Abstract
Ethinylestradiol liposome (EEL) was prepared by thin film evaporation–extrusion technique and its effects on ovariectomized rat osteoporosis were investigated. The liposomes were characterized with clear homogeneous lamellar vesicles and had the average size of 200 ± 1.5 nm. The long term stability of liposome formulations were tested over a 28-day period at 4°C and ethinylestradiol retention was approximately 80% up to 28 day. Release data of ethinylestradiol was tested in PBS pH 7.4 at 37°C using a dialysis method and its release profiles were biphasic, showing a relatively large burst effect over the first four hours, followed by a slower release phase. Ovariectomized rat model was used to investigate EEL effects on osteoporosis. After three months post-surgery, the rats were divided into two groups and injected intraperitoneally (IP) with 2 μg per kilogram per day of either free ethinylestradiol (EE) or encapsulated ethinlyestradiol (EEL). At termination of one month IP injection, the rats were killed and the bone mineral density (BMD) and alkaline phosphatase (ALP) were measured. BMD value in free EE group increased by 20.3 %, while it increased by 37.8 % in EEL group. According to ALP value, the two treatment groups increased by 28.6 % and 42.0 %, respectively. These data indicated that ethinlyetradiol liposome had better effect than that of free ethinylestradiol did in treatment of the ovariectomized rats’ osteoporosis. But the metabolites and biodistribution of ethinylestradiol liposome in vivo need to investigate in the further research work.
Introduction
Postmenopausal osteoporosis is a systemic skeletal disease characterized by low bone mass micro-architectual deterioration of bone tissue after the women menopause, which is a chronic, progressive condition and results in increasing bone fragility and the increasing the susceptibility to fracture [Bonewald and Johnson Citation2008, Timmer, et al. Citation2009]. Menopause is the most important risk factor for bone loss in women in late adulthood [Shargorodsky et al. Citation2009]. Women lose approximately 2% of cortical bone and 5% of trabecular bone per year for the first 5-8 years after the onset of menopause [Demir et al. Citation2008]. The studies show that the postmenopausal osteporosis is caused by the hypofunction of ovary and the diminishing of the estrogen secretion, which results in the disequilibrium of bone couple process [Setchell et al. Citation2005]. In the normal adults, the activity of osteoclasts (bone resorption) is balanced by that of osteoblasts (bone formation). While with the onset of menopause, diminishing estrogen levels lead to excessive bone resorption that is not fully compensated by an increase in bone formation [Gobbi et al. Citation2007, Kita et al. Citation2004].
The supplementation of Calcium and vitamin D is also useful in treating elderly women with osteoporosis for a high prevalence of vitamin D inadequacy among postmenopausal women [Pasco et al. Citation2009, Riera-Espinoza et al. Citation2009, Poiana et al. Citation2009]. Results of large placebo controlled trials had shown that alendronate, raloxifene, risedronate, parathyroid hormone, and calcitonin greatly could reduce the risk of postmenopausal osteoporosis [Schindeler et al. Citation2007, Hagino et al. Citation2009, Rizzoli et al. Citation2009, Black et al. Citation2009, Widler et al. Citation2002, Makras et al. Citation2009]. In the 1930s, estrogen replacement therapy (ERT) was introduced and increased the estrogen level through introducing the exogenous estrogen [Tyler et al. Citation2009, Lindenfeld and Langer Citation2002], which remained as a valuable method to prevent further bone loss in postmenopausal women [Raudaskoski et al. Citation2002, Cuzick Citation2008]. In addition, as a treat method to osteoporosis, ERT was convenient, cheap, precise therapeutic effect, and acceptable to most post-menopausal women. But recent studies [Cederroth and Nef Citation2009, Zhou et al. Citation2009, Mamot et al. Citation2003] showed that the risks could far outweigh the benefits of long-term estrogen therapy in women, which would have the side effects, such as water retention, bloating, nausea, breast soreness, mood swings, irregular bleeding, and headaches. The long-term ERT use might also increase the chance of endometrial and breast cancer and cardiovascular problems, increases the chance of memory loss and brain damage in women over age sixty-five (Peng et al. Citation2009, 2010). In 2003 the FDA joined forces with several congressmen and women and launched a national campaign focusing on the risks of ERT.
Liposomes are self-assembled vesicles consisting of a flexible bilayer, which encapsulates a region of aqueous solution inside a hydrophobic membrane [Barenholz Citation2001]. The aqueous interior can be used as a container for storage, transport, and for the controllable release of hydrophilic compounds, while the hydrophobic compounds will be contained in the bilayer membrane. Several research groups have reported on using liposome as a carrier for drugs to against osteoporosis [Quadir et al. Citation2008, Renoir et al. Citation2006, Hengst et al. Citation2007, Schmidt et al. Citation2005]. Ethinylestradiol, one of the strongest biological activities estrogens, is hydrophobic and could be encapsulated between the bilayer. In this paper, we prepared ethinylestradiol liposome formulations and investigated its action against postmenpausal osteoporosis using the ovariectomized rat model [Otsuka et al. Citation2008, Suzuki et al. Citation2002].
Materials and methods
Materials
Ethinylestradiol▪(EE) and 3-hydroxyl-19-norpregna-1,3,5(10)-trien-20-yne-17-diol were supplied by Kefeng Biotechnological Company (Beijing, China). Egg phosphatidylcholine (EPC) with the purity of 98% was supplied by Xi’an Libang Pharmaceutical Technology Company (Xi’an, China). Cholesterol (Chol) was obtained from Beijing Aoboxing Biotechnology Co. The solvents (analytical grade) used in preparation of liposome, such as methanol and chloroform, were supported by Xi’an Chemical Agent Company (Xi’an, China). Sodium dihydrogen phosphate (NaH2PO4·H2O), disodium hydrogen phosphate dodecahydrate (Na2HPO4·12H2O) and sodium hydroxide (NaOH) were used for prepared phosphate buffer solution (PBS) and also supported by Xi’an Chemical Agent Company (Xi’an, China). Acetonitrile and methanol used were of HPLC grade and purchased from Sigma Corp. Female SPF grade SD rat, weighing 280 ± 2 g, age three months, were supplied from the Experimental Animal Centre of the Fourth Military Medical University (Xi’an, China).
Prepare the Ethinylestradiol Liposome
Ethinylestradiol liposome was prepared by thin film evaporation–extrusion technique, based on a previously published method described by Garg [Garg et al. Citation2006]. The lipid composition was EE/EPC at a molar ratio of 5:95, 10:90, 15:85, 20:80, 25:75, and 30:70, respectively. The lipid composition (10.0 g) was dissolved in chloroform/methanol (1:2, v:v) and mixed for 5 min using a vortex agitator (XW-80A, Ningbo, China). After that, the mixture was allowed to dry under nitrogen protection on a rotary evaporator (N-1001, EYELA, Japan) at 55°C until the organic solvents evaporated thoroughly. The dried, lipid film was hydrated with 50 mL of PBS (pH 7.4) aqueous solution by homogenizing at room temperature for 30 min using ultrasound meter at 20 KHz. The hydrated solution was sheared with high speed snipper (FJ 200-S, Shanghai, China) at speed of 30 000 g for about 2 hours. Then the liposome suspension was extruded for four extrusion cycles with the extruder (Lipex, Northern Lipids Inc., Canada) through the polycarbonate filter membrane (Lipex, Northern Lipids Inc., Canada) having the pore size of 400 and 200 nm, respectively. Untrapped free ethinylestradiol was removed in a 1.1 × 20 cm Sepharose CL-6B column (Sigma Chemical Co., St. Louis, MO, USA) equilibrated with PBS buffer. Later, the liposome suspension was stored in nitrogen-purged vials and left in freeze at 4°C. For control, the blank liposome containing Chol/EPC (30:70) and liposomes containing cholesterol, EE/Chol/EPC(10:20:70) and EE/Chol/EPC(20:10:70) were also prepared using the similar methods.
Liposome properties
Vesicle size and morphology
The size and Zeta potential of liposome were determined by Malvern Zeta Sizer Nano2s (MS 2000, UK). Samples were prepared by diluting the liposome suspension with deionized water and sonicated for 30 s before measurement to ensure the homogeneous. Five measurements were carried out for each sample and the average value was recorded. The shape of liposome was observed at a magnification of 450X through the inverted microscope (TE2000-U, Nikon, Japan).
Encapsulation efficiency (EE)
The encapsulation efficiency (EE) was defined as the ratio of actual and original amount of ethinylestradiol encapsulated in liposomes. The calculation equation is as follows:
The amount of ethinylestradiol entrapped (actual amount value) in the liposome was determined by disrupting the vesicles using 0.1% v/v Triton X-100 and filtering it. Ethinylestradiol content in filtration was determined by high performance liquid chromatography (HPLC) using Water ALLIANCE 2695 series HPLC system with a violet detector at the wave length of 281 nm (U-3310). An Agilent CLC-ODS C18 (4.6mm’250mm, 5 μm, Agilent Technologies Inc., California, USA) column was used at ambient temperature. The mobile phase was an aqueous solution mixture of MeOH and H2O (70:30, v:v). The flow rate was 1.0 mL·min−1 and 50 uL of samples were injected. The chromatographic control system, data acquisition and analysis were performed using Water Empower software.
Liposome stability study
Liposomal drug retention and the size change were used as parameters to preliminarily indicate the physical stability of liposome. The protocol was adapted from Vangala’s report (Vangala et al Citation2006). Physical stability study of ethinylestradiol liposome (after separation of the free drug) at 4°C was conducted in 20 mL PBS for a period of 28 days. Three 5 mL independent samples, EE/EPC (30:70), EE/Chol/EPC(10:20:70), and EE/Chol/EPC(20:10:70), were prepared with the concentration of 0.2 mmol/l. At time intervals of 0 (immediately after preparation), 7, 14 and 28 days, samples were centrifuged (20 000 g, 30 min) and the supernatants were analyzed by HPLC at the appropriate wavelength.
Drug release kinetics
The release of ethinylestradiol from liposome was determined by incubating ethinylestradiol-loaded vesicles (after separation of unencapsulated ethinylestradiol) in PBS (physiological buffer, pH = 7.4) at a constant temperature of 37°C in a shaking (constant; 150 oscillations/min) water bath [Ali et al Citation2010, Hong et al 2002]. Initially, 5 mL of four independent testing samples, free EE, EE/EPC (30:70), EE/Chol/EPC (10:20:70), and EE/Chol/EPC (20:10:70), were prepared and each had the concentration of 0.2 mmol/L. Each sample had 5 parallel samples, so there were 20 samples in summary. The samples were hold in dialysis, which was fasten both ends and put into 30 mL PBS bath and shaking. At time intervals of 0, 2, 4, 8, 16, 24 and 48 h, 5 mL of PBS was withdrawn and the same amount of fresh buffer was replaced and make sure the whole PBS bath volume was 30 mL during the experiment period. The amount of ethinylestradiol release from liposome was determined by HPLC.
Animals and ovariectomized rat model
All studies were conducted in accordance with the internationally accepted “Guide for the Care and Use of Laboratory Animals” published by the US National Institutes of Health (NIH Publication No. 80–23, revised 1996), and approved by the local ethics committees for animal experimentation. Forty virgin female Sprague-Dawley rats, 3-month-old and weighing approximately 280 ± 2 g, were obtained from the Fourth Military Medical University (Xi’an, China). They were randomized by body weight to five groups and labeled separately as group I, II, III, VI, V. Eight rats in group V were killed at the beginning of the experiment for the initial baseline data control. The other 32 rats were underwent ovariectomy (ovx) or sham surgery by the dorsal approach under ether anesthesia. At three months postsurgery, the rats in group I were injected intraperitoneally 2 μg/kg body weight every day with free ethinlyestradiol PBS solution for one month. Those in group II were injected intraperitoneally 2 μg/kg body weight every day with ethinlyestradiol liposome (EE/EPC (20:80), used for the high encapsulation efficiency 98.92%) for one month. The rats in group III (ovx control) and group VI (sham control) were raised without any drug injection during the whole experiment period. At the experiment termination, all rats were killed by exsanguination under ether anesthesia and the right femur was harvested until length test. The blood samples were obtained from each rat’s arteria carotis and the serum was obtained through centrifuge (20 000 g, 10 min).
Body weight
The body weights of rats were measured using electronic balance (MP6001, quantity sensitive: 0.1g, China) every 30 days during the three months post-surgery and every 15 days during the following one month therapy. The changes of body weight were also reflected using the data of ΔG, which was calculated by the difference between the G0d and G120d, that was ΔG = G120d - G0d. Here, G0d and G120d was the rats’ body weight measured at the beginning of experiment (0 day) and 120th days.
BMD and bone length measurement
The bone mineral density (BMD, mg/cm2) of right femur was measured by PRODIGY Direct Digital DEXA (dual-energy X-ray absorptiometry) Bone Densitometry (GE Prodigy, USA). The length of the right femur was measured with a micrometer.
Serum ALP and calcium (Ca) and phosphours (P) measurement
The serum alkaline phosphatase (ALP), calcium (Ca), and phosphours (P) were detected using fully-automatic biochemical meter (7080, HITACHI, Japan).
Statistical analyses
Data were analyzed using the SPSS statistical package (V.10; Chicago, IL) by one-way analysis of variance (ANOVA). Statistically significant differences (P<0.05) were found between or within the experimental groups by ANOVA, individual differences were assessed by post-hoc analyses (Tukey’s test). Data were expressed as mean ± standard deviation (SD) in the text and tables, and as mean ± standard error in the figures.
Results
Liposome properties
Use the same procedure of preparation, the liposome size varied from 175.9 ± 2.6 nm to 235.1 ± 1.9 nm. The zeta potential was negative and varied from -28.3 ± 2.1 mV to -13.8 ± 1.5 mV. The encapsulation efficiency varied from 56.61 ± 0.35% to 98.92 ± 0.83% (). The liposome morphology was observed by microscope and the liposome had clear homogeneous lamellar vesicles ().
Table 1. Liposome properties (pH: 7.4, Mean ± PDI, n = 5 in each series).
Liposome Stability Study
The long term stability of ethinylestradiol liposome formulations was tested over a 28-day period at 4°C and drug retention monitored at weekly intervals (). After 7 days storage at 4°C, drug retention dropped to approximately 80% for all formulations. At time points thereafter, retention values for all preparations remained constant with no further significant loss of ethinylestradiol being detected up to day 28. Ethinylestradiol retention profiles were similar for the liposome with and without cholesterol containing at 4°C over the period of the study. It could be noted that there was no obvious difference in physical stability results between liposome with cholesterol containing and one without. Moreover, during the above period, the liposome size had no significant change and all formulation remained almost the same size (results not shown).
Figure 2. Ethinylestradiol retention of various vesicle formulations. The various formulations were in aqueous buffer, pH = 7.4, stored at 4°C for 28 days. Ethinylestradiol was encapsulated and drug retention was measured on the basis of un-entrapped ethinylestradiol recovered in the suspension following centrifugation at 20 000g for 30 min. Results represent percentage retention of initially incorporated ethinylestradiol and are expressed as the means of five experiments ± SD.
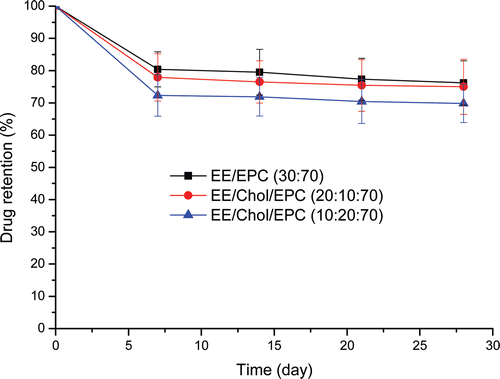
Drug release kinetics
Release data of ethinylestradiol from test liposomal dispersions into PBS pH 7.4 at 37°C using a dialysis method were shown as cumulative percent release over a 48 hours study period (). Diffusion of free ethinylestradiol in solution through the dialysis membrane was used as control. The release profile of free ethinylestradiol showed almost 100% diffusion after 4 hours. While entrapment of ethinylestradiol in liposome expectedly reduced in the drug release rate. The release profile of ethinylestradiol liposome showed just 40% diffusion and reached 70% after 48 hours. Moreover, the release profiles of ethinylestradiol liposomal dispersions were biphasic, showing a relatively large burst effect over the first four hours, followed by a slower release phase. The burst effect showed very little varieties with the cholesterol containing and how much it added. This results was different from the report addressed by Nounou [Nounou et al Citation2006], which showed that the cholesterol reduced the initial release rate and the effect being dependent on the EPC/Chol molar ratio.
Figure 3. Esthinylestradiol release from liposomes. The cumulative release profile was under physiological conditions from formulations in aqueous buffer, pH = 7.4, at 37°C. Esthinylestradiol was encapsulated and drug release was measured on the basis of un-entrapped esthinylestradiolrecovered in the suspension following centrifugation at 20 000g for 30 min at each time-point. Results represent percentage cumulative release of initially incorporated esthinylestradiol and were expressed as the means of four experiments ± SD.
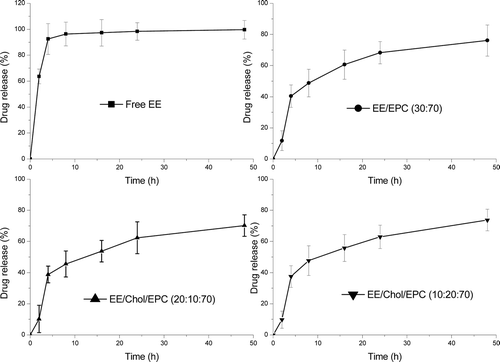
In terms of drug release model, three of the most common kinetic profiles, zero-order, first-order and Higuchi equations were applied [Hughes Citation2005] and the correlation coefficient R2 was used as the mark to determine the mechanism (). Higher correlation coefficients were obtained for Higuchi equation, indicating a diffusion controlled release model for ethinylestradiol liposome. The similar release model for hydrophobic drug-loaded liposome was also reported in the previous literature [Hathout et al. Citation2007; Nounou et al. Citation2006].
Table 2. Kinetic values of release esthinylestradiol from different liposomal formulations with and without cholesterol containing using the correlation coefficient parameter.
EEL effect on ovariectomized rats’ osteoporosis
Rats’ body weight
The ethinlyestradiol liposome EEL (EE/EPC (20:80), encapsulation efficiency: 98.92%) was used in animal test. The rats’ body weights and their weight change were used to show the rats living condition during the whole experiment period 120 days (). At the beginning of experiment, the average body weight of each group was similar (280 ± 2 g), while it had different change with different drug support. The rats in ovx group had the higher increasing than that of other groups, which showed an increasing of 40.0 % and reached 380 ± 3 g at the end of experiment. While the body weights of rats in sham group had the lowest growth, which just presented an increasing of 10.1 % and was 310 ± 2 g at the end of experiment. The rats in group I and group II, with EE and EEL (EE/EPC (20:80)) administration, all showed an increasing in body weight. The body weight change ΔG showed the same clear change tendency. The data analysis results showed the statistically significant differences among the groups (p<0.05).
Figure 4. The body weight changes during the experiment time (vs. ovx: *p<0.05; vs. sham: #p<0.05). The body weights of rats were measured using electronic balance every 30 days during the 3 months post-surgery and every 15 days during the following 1 month therapy. The changes of body weight were reflected using the data of ΔG, which was calculated by the difference between the G0d and G120d, that was ΔG = G120d - G0d. Results represent the rats living condition with the drug administration during the whole experiment period and were expressed as the means of eight animals ± SD.
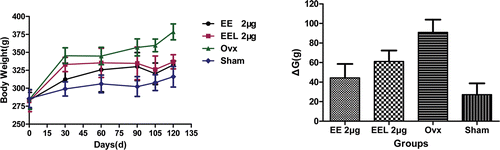
Bone mineral density (BMD) and length of right femur
During the whole experimental time, the BMD profiles in two drug therapy groups were biphasic, showing a relatively decreasing over three months post-surgery, followed by a significant increasing in one month drug injection therapy (, left). But BMD values presented the different increasing tendency, it increased by 20.3 % and 37.8 % with free EE (group I) and EEL (EE/EPC (20:80)) (group II) injection, respectively. The data showed that EEL (EE/EPC (20:80)) supplement made the BMD value increase higher than that of free EE. BMD showed different profiles for sham and ovx control groups, which was increasing in sham control group but decreasing in ovx control group during the whole experiment period.
Figure 5. BMD value and length of rats’ right femur (vs. ovx: *p<0.05). The bone mineral density (BMD, mg/cm2) was measured by PRODIGY Direct Digital DEXA (dual-energy X-ray absorptiometry) Bone Densitometry (GE Prodigy, USA) at different time internal. The length of the right femur was measured with a micrometer. Bone mass was measured by electronic balance (MP6001, quantity sensitive: 0.01g, China). Results were expressed as the means of eight animals ± SD.
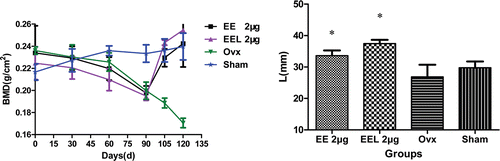
At baseline the average length of rats’ right femur was about 31.50 ± 0.12 mm. At the termination of experiment, the right femur length of the two therapy groups were all increased and their average values were all higher than the baseline values. While the right femur length of rats in sham and ovx control groups were all decreased (, right). In comparison, there were statistically significant differences between ovx group and therapy groups, but no statistically significant differences between ovx and sham group (p = 0.052).
ALP, Ca, P contents in serum
The ALP values of groups I and II were all significant higher than that in ovx group and had statistically significant differences among them (p = 0.007, p = 0.01) (). Compared with the baseline value, ALP value in group I (2 μg/kg free EE) increased by 28.6 %, while it increased by 42.0 % in group II (2 μg/kg EEL (EE/EPC (20:80)). For the serum calcium content, the values of ovx group were significant higher than that of other groups, which had statistically significant differences vs. group II (2 μg/kg EEL(EE/EPC (20:80)) and sham group (p = 0.04, p = 0.031). The serum phosphours contents in two therapy groups were all higher than those of ovx and sham groups.
Figure 6. The serum alkaline phosphatase (ALP), calcium (Ca), and phosphours (P) contents in serum (vs. ovx: *p<0.05▪vs. sham: #p<0.05). ALP, Ca and P contents in serum were detected using fully-automatic biochemical meter (7080, HITACHI, Japan). Results represent the bone formation after esthinylestradiol supplementary therapy and were expressed as the means of eight experiments ± SD.
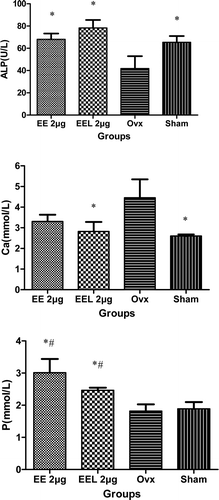
Discussion
Postmenopausal osteoporosis was the most common bone disease in women after menopause [Tabasum Parvez MD, Citation2004], which due to the estrogen declining after menopause. Low estrogen level caused the rate of bone resorption to increase and exceeded the rate of bone formation, potentially caused osteoporosis. Hormone replacement therapy (HRT) could be used to address postmenopausal osteoporosis, by supplementing hormone level, such as estrogen, which would contribute to bone loss. Ethinylestradiol, one kind of estrogen, was used as drug against the postmenopausal osteoporosis in clinical. However, long-term free estrogen used might result in some side effects to the women’s body [Roberts et al. 1986], especially in the high dosage. So the new estrogen dosage form should be developed and used in HRT instead of free estrogen. In our present research work, we formed ethinylestradiol liposome formulations and investigated the ethinylestradiol liposome effects on ovariectomized rats’ osteoporosis, and eventually found whether the liposome formulations had action to postmenopausal osteroprosis as that of free esthinylestradiol did in ERT processing.
Liposome properties
Ethinylestradiol liposome was formed through film evaporation and extrusion technique. The properties of liposome showed the high encapsulation efficiency (the high was almost 99.0% for EE/EPC (20:80)) and the size was lower than 240 nm, which might due to the lipophilicity of ethinylestradiol and it could be trapped between the alkyl chains of EPC. Thought the microphotograph of microscope, we know that the four extrusion cycles through filter made the liposomes have the properties of homogenous lamellar vesicle and homogenous size distribution, as well as the spherical shape.
Cholesterol was the main membrane-stabilizing material and was widely accepted to improve bilayer stability and reduced the permeability of liposomal bilayers [Gregoriadis and Davis Citation1979; Kirby and Gregoriadis Citation1980]. However, Mohammed [Mohammed et al. Citation2004] presented an argument to the use of cholesterol and showed that the presence of cholesterol could reduce drug incorporation efficiency within liposomal bilayers. So the possible alternatives to cholesterol would be advantageous. Ali [Ali et al. Citation2010] investigated the fatty alcohols as an alternative bilayer stabilizer to cholesterol and the results showed that liposomes could be effectively formulated using fatty alcohols as an alternative bilayer stabilizer to cholesterol.
In this paper, we used ethinylestradiol, one of typical representatives of steroid estrogens [Feng et al. Citation2010], as an alternative material to cholesterol and investigated its liposome behavior. The liposome EE/EPC (30:70), without cholesterol containing, exhibited a similar biphasic release pattern as that of the cholesterol formulation. Furthermore, the different amount of cholesterol added did not do much effect to the liposome stability and release protocol. Furthermore, from the data in , adding cholesterol in liposome decreased ethinylestradiol encapsulation yield. So we used ethinylestradiol as an alternative to cholesterol and formed the liposome without cholesterol containing.
Liposome effect on ovariectomized rats’ osteoporosis
All subjected rats had the increasing body weight suggested the good condition of the rats during the whole experiment period. The high body weight growth of rats in ovx groups, without ethinylestradiol given, might have good explanation to the increasing body weight of some postmenopausal women [De et al. 2005, Uusi-Rasi et al. Citation2010].
The bone mineral density (BMD) value at any time point was a combined function of one’s peak bone mass and the amount of bone loss since skeletal maturation. The low bone mineral density value was likely due to progressive bone loss. Daily treatment with free EE and EEL (EE/EPC (20:80)) for one month resulted in the increase of BMD [Eskridge and Morton 2010], which would combine with the increasing bone mass. Although the free ethinylestradiol and its liposome with the same amount supplement (2 μg/kg) all had the positive affection on rats’ BMD and made BMD increase, but EEL(EE/EPC (20:80)) supplement showed the higher BMD value than that of free EE. A monthly EEL (EE/EPC (20:80)) supplement seemly could stimulate more amount of calcium deposition in bone and increase the bone mineral content [Fonseca and Ward Citation2004, Boivin et al. Citation2005]. The above statement could also be the possible reason to explain the similar change trend of rats’ right femur length [Breitman et al. Citation2003].
The activity and osteogenesis of the osteoblastic were related with the content of alkaline phosphatase (ALP) in serum. The increasing value of ALP after one month therapy with free EE and EEL (EE/EPC (20:80)) meaned the active bone deposition, which reflected the high activity of osteoblastic and the active bone formation after therapy. The low Ca content in serum suggested there was almost no calcium loss from bone or bone loss was restricted through one month therapy. All of these date indicated that one month therapy with EEL could make the ovariectomized rats’ osteoporosis condition becoming better.
Possible reason for the positive effect of liposome against osteoporosis
We investigated the effects of ethinylestradiol liposome and free ethinylestradiol to the ovariectomized rats’ osteoporosis and all results of BMD and ALP showed that the ethinylestradiol liposome had the better action than that of free ethinylestradiol did. The following reasons might give the explanations to our research results.
It is well known that liposomes can ensure a physical barrier between the internal and the external compartments and thus protect encapsulated molecules [Kateb et al. Citation2008], which might make ethinylestradiol have chance to react with estrogen receptor and keep the balance between bone resorption and bone formation. Moreover, the liposomes were less capable of passing through barriers with pores smaller than their own diameter. So the liposome would stay a long time in circulation system and maintain a certain blood drug concentration in vivo, which resulted in the better effect against the ovariectomized rats’ osteoporosis than that of free EE. In addition, from the release protocol, the ethinylestradiol liposome was able to provide sustained release of ethinylestradiol, as a result there would be more constant blood levels as compared to the free ethinylestradiol. All of the above reasons made the ethinylestradiol liposome had the better results than that of free ethinylestradiol did.
Conclusion
Ethinylestradiol liposome was prepared and its effect against osteoporosis was investigated using ovariectomized rat model. Compared to free ethinylestradiol, ethinylestradiol liposome had the better effects in improving the condition of ovariectomized rats, showing an increasing in the value of BMD and ALP, which might due to the sustained release of the ethinylestradiol provided by its liposome and more constant blood levels maintained in vivo. But the metabolites and biodistribution of ethinylestradiol liposome in vivo need to investigate in the further research work.
Declaration of interest
This work was financially supported by the Natural Science Foundation of China (NSFC, No. 20872119) and NPU Foundation for Fundamental Research (No. NPU-FFR- WO18104).
References
- Ali MH, Kirby DJ, Mohammed AR, Perrie Y. 2010. Solubilisation of drugs within liposomal bilayers: alternatives to cholesterol as a membrane stabilizing agent. J Pharm Pharmacol 62:1–10.
- Barenholz Y. 2001. Liposome application: problems and prospects. Current Opinion in Colloid & Interface Science 6:66–77.
- Black DM, Eastell R, Boonen S, Bucci-Rechtweg C, Mesenbrink P. 2009. Time to onset of anti-fracture efficacy and persistence of effect of zoledronic acid 5 mg in women with osteoporosis or recent hip fracture. Bone 44: S234–S252
- Boivin G, Vedi S, Purdie DW, Compston JE, Meunier PJ. 2005. Influence of estrogen therapy at conventional and high doses on the degree of mineralization of iliac bone tissue: a quantitative microradiographic analysis in postmenopausal women. Bone 36: 562–567.
- Bonewald LF, Johnson ML. 2008. Osteocytes, mechanosensing and Wnt signaling. Bone 42: 606–615.
- Breitman PL, Fonseca D, Cheung AM, Ward WE. 2003. Isoflavones with supplemental calcium provide greater protection against the loss of bone mass and strength after ovariectomy compared to isoflavones alone. Bone 33: 597–605.
- Cederroth CR, Nef S. 2009. Soy, Phytoestrogens and metabolism: A review. Mol Cell Endocrinol 304: 30–42.
- Cuzick J. 2008. Hormone replacement therapy,and the risk of breast cancer. European J Cancer 44: 2344–2349.
- De Laet C, Kanis JA, Oden A, Johanson H, Johnell O, Delmas P. 2005. Body mass index as a predictor of fracture risk: a meta-analysis. Osteoporos Int 16: 1330–1338.
- Demir B, Haberal A, Geyik P, Baskan B, Ozturkoglu E, Karacay O, Deveci S. 2008. Identification of the risk factors for osteoporosis among postmenopausal women. Maturitas 60:253–256.
- Eskridge SL, Morton DJ. 2010. Estrogen Therapy and Bone Mineral Density in African-American and Caucasian Women. Am J Epidemiol 171: 808–816.
- Feng YJ, Wang C, Liu JF, Zhang ZH. 2010. Electrochemical degradation of 17-alpha-ethinylestradiol (EE2) and estrogenic activity changes. J Environ Monit 12: 404–408.
- Fonseca D, Ward WE. 2004. Daidzein together with high calcium preserve bone mass and biomechanical strength at multiple sites in ovariectomized mice. Bone 35: 489–497.
- Garg M, Mishra D, Agashe H, Jain NK. 2006. Ethinylestradiol-loaded ultraflexible liposomes: pharmacokinetics and pharmacodynamics. J Pharm Pharmacol 58, 459–468.
- Gobbi S, Cavalli A, Negri M, Schewe KE, Belluti F, Piazzi L, Hartmann RW, Recanatini M, Bisi A. 2007. Imidazolylmethylbenzophenones as highly potent aromatase inhibitors. J Med Chem 50:3420–3422.
- Gregoriadis G, Davis C. 1979. Stability of liposomes in vivo and in vitro is promoted by their cholesterol content in the presence of blood cells. Biochim Biophys Res Commun 90: 1287–1293.
- Hagino H, Nishizawa Y, Sone T, Morii H, Taketani Y, Nakamura T, Itabashi A, Mizunuma H, Ohashi Y, Shiraki M, Minamide T, Matsumoto T. 2009. A double-blinded head-to-head trial of minodronate and alendronate in women with postmenopausal osteoporosis. Bone 44: 1078–1084.
- Hathout RM, Mansour S, Mortada ND, Guinedi AS. 2007. Liposomes as an ocular delivery system for acetazolamide: in vitro and in vivo studies. AAPS PharmSciTech 8(1):E1–E12.
- Hengst V, Oussoren C, Kissel T, Storm G. 2007. Bone targeting potential of bisphosphonate-targeted liposomes Preparation, characterization and hydroxyapatite binding in vitro. Int J Pharm 331: 224–227.
- Hughes GA. 2005. Nanostructure-mediated drug delivery. Nanomedicine: Nanotechnol Biol Med 1: 22–30.
- Kateb NE, Cynober L, Chaumeil JC, Dumortier G. 2008. L-cysteine encapsulation in liposomes: Effect of phospholipids nature on entrapment efficiency and stability. J Microencapsulation 25(6): 399–413.
- Kirby C, Gregoriadis G. 1980. Effect of the cholesterol content of small unilamellar liposomes on their stability in vivo and in vitro. Biochem J 186: 591–598.
- Kita M, Kondo M, Koyama T, Yamada K, Matsumoto T, Lee K, Woo J, Uemura D. 2004. Symbioimine exhibiting inhibitory effect of osteoclast differentiation, from the symbiotic marine dinoflagellate symbiodinium sp. J Am Chem Soc 126: 4794–4795.
- Lindenfeld EA, Langer RD. 2002. Bleeding patterns of the hormone replacement therapies in the postmenopausal estrogen and progestin interventions trial. Obstet Gynecol 100: 853–863.
- Makras P, Hamdy NAT, Zwinderman AH, Ballieux BEPB, Papapoulos SE. 2009. Bisphosphonate dose and incidence of fractures in postmenopausal osteoporosis. Bone 44: 766–771.
- Mamot C, Drummond DC, Hong K, Kirpotin D, Park JW. 2003. Liposome-based approaches to overcome anticancer drug resistance. Drug Resist Updat 6:271–279.
- Mohammed AR, Weston N, Coombes AGA, Fitzgerald M, Perrie Y. 2004. Liposome formulation of poorly water soluble drugs: optimisation of drug loading and ESEM analysis of stability. Int J Pharmaceutics 285: 23–34.
- Nounou MM, EL-Khordagui LK, Khalafalla NA, Khalil SA. 2006. In vitro release of hydrophilic and hydrophobic drugs from liposomal dispersions and gels. Acta Pharm 56: 311–324.
- Otsuka M, Oshinbe A, LeGeros RZ, Tokudome Y, Ito A, Otsuka K, Higuchi WI. 2008. Efficacy of the injectable calcium phosphate ceramics suspensions containing magnesium, zinc and fluoride on the bone mineral deficiency in ovariectomized rats. J Pharm Sci 97: 421–432.
- Pasco JA, Henry MJ, Nicholson GC, Brennan SL, Kotowicz MA. 2009. Behavioural and physical characteristics associated with vitamin D status in women. Bone 44: 1085–1091.
- Peng KW, Wang HL, Qin ZH, Wijewickrama GT, Lu ML, Wang ZC, Bolton JL, Thatcher GRJ. 2009. Selective Estrogen Receptor Modulator Delivery of Quinone Warheads to DNA Triggering Apoptosis in Breast Cancer Cells. ACS Chem. Biol. 4: 1039–1049
- Peng KW, Chang MS, Wang YT, Wang ZC, Qin ZH, Bolton JL, Thatcher GRJ. 2010. Unexpected Hormonal Activity of a Catechol Equine Estrogen Metabolite Reveals Reversible Glutathione Conjugation. Chem. Res. Toxicol. 23: 1374–1383
- Poiana C, Carsote M, Neacsu E, Barbu C, Gruia A, Samoila R, Ene C. 2009. Vitamin D levels and bone mineral density in 174 postmenopausal. Bone 44: S398.
- Quadir MA, Radowski MR, Kratz F, Licha K, Hauff P, Haag R. 2008. Dendritic multishell architectures for drug and dye transport. J Control Release 132: 289–294.
- Raudaskoski T, Tapanainen J, Tomás E, Luotola H, Pekonen F, Ronni-Sivula H, Timonen H, Riphagen F, Laatikainen T. 2002. Intrauterine 10μg and 20μg levonorgestrel systems in postmenopausal women receiving oral oestrogen replacement therapy: clinical, endometrial and metabolic response. BJOG: An Int J Obstet Gynaecol 109: 136–144.
- Renoir J, Stella B, Ameller T, Connault E, Opolon P, Marsaud V. 2006. Improved anti-tumoral capacity of mixed and pure anti-oestrogens in breast cancer cell xenografts after their administration by entrapment in colloidal nanosystems. J Steroid Biochem Mol Biol 102: 114–127.
- Riera-Espinoza GS, Ramos J, Belzares E. 2009. Vitamin D deficiency in postmenopausal women with osteoporosis in Venezuela. Bone 44: S359.
- Rizzoli R, Felsenberg D, Laroche M, Seeman E, Krieg M, Frieling I, Thomas T, Delmas P. 2009. Strontium ranelate has a more positive influence than alendronate on distal tibia cortical and trabecular bone microstructure in women with postmenopausal osteoporosis. Bone 44: S211–S226.
- Schindeler A, Little DG. 2007. Bisphosphonate action: Revelations and deceptions from in vitro studies. J Pharm Sci 96: 1872–1878.
- Schmidt JM, Tremblay GB, Plastina MA, Ma F, Bhal S, Feher M, Dunn-Dufault R., Redden PR. 2005. In vitro evaluation of the anti-estrogenic activity of hydroxyl substituted diphenylnaphthyl alkene ligands for the estrogen receptor. Bioorg Med Chem 13: 1819–1828.
- Setchell KDR, Brzezinski A, Brown NM, Desai PB, Melhem M, Meredith T, Zimmer-Nechimias L, Wolfe B, Cohen Y, Blatt Y. 2005. Pharmacokinetics of a slow-release formulation of soybean isoflavones in healthy postmenopausal women. J Agric Food Chem 53:1938–1944.
- Shargorodsky M, Boaz M, Luckish A, Matas Z, Gavish D, Mashavi M. 2009. Osteoprotegerin as an independent marker of subclinical atherosclerosis in osteoporotic postmenopausal women. Atherosclerosis 204: 608–611.
- Suzuki Y, Nagase Y, Iga K, Kawase M, Oka M, Yanai S, Matsumoto Y, Nakagawa S, Fukuda T, Adachi H, Higo N, Ogawa Y. 2002. Prevention of bone loss in ovariectomized rats by pulsatile transdermal iontophoretic administration of human PTH(1-34). J Pharm Sci 91: 350–361.
- Tabasum Parvez MD, 2004. Postmenopausal osteoporosis. JK-Practitioner 11(4): 281–283
- Timmer MHM, Samson MM, Monninkhof EM, Ree BD, Verhaar HJJ. 2009. Predicting osteoporosis in patients with a low-energy fracture. Arch Gerontol Geriatr 49: e32–e35
- Tyler CR, Filby AL, Bickley LK, Cumming RI, Gibson R, Labadie P, Katsu Y, Liney KE, Shears JA, Silva-Castro V, Urushitani H, Lange A, Winter MJ, Iguchi T, Hill EM. 2009. Environmental health impacts of equine estrogens derived from hormone replacement therapy. Environ Sci Technol 43: 3897–3904.
- Uusi-Rasi K, Rauhio A, Kannus P, Pasanen M, Kukkonen-Harjula K, Fogelholm M, Sievänen H. 2010. Three-month weight reduction does not compromise bone strength in obese premenopausal women. Bone 46:1286–1293.
- Vangala A, Kirby D, Rosenkrands I, Agger EM, Andersen P, Perrie Y. 2006. A comparative study of cationic liposome and niosome-based adjuvent systems for protein subunit vaccines: characterisation, environmental scanning electron microscopy and immunisation studies in mice. J Pharm Pharmacol 58:787–799.
- Widler L, Jaeggi KA, Glatt M, Müller K, Bachmann R, Bisping M, Born A, Cortesi R, Guiglia G, Jeker H, Klein R, Ramseier U, Schmid J, Schreiber G, Seltenmeyer Y, Green JR. 2002. Highly potent geminal bisphosphonates. From pamidronate disodium (aredia) to zoledronic acid (zometa). J Med Chem 45: 3721–3738.
- Zhou Y, Zhu Z, Guan XU, Hou W, Yu H. 2009. Reciprocal roles between caffeine and estrogen on bone via differently regulating cAMP/PKA pathway: The possible mechanism for caffeine-induced osteoporosis in women and estrogen’s antagonistic effects. Med Hypotheses 73: 83–85.