Abstract
This work studied the intravenous injection formulation of nanostructured lipid carriers (NLCs) loaded with dexamethasone acetate (DA), a poorly water-soluble drug. The goal of this study was to design nanoparticles which could improve therapeutic efficacy of DA on inflammations. Based on the optimized results of single-factor screening experiment, DA-loaded NLCs (DA-NLCs) prepared by an emulsification-ultrasound method were found to be relatively uniform in size (178 ± 4 nm) with a negative zeta potential (-38 ± 4 mV). The average drug entrapment efficiency was 91 ± 3 %. In vitro release tests indicated DA-NLCs possessed a sustained release characteristic and the accumulative release percentage was near 80 % at 23 h. DA-NLCs exhibited an average peak concentration of DA (7.6 μg/ml) in the pleural exudate after intravenous administration to an experimental model of γ-carrageenan-induced pleuritis rats, which was 8.3 times higher than that of free DA (0.9 μg/ml). The γ-carrageenan-induced edema test showed that the anti-acute inflammatory activity of DA-NLCs was stronger than that of free drug at the same drug concentration (P<0.05). In addition, biodistribution results clearly indicated that DA-NLCs preferentially accumulated in mice livers and lungs after intravenous injection. These results revealed that injectable NLCs may serve as a promising carrier for DA, greatly enhancing the selective effect on inflammatory sites, reducing systematic side effects and may be a potential carrier to increase therapeutic efficacy on inflammatory diseases.
Introduction
Dexamethasone acetate (DA) is a synthesized glucocorticoid, which can be employed to treat chronic or active hepatitis for the prevention of liver fibrosis (CitationSung et al., 2005) and pulmonary diseases, such as infective atypical pneumonia, bronchial asthma, pulmonary fibrosis, acute lung injury and acute respiratory distress syndrome (CitationGroeneveld et al., 2002; CitationDik et al., 2003; CitationLi et al., 2004). However, the response rates obtained from recent clinical trials of DA were poor because of its poor solubility in water and non-significantly selective effect on inflammatory sites, which may further result in many systematic side effects (CitationBarbro et al., 2000; CitationChina Pharm., 2005). Considering these drawbacks, a serial of micro/nanoparticulate delivery systems were developed to solve these problems. For instance, PLGA nanoparticles were prepared to improve the aqueous solubility and in vitro release of dexamethasone, but burst release ocurred significantly (CitationCarolina et al., 2007). Although polytrimethylene carbonate was also used to encapsulate dexamethasone and sustained release was finally achieved, there were no in vivo results reported (CitationZhang et al., 2006). Nanostructured lipid carriers (NLCs), a new generation of lipid nanoparticles, consist of solid lipid matrices with spatially incompatible liquid lipids leading to a structure with more imperfections in crystal to improve drug incorporation and release properties (CitationMüller et al., 2002; CitationSouto et al., 2004). In our research group, CitationZhao et al (2011) indicated that employing phospholipids complex encapsulated in nanostructured lipid carriers was a novel strategy for incorporating dexamethasone and thus relatively high entrapment efficiency (89.82 ± 1.64%) and drug loading efficiency (2.13 ± 0.13%) were achieved. However, no in vivo results or anti-inflammatory activity study was reported to further confirm the improvement of dexamethasone’ pharmacologic actions by using such a novel strategy. In addition, tween 80 which could induce severe haemolysis was used in the study of CitationWang et al (2010). Due to the defects described above, the present work aimed to investigate the improvement of drug entrapment efficiency and release properties of DA loaded NLCs without employing tween 80 in the prescription. Moreover, the in vivo studies such as the enhancement of DA accumulation in inflammatory site, increased anti-inflammatory activity of DA in acute inflammation models of rats, and in vivo biodistribution of DA-NLCs and the free DA were investigated.
Materials and methods
Chemicals and animals
Dexamethasone acetate (DA) was supplied by Tianyao Pharmaceutical Co. Ltd. (Tianjin, P.R.C.). Compritol 888 ATO (glyceryl behenate, tribehenin) was a gift from Gattefossé (Gennevilliers, France). Pluronic 188 (F68) and γ-carrageenan were purchased from Sigma (St. Louis, MO, USA). Soybean oil for parenteral use was provided by Beiya Co. Ltd. (Tieling, P.R.C.). All other chemicals were of analytical grade.
Kunming strain mice weighing between 18 and 22 g, female or male and Wistar rats weighing between 180 and 220 g were purchased from the Laboratory Animal Center of Sichuan University (Chengdu, P.R.C.).
Preparation of DA-NLCs
DA loaded nanostructured lipid carriers (DA-NLCs) were prepared by emulsification-ultrasound method (CitationMehnert & Ma¨der, 2001). Firstly, lipid (Compritol 888 ATO and soybean oil) was heated to 80 °C and DA was dissolved in the melted lipid. Secondly, F68 was dissolved in double-distilled water, then the aqueous phase was heated to 80 °C as well. Lastly, the heated aqueous phase was added to the melting lipid and emulsified by probe sonication for 10 min. The resultant DA-NLCs were purified immediately by filtration through 0.45 μm cellulose acetate filter membrane to remove non-incorporated drug crystals. In addition, the blank NLCs were prepared according to the same method without adding DA. All batches of NLCs were produced at least in triplicate.
Characterization of DA-NLCs
The surface morphology of DA-NLCs was examined using a transmission electron microscope (TEM, H-600IV, Hitachi, Japan). Before analysis, the samples were diluted (1:5, v:v) and stained with 2 % (w:v) phosphotungstic acid and placed on copper grids with films for observation.
The mean particle size (Z-Average) and zeta potential of the DA-NLCs colloidal suspension were determined in triplicates by photon correlation spectroscopy (PCS, Malvern Zeta-Size Nano ZS90, UK). A proper dilution of the suspension was prepared with double-distilled water before each analysis.
Freeze-dried DA-NLCs (Freeze Dryer, Savant SNL 216V, USA, −60 °C, 24h) were accurately weighed in aluminum sample pans and then analyzed on a differential scanning calorimeter (EXSTAR6000 DSC, Japan).The samples were heated at speed of 10 °C/min in the range of 30–250 °C under nitrogen atmosphere at a flow rate of 50 ml/min. Free DA, F68, Compritol 888 ATO, blank nanoparticles and the physical mixture of the two substances (the proportion was consistent with that in optimized DA-NLCs) were chosen as references. The determinations were repeated three times for the formulations from three different batches
To determine the entrapment efficiency (EE), DA-NLCs were separated using Sephadex-G50 column (20 cm × 1.5 cm), washing with double-distilled water at a flow rate of 1.0 ml/min. The entrapped and free DA was respectively collected at a volume interval of 2.0 ml. The collected samples were diluted using a mixed solvent of methanol and water (7:3, v:v) and sonicated. DA was analyzed by reversed phase HPLC. The HPLC system consisted of an Alltech Model 426 HPLC Pump, an Alltech UVIS-201 Absorbance Detector, and an Allchrom plus Client/Server data operator (Alltech International, Inc., Virginia, USA). A Shimpack CLC-ODS C18 column (150 × 4.6 mm, 5 μm, Dikma Diamonsil, Dikma Inc., Shanghai, P.R.C) was eluted using a mobile phase of methanol:water (70:30, v:v) at a flow rate of 1.0 ml/min. The eluate was monitored at 30 °C and DA was assayed at a wavelength of 240 nm. Entrapment efficiency (EE) was calculated as given below:
EE % = (The amount of entrapped drug in DA-NLCs / the amount of entrapped drug in DA-NLCs and free drug in dispersion) × 100 %
In vitro drug release
The dialysis sac method was used to investigate the in vitro release of DA-NLCs under sink conditions. Briefly, 1 ml DA-NLCs colloidal suspension diluted with the phosphate-buffered saline (PBS, 0.1 M, pH7.4) was transferred into the dialysis sac (8∼12 KDa, Serua, Germany) and dialyzed against 100 ml PBS. During the dialysis, samples were protected from light and a constant temperature culturing shaking incubator (ZHWY-103B, Shanghai ZHICHENG Analytical Instrument Manufacturing Co., Ltd.) was used, which was thermostatted at 37 ± 1 °C and mechanically shaked at 70 rpm. At designated time internals, 0.5 ml dialysis medium was taken for measurement and the same volume of fresh medium was added. The amount of released DA was determined by HPLC using the method described previously. Experiments were performed in triplicate.
Drug distribution into an inflamed site
To determine the efficiency of DA-NLCs to deliver DA into an inflamed site, an experimental model of pleuritis in rats was induced by an intrapleural injection of 0.1 ml of 2 % γ-carrageenan to rats. Wistar rats were randomly divided into 14 groups (n = 5). 8 groups received DA-NLCs, and another 6 groups received DA 50 % propylene glycol solution. 2 h after the intrapleural injection of γ-carrageenan, DA-NLCs was administered intravenously to the rats at a dose of 3 mg/kg of DA. The rats were sacrificed at various intervals after intravenous administration by collecting blood from the aorta under ether anesthesia. The pleural cavity was opened and the pleural exudates collected. An aliquot of the exudate was centrifuged (2,500 g for 10 min) and the pleural cells were removed. The pleural exudate and the supernatant were used to determine DA by HPLC analysis as described above.
Anti-inflammatory activity
An anti-inflammatory test of DA-NLCs was performed according to former studies. (CitationLange et al., 1994; CitationChin et al., 1999; CitationGhamdi., 2001; CitationMei et al., 2005) Wistar rats were randomly divided into 5 groups with 5 animals per group. Group 1 (control) received normal saline (N.S.); groups 2 and 3 were injected with DA-NLCs (DA equivalent dose of 3 mg/kg and 0.6 mg/kg); and groups 4 and 5 received DA 50 % propylene glycol solution (3 mg/kg and 0.6 mg/kg). Drugs and normal saline were given through the tail veins 1 hour before subcutaneous injection of γ-carrageenan into the right hind paws. The paw volume was measured before drug administration and then at 1, 2, 3, 4, 5 and 6 hours after γ-carrageenan injection, using a digital plethysmometer. The amount of paw swelling was determined from time to time and expressed as follows: the percentage inhibition rate = (edema value of control group − edema value of drug group)/edema value of control group × 100 %.
Biodistribution studies in mice
Two groups of 130 Kunming strain mice were used for the in vivo distribution studies (CitationYe et al., 2008). One group was administered with DA-NLCs at a DA equivalent dose of 7 mg/kg and the other group with free DA as control. Five mice in each group were taken out randomly at each time interval (0.08, 0.25, 0.5, 1, 2, 4, 6, 8, 10, 12, 15, 16, 18, 20 h). Blood samples of mice were collected from the orbit venous plexus directly after eyeball removal and placed into heparinized test tubes, then centrifugated at 5,000 g for 10 min to plasma samples. Mice were sacrificed, dissected and tested and tissues of heart, liver, spleen, lung, kidney and brain were removed. Plasma and tissue samples within the same time interval were pooled together for further process (n = 5). After that, accurately weighted organ specimen was placed in a homogenizing tube with double volume of double-distilled water and homogenized in an ice-bath. The extraction of DA in plasma and the homogenized tissues was operated using the methods described below (Minting et al., 2010). Plasma or tissue homogenates (0.5 ml) were mixed with 0.1 ml methyltestosterone solution (8.7 μg/ml) as the internal standard and 0.2 ml PBS (pH = 2.0). The mixture was vortexed with 3 ml acetoacetate and then centrifuged (5,900 g, 10 min). The obtained supernatant was evaporated to dryness at 30 °C under vacuum (Vacuum Drying Oven, DZF-6050, Shanghai Jing Hong Laboratory Instrument Co., Ltd.). After that, the residue was redissolved in 0.2 ml methanol, following centrifugation at 14,000 g for 10 min. Finally, 20 μl of the supernatant was injected into the HPLC system. The same HPLC condition was used as described above.
Statistical analysis
Values were expressed as mean ± S.D. The data obtained were analyzed statistically by one-way analysis of variance and the Student’s t-test for comparison between the means. Difference was considered significant at a P-value of less than 0.05.
Results and discussion
Preparation and characterization of DA-NLCs
To enhance the incorporation efficiency of DA, both solid lipid (Compritol 888 ATO) and liquid lipid (soybean oil) were employed to prepare NLCs (CitationMuller et al., 2002) in this experiment. Taking EE as an index, the preparation was optimized by single factor screening experiment. The results showed that the volume of water phase had the least influence on EE of DA-NLCs, while initial DA mass, F68 concentration and the ratio of Compritol 888 ATO and soybean oil (w:w) exerted a significant effect (P<0.05). When initial DA mass increased, the EE increased significantly while the EE decreased gradually with the increase of F68 concentration. Considering the foamy emulsion and the potential cytotoxicity resulted from higher surfactant concentrations (CitationSpeiser, 1990), 3 % F68 was selected in the optimized preparation. In addition, 6 mg of DA was chosen in the optimal formulation because of the deposit of DA crystals with the further increase of initial DA mass. As for the effect of the lipid composition, the EE first increased dramatically with the increase of the ratio of Compritol 888 ATO and soybean oil, until it reached 77 ± 6 % for the mass ratio of 450:150. However, with the further increase of the mass ratio, the EE decreased significantly (). Accordingly, the optimized formulation was prepared using 6 mg DA, 3 % F68, 450 mg Compritol 888 ATO and 150 mg soybean oil and finally the optimized EE of 91 ± 3 % was obtained.
Table 1. Influence of the mass ratio of solid lipid materials to liquid lipid materials on the properties of DA-NLCs (Mean ± S.D., n = 3).
TEM was conducted to investigate the morphology of DA-NLCs. It can be seen that the nanoparticles had relatively spherical shapes (). The particle size determined by TEM agreed well with the results from PCS (). Apparently, the encapsulation of DA into NLCs had little effect on the average particle size and the surface charge of NLCs.
Table 2. Average particle size, polydispersity index and zeta potential of blank NLCs and DA-NLCs (mean±S.D., n = 3).
The broad water peak (∼100 °C) in DSC may greatly affect the judgment of the curves of the lipid nanoparticles. In view of this, the NLCs were lyophilized before the DSC analysis (CitationCavalli et al., 2000; CitationVighi et al., 2007) and powdered DA-NLCs were subjected to DSC measurements. showed DSC thermograms of Compritol 888 ATO, DA, F68, the physical mixture and freeze-dried nanoparticulate systems with or without DA. Compritol 888 ATO and F68 exhibited a peak at 75.1 °C and 55.6 °C, respectively ( and ). DA had a melting point at 235.1 °C. The DSC results indicated no drug peak for any of the particles around 235.1 °C (, , ). The peak of Compritol 888 ATO in the DA-NLCs displayed a shift from 75.1 °C to 72.9 °C, which was the same as the depression of Compritol 888 ATO’s melting point (from 75.1 °C to 72.9 °C) detected in the physical mixture (). Compared with blank NLCs, there was a similar depression of the melting point for Compritol 888 ATO in DA-NLCs and the physical mixture (), which revealed that the presence of DA did not influence the thermograms of NLCs.
In vitro drug release
In this experiment, dynamic dialysis was employed for the separation of free DA from DA-NLCs. showed DA release profile from DA solution and DA-NLCs in PBS. The release of DA from DA-NLCs was well fitted to the Weibull equation (r = 0.9892). The release profile of DA from NLCs was biphasic. The initial burst release of about 30 % of drug from the DA-NLCs was observed within the first 2 h incubation, which could be attributed to drug desorption from the surface of the nanoparticles. Subsequently, DA-NLCs exhibited a certain sustained release characteristic and the accumulative release percentage at 23 h was near 80%. The slow release of the drug in the latter phase was explained by the fact that the solublized or dispersed DA can be released gradually from the lipid matrices through dissolution and diffusion (CitationGrassi et al., 2003). By contrast, the release of crude DA was much faster, with almost 100% of DA dissolving in the release medium within 2 h. These results revealed that DA-NLCs greatly improved the drug release property compared with dexamethasone-loaded PLGA nanoparticles characterized by significant burst release of DA approximate to 90 % during the first 4 h of incubation (CitationCarolina et al., 2007).
Drug distribution into an inflamed site
The distribution of lipid nanoparticles into acute-inflammatory site was conducted for the first time in an experimental rats model of γ-carrageenan-induced pleuritis. DA-NLCs displayed an average peak concentration of DA (7.6 μg/ml) at 1h in the pleural exudate after intravenous administration, which was 8.3 times higher than that of free DA (0.9 μg/ml) at 0.5 h (). In addition, the AUC for free DA and DA-NLCs were around 1.3 and 13.8 mg/L.h, respectively. After 4h, 0.8 μg/ml of DA remained in the pleural exudates for DA-NLCs, while free DA had already been cleared completely at 2.5 h. These results demonstrated that DA-NLCs greatly enhanced the distribution and prolonged the residence time of DA at the inflamed site. It was reported nanoparticles could offer outstanding systems to optimize infection specificity (CitationDouglas et al., 2010). Acute inflammation was characterized by vasodilation, increased permeability of blood vessels and the slowing of blood flow, which allowed nanoparticles enter extravascular space and accumulate at sites of inflammation. Hence, such efficient delivery of DA into the inflamed site would potentially improve the therapeutic efficacy on inflammations.
Anti-inflammatory activity
To investigate the therapeutic efficacy of DA and DA-NLCs on inflammations, the model of γ-carrageenan induced paw edema was used. The rats’ right hind paw became edemateous soon after injection of γ-carrageenan and paw edema volume peak reached around 3 h in this experiment. The results obtained with different dosages of free DA, DA-NLCs and normal saline in the γ-carrageenan-induced edema test at specific time intervals were shown in . Results indicated that the anti-acute inflammatory activity of DA-NLCs was stronger than that of free DA at the same concentration (p<0.05), which was in coincidence with the higher concentration of DA distributed in inflammatory sites for DA-NLCs. The activity of DA-NLCs at high dose (3 mg/kg) was approximately 1.3- to 1.7-time higher than that of free DA. Similarly, the activity of DA-NLCs at low dose (0.6 mg/kg) were about 1.3- to1.6-fold higher than that of free DA. Moreover, an increase in anti-inflammatory activity was observed in a concentration dependent manner.
Biodistribution studies in mice
The DA concentrations in plasma and tissues after administration of free DA and DA-NLCs were summarized in and showed the pharmacokinetic parameters of DA calculated in plasma and tissues.
Table 3. Major pharmacokinetic parameters of free DA and DXM-NLCs in plasma and tissues.
Figure 6. The DA concentrations in plasma, liver and lung (A) and other tissues (B) after intravenous administration of free DA and DA-NLCs.
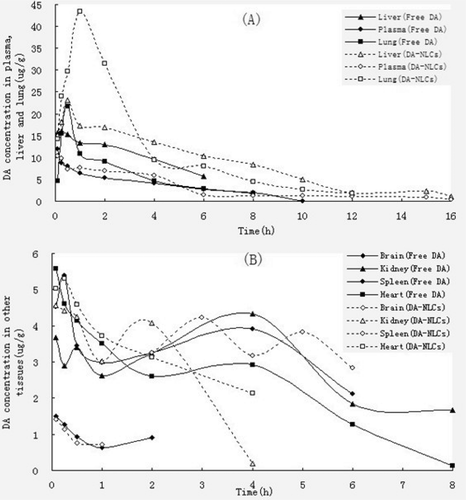
These results clearly indicated that DA-NLCs preferentially accumulated in liver and lung, which are important reticuloendothelial system (RES) organs, after intravenous injection. In liver, the peak DA concentrations increased from 15.3 μg/g to 32.2 μg/g (0.5 h) and a 1.8-fold larger area under the curve for DA-NLCs was achieved compared to that of free DA. These results was well in accordance with earlier reports on 5-fluorouracil SLNs (CitationYu et al., 2000), clozapine SLNs (CitationManjunath et al., 2005) and oxymatrine SLNs (CitationSun et al., 2007) that proved the passive targeting properties of SLNs towards the RES organs. In lung, the peak concentration of DA-NLCs (43.4 μg/g) was reached at 1 hour. In contrast, the peak concentration (21.8 μg/g) of free DA in the lung occurred at 0.5 hours. Besides, a 2.6-fold larger area under the curve for DA-NLCs was achieved compared to that of free DA (). Other groups also showed that using nanoparticulate delivery system could increase the drug biodistribution in the lung (CitationLöbenberg et al., 1998; CitationZara et al., 1999; CitationSanthi et al., 2000).
The results of in vivo studies convincingly confirmed that DA-NLCs improved the DA accumulation in inflammatory sites and enhanced the therapeutic efficacy of DA on inflammations. Since DA-NLCs could be targeted to liver and lung, it may be used to treat chronic and/or active hepatitis and some pulmonary diseases.
Conclusions
In this study, we successfully incorporated the lipophilic DA into nanostructured lipid carriers (NLCs). In vitro release tests showed DA-NLCs possessed sustained release after an initial burst release. Compared with free DA, DA-NLCs demonstrated that higher drug levels in the pleural exudate and superior pharmacological activity were found in DA-NLCs. Furthermore, DA-NLCs exhibited a prolonged residence time of the drug in plasma, and significantly improved the biodistribution of DA to liver and lung of mice. These results indicated that injectable NLCs may serve as an excellent carrier for DA, greatly enhancing the selective effect on inflammatory sites, reducing systematic side effects such as diabetes (CitationHoogwerf et al., 1999) and increasing therapeutic efficacy on chronic active hepatitis and some pulmonary diseases.
Declaration of interest
This research was supported by National S&T major project (2009zx09310-002) and Shenzhen Science and Technology Planning Project (201002046)
References
- Azarmi, S., Roa, W.H., Löbenberg, R. (2008). Targeted delivery of nanoparticles for the treatment of lung diseases. Adv Drug Deliv Rev. 60:863–875.
- Barbro, N.M., Peter, O., Vivian, K.J. (2000). Dexamethasone coupled to albumin is selectively taken up by rat nonparenchymal liver cells and attenuates LPS-induced activation of hepatic cells. J Hepatol. 32:603–611.
- Cavalli, R., Caputo, O., Gasco, M.R. (2000). Preparation and characterization of solid lipid nanospheres containing paclitaxel. Eur J Pharm Sci. 10:305–309.
- Carolina, G.G., Nicolas, T., Madeleine, B., Amelie, B., Elias, F. (2007). Encapsulation of dexamethasone into biodegradable polymeric nanoparticles. Int J Pharm. 331:153–159.
- Chin C.T., Chun C.L. (1999). Anti-inflammatory effects of Taiwan folkmedicine ‘Teng-Khia-U’ on carrageenan-and adjuvant-induced paw edema in rats. J Ethnopharmacol. 64:85–89.
- China Pharmacopeial Convention. (2005). Chinese Pharmacopoeia. Section 2:184–185.
- Dik, W.A., McAnulty, R.J., Versnel, M.A., Naber, B.A., Zimmermann, LJ., Laurent, G.J., and Mutsaers, S.E. (2003). Short course dexamethasone treatment following injury inhibits bleomycin induced fibrosis in rats. Thorax. 58:765–71.
- Douglas, T., Young, M.J., Suci, P. (2010). Novel nanoparticles for biofilm targeting. United States Patent USP0021391.
- Fundaro, A., Cavalli, R., Bargoni, A., Vighetto, D., Zara, G. P., and Gasco, M. R., Non-stealth,and stealth solid lipid nanoparticles (SLN). (2000). carrying doxorubicin: pharmacokinetics and tissue distribution after i.v. administration to rats. Pharm Res. 42:337–343.
- Gert, S., Sheila, 0.B., Toos, D., and Danilo, D.L. (1995). Surface modification of nanoparticles to oppose uptake by the mononuclear phagocyte system. Adv Drug Deliv Rev.17:31–48.
- Ghamdi MSA. (2001). The anti-inflammatory analgesicand antipyretic activityof Nigella Sativa.. J Ethnopharmacol.76:45–48.
- Grassi, M., Voinovich, D., Franceschinis, E., Perissutti, B., Filipovic-Grcic, J. (2003). Theoretical and experimental study on theophylline release from stearic acid cylindrical delivery systems. J Control Release 92:275–289.
- Groeneveld, AB. (2002). Vascular pharmacology of acute lung injury and acute respiratory distress syndrome. Vasc. Pharmacol. 39:247–256.
- Hoogwerf B., Danese R.D. (1999). Drug selection and the management of corticosteroid-related diabetes mellitus. Rheum Dis Clin North Am. 25:489–505.
- Lange M., Malyusz M. (1994). Improved determination of small amounts of free hydroxyproline in biological fluids. Clin Chem. 409:1735–1738.
- Lu W., He L.C., Wang C.H., Li Y.H., Zhang S.Q. (2008). The use of solid lipid nanoparticles to target a lipophilic molecule to the liver after intravenous administration to mice. Int J Biol Macromol. 43:320–324.
- Li, H.P., Li, X., He, G.J., Yi, X.H., Kaplan, A.P. (2004). The influence of dexamethasone on the proliferation and apoptosis of pulmonary inflammatory cells in bleomycininduced pulmonary fibrosis in rats. Respirology. 9:25–32.
- Löbenberg, R., Araujo, L., Von Briesen, H., Rodgers, E., Kreuter, J. (1998). Body distribution of azidothymidine bound to hexyl-cyanoacrylate nanoparticles after i.v. injection to rats. J Control Release. 50:21–30.
- Manjunath, K., Venkateswarlu, V. (2005). Pharmacokinetics, tissue distribution and bioavailability of clozapine solid lipid nanoparticles after intravenous and intraduodenal administration. J Control Release. 107:215–228.
- Mehnert, W., Ma¨der, K. (2001). Solid lipid nanoparticles: Production, characterization and applications. Adv Drug Deliv Rev. 47:165–196.
- Mei, Z.N., Li, X. K., Wu, Q. R., Hu, S., Yang, X. L. (2005). The research on the anti-inflammatory activity and hepatotoxicity of triptolide-loaded solid lipid nanoparticle. Pharmacological Research. 51: 345–351.
- Moghimi, S.M. (1995). Mechanisms regulating body distribution of nanospheres conditioned with pluronic and tetronic block copolymers. Adv Drug Deliv Rev.16:183–193.
- Müller, R.H., Maaseen, S., Schwarz, C., Mehnert, W. (1997). Solid lipid nanoparticles (SLN) as potential carrier for human use: interaction with human granulocytes. J Control Release. 47:261–269.
- Müller, R.H., Radtke, M., Wissing, S.A. (2002). Nanostructured lipid matrices for improved microencapsulation of drugs. Int J Pharm. 242:121–128.
- Santhi, K., Dhanaraj, S.A., Koshy, M., Ponnusankar, S., Suresh, B. (2000). Study ofbiodistribution of methotrexate-loaded bovine serum albumin nanospheres in mice. Drug Dev Ind Pharm. 26:1293–1296.
- Scholer, N, Hahn, H, Müller, R.H, Liesenfeld, O. (2002). Effect of lipid matrix and size of solid lipid nanoparticles (SNLC) on the viability and cytokine production of macrophages. Int J Pharm. 231:167–176.
- Scholer, N, Olbrich, C, Tabatt, K, Müller, R.H, Hahn, H, Liesenfeld O. (2001). Surfactant, but not the size of solid lipid nanoparticles (SNLC) influences viability and cytokine production of macrophages. Int J Pharm. 221:57–67.
- Souto, E.B., Wissing, S.A., Barbosa, C.M., Müller, R.H. (2004). Evaluation of the physical stability of SLN and NLC before and after incorporation into hydrogel formulations. Eur J. Pharm Biopharm. 58:83–90.
- Speiser, P. (1990). Lipidnanopellets als Tragersystem fur Arzneimittel zur peroralen Anwendung. European Patent EP0167825.
- Sun, J.Y., Zhou, Z.F., Liu, F., Chen, G.S. (2007). Pharmacokinetics and tissue distribution of oxymatrine-SLN. Chin Pharm J. 42:1091–1095.
- Sung, H.K., Dal, W.C., Choon, W.K., Sang, G.K. (2005). Lack of therapeutic improvement of liver fibrosis in rats by dexamethasone in spite of ascites amelioration. Chem Biol Interact. 152:37–47.
- Vighi, E., Ruozi, B., Montanari, M., Battini, R., Leo, E. (2007). Re-dispersible cationic solid lipid nanoparticles (SLNs) freeze-dried without cryoprotectors: characterization and ability to bind pEGFP-plasmid. Eur J Pharm Biopharm. 67:320–328.
- Wang, M.T., Jin, Y., Yang, Y.X., Zhao, C.Y., Yang, H.Y., Xu, X.F., Qin, X., W, Z.D., Zhang, Z.R., Jian, Y.L., Huang. Y. (2010). In vivo biodistribution, anti-inflammatory, and hepatoprotective effects of liver targeting dexamethasone acetate loaded nanostructured lipid carrier system. Int J Nanomedi. 5:487–497.
- Wang, J.X., Sun, X., Zhang, Z.R. (2002). Enhanced brain targeting by synthesis of 3, 5-dioctanoyl-5-fluoro-2-deoxyuridine and incorporation into solid lipid nanoparticles. Eur J Pharm Biopharm. 54:285–290.
- Yang, S.C., Lu, L.F., Cai, Y., Zhu, J.B., Liang, B.W., Yang, C.Z. (1999). Body distribution in mice of intravenously injected camptothecin solid lipid nanoparticles and targeting effect on brain. J Control Release. 59:299–307.
- Ye, J.S., Wang, Q., Zhou, X.F., Zhang, N. (2008). Injectable actarit-loaded solid lipid nanoparticles as passive targeting therapeutic agents for rheumatoid arthritis. Int J Pharm. 352:273–279.
- Yu, B.T., Zhang, Z.R., Zeng, R.J. (2000). Study on the liver targeted 5-fluorouracil solid lipid nanoparticles. Yaoxue Xuebao. 35:704–705.
- Zara, G.P., Cavalli, R., Fundarò, A., Bargoni, A., Caputo, O., Gasco, M.R. (1999). Pharmacokinetics of doxorubicin incorporated in solid lipid nanospheres (SLN). Pharmacol Res. 40:281–286.
- Zhang, Z., Dirk, W., Grijpma, J.F. (2006). Poly(trimethylene carbonate) and monomethoxy poly(ethylene glycol)-block-poly(trimethylene carbonate) nanoparticles for the controlled release of dexamethasone. J Control Release. 111:263–270.
- Zhao, C.Y., Liu, Y., Fan, T.T., Zhou, D., Yang, Y., Jin, Y., Zhang, Z.R., Huang, Y. (2011). A novel strategy for encapsulating poorly soluble drug into nanostructured lipid carriers for intravenous administration. Pharm Dev Technol. 1–14, Early Online.