Abstract
Limited penetration of neuroprotective drug citicoline into the central nervous system (CNS) by systemic administration led to poor efficiency. A novel method of stereotactic drug delivery was explored to make citicoline bypass the blood brain barrier (BBB) and take effect by direct contact with ischemic neurons. A permanent middle cerebral artery occlusion (pMCAO) model of rats was prepared. To get the optimal conditions for citicoline administration by the novel stereotactic delivery pathway, magnetic resonance imaging (MRI) tracer method was used, and a dose-dependent effect was given. Examinations of MRI, behavior evaluation, infarct volume assessment and histological staining were performed to evaluate the outcome. This MRI-guided stereotactic delivery of citicoline resulted in a notable reduction (>80%) in infarct size and a delayed ischemic injury in cortex 12 hours after onset of acute ischemia when compared with the systematic delivery. The improved neuroprotective efficiency was realized by a full distribution of citicoline in most of middle cerebral artery (MCA) territory and an adequate drug reaction in the involved areas of the brain. Brain lesions of treated rats by stereotactic delivery of citicoline were well predicted in the lateral ventricle and thalamus due to a limited drug deposition by MRI tracer method. Our study realized an improved neuroprotective efficiency of citicoline by stereotactic delivery, and an optimal therapeutic effect of this administration pathway can be achieved under MRI guidance.
1. Introduction
Citicoline (CDP-choline, cytidine 5′-diphosphocholine) has been widely considered as one of the most promising candidates for the treatment of acute ischemic stroke (CitationMinnerup & Schabitz, 2009). However, the extremely low brain uptake (only 0.5% of the oral dose and 2% of intravenous (IV) dose (CitationAdibhatla & Hatcher, 2002) is one of the obstacles affecting its neuroprotective efficiency. The strong polarity of citicoline is apparently the leading cause of its poor penetration into the brain across the blood brain barrier (BBB), though it has a low molecular weight (MW) (CitationFresta, Puglisi, Di Giacomo, & Russo, 1994). The limited volume fraction of brain microvessels (merely 3% of the total brain volume) (CitationSykova & Nicholson, 2008), and the postischemic hypoperfusion state before a successful vascular recanalization make citicoline in blood even more difficult to reach the infarct brain tissues. Practically, not more than 10% of patients will benefit from a vascular recanalization therapy (CitationDel, 1995).
Several strategies have been explored, such as dose promotion (CitationHurtado et al., 2005), hyperosmotic BBB disruption (CitationKrauze, Forsayeth, Park, & Bankiewicz, 2006), or vector-mediated delivery (using liposome to enhance BBB permeability (CitationSureshVenkateswarlu & Koning, 2006)) to increase the brain uptake. Nevertheless, the risk of systemic toxicity associated with overdose (CitationAdibhatla & Hatcher, 2002) and side effects by introducing exogenous substances into the brain is greatly increased. A novel method of stereotactic drug delivery (local intracranial (IC) injection) has become an accepted method for treatment of central nervous system (CNS) diseases, for example, the convection enhanced delivery (CED) in the treatment of malignant glioma (CitationDebinski & Tatter, 2009). As an endogenous substance in normal brain, citicoline is safer than other drugs for this delivery. To our best knowledge, no similar study has been reported in the field of cerebral ischemia.
However, there is no reference for the administration by the pathway. An optimal delivery occurred in as few as 20% of subjects due to the subject-specific anatomy of target tissues, which played a major role in drug distribution and metabolism (CitationSampson et al., 2007). To get the optimal conditions for citicoline delivery, magnetic resonance imaging (MRI) tracer method was used in pharmacokinetic modeling. Gadolinium-diethylenetriaminepentaacetic acid (Gd-DTPA), which has several similarities in size and nature with citicoline, is an appropriate tracer for pharmacokinetic modeling of citicoline; both of them are micromolecular (938.02 Da vs. 510.31 Da), ionic, and hyperosmotic (CitationCaravan, Ellison, McMurry, & Lauffer, 1999). We hypothezed that stereotactic delivery of citicoline without the hindrance by the BBB would exert neuroprotective functions more than systemic delivery.
2. Methods
2.1. Animals.
The study was conducted in accordance with national guidelines for the use of experimental animals, and the protocols were approved by the Ethics Co mmittee of Peking University Health Science Center (Approved No. LA2009-008). We used age-matched, mature male Sprague Dawley (SD) rats weighing 250–300 g for all animal experiments.
2.2. Experimental groups.
Citicoline (Qilu Pharmaceutical Co., Ltd, Shandong, China) was administered by IC microinjection. The pharmacokinetic parameters of original injection site, administration time and dose were determined according to MRI tracer method. 30 SD rats were randomly divided into 4 groups with different surgical operations: (1) sham-operated animals (Sham; n = 6); (2) permanent middle cerebral artery occlusion (pMCAO) 2 h after IC microinjection of saline (Vehicle group; n = 8); (3) pMCAO 1 h after intraperitoneal (IP) injection of 2 g/kg citicoline (CitationHurtado et al., 2005)(IP group; n = 8); (4) pMCAO 2 h after IC microinjection of 0.0005 g/kg citicoline (IC group; n = 8).
2.3. MRI tracer method.
2 μL 10 mmol/L Gd-DTPA (Magnevist, Bayer Schering Pharma AG, Berlin, Germany) was administered by IC microinjection into the caudate putamen (bregma: +1 mm, lateral: 3 mm, vertical: 4.5 mm), the external capsule (bregma: +1 mm, lateral: 3 mm, vertical: 2.5 mm) and the thalamus (bregma: -1 mm, lateral: 3 mm, vertical: 6 mm) respectively, and 2 μL 200 mmol/L Gd-DTPA was administered by intracerebral ventricle (ICV) microinjection into the contralateral ventricle (bregma: -1 mm, lateral: 1.5 mm, vertical: 4 mm) using a stereotactic coordinate (Lab Standard Stereotaxic-Single, Stoelting Co, Illinois, USA). 3D T1 weighted (T1W) imaging was performed at different time points of 0 h, 1 h, 2 h, 3 h, 4 h and 6 h after injection. The signal intensity (SI) change (ΔSI=SIpostcontrast-SIprecontrast) of the target brain region was calculated with Siemens workstation (NUMARIS/4, Siemens Medical Solutions, Erlangen, Germany). Extra rats were required for quantitative determination of Gd-DTPA concentration by the method described in our previous study (CitationXu et al., 2011). The target brain tissue was dissected according to the MR image, and the absolute concentration of Gd-DTPA was measured by inductively coupled plasma mass spectrometry (ICP-MS). The ΔSI versus concentration (ΔSI-C) curve was fitted using linear regression analysis of SPSS 13.0, and the quantitative equation was obtained.
2.4. pMCAO model preparation and outcome evaluation.
A permanent focal cerebral ischemia model was performed to simulate the real state of patients before a successful vascular recanalization therapy. SD Rats were anaesthetized with chloral hydrate by IP administration (400mg/kg). Permanent focal cerebral ischemia was induced by modified Longa’s method (CitationLonga, Weinstein, Carlson, & Cummins, 1989). Neurological function was evaluated 12 h after surgery before MRI according to Longa 5-grade standard (CitationLonga et al., 1989).
MRI was performed dynamically at 3 h, 6 h, 9 h and 12 h after stroke onset to monitor the progress of infarction in a 3.0T MR system (Magnetom Trio, Siemens Medical Solutions, Erlangen, Germany). Typical manifestations of infarction were decreased signal intensity on T1W images and increased signal intensity on T2 weighted (T2W) images (CitationMoseley et al., 1990). A wrist coil was used, parameters for the imaging sequence were as follows: (1) 3D MP-RAGE T1WI (echo time [TE] = 3.7ms; repetition time [TR] = 1500ms; flip angle α = 9°; inversion time [TI] = 900ms; field of view (FOV) = 267 mm; voxel = 0.5 × 0.5 × 0.5 mm); (2) TSE-T2WI (TE = 92ms; TR = 3620ms; flip angle α = 120°; slice thickness: 2 mm; FOV = 80 mm).
Brains of all subjects were removed after MRI. Series of 2 mm of coronal brain slices were obtained (Brain Matrix, EHSY, Shanghai, China) and stained at 37°C in 0.2% TTC (2,3,5-triphenyl-tetrazolium chloride, Sigma, Missouri, USA) for 30min. The infarct volume was analyzed by an image analyzer (Image Pro Plus 6.0, Media Cybernetics, Inc., Georgia, USA). The infarct area, which was not stained, was determined by counting pixels contained within the outlined regions of interest. Infarct volume was expressed in the ratio of pixels counted from corrected infarct area to that from the whole contralateral hemisphere (CitationJoshiJain & Murthy, 2004).
Histological assessment was performed to evaluate the severity of ischemic injury at the cellular level. Treated rats were anesthetized and perfused with 4% paraformaldehyde. The fixed brains were cut into sections. After comparing with the brain slices in TTC staining, the corresponding brain sections were stained by Hematoxylin and Eosin (H&E).
2.5. Statistical analysis.
Results are presented as mean±standard deviation (S.D). Statistical analysis was performed by SPSS 13.0. One-way analysis of variance (ANOVA) followed by individual comparisons of means (LSD test, or Dunnet’s method when the data were not normally distributed) was used for the comparison of grouped data. P<0.05 indicated statistical significance.
3. Results
3.1. Determination of the appropriate injection site for stereotactic delivery
Caudate putamen was shown to be the appropriate injection site for stereotactic delivery as compared to the contralateral ventricle, the external capsule and the thalamus (). Gd-DTPA diffused to most of MCA territory, including the frontotemporal cortex and the posterior caudate nucleus over a period of 6 hours (). After injection into the contralateral ventricle and the external capsule, Gd-DTPA was nevertheless restricted to a small region ( and ). The limited deposition of Gd-DTPA 1 hour after injection into the thalamus indicated a different diffusion pattern with a fast clearance rate ().
Figure 1. Diffusion pattern of Gd-DTPA after injection into different brain regions. (A) Distribution of Gd-DTPA after IC injection into the caudate putamen over a period of 6 hours. The distribution area was outlined, and symbols of ‘*’, ‘#’ and ‘†’ presented the posterior caudate nucleus, the frontotemporal cortex and the anterior thalamus individually. (B) Distribution of Gd-DTPA 2 hours after ICV injection. To avoid the influence on the distribution by the reflux from the needle track to the cortex, Gd-DTPA was injected into the contralateral ventricle. Gd-DTPA could hardly diffuse to the parenchyma from the contralateral ventricle. Most of the MCA territory (‘*’ and ‘#’) except for the thalamus (‘†’) was excluded from the distribution area of Gd-DTPA. The subarachnoid space (‘→’) was significantly enhanced by Gd-DTPA. (C) Gd-DTPA diffused in a manner of anisotropy and spread along the white fiber after IC injection into the external capsule. (D) Limited deposition of Gd-DTPA was found 1 hour after IC injection into the thalamus.
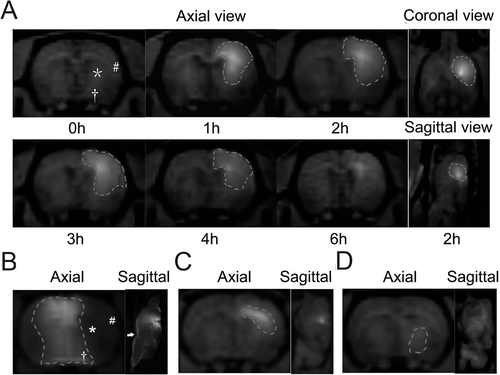
3.2. Determination of the administration time for stereotactic delivery
The distribution area of Gd-DTPA expanded gradually during the first 3-hour period after IC injection into the center of caudate putamen (). The great attenuation of MR signal intensity showed a significant clearance of Gd-DTPA in 4 hours (). The best administration time was presumed to be 2 hours before pMCAO procedure, and an adequate drug reaction was allowed to occur for the prevention of ischemic injury.
3.3. Determination of the injection dose for stereotactic delivery
After a conversion of MR signal enhancement induced by Gd-DTPA into its absolute quantity (ΔSI = 2089.0C+32.4, R2 = 0.94; ), time-dependent concentration curve of Gd-DTPA in MCA territory was obtained ( and ). Patterns of change in concentration varied among regions. In the posterior caudate nucleus, the concentration reached the peak in less than 1 hour, and then decreased. In the frontotemporal cortex, the concentration did not climb to the top until 4 hours after injection. Concentrations in both regions were maintained stably at 0.02 mmol/L, which was 1/500 of the initial concentration (10 mmol/L) for 2 hours. Considering the effective concentration of citicoline in experiment in vitro was 0.1 mmol/L (CitationHurtado et al., 2008), the injection dose of citicoline was therefore presumed to be 50 mmol/L according to the result of MRI tracer method. No benefit from either dose reduction (25 mmol/L) or increase (100 and 250 mmol/L) was revealed in animal experiments ( and ).
Figure 2. Pharmacokinetic modeling of citicoline by MRI tracer method. (A) The quantitative linear curve of MRI signal enhancement (ΔSI) versus Gd-DTPA concentration (C) in rat brain tissues over the range of 0~0.1mmol/L. (B) Sketch map of the potential infarct regions after pMCAO procedure. (C) Distribution of Gd-DTPA concentrations in different brain regions over a period of 6 hours. (D) TTC staining of ischemic lesions in groups treated with different dose of citicoline. (E) Comparison of the infarct volume ratio among groups treated with different dose of citicoline. Data were mean±SD, n=5~8. *P < 0.05, **P < 0.01.
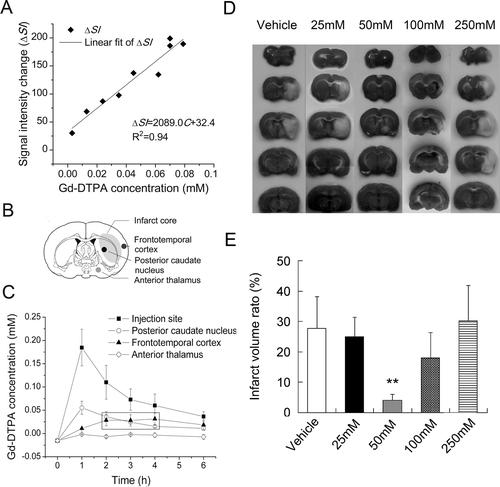
3.4. Effect of citicoline by stereotactic delivery on infarct size
A pMCAO produced severe lesions covering both the cortex and the subcortical regions (AstrupSiesjo & Symon, 1981) in vehicle group (). Stereotactic delivery of citicoline resulted smaller lesions only in subcortical regions (). Although 2 rats in IP group suffered minor injuries, the rest did not receive an effective protection by systemic delivery ().
Figure 3. Stereotactic delivery of citicoline reduced the infarct volume ratio and the neurological deficit. (A) TTC staining and MRI of ischemic lesions in groups treated with different drug delivery. Infarct tissues were not stained by TTC. Typical manifestations of infarct tissues were decreased signal intensity on T1W images and increased signal intensity on T2W images. The characteristics lesions were observed to be involving both the cortex (arrow with a round head) and the subcortical regions (arrows with a square head and a triangular head). (B) Comparison of the infarct volume ratio among groups. Data were mean±SD, n=8. *P < 0.05, **P < 0.01. (C) Comparison of the neurological deficit score among groups. Data were mean±SD, n=8. *P < 0.05, **P < 0.01. (D) H&E staining of neurons. Normal neurons (‘→’) with an intact mesenchyme (‘*’) was shown in sham group. In vehicle group and IP group, severe eosinophilic degeneration (‘→’) was observed in most neurons with a collapsed mesenchyme (‘*’). Lymphocytes were found around necrotic cells (‘▸’). In IC group, a milder injury occurred in the cortex and the caudate nucleus except for the thalamus.
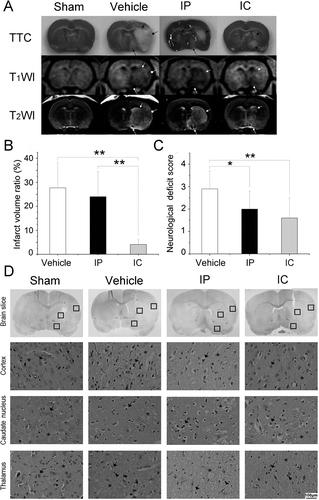
The infarct volume ratio showed a statistical significance among groups (n = 8, F = 17.381, P = 0.000, P<0.01). A notable reduction of 85.2% (4.1 ± 2.0 vs. 27.7 ± 10.5) and 82.9% (4.1 ± 2.0 vs. 24.0 ± 10.4) was demonstrated in IC group as compared respectively to vehicle group and IP group (n = 8, P = 0.000, P<0.01, ). No statistical difference was shown between vehicle group and IP group (27.7 ± 10.5 vs. 24.0 ± 10.4), though there was a trend toward reduced infarct size (n = 8, P = 0.397, P>0.05, ).
3.5. Effect of citicoline by stereotactic delivery on neurological function
The neurological deficit scoring showed a statistical significance among groups (n = 8, F = 6.320, P = 0.007, P<0.01). An improved neurological function was observed in IP group and IC group as compared respectively to vehicle group (2.0 ± 0.8 vs. 2.9 ± 0.8, n = 8, P = 0.034, P<0.05; 1.6 ± 0.9 vs. 2.9 ± 0.8, n = 8, P = 0.002, P<0.01, ), whereas no statistical difference was found between IP group and IC group (2.0 ± 0.8 vs. 1.6 ± 0.9, n = 8, P = 0.215, P>0.05, ).
3.6. Effect of citicoline by stereotactic delivery on neurons at the cellular level
H&E staining revealed the morphological changes of neurons in each subregion of MCA territory (). Normal neurons had little eosinophilic degeneration, and the mesenchyme remained intact as shown in sham group. However, severe eosinophilic degeneration of necrotic neurons and collapse of mesenchyme was occurred throughout the MCA territory in vehicle group. Normal neurons with an intact mesenchyme were observed in the frontotemporal cortex and the posterior caudate nucleus in IC group. A milder injury of neurons in the frontotemporal cortex was found in IP group as compared to vehicle group. Since behavioral deficits have a good correlation with the severity of damage in the cortex (CitationLonga et al., 1989), the improved neurological function in IC and IP group can be attributed to the effective protection by citicoline.
3.7. Potentially protected regions predicted by MRI tracer method
The non-deposition areas of Gd-DTPA matched exactly with the poorly protected regions in pMCAO experiment by stereotactic delivery of citicoline. The potentially protected regions can be outlined with areas with a full distribution of Gd-DTPA by using MRI tracer method ().
Figure 4. Potentially protected regions predicted by MRI tracer method. (A) Axial image of Gd-DTPA enhanced T1WI provided the anatomical information of brain tissues neighboring the ventricle (arrow with a triangular head), the thalamus (arrow with a square head) and the skull (arrow with a round head). (B) Pseudo-color image of MR signal enhancement 2 hours after IC injection of Gd-DTPA. (C) Characteristic ischemic lesions involved both the cortex and subcortical regions. (D) Non-deposition areas of Gd-DTPA matched exactly with the poorly protected regions in pMCAO experiment by stereotactic delivery of citicoline.
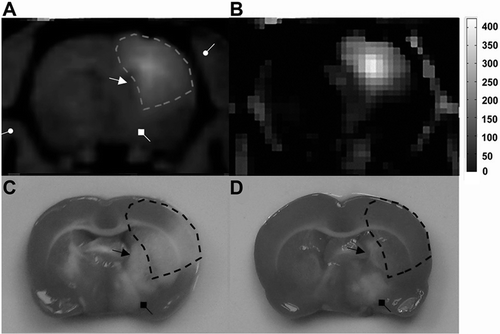
4. Discussion
In the present study, we administered citicoline before stroke in an experimental model to prevent ischemic damage by stereotactic delivery, which significantly promoted the neuroprotective efficiency of microdose citicoline without the hindrance of BBB. Although prior administration of citicoline seems cannot be applied to patients after the fact, it might be a potentially preventable strategy for coronary artery bypass surgery. Also, the MRI-guided stereotactic delivery was firstly proposed as a novel approach to use MRI tracer to simulate drug distribution and is hopeful to develop a brain atlas for stroke therapy in the near future. Briefly, our present work has great significance in characterizing the basic parameters and hence to laid the ground work for following experiments that are more clinical-relevant.
Brain extracellular space (ECS), which has been recognized as the exchange channel between brain cells and microvessels for essential substances since 1960s (CitationSykova & Nicholson, 2008), provides drugs with sufficient spaces for stereotactic delivery due to its higher volume fraction about 20% than microvessels. With our deep knowledge of the architecture of brain ECS (CitationSykova & Nicholson, 2008), distribution pattern of drugs in brain ECS is known to be strongly associated with the specific anatomy of target tissues. Although the pathway by ICV injection was co mmon used in CNS delivery, the caudate putamen was demonstrated to be the most appropriate injection site for infusing a drug into MCA territory, which suffered the ischemic damage greatly (CitationSzentistvanyi, Patlak, Ellis, & Cserr, 1984). The initial dose can also be reduced dramatically without the dilution of cerebral spinal fluid (CSF). Compared to other subregions of MCA territory, such as the external capsule and the thalamus, the caudate putamen allowed the drug to diffuse more extensively and stay longer until an adequate drug reaction had taken place.
The neuroprotective efficiency of citicoline is determined by the local concentration in target tissues besides the severity of injury. The effective concentration of citicoline in experiment in vitro is considered to be around 0.1 mmol/L (CitationHurtado et al., 2008), which was exactly the local concentration in most regions rendered ischemia in our study. According to the diffusion formula, drug concentration in brain ECS depends on both the diffusion and the clearance. In caudate putamen only 10%∼15% of efflux has been reported to drainage into the bulk CSF, and nearly 90% of tracers were remained in the brain in 2 hours (CitationSzentistvanyi et al., 1984), thus a significant neuroprotection occured. However, in thalamus, a limited drug deposition, which might be due to a faster clearance rate because of the absence of an intact BBB (CitationNorstedGomuc & Meister, 2008) led to poor protection.
Interestingly, citicoline appears to loose its efficiency at higher concentrations. Exogenous citicoline is known to be hydrolyzed as cytidine and choline after administration into the brain, and can be resynthesized from cytidine triphosphate (CTP) and phosphocholine by cytidylyltransferase (CitationAdibhatla & Hatcher, 2005). The hydrolysis product of citicoline partly serves as a choline donor in the biosynthesis of the neurotransmitter acetylcholine (ACh) (CitationAdibhatlaHatcher & Dempsey, 2002). During the early period of ischemia, a transient increase of acetylcholinesterase (AChE) rapidly degrades most of the endogenous ACh (CitationSaez-ValeroGonzalez-Garcia & Cena, 2003). This depletion of ACh triggers hydrolysis of citicoline, which in turn drives the reaction from choline to ACh (CitationAdibhatla et al., 2002). ACh is essential for neurotransmission and helps the rescue of cognitive deficits (Woolf, 2006). In the case of high dose of citicoline, the process described above could still produce beneficial effects at the first. However, after a prolonged time period, the activity of AChE is significantly reduced by the aggravated injury (CitationSaez-Valero et al., 2003). As a result, ACh accumulated in the interstitial fluid of the brain (Phillis, Smith-Barbour, Perkins, & O’Regan, 1993). High level of ACh is regarded to potentiate L-glutamate-induced excitotoxic neuron death (Mattson, 1989; Phillis et al., 1993). Therefore, at this time point citicoline appears to be toxic at higher concentrations. Beside of excitotoxic neuron death, the excessive release of ACh will lead to persistent depolarization at the neuromuscular junction, and induce several clinical features including muscle fasciculation, cramps, twitching and weakness (CitationKristian & Siesjo, 1998) (Supplementary Video 1). A more precise analysis of citicoline efficiency will be conducted over a narrower range of dose around 50 mmol/L in our future study.
5. Conclusions
Our study realized an improved neuroprotective efficiency of citicoline by stereotactic delivery in prevention of ischemic damage, and an optimal therapeutic effect of this administration pathway can be achieved under MRI guidance. The encouraging results provide possibilities for performing stereotactic delivery of neuroprotective drugs after stroke onset in therapy. Our findings can also be utilized to develop a software algorithm that generates an atlas of drug distribution in the ischemic brain to give a computational reference for performing stereotactic delivery of drugs in practice.
Supplementary Material
Download QuickTime Video (857.2 KB)Supplementary Material
Download PDF (374.2 KB)Acknowledgements
We thank Zuoli Xia and Yu Fu for suggestions on experiment design of neuroprotection. We also thank Project of New Century Excellent Talents in University of China (Grant number NCET-05-0019), National Natural Science Foundation (Grant number 30972811) and Beijing Municipal Natural Science Foundation (Grant number 7093137) for supporting this work.
Declaration of interest
We declare that we have no financial and personal relationships with other people or organizations that can inappropriately influence our work; there is no professional or other personal interest of any nature or kind in any product, service and/or company that could be construed as influencing the position presented in, or the review of the paper.
References
- Adibhatla, R. M., Hatcher, J. F., & Dempsey R. J. (2002). Citicoline: neuroprotective mechanisms in cerebral ischemia. J Neurochem, 80(1), 12–23.
- Adibhatla, R. M., & Hatcher J. F. (2002). Citicoline mechanisms and clinical efficacy in cerebral ischemia. J Neurosci Res, 70(2), 133–139.
- Adibhatla, R. M., & Hatcher J. F. (2005). Cytidine 5′-diphosphocholine (CDP-choline) in stroke and other CNS disorders. Neurochem Res, 30(1), 15–23.
- Astrup, J., Siesjo, B. K., & Symon L. (1981). Thresholds in cerebral ischemia - the ischemic penumbra. Stroke, 12(6), 723–725.
- Caravan, P., Ellison, J. J., McMurry, T. J., & Lauffer R. B. (1999). Gadolinium(III) Chelates as MRI Contrast Agents: Structure, Dynamics, and Applications. Chem Rev, 99(9), 2293–2352.
- Debinski, W., & Tatter S. B. (2009). Convection-enhanced delivery for the treatment of brain tumors. Expert Rev Neurother, 9(10), 1519–1527.
- Del, Z. G. (1995). Acute stroke–on the threshold of a therapy? N Engl J Med, 333(24), 1632–1633.
- Fresta, M., Puglisi, G., Di Giacomo, C., & Russo A. (1994). Liposomes as in-vivo carriers for citicoline: effects on rat cerebral post-ischaemic reperfusion. J Pharm Pharmacol, 46(12), 974–981.
- Hurtado, O., Moro, M. A., Cardenas, A., Sanchez, V., Fernandez-Tome, P., & Leza J. C., et al. (2005). Neuroprotection afforded by prior citicoline administration in experimental brain ischemia: effects on glutamate transport. Neurobiol Dis, 18(2), 336–345.
- Hurtado, O., Pradillo, J. M., Fernandez-Lopez, D., Morales, J. R., Sobrino, T., & Castillo J., et al. (2008). Delayed post-ischemic administration of CDP-choline increases EAAT2 association to lipid rafts and affords neuroprotection in experimental stroke. Neurobiol Dis, 29(1), 123–131.
- Joshi, C. N., Jain, S. K., & Murthy P. S. (2004). An optimized triphenyltetrazolium chloride method for identification of cerebral infarcts. Brain Res Brain Res Protoc, 13(1), 11–17.
- Krauze, M. T., Forsayeth, J., Park, J. W., & Bankiewicz K. S. (2006). Real-time imaging and quantification of brain delivery of liposomes. Pharm Res, 23(11), 2493–2504.
- Kristian, T., & Siesjo B. K. (1998). Calcium in ischemic cell death. Stroke, 29(3), 705–718.
- Longa, E. Z., Weinstein, P. R., Carlson, S., & Cummins R. (1989). Reversible middle cerebral artery occlusion without craniectomy in rats. Stroke, 20(1), 84–91.
- Minnerup, J., & Schabitz W. R. (2009). Multifunctional actions of approved and candidate stroke drugs. Neurotherapeutics, 6(1), 43–52.
- Moseley, M. E., Cohen, Y., Mintorovitch, J., Chileuitt, L., Shimizu, H., & Kucharczyk J., et al. (1990). Early detection of regional cerebral ischemia in cats: comparison of diffusion- and T2-weighted MRI and spectroscopy. Magn Reson Med, 14(2), 330–346.
- Norsted, E., Gomuc, B., & Meister B. (2008). Protein components of the blood-brain barrier (BBB) in the mediobasal hypothalamus. J Chem Neuroanat, 36(2), 107–121.
- Saez-Valero, J., Gonzalez-Garcia, C., & Cena V. (2003). Acetylcholinesterase activity and molecular isoform distribution are altered after focal cerebral ischemia. Brain Res Mol Brain Res, 117(2), 240–244.
- Sampson, J. H., Raghavan, R., Brady, M. L., Provenzale, J. M., Herndon, J. N., & Croteau D., et al. (2007). Clinical utility of a patient-specific algorithm for simulating intracerebral drug infusions. Neuro Oncol, 9(3), 343–353.
- Suresh, R. J., Venkateswarlu, V., & Koning G. A. (2006). Radioprotective effect of transferrin targeted citicoline liposomes. J Drug Target, 14(1), 13–19.
- Sykova, E., & Nicholson C. (2008). Diffusion in brain extracellular space. Physiol Rev, 88(4), 1277–1340.
- Szentistvanyi, I., Patlak, C. S., Ellis, R. A., & Cserr H. F. (1984). Drainage of interstitial fluid from different regions of rat brain. Am J Physiol, 246(6 Pt 2), F835–F844.
- Xu, F., Han H, Zhang, H., Pi, J., & Fu, Y. (2011). Quantification of Gd-DTPA concentration in neuroimaging using T1 3D MP-RAGE sequence at 3.0T. Magn Reson Imag (In Press), DOI:2011. 10.1016/j.mri.2011.02.019.