Abstract
In the present era, gastroretentive dosage forms (GRDF) receive great attention because they can improve the performance of controlled release systems. An optimum GRDF system can be defined as a system which retains in the stomach for a sufficient time interval against all the physiological barriers, releases active moiety in a controlled manner, and finally is easily metabolized in the body. Physiological barriers like gastric motility and gastric retention time (GRT) act as obstacles in developing an efficient GRDF. Gastroretention can be achieved by developing different systems like high density systems, floating drug delivery systems (FDDS), mucoadhesive systems, expandable systems, superporous systems, and magnetic systems. All these systems have their own merits and demerits. This review focused on the various aspects useful in development of GRDF including the current trends and advancements.
Introduction
The oral route is the predominant and most preferable route for drug delivery, but drug absorption is unsatisfactory and highly variable in the individuals despite excellent in vitro release patterns (CitationDavis, 2005; CitationStreubel et al., 2006). The major problem is in the physiological variability such as gastrointestinal transit as well as GRT; the later plays a dominating role in overall transit of the dosage forms. GRT of the oral controlled release system is always less than 12 h (CitationChawla et al., 2003). These aspects lead to development of a drug delivery system which will remain in the stomach for a prolonged and predictable time.
Recent scientific and patent literature has shown increased interest in novel dosage forms that can be retained in the stomach for a prolonged and predictable period of time. One of the most feasible approaches for this in the gastrointestinal tract (GIT) is to control GRT using GRDF that will provide us with new and important therapeutic options. GRDF are designed on the basis of one of the several approaches like formulating low density dosage form that remain buoyant above the gastric fluid (FDDS) or high density dosage form that is retained at the bottom of the stomach, imparting bio-adhesion to the stomach mucosa, reducing motility of the GIT by concomitant administration of drugs or pharmaceutical exicipients, expanding the dosage form by swelling or unfolding to a large size which limits the emptying of the dosage form through the polymeric sphincter, utilizing ion–exchange resin which adheres to mucosa, or using a modified shape system.
Drug targeting to the stomach can also be attractive for several other reasons:
To produce a prolonged local action on to the gastrodudonal wall for e.g. drugs used in the eradication of H. pylori infection like amoxicillin (CitationBardronnet et al., 2006).
For weakly basic drugs with poor solubility in a basic environment.
For drugs which have poor stability in the colon.
For drugs which have a narrow absorption window.
For the drugs which have primarily absorption in the stomach.
From the formulation and technological point of view, FDDS is a considerably easy and logical approach in the development of GRDF. Hence, this review article focuses on the current technological development in FDDS with special emphasis on its potential for oral controlled drug delivery.
Gastrointestinal tract physiology
Stomach
The stomach is situated in the left upper part of the abdominal cavity immediately under the diaphragm. Its size varies according to the amount of distension: up to 1500 ml following a meal; after food has emptied, a collapsed state is obtained with resting volume of 25–50 ml (CitationWaugh & Grant, 2001). The stomach is anatomically divided into three parts: fundus, body, and antrum (or pylorus). The proximal stomach, made up of fundus and body regions, serves as a reservoir for the ingested materials, while the distal region (antrum) is the major site of mixing motions, acting as a pump to accomplish gastric emptying.
Gastrointestinal motility
Two distinct patterns of gastrointestinal motility and secretion exist corresponding to the fasted and fed states. As a result the bioavailability of orally administered drugs will vary depending on the state of feeding. In the fasted state, it is characterized by an inter-digestive series of electrical event and cycle, both through the stomach and small intestine every 2–3 h. This activity is called the interdigestive myoelectric cycle or Migrating motor complex (MMC). MMC is often divided into four consecutive phases: basal (Phase I), pre-burst (Phase II), burst (Phase III), and Phase IV intervals.
Phase I (basal phase) lasts from 40–60 min with rare contractions.
Phase II (pre-burst phase) lasts for 40–60 min with intermittent action potential and contractions. As the phase progresses the intensity and frequency also increases gradually.
Phase III (burst phase) lasts for 4–6 min. It includes intense and regular contractions for short periods. Due to this contraction all the undigested material is swept out of the stomach down to the small intestine. This is also known as the housekeeper wave.
Phase IV lasts for 0–5 min and occurs between phases III and I for two consecutive cycles.
The motor activity in the fed state is induced 5–10 min after the ingestion of a meal and persists as long as food remains in the stomach. The larger the amount of food ingested, the longer the period of fed activity, with usual time spans of 2–6 h, and more typically 3–4 h, with phasic contractions similar to Phase II of MMC.
Emptying of dosage form from the stomach
To achieve gastric retention, the dosage form must resist premature gastric emptying. For this, the dosage form must be able to withstand in the stomach against the force caused by peristaltic waves. Furthermore, once its purpose has been served the dosage form should be removed from the body with ease. explains the GIT transit time of various dosage forms.
Table 1. Transit times of various dosage forms across the GIT.
Factors affecting gastric retention (CitationSoppimath et al., 2001b; CitationArora et al., 2005; CitationJulan et al., 2005)
Density: GRT is a function of dosage form buoyancy that is dependent on the density.
Size: Dosage form units with a diameter of more than 7.5 mm are reported to have an increased GRT compared to those with a diameter of 9.9 mm.
Shape of dosage form: Tetrahedron and ring shaped unfolding expandable GRDF with a flexural modulus of 48 and 22.5 kilo pounds per square inch (KSI), respectively, are reported to have better GRT ≈ 90–100% retention at 24 h compared with other shapes like continuous stick, planar disc, planar multilobe, and string.
Single or multiple unit formulation: Multiple unit formulations show a more predictable release profile and insignificant impairing of the performance due to the failure of units, allow co-administration of units with different release profiles or containing incompatible substances, and permit a larger margin of safety against dosage form failure compared with single unit dosage forms.
Fed state: Under fasting conditions, the gastrointestinal motility is characterized by the periods of strong motor activity or the MMC that occur every 2–3 h. The MMC sweeps undigested material from the stomach and, if the timing of administration of formulation coincides with that of the MMC, then GRT of the unit may be expected to be very short. However, in the fed state, MMC is delayed and GRT is considerably longer.
Nature of meal: Feeding of indigestible polymers or fatty acid salts like cellulose, starch, polydextrose, and reffinose can change the motility pattern of the stomach by delaying the MMC, thus decreasing the gastric emptying rate and prolonging drug release.
Caloric content: GRT can be increased by 4–10 h with a meal that is high in proteins and fats.
Frequency of feed: The GRT can increase by over 400 min when successive meals are given compared with a single meal due to the low frequency of MMC.
Gender: It was observed that mean GRT in males (3.4 ± 0.6 h) is less than the female subjects (4.6 ± 1.2 h) of same age and race. Females emptied their stomach slowly in comparison to male candidates, regardless of their weight, height, and body surface area.
Age: Elderly people, especially those over 70, have a significantly longer GRT.
Posture: GRT can vary between supine and upright ambulatory states of the patient. For the floating systems it was reported that when subjects were kept in the upright ambulatory position the dosage form stayed continuously on gastric content in comparison to the supine state of the patients. Thus, in the upright position of the patients floating dosage forms protected against post-prandial emptying.
Concomitant drug administration: Clonidine, lithium, nicotine, progesterone, anti-cholinergics like atropine and propantheline, and opiates like codeine prolong GRT. On the other hand, erythromycin and octreotide enhance the gastric emptying.
GRDF
Dosage forms that can be retained in the stomach are called GRDF. Over the last two decades, numerous GRDF have been designed to prolong gastric residence time (CitationTalukder & Fassihi, 2004a). describes how the drug absorption takes place in the case of conventional dosage forms and GRDF.
Classification of GRDF
They may be broadly classified into:
High density systems;
Floating systems;
Expandable systems;
Superporous hydrogels;
Mucoadhesive or bioadhesive systems;
Magnetic systems; and
Dual working systems.
High density systems
These systems, which have a density of ∼ 3 g/cm3, are retained in the rugae of the stomach and are capable of withstanding its peristaltic movements. Above a threshold density of 2.4–2.8 g/cm3, such systems can be retained in the lower part of the stomach (CitationRouge et al., 1998). Results of a clinical study showed that an enteric-coated sinking ursodeoxycholic acid (UDCA) tablet formulation gives better bioavailability in comparison to enteric-coated floating tablets and hard gelatin capsule formulations of UDCA in 12 healthy subjects. The area under the curve [AUC, μmol/1 (8 h)] following oral administration of enteric-coated, sinking UDCA (39.0 ± 8.5) was significantly higher than that obtained after both conventional UDCA (30.5 ± 4.9) and floating enteric-coated UDCA (29.3 ± 3.4) (CitationSimoni et al., 1995). These systems comprise some drawbacks like, firstly, they are technically difficult to manufacture with a large amount of drug because the dry material of which it is made up of progressively reacts or interacts within the gastric fluid to release its drug contents and, secondly, till now, no such system is available on the market (CitationGarg & Sharma, 2003).
Swelling and expandable systems
The expandable GRDF are usually based on three configurations: a small (‘collapsed’) configuration which enables convenient oral intake; an expanded form that is achieved in the stomach and thus prevents passage through the pyloric sphincter; and finally another small form that is achieved in the stomach when retention is no longer required, i.e. after the GRDF has released its active ingredient, thereby enabling evacuation (CitationGroning et al., 2007).
The expansion can be achieved by swelling or by unfolding in the stomach (CitationKlausner et al., 2002). Swelling usually occurs because of osmosis. Unfolding takes place due to mechanical shape memory, i.e. the GRDF is fabricated in a large size and is folded into a pharmaceutical carrier, e.g. a gelatin capsule, for convenient intake. In the stomach, the carrier is dissolved and the GRDF unfolds or opens out to achieve extended configuration. The storage should maintain unfoldable properties for extended time spans (CitationKagan et al., 2006).
In spite of their interesting characteristics, expandable systems have drawbacks. Storage of such easily hydrolysable, biodegradable polymers is difficult (CitationTorrado et al., 2004). For the unfolding systems, the mechanical shape memory is relatively short-lived. Moreover, this kind of dosage form is probably the most difficult to industrialize and may not be cost-effective. Finally, expandable systems should not interfere with gastric motility, must be easily biodegradable, and must not have sharp edges or cause local damage on prolonged retention. Permanent retention of such rigid, large single-unit dosage forms may cause bowel obstruction, intestinal adhesion, and gastropathy (CitationKlausner et al., 2003c).
Mucoadhesive or bioadhesive systems
These systems permit a given drug delivery system to be incorporated with the bio/mucoadhesive agents, enabling the device to adhere to the stomach (or other gastrointestinal) walls, thus resisting gastric emptying. However, the mucus on the walls of the stomach is in a state of constant renewal, resulting in an unpredictable adherence (CitationChun et al., 2005).
A bio/mucoadhesive substance is a natural or synthetic polymer capable of adhering to biological membrane (bioadhesive polymer) or the mucus lining of the GIT (mucoadhesive polymer). Even though some of these polymers are effective at producing bioadhesion, it is very difficult to maintain it effectively because of the rapid turnover of mucus in the GIT (CitationUmamaheshwari et al., 2004). Furthermore, the stomach content is highly hydrated; decreasing the bioadhesiveness of polymers, and it is difficult to target specifically the gastric mucus with bioadhesive polymers. In addition, the possibility of esophageal binding might present a challenge regarding safety aspects (CitationWang et al., 2000).
Superporous hydrogel
Although these are swellable systems, they differ sufficiently from the conventional types to warrant separate classification. With pore size ranging between 10 nm to 10 µm, absorption of water by conventional hydrogels is a very slow process and several hours may be needed to reach an equilibrium state during which premature evacuation of the dosage form may occur. Superporous hydrogels, average pore size > 100 µm, swell to equilibrium within a minute, due to rapid water uptake by capillary wetting through numerous interconnected open pores. Moreover, they swell to a large size and are intended to have sufficient mechanical strength to withstand pressure by the gastric contraction (CitationPark et al., 2005).
Magnetic systems
The magnetic dosage forms contain a small internal magnet and an extra-corporal magnet that controls the gastrointestinal transit of the dosage form (CitationGroning et al., 1998). Although these systems seem to work, the external magnet must be positioned with a degree of precision that might compromise patient compliance.
FDDS
Floating systems, first described by CitationDavis in 1968, are low-density systems that have sufficient buoyancy to float over the gastric contents and remain in the stomach for a prolonged period. While the system floats over the gastric contents, the drug is released slowly at the desired rate, which results in increased GRT and reduces fluctuation in plasma drug concentration (CitationGangadharappa et al., 2007).
Advantages of FDDS
Improves patient compliance by decreasing dosing frequency.
Better therapeutic effect of short half-life drugs can be achieved.
Gastric retention time is increased because of buoyancy.
Drug releases in a controlled manner for a prolonged period.
Site-specific drug delivery to stomach can be achieved.
Enhanced absorption of drugs which solubilize only in the stomach.
Superior to single unit floating dosage forms as such microspheres release drug uniformly and there is no risk of dose dumping.
Limitations of FDDS
High level of fluids in the stomach is required for maintaining buoyancy; float efficient working of dosage form.
Not feasible for drugs having solubility or stability problems in gastric fluid.
Drugs such as nifedipine, which is well absorbed along the entire GIT and which undergoes significant first-pass metabolism, may not be desirable candidates for FDDS since the slow gastric emptying may lead to reduced systemic bioavailability.
Limitations to the applicability of FDDS for drugs that are irritating gastric mucosa.
Formulation requirements for FDDS
The device must comply with the following criteria:
It must have sufficient structure to form a cohesive gel barrier.
It must maintain an overall specific gravity lower than that of gastric contents (1.004–1.010).
It should dissolve slowly enough to serve as a drug reservoir.
Classification of FDDS
Floating systems can be classified as effervescent and non-effervescent systems.
Effervescent systems
Flotation of a drug delivery system in the stomach can be achieved by incorporating a floating chamber filled with vacuum, air, or an inert gas (CitationPatel et al., 2005). Gas can be introduced into the floating chamber by the volatilization of an organic solvent (e.g. ether or cyclopentane) or by the CO2 produced as a result of an effervescent reaction between organic acids and carbonate–bicarbonate salts. These devices contain a hollow deformable unit that converts from a collapsed to an expanded position and returns to the collapsed position after a pre-determined amount of time to permit the spontaneous ejection of the inflatable system from the stomach. describes a multiple-unit oral floating drug delivery system and explains the working principle of an effervescent floating drug delivery system.
Figure 2. (A) Multiple-unit oral floating drug delivery system. (B) Working principle of effervescent floating drug delivery system.
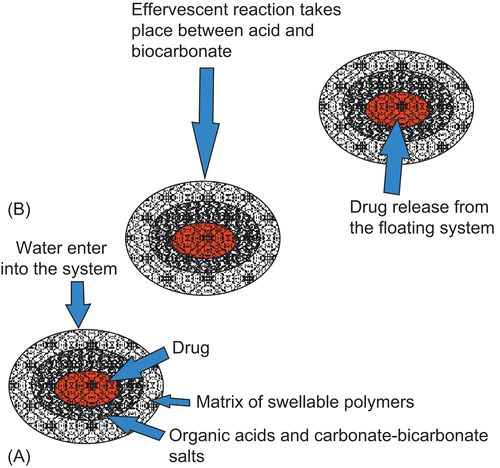
These buoyant systems utilize matrices prepared with swellable polymers like methocel, polysaccharides like chitosan, effervescent components like sodium bicarbonate, citric acid and tartaric acid, or chambers containing a liquid that gasifies at body temperature. The common approach for preparing these systems involves resin beads loaded with bicarbonate and coated with ethylcellulose. The coating, which is insoluble but permeable, allows permeation of water. Thus, carbon dioxide is released, causing the beads to float in the stomach.
CitationStrubing et al. (2008a) investigated the mechanism of floating and drug release behaviour of poly(vinyl acetate)-based floating tablets with membrane controlled drug delivery. Benchtop MRI studies of selected samples were performed and the results suggested that the drug release was delayed efficiently within a time interval of 24 h by showing linear drug release characteristics.
CitationSungthongjeen et al. (2008) designed floating multi-layer coated tablets based on gas formation using acrylic polymers (Eudragit RL 30D, RS 30D, NE 30D) and ethylcellulose. The effect of formulation variables on floating properties and drug release was investigated and the results showed that an increase in amount of a gas forming agent did not affect the time to float, but increased the drug release from the floating tablets.
CitationPatel et al. (2007a) used hydroxypropyl methylcellulose, ethyl cellulose, and sodium bicarbonate to prepare floating tablets and optimization was done using a simplex lattice design. All the tablet formulations remained buoyant for more than 12 h and the release profile of the optimized batch fitted best to the zero order model.
CitationJaimini et al. (2007) prepared floating tablets of famotidine by employing two different grades of methocel K100 and methocel K15M by effervescent technique. It was observed that the tablet remained buoyant for 6–10 h and the drug release from the tablets was sufficiently sustained.
CitationShishu et al. (2007a) developed a FDDS using gas-forming agents, like sodium bicarbonate, citric acid, and hydrocolloids, like hydroxypropyl methylcellulose (HPMC) and Carbopol 934P. The results of the in vitro release studies showed that the optimized formulation could sustain drug release for 24 h and remain buoyant for 16 h.
CitationNakagawa et al. (2006) developed a novel intra-gastric FDDS by pulsed plasma-irradiation on the double-compressed tablet of 5-Fluorouracil as a core material with outer layer composed of a 68/17/15 weight ratio of Povidone, Eudragit RL, and sodium bicarbonate, and the result showed that the release of 5-Fluorouracil from the tablet was sustained by occurrence of a plasma-induced cross-link reaction on the outer layer of the tablet
CitationAtyabi et al. (1996) prepared ion exchange resin beads loaded with bicarbonate and coated with a semi-permeable membrane. These prepared beads exhibit prolonged gastric residence due to the release of carbon dioxide which is trapped inside the coating of the beads.
Non-effervescent systems
Non-effervescent systems incorporate a high level (20–75% w/w) of one or more gel-forming, highly swellable, cellulosic hydrocolloids (e.g. hydroxyethyl cellulose, hydroxypropyl cellulose, hydroxypropyl methylcellulose, and sodium carboxymethylcellulose), polysaccharides, or matrix-forming polymers (e.g. polycarbophil, polyacrylates, and polystyrene) into tablets or capsules. On contact with gastric fluid, these gel former polysaccharides and polymers hydrate and form a colloidal gel barrier that controls the rate of fluid penetration into the device and consequent drug release. As the exterior surface of the dosage form dissolves, the gel layer is maintained by the hydration of the adjacent hydrocolloid layer. The air trapped by the swollen polymer lowers the density and confers buoyancy to the dosage form.
Hydrodynamically balanced systems (HBS) are best suited for drugs having a better solubility in an acidic environment and also for the drugs having a specific site of absorption in the upper part of the small intestine (CitationRocca et al., 2003). HBS systems can remain in the stomach for long periods and hence can release the drug over a prolonged period of time. Hence, the problem of short gastric residence time encountered with an oral controlled release formulation can be overcome with these systems. These systems have a bulk density less than gastric fluid as a result of which they can float on the gastric contents. These systems are relatively large in size and passing from the pyloric opening is prohibited.
These single unit dosage forms contain one or more gel-forming hydrophilic polymers. Hydroxypropylmethylcellulose is the most common used excipient, although hydroxyethylcellulose, hydroxypropylcellulose, sodium carboxymethycellulose, agar, carrageenans or alginic acid are also used. The polymer is mixed with drug and usually administered in a gelatin capsule. The capsule rapidly dissolves in the gastric fluid, and swelling of the surface polymers produces a floating mass. Drug release is controlled by the formation of a hydrated boundary at the surface. Continuous erosion of the surface allows water penetration to the inner layers, maintaining surface hydration and buoyancy.
HBS enjoys several advantages, including ease of manufacturing and excellent uniformity of matrix bed. Various in-vivo studies like radiographic (2008) and gamma scintigraphy (CitationAli et al., 2007a; Citationb) have been done to evaluate in-vivo buoyancy of these systems. CitationQureshi, et al. (2007) prepared a HBS system of celiprolol hydrochloride using various low density polymers. Optimization was done on the basis of in vitro buoyancy and release and the capsule prepared with Hydroxypropylmethylcellulose K4M and liquid paraffin gave the best release profile. In another study, CitationMendyk et al. (2006) used artificial neural networks as modeling tools for prediction of various drugs release patterns from HBS composed with Metholose 90SH (hydroxypropylmethylcellulose). It was found that artificial neural networks are capable to accurately predict release patterns of different drugs from HBS based on the description of the formulation as well as the chemical structure of the drug.
The main drawback is the passivity of the operation. This depends on the air sealed in the dry mass centre following hydration of the gelatinous surface layer and hence the characteristics and amount of polymer.
A fluid-filled floating chamber (CitationZou et al., 2007) includes incorporation of a gas-filled floatation chamber into a microporous component that houses a drug reservoir. Apertures or openings are present along the top and bottom walls through which the gastrointestinal tract fluid enters to dissolve the drug. The other two walls in contact with the fluid are sealed so that the undissolved drug remains therein. The fluid present could be air, under partial vacuum or any other suitable gas, liquid, or solid having an appropriate specific gravity and an inert behavior. The device is of swallowable size, remains afloat within the stomach for a prolonged time, and after the complete release the shell disintegrates, passes off to the intestine, and is eliminated.
In situ gelling system
Here, gel forming solution swells and forms a viscous cohesive gel containing entrapped CO2 bubbles on contact with gastric fluid. Formulations also typically contain antibiotics and antacids. Because in situ gelling systems produce a layer on the top of gastric fluid, they are often used for gastroesophageal reflux treatment. CitationRajinikanth and Mishra (2008) prepared a floating in situ gelling system of clarithromycin to eradicate H. pylori using gellan as a gelling polymer and calcium carbonate as a floating agent. The in vivo H. pylori clearance efficiency of the prepared system and clarithromycin suspension following oral administration, to H. pylori infected Mongolian gerbils was examined by polymerase chain reaction technique and by a microbial culture method. A floating in situ gelling system showed a more significant anti-H. Pylori effect than that of the clarithromycin suspension.
Low-density systems
Low density systems inevitably have a lag time before floating on the stomach contents, during which the dosage form may undergo premature evacuation through the pyloric sphincter. Low density systems (< 1 g/cm3) with immediate buoyancy have therefore been developed. They are made of low-density materials, entrapping oil or air (CitationGroning et al., 2007). Most are multiple unit systems, and are also called ‘microballoons’ (hollow microspheres) because of the low-density core. Microspheres have a characteristic internal hollow structure and show an excellent in vitro floatability (CitationTanwar et al., 2007a). These microspheres are characteristically free flowing powders consisting of proteins or synthetic polymers, ideally having a size less than 200 μm. Microspheres have high loading capacity (CitationDaharwal et al., 2005) and many polymers have been used such as albumin, gelatin, starch, polymethacrylate, polyacrylamine, and polyalkylcyanoacrylate. Solid biodegradable microspheres incorporating a drug dispersed or dissolved throughout particle matrix have the potential for controlled release of drugs.
Various types of tablets (bilayered and matrix) are tested to have floatable characteristics. Some of the polymers used are hydroxypropyl cellulose, hydroxypropyl methylcellulose, crosspovidone, sodium carboxymethyl cellulose, ethyl cellulose eudragit RS PO, and eudragit EPO (CitationNarendra et al., 2006).
The 3-layer principle (CitationYang et al., 1999) has been used for the development of an asymmetric configuration drug delivery system in order to modulate the release extent and achieve zero-order release kinetics by initially maintaining a constant area at the diffusing front with subsequent dissolution/erosion towards the completion of the release process.
The effect of formulation and processing variables on buoyancy and drug release behavior has been studied by a number of investigators (CitationShimpi et al., 2004; CitationWakode & Bajaj, 2008). CitationStrubing et al. (2008b) developed floating Kollidon® SR matrix tablets containing Propranolol. Tablet floating started immediately and continued for 24 h. Floating strength was related to Kollidon® SR level with improved floating characteristics for samples with a high polymer/drug ratio. CitationPatel et al. (2007b) and CitationPatel and Patel (2007) prepared floating granules of ranitidine HCL using compritol, gelucire 50/13, and gelucire 43/01 as a lipid carrier via melt granulation technique. A full 32 factorial was used for optimization. The results revealed that the moderate amount of gelucire 43/01 and ethyl cellulose provides desired release of ranitidine HCL from the developed floating granules. In another study they described influence of viscosity of hydroxypropyl methylcellulose, and types of filler on release from floating matrix tablets using 32 full factorial design. It was observed that as viscosity of polymer increases, the release rate constant was decreased. CitationRaval et al. (2007) developed a matrix controlled drug delivery system consisting of a poly (styrene-divinyl benzene) copolymer, a matrix-forming polymer, and this highly porous copolymer provided a low density and, thus, excellent in vitro floating behavior of the tablets at a concentration of 15% (w/w). CitationSoppimath et al. (2006) determined the effect of co-excipients on drug release and floating property of the hollow microspheres containing nifedipine. Microspheres floated on simulated gastric fluid for more than 12 h and their buoyancy followed the rank order of: blank (no-coexcipient) > dibutylpathalate > polyethyleneglycol > poly(έ-caprolactone) after15 h of floating and the drug was released in a controlled manner. CitationChauhan et al. (2004) developed risedronate sodium and Gelucire® 39/01 floating matrices using melt solidification techniques. Ageing of the matrices was studied by differential scanning calorimetry, and in vitro drug release. Ageing causes changes in the crystal structure of Gelucire®, which is responsible for an increase in drug release. CitationDave et al. (2004) investigated the effect of stearic acid and citric acid on drug release from floating tablets. The results showed that low amount of citric acid and high amount of stearic acid favors sustained release of drug from the system. CitationIannuccelli et al. (2000) prepared a floating multiple unit system with solid dispersion of furosemide with polyvinylpyrrolidone. A decrease in crystallinity of furosemide was observed by making solid dispersion; thereby 15-fold or 20-fold increased both solubility and dissolution of furosemide over that for untreated furosemide. CitationDurig and Fassihi (2000) developed swellable hydrocolloid (guar) based matrix tablets containing verapamil HCl, which were evaluated using USP dissolution apparatus I and II. Results concluded that a double mesh device may provide an alternative to current compendial dissolution methods when the reliable determination of the true release kinetics of floating and sticking delivery systems is desired. CitationKrogel and Bodmeier (1999) developed a multi-functional drug delivery system based on hydroxypropyl methylcellulose (HPMC)-matrices (tablets) placed within an impermeable polymeric cylinder (open at both ends). The results showed that the drug release increased with a reduced HPMC viscosity grade, higher aqueous drug solubility, decreased HPMC content, and the increased surface area of the matrix. CitationRouge et al. (1997) developed hydrophillic mini-matrices filled into hard gelatin capsules which disperse and float on the contents of the stomach. The degree of dispersion of the mini-tablets was described using an equation based on the actual area generated by the individual or aggregated mini-tablets upon contact with the dissolution medium. CitationStreubel et al. (2003) prepared floating matrix tablets based on low density foam powder. The release rate could effectively be modified by varying the ‘matrix-forming polymer/foam powder’ ratio; the initial drug loading and the floating behavior of the low density drug delivery systems could successfully be combined with an accurate control of the drug release patterns. CitationSawicki and Lunio (2005) prepared floating pellets of verapamil hydrochloride as model drug. The best formulation was evaluated taking into account the effect of compression force and tablet hardness and friability, and pellet agglomeration and flotation.
In the last decade, the Emulsification Solvent Evaporation (CitationMuthusamy et al., 2005; CitationVarshosaz et al., 2007; CitationNepal et al., 2007; CitationChoudhury et al., 2008) method for preparation of low density systems achieved tremendous popularity. Numbers of investigations have been done employing this particular method, and floating microspheres (CitationStithit et al., 1998; CitationSoppimath et al., 2001a) were primarily dosage form of choice. The effect of formulation and process variables such as: polymer type, drug and polymer ratio, type of solvent, organic solvents ration, concentration of plasticizer in aqueous phase, temperature of aqueous phase, stirring rate, time of stirring were evaluated on the yield, particle size, loading, release, and floating behavior of microspheres (CitationLeet et al., 1999; CitationStreubel et al., 2002; CitationSato et al., 2004). Shape and internal hollow cavity of floating microspheres were evaluated by SEM and optical microscopy, and it was found that prepared systems were perfect spheres with an internal hollow cavity enclosed by a rigid shell of polymers (CitationSrivastava et al., 2005; CitationKale & Tyade, 2007). Some in-vivo studies suggested that developed formulations remained buoyant over a long period of time in the gastric cavity (CitationJain et al., 2006a) and encapsulated active moiety showed a high bioavailability (CitationJoseph et al., 2002; CitationSato et al., 2003). Drug encapsulated in polymer matrix followed different release patterns like zero order release (CitationGibley, 2002), Higuchi matrix model, and Pappas Korsmeyer model (CitationJain et al., 2006b). Ionic gelation method, for preparation of floating beads (CitationWhitehead et al., 1998; CitationTalukder & Fassihi, 2004b, CitationPawar et al., 2008), was also adopted by a number of investigators. Different classes of pharmacotherapeutic agents were encapsulated in these systems, like anti-bacterial agent metronidazole (CitationMurata et al., 2000; CitationSriamornsak et al., 2005; CitationIshak et al., 2007), anti-cancer agent like 5-fluorouracil (CitationShishu et al., 2007b), non-steroidal anti-inflammatory drug like piroxicam (CitationLee et al., 2001), anti-hypertensive agent like verapamil hydrochloride (CitationSawicki, 2002), and bronchodilator like theophylline (CitationYang et al., 2004). In a study (CitationGohel & Sarvaiya, 2007) it was revealed that gastroretentive tablets of rifampicin and isoniazid minimize the degradation of rifampicin and isoniazid in acidic medium because the developed system minimized the physical contact between the two drugs and also controlled release rifampicin in acidic medium. Gastroretentive systems are gaining more popularity day-by-day, which can be easily seen by availability of a number of commercialized gastroretentive products in the market. From them some are listed in .
Table 2. Different marketed product of GRDF.
Single and multiparticulate systems of FDDS
Unfortunately, floating devices administered in a single unit form such as HBS are unreliable in prolonging the GRT owing to their ‘all or none’ emptying process, and, thus, they may cause high variability in bioavailability and local irritation due to the large amount of drug delivered at particular sites of GIT (CitationSingh & Kim, 2000). In contrast, multiple unit particulate doses forms (e.g. microspheres) have the advantages that they pass uniformly through the GIT to avoid the vagaries of gastric emptying and provide an adjustable release, thereby reducing the inter-subject variability in absorption and risk of local irritation. At present, hollow microspheres are considered to be one of the most promising buoyant systems as they combine the advantages of multiple systems with good floating properties.
Current trends and advancements
Dual working systems
These systems are based on the two working principles of either floating and bioadhesion or swelling and bioadhesion. FDDS are formulated to persist floating on the gastric fluid when the stomach is full after a meal. However, as the stomach empties and the tablet reaches the pylorus, the buoyancy of the dosage form may be reduced. It may be that the dosage form will then pass through the pylorus into the small intestine. Thus, the buoyancy of an FDDS in the stomach may be limited to only 3–4 h. Furthermore, floating systems do not always release the drug at the intended site. In a bioadhesive drug delivery system, it is quite likely that the system becomes dislodged from the stomach mucosa wall when the system is full and the semi-liquid contents are churning around due to the effect of peristalsis. A dual working system would overcome drawbacks associated with bioadhesive, swelling, and floating systems, and would have a significant effect on improving the therapeutic effect of the drug involved. CitationSonar et al. (2007) developed a bilayer and floating-bioadhesive drug delivery system exhibiting a unique combination of floatation and bioadhesion to prolong residence in the stomach using rosiglitazone maleate as a model drug. The release of rosiglitazone maleate from the tablets followed the matrix first-order release model. The tablet was buoyant for up to 8 h in the human stomach. CitationVarshosaz et al. (2006) prepared floating-bioadhesive tablets of ciprofloxacin using sodium carboxymethylcellulose, polyacrylic acid, citric acid, and sodium bicarbonate. All the tablets were floated for more than 23–24 h and increasing sodium carboxymethylcellulose caused higher mucoadhesion than polyacrylic acid. CitationZheng et al. (2006) developed floating-bioadhesive microparticles of clarithromycin for the eradication of H. pylori using ethylcellulose and chitosan. In vivo mucoadhesion testing showed that 61% of the microparticles could be retained in the stomach for 4 h. CitationChavanpatil et al. (2006) developed a swellable and bioadhesive formulation of ofloxacin using psyllium husk, hydroxypropylmethylcellulose, and crosspovidone. The swelling property was increased with the increasing concentration of crosspovidone. The bioadhesive property of the developed formulation was found to be significantly increasing in combination as compared to the hydroxypropylmethylcellulose and psyllium husk alone. CitationUmamaheshwari et al. (2002; Citation2003) developed floating-bioadhesive dosage forms of acetohydroxamic acid coated with cellulose acetate butyrate. The cellulose acetate butyrate coated dosage form showed higher buoyancy time than uncoated resin particles, but the amount of cellulose acetate butyrate coated microcapsules that remained in the stomach was slightly lower than that of uncoated resin particles. Results of in vitro growth inhibition studies suggested a better inhibition rate than the plain acetohydroxamic acid.
Floating osmotic systems
A floating osmotic drug delivery system employs the principal of osmotic pressure to float on the gastric fluid. Basically these systems comprise of three parts; an osmotic core (containing drug reservoir, osmotic agents, and other excipients), a shape retaining semi-permeable membrane; and an outer compression coating consisting of gas generating and gel forming agents. For delivery of drug an orifice is bored through both the outer layers. After administration when this system comes in contact with gastric fluid, initially CO2 is generated due to the presence of a gas forming agent and this generated gas entraps within the bed of swelled gel, thus the system became buoyant due to diminished density. Delivery of drug then totally depends upon the osmotic pressure generated inside the osmotic core. First a saturated solution of drug is formed due to the flow of fluid through the semi-permeable membrane and second expulsion of drug through the orifice due to osmotic pressure develops within the osmotic core. A major advantage of floating osmotic drug delivery systems is that they deliver drug independent to physiological parameters like pH of gastric fluid (CitationKumar et al., 2008).
Floating-pulsatile systems
Pulsatile drug delivery systems release the drug rapidly and completely after certain lag times. However, an uncertainty is always associated with such systems, they may expel out from the body without releasing drug content due to the presence of lag time. Floating pulsatile systems develop to overcome this drawback and have gained increasing interest during recent years for a number of drug therapies. In a study CitationZou et al. (2008) developed a floating-pulsatile drug delivery system of verapamil HCL. The dry-coated tablet consists of a drug-containing core coated with a hydrophilic erodible polymer followed by a buoyant layer, prepared with Methocel K4M, carbopol 934P, and sodium bicarbonate. Developed formulations were evaluated for their buoyancy and a dissolution, pharmacokinetic, and gamma-scintigraphic study was also done. Results showed that the prepared system remained in gastric fluid and gave rise to controlled release of drug. CitationBadve et al. (2007) developed hollow calcium pectinate beads for floating-pulsatile release of diclofenac sodium. The floating beads obtained were porous (34% porosity), hollow with bulk density < 1, and had a floating time of 14–24 h. In vivo studies by gamma scintigraphy determined on rabbits showed gastroretention of beads up to 5 h.
In-vivo methods of evaluation of GRDF (CitationParikh & Amin, 2008)
γ-Scintigraphy
γ-Scintigraphy can be use to evaluate in-vivo buoyancy and in-vivo release performance of different type of GRDF. In this technology a stable radioisotope like 111In is formulated within the developed system and administered in healthy human volunteers (CitationGoole et al., 2008). Major drawbacks with such a technique are associated ionization radiations, limited topographic information, low resolution, and complicated and expensive preparation of radiopharmaceuticals (CitationWilding et al., 2001).
Radiology
This method includes pre-clinical estimation of gastroretention. In comparison to γ-scintigraphy, radiology is a more simple and cost effective technique. However, limitations regarding exposure to X-rays decline its popularity because for optimum evaluation of buoyancy a high amount of contrasting agent (BaSo4) is generally required (CitationTanwar et al., 2007b). Radiographs were taken after ingestion of the dosage form, to locate the floating and non-floating (fabricated) dosage forms at various periodic time intervals (CitationIannuccelli et al., 1998; CitationBaumgartner, et al., 2000; CitationKlausner et al., 2003a).
Gastroscopy
This is considered a minimally invasive procedure since it does not require an incision into one of the major body cavities. This technique involves visual inspection of GRDF in the stomach (CitationKlausner et al., 2003b). Basically it is a type of peroral endoscopy which comprises optic-fibers and a video camera. For more detailed information the evaluated system can be drawn out from the stomach. However, on the other hand the quality of study and its interpretation are highly dependent on the expertise of the endoscopist. Active uncontrolled bleeding, retained blood in the stomach, and retained food or antacids may also lead to an inadequate study.
Ultrasonography
Ultrasonic waves are used to produce images of body structures. The waves travel through tissues and are reflected back where density differs. The reflected echoes are received by an electronic apparatus that measures their intensity level and the position of the tissue reflecting them. The results can be displayed as still images or as a moving picture of the inside of the body (CitationHendee, 1994). Most dosage forms do not have sharp acoustic mismatches across their interface with the physiological milieu. Therefore, ultrasonography is not routinely used for the evaluation of FDDS. The characterization included assessment of intra-gastric location of the hydrogels, solvent penetration into the gel, and interactions between the gastric wall and FDDS during peristalsis.
Magnetic resonance imagining (MRI)
MRI is a non-invasive diagnostic technology. MRI uses a powerful magnetic field, radio frequency pulses, and a computer to produce detailed pictures of organs, soft tissues, bone, and virtually all other internal body structures. The images can then be examined on a computer monitor, transmitted electronically, and printed or copied to a CD. MRI does not use ionizing radiation (x-rays). In the last couple of years, MRI was shown to be a valuable tool in gastrointestinal research for the analysis of gastric emptying, motility, and intra-gastric distribution of macronutrients and drug models. The advantages of MRI include high soft tissue contrast, high temporal and spatial resolution, as well as a lack of ionizing irradiation. Also, harmless paramagnetic and supra-magnetic MR imaging contrast agents can be applied to specifically enhance or suppress signal of fluids and tissues of interest and thus permit better delineation and study of organs (CitationDorozynski et al., 2007).
Conclusion
Increased GRT of a controlled release system has great practical applications. A controlled release system with gastroretentive ability can significantly improve bioavailability and improve the efficiency of medical treatment. These systems also meliorate the efficacy of drugs which have altered stability, solubility, and absorption in GIT. All the gastroretentive systems have their positive aspects and drawbacks. Concerning this floating drug delivery system based on effervescent and non-effervescent systems for modulation of oral controlled drug delivery was found to be of great importance. These systems have special additional advantages for the drugs that are primarily absorbed from the upper segment of the GIT. It is also evident that the maximum number of commercial products and patents of gastroretentive systems belong to the class of floating drug delivery systems. So, with an improved knowledge of formulation aspects and physiochemical and pharmacological prospects of drugs someone can design an optimum system for drug delivery in the gastric cavity.
Declaration of interest
The author reports no declaration of interest.
References
- Ali, J., Arora, S., Ahuja, A., Babbar, A.K., Sharma, R.K., Khar, R.K. (2007a). Formulation and development of floating capsules of celecoxib: in vitro and in vivo evaluation. AAPS PharmSciTech. 8: Article 119. Available online at: http://www.aapspharmscitech.org, accessed on 8 Sep, 2009.
- Ali, J., Arora, S., Ahuja, A., Babbar, A.K., Sharma, R.K., Khar, R.K., Baboota, S. (2007b). Formulation and development of hydrodynamically balanced system for metformin: in vitro and in vivo evaluation. Eur J Pharm Biopharm. 67:196–201.
- Arora, S., Ali, J., Ahuja, A., Khar, R.K., Baboota, S. (2005). Floating drug delivery system: a review. AAPS PharmSciTech. 6: Article-47. Available online at: http://www.aapspharmscitech.org, accessed on 8 Sep, 2009.
- Atyabi, F., Sharma, H.L., Mohammad, H.AH., Fell, J.T. (1996). Controlled drug release from coated floating ion exchange resin beads. J Contr Rel. 48:25–8.
- Badve, S.S., Sher, P., Korde, A., Pawar, A.P. (2007). Development of hollow/porous calcium pectinate beads for floating-pulsatile drug delivery. Eur J Pharm Biopharm. 65:85–93.
- Bardronnet, P., Faivre, V., Pugh, W.J., Piffaretti, J.C., Falson, F. (2006). Gastroretentive dosage forms: overview and special case of Helicobacter pylori. J Contr Rel. 111:1–18.
- Baumgartner, S., Krist, J., Vreer, F., Vodopivec, P., Zorko, B. (2000). Optimisation of floating matrix tablets and evaluation of their gastric residence time. Int J Pharm. 195:125–35.
- Chauhan, B., Shimpi, S., Mahadik, K.R., Paradkar, A. (2004). Preparation and evaluation of floating risedronate sodium Gelucire® 39/01 matrices. Acta Pharm. 54:205–14.
- Chavanpatil, M.D., Jain, P., Chaudhari, S., Shear, R., Vavia, P.R. (2006). Novel sustained release swellable and bioadhesive gastroretentive drug delivery system for ofloxacin. Int J Pharm. 316:86–2.
- Chawla, G., Gupta, P., Koradia, V., Bansal, A.K. (2003). Gastroretention a means to address regional variability in intestinal drug absorption. Pharma Technol July:50–68.
- Choudhury, P.K., Kar, M., Chauhan, C.S. (2008). Cellulose acetate microspheres as floating depot system to increase gastric retention of antidiabetic drug: formulation, characteristics and in vitro- in vivo evaluation. Drug Dev Ind Pharm. 34:349–54.
- Chun, M.K., Sah, H., Choi, H.K. (2005). Preparation of mucoadhesive microspheres containing antimicrobial agents for eradication of H. pylori. Int J Pharm 297:172–9.
- Daharwal, S.J., Koner, P., Saudagar, R.B. (2005). Gastro-retentive drugs: a novel approach towards floating therapy. Available online at: http://www.pharmainfo.net, accessed on 27 Sep, 2009.
- Dave, B.S., Amin, A.F., Patel, M.M. (2004). Gastroretentive drug delivery system of ranitidine hydrochloride: formulation and in vitro evaluation. AAPS PharmSciTech. 5: Article 34. Available online at: http://www.aapspharmscitech.org, accessed on 8 Sep, 2009.
- Davis, D.W. (1968). Method of swallowing a pill. US Patent, 3, 418, 999, December 31, 1968.
- Davis SS. (2005). Formulation strategies for absorption window. Drug Discov Today. 10:249–57.
- Dorozynski, P., Kulinowski, P., Jachowicz, R., Jasinski, A. (2007). Development of a system for simultaneous dissolution studies and magnetic resonance imaging of water transport in hydrodynamically balanced systems: a technical note. AAPS PharmSciTech 8:E1–E4.
- Durig, T., Fassihi, R. (2000). Evaluation of floating and sticking extended release deliverysystems: an unconventional dissolution test. J Contr Rel. 67:37–44.
- Gangadharappa, H.V., Kumar, P., Kumar, S. (2007). Gastric floating drug delivery systems: a review. Ind J Pharm Educ Res. 41:295–305.
- Garg, S., Sharma, S. (2003). Gastroretentive drug delivery systems. Pharma Technol. May:160–6. Available online at: http://www.touchbriefings.com, accessed on 9 Oct, 2009.
- Gibley, I.E. (2002). Development and in vitro evaluation of novel floating chitosan microcapsule for oral use: comparison with non-floating chitosan microspheres. Int J Pharm. 249:7–21.
- Gohel, M.C., Sarvaiya, K.G. (2007). A novel solid dosage form of rifampicin and isoniazid with inproved functionality. AAPS PharmSciTech 8: Article 68. Available online at: http://www.aapspharmscitech.org, accessed on 8 Sep, 2009.
- Goole, J., Gansbeke, B.V., Pilcer, G., Deleuze, P.H., Blocklet, D., Goldman, S., Pandolfo, M., Vanderbist, F., Amighi. K. (2008). Pharmacoscintigraphic and pharmacokinetic evaluation on healthy human volunteers of sustained-release floating minitablets containing levodopa and carbidopa. Int J Pharm 364:54–63.
- Groning, R., Berntgen, M., Georgarakis, M. (1998). Acyclovir serum concentration following peroral administration of magnetic depot tablets and the influence of extracorporal magnet to control gastroretentive transit. Eur J Pharm Biopharm 46:285–91.
- Groning, R., Cloer, C., Georgarakis, M., Muller, R.S. (2007). Compressed collagen sponges as gastroretentive dosage forms: in vitro and in vivo studies. Eur J Pharm Sci. 30:1–6.
- Hendee, W.R. (1994). In: Textbook of diagnostic imaging Volume 1, Fundamentals of diagnostic imaging:Characteristics of the radiographic image (C.E., Putman, C.E., Ravin, eds.), WB Saunders Co., Philadelphia, pp.9–10.
- Iannuccelli, V., Coppi, G., Leo, E., Fontana, F., Bernabei, M.T. (2000). PVP solid dispersion for the controlled release of furosemide from a floating multiple unit system. Drug Dev Ind Pharm. 26:595–603.
- Iannuccelli, V., Coppi, G., Sansone, R., Ferolla, G. (1998). Air compartment multiple-unit for prolonged gastric residence part II. In vivo evaluation. Int J Pharm 174:55–62.
- Ishak, R.A.H., Awad, G.A.S., Mortada, N.D., Nour, S.A.K. (2007). Preparation, in vitro and in vivo evaluation of stomach-specific metronidazole-loaded alginate beads as local anti- Helicobacter pylori therapy. J Contr Rel. 119:207–14.
- Jaimini, M., Rana, A.C., Tanwar, Y.S. (2007). Formulation and evaluation of famotidine floating tablets. Curr Drug Deliv. 4:51–5.
- Jain, S.K., Agrawal, G.P., Jain, N.K. (2006a). A novel calcium silicate based microspheres of repaglinide: in vivo investigations. J Contr Rel. 113:111–6.
- Jain, S.K., Agrawal, G.P., Jain, NK. (2006b). Evaluation of porous carrier-based floating orlistat microspheres for gastric delivery. AAPS PharmSciTech 7: Article 90. Available online at: http://www.aapspharmscitech.org, accessed on 11 Sep, 2009.
- Joseph, N.J., Lakshmi, S., Jayakrishnan, A. (2002). A floating type oral dosage form for piroxicam based on hollow polycarbonate microspheres: in-vitro and in-vivo evaluation in rabbits. J Cont Rel. 79:71–9.
- Julan, M., Desai, U., Parikh, J.R., Parikh, R.H. (2005). Floating drug delivery systems: an approach to gastroretention. 2005. Available online at: http://www.pharmainfo.net, accessed on 27 Sep, 2009.
- Kagan, L., Lapidot, N., Afargan, M., Kirmayer, D., Moor, E., Mardor, Y., Friedman, M., Hoffman, A. (2006). Gastroretentive accordion pill: enhancement of riboflavin bioavailability in humans. J Contr Rel. 113:208–15.
- Kale, R.D., Tyade, PT. (2007). A multiple unit floating drug delivery system of piroxicam using eudragit polymer. Indian J Pharm Sci. 69(1):120–3.
- Klausner, E.A., Eyal, S., Lavy, E., Friedman, M., Hoffman, A. (2003a). Novel levodopa gastroretentive dosage form: iv-vivo evaluation in dogs. J Contr Rel. 88:117–26.
- Klausner, E.A., Lavy, E., Barta, M., Cserepes, E., Friedman, M., Hoffman, A. (2003b). Novel gasrtroretentive dosage forms: evaluation of gastroretentivity and its effect on levodopa absorption in humans. Pharmacol Res. 20:1466–73.
- Klausner, E.A., Lavy, E., Friedman, M., Hoffman, A. (2003c). Expandable gastroretentive dosage forms. J Contr Rel. 90:143–62.
- Klausner, E.A., Lavy, E., Stepensky, D., Friedman, M., Hoffman, A. (2002). Novel gastroretentive dosage form evaluation of gastroretentivity and its effect on riboflavin absorption in dogs. Pharmacol Res. 19:1516–23.
- Krogel, I., Bodmeier, R. (1999). Development of a multifunctional matrix drug delivery system surrounded by an impermeable cylinder. J Contr Rel. 61:43–50.
- Kumar, P., Singh, S., Mishra, B. (2008). Floating osmotic drug delivery system of ranitidine hydrochloride: development and evaluation—a technical note. AAPS PharmSciTech. 9:480–5.
- Lee, J.H., Park, T.G., Choi, H.K. (1999). Development of oral drug delivery system using floating microspheres. J Microencapsul. 16:715–29.
- Lee, J.H., Park, T.G., Lee, Y.B., Shin, S.C., Choi, H.K. (2001). Effect of adding non-volatile oil as a core material for the floating microspheres prepared by emulsion solvent diffusion method. J Microencapsul 18:65–75.
- Mendyk, A., Jachowicz, R., Dorozynski, P. (2006). Artificial neural networks in the modeling of drugs release profiles from hydrodynamically balanced systems. Acta Pol Pharm. 63:75–80.
- Murata, Y., Sasaki, N., Miyamoto, E., Kawashima, S. (2000). Use of floating alginate gel beads for stomach-specific drug delivery. Eur J Pharm Biopharm. 50:221–6.
- Muthusamy, K., Govindarazan, G., Ravi, T.K. (2005). Preparation and evaluation of Lansoprazole floating micropellets. Indian J Pharm Sci. 67:75–9.
- Nakagawa, T., Kondo, S.I., Sasai, Y., Kuzuya, M. (2006). Preparation of floating drug delivery system by plasma technique. Chem Pharm Bull. 54:514–8.
- Nama, M., Gonugunta, C.S.R., Veerareddy, P.R. (2008). Formulation and evaluation of gastroretentive dosage forms of clarithromycin. AAPS PharmSciTech. 9:231–7. Available online at: http://www.aapspharmscitech.org., accessed on 08 Sep, 2009.
- Narendra, C., Srinath, M.S., Babu, G. (2006). Optimization of bilayer floating tablet containing metoprolol tartrate as a model drug for gastric retention. AAPS PharmSciTech. 7: Article-34. Available online at: http://www.aapspharmscitech.org, accessed on 08 Sep, 2009.
- Nepal, P.R., Chun, M.K., Choi, H.K. (2007). Preparation of floating microspheres for fish farming. Int J Pharm 341:85–90.
- Parikh, D.C., Amin, A.F. (2008). In vitro and in vivo techniques to assess the performance of gastro-retentive drug delivery systems: a review. Expert Opin Drug Deliv. 5:951–65.
- Park, H., Park, K., Kim, D. (2005). Preparation of swelling behavior of chitosan based superporous hydrogels for gastric retention application. 2005. Available online at: http://www.interscience.wiley.com, accessed on 19 Aug, 2009.
- Patel, D.M., Patel, N.M., Pandya N.N., Jogani, P.D. (2007a). Gastroretentive drug delivery system of carbamazepine: formulation optimization using simplex lattice design: a technical note. AAPS PharmSciTech. 8: Article 11. Available online at:http://www.aapspharmscitech.org, accessed on 11 Sep, 2009.
- Patel, D.M., Patel, N.M., Patel, V.F., Bhatt, D.A. (2007b). Floating granules of ranitidine hydrochloride-gelucire 43/01: formulation optimization using factorial design. AAPS PharmSciTech 8: Article 30. Available online at: http://www.aapspharmscitech.org., accessed on 11 Sep, 2009.
- Patel, G., Patel, H., Patel, M. (2005). Floating drug delivery system: an innovative approach to prolong gastric retention. 2005. Available online at: http://www.pharmainfo.net, accessed on 27 Sep, 2009.
- Patel, V.F., Patel, N.M. (2007). Statistical evaluation of influence of viscosity of polymer and types of filler on dipyridamole release from floating matrix tablets. Indian J Pharm Sci. 69(1):51–7.
- Pawar, A.P., Gadhe, A.R., Venkatachalam, P., Sher, P., Mahadik, K.R. (2008). Effect of core and surface cross-linking on the entrapment of metronidazole in pectin beads. Acta Pharm 58:75–85.
- Qureshi, M.J., Ali, J., Ahuja, A, Baboota, S. (2007). Formulation strategy for low absorption window antihypertensive agent. Indian J Pharm Sci. 69 (3)360–4.
- Rajinikanth, P.S., Mishra, B. (2008). Floating in situ gelling system for stomach site-specific delivery of clarithromycin to eradicate H. pylori. J Contr Rel. 125:33–41.
- Raval, J.A., Patela, J.K., Li, N., Patela, M.M. (2007). Ranitidine hydrochloride floating matrix tablets based on low density powder: effects of formulation and processing parameters on drug release. Asian J Pharm Sci. 2:130–42.
- Rocca, J.G., Omidian, H., Shah, K. (2003). Progresses in gastroretentive drug delivery systems. Pharma Technol. May:152–6. Available online at: http://www.touchbriefings.com, accessed on 16 Oct, 2009.
- Rouge, N., Allemann, E., Gex-Fabry, M., Balant, L., Cole, E.T., Buri, P., Doelker, E. (1998). Comparative pharmacokinetic study of a floating multiple-unit capsule, a high-density multiple-unit capsule and an immediate-release tablet containing 25 mg atenolol. Pharm Acta Helv. 73:81–7.
- Rouge, N., Leroux, J.C., Cole, E.T., Doelker, E., Buri, P. (1997). Prevention of the sticking tendency of floating minitablets filled into hard gelatin capsules. Eur J Pharm Biopharm 43:165–71.
- Sato, Y., Kawashima, Y., Takeuchi, H., Yamamoto, H. (2003). In-vivo evaluation of riboflavin-containing microballoons for a controlled drug delivery system in healthy human volunteers. J Contr Rel. 93:39–47.
- Sato, Y., Kawashima, Y., Takeuchi, H., Yamamoto, H. (2004). In- vitro and in-vivo evaluation of riboflavin-containing microballoons for a controlled drug delivery system in healthy humans. Int J Pharm. 275:97–107.
- Sawicki, W. (2002). Pharmacokinetics of verapamil and norverapamil from controlled release floating pellets in humans. Eur J Pharm Biopharm. 53:29–35.
- Sawicki, W., Lunio, R. (2005). Compressibility of floating pellets with verapamil hydrochloridecoated with dispersion Kollicoat SR 30D. Eur J Pharm Biopharm 60:153–8.
- Shimpi, S., Chauhan, B., Mahadik, R.K., Paradkar, A. (2004). Preparation and evaluation of diltiazem hydrochloride-glucire 43/01 floating granules prepared by melt granulation. AAPS PharmSciTech. 5: Article 43. Available online at: http://www.aapspharmscitech.org, accessed on 08 Sep, 2009.
- Shishu, Gupta, N., Aggarwal, N. (2007a). A gastro-retentive floating delivery system for 5-fl uorouracil. Asian J Pharm Sci. 2:143–9.
- Shishu, Gupta, N., Aggarwal, N. (2007b). Stomach-specific drug delivery of 5-fluorouracil using floating alginate beads. AAPS PharmSciTech 8: Article 48. Available online at: rom: http://www.aapspharmscitech.org, accessed on 11 Sep, 2009
- Simoni, P., Cerrb, C., Cipolla, A., Polimeni, C., Pistillo, A., Ceschel, G., Roda, E., Roda, A. (1995). Bioavailability study of a new, sinking, enteric-coated ursodeoxycholic acid formulation. Pharmacol Res. 31:115–9.
- Singh, B.M., Kim, K.H. (2000). Floating drug delivery system: an approach to oral controlled drug delivery via gastric retention. J Contr Rel. 63:235–59.
- Soppimath, K.S., Aminabhavi, T.M., Agnihotri, S.A., Mallikarjuna, N.N., Kulkarni, P.V. (2006). Effect of coexipients on nifedipine hollow microspheres: a novel gastroretentive drug delivery system. J Appl Polym Sci Symp. 100:486–94.
- Soppimath, K.S., Kulkarni, A.R., Aminabhavi, T.M. (2001a). Development of hollow microspheres as floating controlled-release system for cardiovascular drugs: preparation and release characteristics. Drug Dev Ind Pharm. 27:507–15.
- Soppimath, K.S., Kulkarni, A.R., Rudzinski, W.E., Aminabhavi, T.M. (2001b). Microspheres as floating drug-delivery systems to increase gastric retention of drugs. Drug Metab Rev. 33:149–60.
- Sonar, G.S., Jaina, D.K., Moreb, D.M. (2007). Preparation and in vitro evaluation of bilayer and floating-bioadhesive tablets of rosiglitazone maleate. Asian J Pharm Sci. 2:161–9.
- Sriamornsak, P., Thirawong, N., Puttipipatkhachorn, S. (2005). Emulsion gel beads of calcium pectinate capable of floating on the gastric fluid: effect of some additives, hardening agent or coating on release behavior of metronidazole. Eur J Pharm Sci. 24:363–73.
- Srivastava, A.K., Ridhurkar, D.N., Wadhwa, S. (2005). Floating microsphere of cimetidine: formulation, characterization and in-vivo evaluation. Acta Pharm. 55:277–85.
- Stithit, S., Chen, W., Price, J.C. (1998). Development and characterization of theophylline microspheres with near zero order release kinetics. J Microencapsul. 15:725–37
- Streubel, A., Siepmann, J., Bodmeier, R. (2002). Floating microparticles based on low density foam powder. Int J Pharm. 241:279–92.
- Streubel, A., Siepmann, J., Bodmeier, R. (2003). Floating matrix tablets based on low density foam powder: effects of formulation and processing parameters on drug release. Eur J Pharm Sci. 18:37–45.
- Streubel, A., Siepmann, J., Bodmeier, R. (2006). Drug delivery to the upper small intestine window using gastroretentive technologies. Curr Opin Pharmacol. 6:501–8.
- Strubing, S., Abboud, T., Contri, R.V., Metz, H., Mader, K. (2008a). New insights on poly(vinyl acetate)-based coated floating tablets: characterisation of hydration and CO2 generation by benchtop MRI and its relation to drug release and floating strength. Eur J Pharm Biopharm. 69:708–17.
- Strubing, S., Metz, H., Mader, K. (2008b). Characterization of poly(vinyl acetate) based floating matrix tablets. J Contr Rel. 126:149–55.
- Sungthongjeen, S., Sriamornsak, P., Puttipipatkhachorn, S. (2008). Design and evaluation of floating multi-layer coated tablets based on gas formation. Eur J Pharm Biopharm. 69:255–63.
- Talukder, R., Fassihi, R. (2004a). Gastroretentive drug delivery system: a mini review. Drug Dev Ind Pharm. 30:1019–28.
- Talukder, R., Fassihi, R. (2004b). Gastroretentive delivery systems: hollow beads. Drug Dev Ind Pharm. 30:405–12.
- Tanwar, Y.S., Chauhan, C.S., Naruka, P.S., Ojha, G. (2007a). Floating microspheres: development, characterization and applications. Available online at: http://www.pharmainfo.net, accessed on 27 Sep, 2009.
- Tanwar, Y.S., Naruka, P.S., Ojha, G.R. (2007b). Development and evaluation of floating microspheres of verapamil hydrochloride. [Updated 23 December 2007]. Available from: http://www.rbcf.usp.br.com, accessed 12 May 2008.
- Torrado, S., Prada, P., Paloma, M., Torre, D.L., Torrado, S. (2004). Chitosan-poly(acrylic) acid polyionic complex: in vivo study to demonstrate prolonged gastric retention. Biomaterials. 25:917–23.
- Umamaheshwari, R.B., Jain, S., Jain, N.K. (2003). A new approach in gasreoretentive drug delivery system using cholestyramine. Drug Deliv 10:151–60.
- Umamaheshwari, R.B., Jain, S., Tripathi, P.K., Agrawal, G.P., Jain, N.K. (2002). Floating bioadhesive microspheres containing acetohydroxamic acid for clearance of Helicobacter pylori. Drug Deliv. 9:233–1.
- Umamaheshwari, R.B., Ramteke, S., Jain, N.K. (2004). Anti-Helicobacter pylori effect of mucoadhesive nanoparticles bearing amoxicillin in experimental gerbils model. AAPS PharmSciTech, 5: Article-32. Available online at: http://www.aapspharmscitech.org, accessed on 11 Sep, 2009.
- Varshosaz, J., Tabbakhian, M., Zahrooni, M. (2007). Development and characterization of floating microballoons for oral delivery of cinnarizine by a factorial design. J Microencapsul. 24:253–62.
- Varshosaz, J., Tavakoli, N., Roozbahani, F. (2006). Formulation and in vitro characterization of ciprofloxacin floating and bioadhesive extended release tablets. Drug Deliv. 13:277–85.
- Wakode, R.R., Bajaj, AN. (2008). Formulation and characterization of pramipexole loaded microspheres. 2008 [Updated 2008 February]. Available online at: http://www.priory.com, accessed 7 May 2008.
- Wang, J., Tauchi, Y., Deguchi, Y., Morimoto, K. (2000). Positively charged gelatin microspheres as gastric mucoadhesive drug delivery system for eradication of H. pylori. Drug Deliv. 7:237–43.
- Waugh, A., Grant, A. (2001). Anatomy and physiology in health and illness. 9th ed. Elsevier Churchill Livingstone, Edinburgh; 2001.
- Whitehead, L., Fell, J.T., Collett, J.H., Sharma, H.L., Smith, A.M. (1998). Floating dosage forms: an in-vivo study demonstrating prolonged gastric retention. J Contr Rel 55:3–12.
- Wilding, I.R., Coupe, A.J., Davis, S.S. (2001). The role of γ-scintigraphy in oral drug delivery. Adv Drug Deliv Rev. 46:103–24.
- Yang, L., Eshraghi, J., Fassihi, R. (1999). A new intragastric delivery system for the treatment of Helicobacter pylori associated gastric ulcer: in vitro evaluation. J Contr Rel. 57:215–22.
- Yang, Z., Song, B., Li, Q., Fan, H., Ouyang, F. (2004). Preparation of microspheres with microballoons inside for floating drug delivery systems. J Appl Polym Sci Symp 94:197–202.
- Zheng, J., Liu, C., Bao, D., Zhao, Y., Ma, X. (2006). Preparation and evaluation of floating bioadhesive microparticles containing clarithromcin for the eradication of Helicobacter pylori. J Appl Polym Sci Symp 102:2226–32.
- Zou, H., Jiang, X., Kong, L., Gao, S. (2007). Design and gamma-scintigraphic evaluation of a floating and pulsatile drug delivery system based on an impermeable cylinder. Chem Pharm Bull. 55:580–5.
- Zou, H., Jing, X., Kong, L., Gao, S. (2008). Design and evaluation of a dry coated drug delivery system with floating-pulsatile release. J Pharm Sci 97:263–73.