Long Evans rats and pre-pubertal development of their offspring. Toxicology, 205, 131–7
- Schmidt JS, Schaedlich K, Fiandanese N, et al. (2012). Di(2-ethylhexyl) Phthalate (DEHP) impairs female fertility and promotes adipogenesis in C3H/N mice. Environ Health Perspect, 120, 1123--9
- Sekiguchi S, Ito S, Suda M, Honma T. (2006). Involvement of thyroxine in ovarian toxicity of di-(2-ethylhexyl) phthalate. Ind Health, 44, 274–9
- Seth PK, Srivastava SP, Agarwal DK, Chandra SV. (1976). Effect of di-2-ethylhexyl phthalate (DEHP) on rat gonads. Environ Res, 12, 131–8
- Shirota M, Saito Y, Imai K, et al. (2005). Influence of di-(2-ethylhexyl)phthalate on fetal testicular development by oral administration to pregnant rats. J Toxicol Sci, 30, 175–94
- Struve MF, Gaido KW, Hensley JB, et al. (2009). Reproductive toxicity and pharmacokinetics of di-n-butyl phthalate (DBP) following dietary exposure of pregnant rats. Birth Defects Res B Dev Reprod Toxicol, 86, 345–54
- Suzuki Y, Niwa M, Yoshinaga J, et al. (2010). Prenatal exposure to phthalate esters and PAHs and birth outcomes. Environ Int, 36, 699–704
- Svechnikova I, Svechnikov K, Soder O. (2007). The influence of di-(2-ethylhexyl) phthalate on steroidogenesis by the ovarian granulosa cells of immature female rats. J Endocrinol, 194, 603–9
- Tabacova S, Little R, Balabaeva L. (1999). Maternal exposure to phthalates and complications of pregnancy. Epidemiology, 10, S127
- Takai R, Hayashi S, Kiyokawa J, et al. (2009). Collaborative work on evaluation of ovarian toxicity. Two- or four-week repeated dose studies and fertility study of di-(2-ethylhexyl) phthalate (DEHP) in female rats. J Toxicol Sci, 34, SP111–19
- Tanaka T. (2002). Reproductive and neurobehavioural toxicity study of bis(2-ethylhexyl) phthalate (DEHP) administered to mice in the diet. Food Chem Toxicol, 40, 1499–506
- Tanaka T. (2003). Effects of bis(2-ethylhexyl) phthalate (DEHP) on secondary sex ratio of mice in a cross-mating study. Food Chem Toxicol, 41, 1429–32
- Toft G, Jonsson BA, Lindh CH, et al. (2012). Association between pregnancy loss and urinary phthalate levels around the time of conception. Environ Health Perspect, 120, 458–63
- Tomita I, Nakamura Y, Yagi Y, Tutikawa K. (1982). Teratogenicity/fetotoxicity of DEHP in mice. Environ Health Perspect, 45, 71–5
- Tomonari Y, Kurata Y, David RM, et al. (2006). Effect of di(2-ethylhexyl) phthalate (DEHP) on genital organs from juvenile common marmosets: I. Morphological and biochemical investigation in 65-week toxicity study. J Toxicol Environ Health A, 69, 1651–72
- Treinen KA, Dodson WC, Heindel JJ. (1990). Inhibition of FSH-stimulated cAMP accumulation and progesterone production by mono(2-ethylhexyl) phthalate in rat granulosa cell cultures. Toxicol Appl Pharmacol, 106, 334–40
- Tyl RW, Price CJ, Marr MC, Kimmel, CA. (1988). Developmental toxicity evaluation of dietary di(2-ethylhexyl)phthalate in Fischer 344 rats and CD-1 mice. Fundam Appl Toxicol, 10, 395–412
- Uriu-Adams JY, Kevin RC, Nguyen LK, et al. (2001). Effect of butyl benzyl phthalate on reproduction and zinc metabolism. Toxicology, 159, 55–68
- Walvoord EC. (2010). The timing of puberty: is it changing? Does it matter?. J Adolesc Health, 47, 433–9
- Wang W, Craig ZR, Basavarajappa MS, et al. (2012a). Di (2-ethylhexyl) phthalate inhibits growth of mouse ovarian antral follicles through an oxidative stress pathway. Toxicol Appl Pharmacol, 258, 288–95
- Wang W, Craig ZR, Basavarajappa MS, et al. (2012b). Mono (2-ethylhexyl) phthalate induces oxidative stress and inhibits growth of mouse ovarian antral follicles. Biol Reprod, 87, 152
- Ward JM, Peters JM, Perella CM, Gonzalez FJ. (1998). Receptor and nonreceptor-mediated organ-specific toxicity of di(2-ethylhexyl)phthalate (DEHP) in peroxisome proliferator-activated receptor alpha-null mice. Toxicol Pathol, 26, 240–6
- Waterman SJ, Keller LH, Trimmer GW, et al. (2000). Two-generation reproduction study in rats given di-isononyl phthalate in the diet. Reprod Toxicol, 14, 21–36
- Weuve J, Hauser R, Calafat AM, et al. (2010). Association of exposure to phthalates with endometriosis and uterine leiomyomata: findings from NHANES, 1999–2004. Environ Health Perspect, 118, 825–32
- Whyatt RM, Adibi JJ, Calafat AM, et al. (2009). Prenatal di(2-ethylhexyl)phthalate exposure and length of gestation among an inner-city cohort. Pediatrics, 124, e1213–20
- Willoughby CR, Fulcher SM, Creasy DM, et al. (2000). Two-generation reproduction toxicity studies of di-(C(7)-C(9) alkyl) phthalate and di-(C(9)-C(11) alkyl) phthalate in the rat. Reprod Toxicol, 14, 427–50
- Wine RN, Li LH, Barnes LH, et al. (1997). Reproductive toxicity of di-n-butylphthalate in a continuous breeding protocol in Sprague–Dawley rats. Environ Health Perspect, 105, 102–7
- Wittassek M, Koch HM, Angerer J, Bruning T. (2011). Assessing exposure to phthalates – the human biomonitoring approach. Mol Nutr Food Res, 55, 7–31
- Wolff MS, Engel SM, Berkowitz GS, et al. (2008). Prenatal phenol and phthalate exposures and birth outcomes. Environ Health Perspect, 116, 1092–7
- Wolff MS, Teitelbaum SL, Pinney SM, et al. (2010). Investigation of relationships between urinary biomarkers of phytoestrogens, phthalates, and phenols and pubertal stages in girls. Environ Health Perspect, 118, 1039–46
- Yamasaki K, Okuda H, Takeuchi T, Minobe Y. (2009). Effects of in utero through lactational exposure to dicyclohexyl phthalate and p,p′-DDE in Sprague–Dawley rats. Toxicol Lett, 189, 14–20
- Zhang Y, Jiang X, Chen B. (2004). Reproductive and developmental toxicity in F1 Sprague–Dawley male rats exposed to di-n-butyl phthalate in utero and during lactation and determination of its NOAEL. Reprod Toxicol, 18, 669–76
- Zhang Y, Lin L, Cao Y, et al. (2009). Phthalate levels and low birth weight: a nested case-control study of Chinese newborns. J Pediatr, 155, 500–4