Abstract
Owing to its rationale of targeting the drug to the site of action and minimizing systemic toxic effects of the drug, intra-articular drug delivery system has gained growing interests. In this study, emphasis was placed on intra-articular Lornoxicam -loaded PLGA microspheres (Lnxc-PLGA-MS) preparation and improving the targeting of lornoxicam (Lnxc) in knee joint. The microspheres were prepared by a process involving solid-in-oil-in-water(S/O/W) emulsion, and evaluated for physicochemical properties. Joint cavity′s drug leakage into systemic circulation in rabbits was examined to define the drug stagnation. Meanwhile, drug retention in synovial fluid in rats was investigated to further validate the drug targeting. The microspheres were spherical as evidenced by the SEM photographs with mean size of 7.47μm, and encapsulation efficiency was observed 82.22% along with drug loading 12.17%. DSC revealed that the drug in the microspheres existed in the phase of uncrystallization. The formulated microspheres could prolong the drug release up to 32 days in vitro. Comparing with animals injected with lornoxicam solution, the plasma drug concentration decreased in rabbits and retention time increased in rats’ synovial fluid with intra-articular injections of microspheres, revealing good targeting efficiency. In conclusion, PLGA microspheres could be used to deliver lornoxicam following intra-articular administration for enhancing targeting efficiency.
Introduction
Osteoarthritis (OA) is a degenerative joint disease common among elderly people that causes progressive joint degeneration leading to chronic pain and reduced quality of life (CitationHunter et al. 2008; CitationM. Fransen 2010; CitationSayre et al. 2010). The incidence of OA increases with age, approximately 100% of men and women at age 75–79 years show some signs of OA (CitationReva C. Lawrence1 1998). Because of the chronic nature, OA requires the development of drugs suitable for chronic systemic treatment. Nevertheless, the oral or systemic administration of such drugs is hindered by numerous side effects. Due to the localized nature of the disease, Intra-articular delivery could be a pretty good choice by compartmentally targeting drugs into arthritic joint and has been used widely for patients suffering OA. The rationale for intra-articular therapy is to target the drug to the site of action and to minimize systemic toxic effects of the drug. However, most intra-articular preparations leak quickly into the systemic circulation after administration, thus sustained release preparations are needed imperiously to achieve a prolonged therapeutic effect. Strategies that could significantly increase the intra-articular retention time of anti-arthritic drugs would have the potential to favorably impact the progression of arthritis in a targeted joint(CitationBetre et al. 2006).
Recently, the use of polymeric carriers in formulations of therapeutic drug delivery systems, such as gelatin (CitationTabataIkada 1989; CitationTabata et al. 1999; CitationSakakibara et al. 2002), chitosan(CitationGuo et al. 2010; CitationPatil et al. 2010), Poly(lactide-co-glycolide) (PLGA)(CitationTsungBurgess 2001) and poly(lactic acid) (PLA)(CitationShiveAnderson 1997), has gained widespread application, due to their advantage of being biodegradable and biocompatible(CitationTuncay et al. 2000). Among the various polymeric carrier systems, PLGA microspheres have been shown to be very suitable systems for drug targeting and drug delivery, due to their advantages in longer release time, biodegradability, lack of toxicity and antigenicity. PLGA-hydrolysis produces lactic and glycolic acids, which are metabolised in the Krebs cycle to CO2 and water(CitationTamber et al. 2005). Incorporation of drugs within biodegradable polymer particles proved to be effective in improving the retention of drug within the joint cavity (CitationHorisawa et al. 2002; CitationGerwin et al. 2006; CitationInoue et al. 2006).
Lornoxicam (Lnxc) is a novel non-steroidal anti-inflammatory drug (NSAID) of the oxicam class of NSAIDs which leads to inhibition of cyclooxygenase(COX), an enzyme involved in the production of chemicals, including some prostaglandins, in the body(CitationBerg et al. 1999; CitationSen et al. 2006; CitationAyan et al. 2008; CitationPatil et al. 2009). Lornoxicam’s powerful inhibition of cyclo-oxygenase has manifested itself as highly potent analgesic and anti-inflammatory effects in animal studies and also prevented the bone destruction which normally occurs in the adjuvant polyarthritic rat(CitationPruss et al. 1990). Animal studies have shown that lornoxicam in therapeutic doses can also stimulate the synthesis of proteoglycans in cartilage which is the main protein involved in cartilage formation. Intra-articular injection of lornoxicam could relieve pain, reduce inflammation and promote the repair of damaged joints(CitationZhang hu 2006). It varies in its ability to control symptoms and also has disease-modifying effects. Because of the quick clearance of Lnxc solution (Lnxc-Sol) from the arthticular joint(CitationDing Yue 2004; CitationSaricaoglu et al. 2008), frequent IA injections are required. Therefore, research and development of safe, long-term, low side effects formulation to treat arthritis is the common aspiration of medical researchers and patients. Unfortunately, little attention has been taken to the Lnxc microspheres preparation.
The objective of the present study was to prepare intra-articular Lnxc-PLGA-MS for targeting drugs into arthritic joint and achieving sustained drug release and prolonged articular retention. The microspheres were prepared by a process involving solid-in-oil-in-water(S/O/W) emulsion, and evaluated for encapsulation efficiency, drug loading, size distribution, scanning electron microscopy (SEM), differential scanning calorimetry (DSC) and in vitro drug release. Drug leakage from joint cavity into blood in rabbits was examined to define the drug stagnation indirectly. Meanwhile, drug retention in synovial fluid in rats was investigated to further validate the drug targeting. This study explored a new way for Lnxc intra-articular administration, and enriched the research content of articular cavity delivery system.
Material and methods
Materials
Poly (D,L-lactic-co-glycolic) (PLGA, 75:25, Av. MW: 25kDa. Viscosity: 0.27dl/g (CHCl3/25°C)) was purchased from Shandong Institute of Medical Instrument (Shandong, China). Lnxc was provided by Zhejiang Zhenyuan Pharmaceutical Co. Ltd (Zhejiang, China). Gelatin (G9382, from bovine skin, gel strength: ~225g Bloom) and Dialysis bags with a molecular mass cut-off of 12,000Da were obtained from Sigma Chemical Co. (Beijing, China). All other chemicals and reagents used in this study were of analytical grade.
Preparation of dried PLGA microspheres loaded with Lnxc
Lnxc-PLGA-MS were prepared by a process involving solid-in-oil-in-water (S/O/W) multi emulsion. A sample of 400μl suspension including 4mg Lnxc was suspended in 2ml of 4% PLGA (w/v) solution dissolved in dichloromethane. After stirring at high speed for 1 min, the resulting primary emulsion was injected to 15 mL of 4% gelatin solution to form emulsion. A high-speed homogenizer (DIAX900, Heidolph & Co. Germany) was used for the multi emulsion, operating at 12000 rpm for 2 min. The resultant emulsion was continuously stirred for 3h at room temperature under ambient pressure until all the dichloromethane had evaporated. The solidified microspheres were recovered by centrifugation and washed four times with distilled water.
The dried microspheres were prepared with two dry methods-vacuum drying method and freeze drying method. Centrifuged microspheres were spread on a slide and kept in a vacuum tank (ZDF-1, Lvdeng, China) at room temperature (25°C) for 72h. Meanwhile, another batch was suspended in 2 % mannitol solution. The suspension was rapidly freezed to -80°C and then dried in a freeze-drier (Virtis freeze mobile, Virtis Co., USA) (-45°C) for 12 h.
Determination of encapsulation efficiency and drug loading
To measure encapsulation efficiency and drug loading, 20mg of dried microspheres was dissolved in 2 ml of acetonitrile and then diluted into 10 ml with water followed by centrifugation for 10 min at 10000 rpm. The supernatant was determined using UV-Visible Recording Spectrophotometer (UV-2102PCS, Unico, Shanghai) at 376 nm. The dissolved polymers have almost no absorption at 376 nm, so the presence of dissolved polymers does not interfere with the absorbance of the drug. The calibration curve for the quantification of Lnxc was drawn in advance. It was confirmed from preliminary UV studies that the presence of dissolved polymers did not interfere with the absorbance of the drug at 376 nm. The encapsulation efficiency and drug loading was calculated using following equations (1) and (2), respectively.
Particle size analysis
Particle size distributions were determined by laser light scattering (Mastersizer X, Malvern, Worcestershire, UK, 136 equipped with a 100-mm lens) using a Fraunhofer diffraction model. The PLGA microspheres (20 mg) were dispersed in distilled water (70 ml) with a few drops of 1% polysorbate 80 solutions. All size distributions were presented in the volume-weighted mode and characterized by their 10%, 50%, and 90% undersize diameters. The 50% undersize diameter was referred to as the mean diameter, and d (v, 0.1) and d (v, 0.9) were used to characterize the span of the distribution.
Morphological studies
The Lnxc-PLGA-MS were dispersed in distilled water, and a droplet was dropped directly on a slide and immediately observed under optical microscopy (Olympus, Tokyo, Japan) with a Polaroid camera attached (Model MF-10, Newton, MA, USA). Photographs were taken during examinations.
Scanning Electron Microscopy (SEM) of Lnxc-PLGA-MS was performed using a JEOL 5800LV scanning electron microscope at an accelerating voltage of 15–20 kV. The samples were coated with gold using a Denton Vacuum DV-502A.
Thermal Properties of Microspheres
DSC (Mettler Toledo DSC 822e) was performed on free Lnxc, accessories, blank PLGA microspheres, and Lnxc-PLGA-MS to determine the thermal behavior. It was also used to determine the existence of possible interaction between the polymer and drug. Samples were heated from 40°C to 300°C at the heating rate of 10°C/min.
In vitro release of Lnxc
The in vitro release profile of Lnxc from PLGA microspheres was determined by adding 2 mL of phosphate buffer (pH 7.4, containing 1% polysorbate 80) to Lnxc-PLGA-MS (containing 2 mg Lnxc). The release medium was selected based on solubility to maintain the sink condition, and the suspension was put into the analysis bags (Sigma) with a molecular mass cut-off of 12000Da using 2 ml Lnxc solution (containing 2 mg Lnxc) as a control. Then the bags were suspended in vials containing 28 ml of PBS. All the vials were kept in shaker water bath maintained at 37°C, 100 rpm. At predetermined time intervals, 5 ml of supernatant was taken for analysis. Fresh medium of the equal volume was added in the meantime. The concentration of Lnxc in the release medium was measured by UV-Visible spectrophotometer at 376 nm. The release experiments were carried out in triplicate and results of in vitro release were expressed as mean ± SD (n = 3).
In order to determine the release mechanism of Lnxc from PLGA microspheres, the release data were evaluated by model-dependent methods.
Determination of targeting efficiency
The targeting efficiency studies were complied with the requirements of the Nation Act on the use of experimental animals (People’s Republic of China)
Joint cavity leakage in rabbits after intra-articular injection administration
Ten New Zealand white rabbits (2.5 ± 0.5kg) were divided into two groups. The animals were fasted over-night prior to the experiment, with free access to water. The left knee joint of each rabbit was injected intra-articularly with Lnxc-PLGA-MS (equal to 4mg Lnxc /kg) or Lnxc dispersed in 0.4ml of N.S. using a 30 gauge needle. For each preparation and each sampling time point, five rabbits were treated with a single dose of 4mg Lnxc /kg after the injection of Lnxc-PLGA-MS and the Lnxc-Sol.
Blood samples were obtained from the marginal ear vein of the rabbits at 5, 15, and 30 min, 1, 1.5, 2, 3, 4, 5, 6, 8, 12, 24, 36, 48, 60, 72, and 96h after the intra-articular injection followed by centrifugation at 4000rpm for 15min. And the supernatant was collected and stored at -20°C for drug analysis.
Drug retention in the synovial fluid in rats
Fifty Wister rats (250 ± 20g) were used in the in vivo test and divided into two groups. One group was administered intra-articularly using a 30 gauge needle with Lnxc-PLGA-MS (equal to 4mg Lnxc /kg) dispersed in 0.2ml of N.S. and the other group with Lnxc-Sol as control. The synovial joints were flushed with 1 ml of NS and the fluid was filtered for drug analysis.
Lnxc in the rat synovial fluid were extracted according to the method previously reported with modification (CitationLiang et al. 2004). Briefly, 2 ml acetonitrile was added to the sample (0.5 ml) vortex mixed for 2min and centrifuged at 4000rpm for 5min, the supernatant was transferred to glass tube and dried under a stream of nitrogen gas at 40°C. The dried sample was reconstituted with 100μl acetonitrile-water (2:0.5) and 20 µL of it was injected for analysis.
Chromatographic conditions
The determination of Lnxc in various samples was carried out using the reversed phase high performance liquid chromatography method with the analytical column Shimadzu-C18 column (4.6 mm × 250 mm). Lnxc was monitored at a wavelength of 376 nm. The mobile phase comprised methanol and 0.05mol·L−1 Sodium acetate (60:40, v/v); the flow rate was 1.0 mL/min. All chromatography was performed at room temperature. Aliquots of supernatants (20 µL) were injected into the HPLC column for all the analyses.
Results and discussions
Preparation of microspheres
The solid-in-oil-in-water(S/O/W) emulsion method was employed to prepare the Lnxc-PLGA-MS considering the poor aqueous and lipid solubility of Lnxc. While the primary emulsion was dispersed into the aqueous solution, the emulsion formed was stabilized using a hydrophilic surfactant gelatin as an emulsifier. A high stirring speed (12000rpm) was maintained to ensure the microspheres formed were in the sub-10μm size range which has good syringeability. Stirring time was optimized as 3 h, which was found to be adequate for the complete evaporation of dichloromethane from the medium.
The resulting higher viscosity restricts the migration of the compound from the solidifying microspheres to the external phase by means of lowered diffusion and increased stability of the drug/matrix dispersion(CitationFreitas et al. 2005). The solvent evaporation method has been used extensively to prepare PLA and PLGA microspheres containing many different drugs. Several variables have been identified which can influence the properties of the microspheres, including drug solubility, internal morphology, solvent type, diffusion rate, temperature, polymer composition and viscosity, and drug loading. These variables must be considered in order to develop a successful controlled release PLGA microspehres containing drugs (McGinityO’Donnell 1997; CitationMorita et al. 2000).
In a special embodiment, gelatin, which is particularly well accepted as a product which can be injected into the organism has been used as emulsifying agent(A. Kishida 1990). Gelatin stabilized emulsions remained moderately stable to droplet aggregation(CitationSurh et al. 2006). Gelatin is a biodegradable, biocompatible and nonimmunogenic product, suitable for medical applications and widely used in the pharmaceutical industry as well as in the biomedical field: hard and soft capsules, microspheres, sealants for vascular prostheses, wound dressing and adsorbent pad for surgical use are among its most frequent applications(CitationDraye et al. 1998). Gelatin does not express antigenicity in physiological conditions, and it is much cheaper and easier to obtain in concentrate solutions(CitationBigi et al. 2001).
Encapsulation efficiency and drug loading
The incorporation of therapeutic agent into PLGA microspheres is affected by factors like the method of preparation, drug physicochemical characteristics, drug and polymer concentrations, phase ratio, and stabilizer species and concentrations. Encapsulation efficiency was defined as the Lnxc found in the microspheres as a percentage of the input one. An average entrapment efficiency of (82.22 ± 1.21) % and an average drug loading of (12.17 ± 0.68) % were achieved in the Lnxc-MS with the optimized formulation.
The encapsulation of Lnxc by the conventional O/W or W/O/W solvent evaporation method will generally result in microspheres with little drug loading due to the poor solubility both in water and oil phase. To achieve the goal of successful encapsulation of Lnxc in the microspheres, modified S/O/W multiple emulsion technique has been used to encapsulate the drug into PLGA micropsheres with relative high drug loading and encapsulation efficiency. The results showed that encapsulation efficiency and drug loading were high enough to do further research.
Particle size analysis
Size and size distribution of microspheres were recorded by laser light diffraction technique. displayed the particle size distribution pattern typically for three kinds. On an undried Lnxc-loaded PLGA microsphere basis, particle size distribution was found to be unimodal with narrow size distribution. The mean particle size of the microspheres was found to be 7.47μm, which had no obvious difference from the freeze-dried microsheres. The microspheres dried with vacuum drying method showed wide size distribution and bigger size, indicating the severe adhesion among the microspheres.
Figure 1. Size distribution of Lnxc-PLGA-MS. A: undried microspheres; B: freeze dried microspheres; C: vacuum dried microspheres
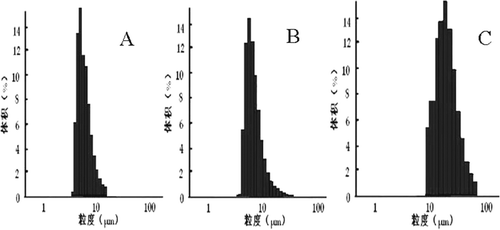
There are residual organic solvent in the core of the microspheres after solvent evaporation. During a drying process, microspheres may become soften due to the residual solvent and water migrating into the surface of microspheres. The elastic microspheres tended to aggregate during vacuum drying(CitationH 1997). As this event leads to the aggregation of adjacent microspheres, a drying method should be taken into deliberation.
SEM
SEM of microspheres dried by two methods-vacuum drying method and freeze drying method were shown in . By SEM examination, the Lnxc microspheres prepared by freeze drying method were spherical and smooth, while the ones prepared by vacuum drying method adhered to each other severely.
Results from the above indicated that Lnxc-PLGA-MS which were dried with vacuum drying method adhered severely, while the freeze-dried microspheres showed no adhesion. Meanwhile, vacuum drying takes too much time (72 h), which is not advisable. Thus, freeze drying is more suitable for the drying of PLGA microspheres.
DSC
DSC thermograms of Lnxc-PLGA-MS (freeze drying without mannitol)(A), Lnxc-PLGA-MS (freeze drying with mannitol) (B), physical mixture(with mannitol) (C), physical mixture(without mannitol) (D), mannitol(E), PLGA(F), gelatin(G), Lnxc (H) are displayed in . Thermogram of Lnxc showed a sharp endothermic peak at 225°C. While there was no peak corresponding to Lnxc in the DSC curve of Lnxc-PLGA-MS (A and B), it was most likely because that the drug existed in the phase of uncrystallization in the microspheres. Since the melting point of drug was not altered in the physical mixture (C and D), it indicated the absence of any chemical interaction of drug either with the polymer or the stabilizer agent. In a word, in the Lnxc-PLGA-MS, Lnxc is molecularly dispersed or in an amorphous state in the polymer matrix.
Figure 3. The DSC results of Lnxc loaded PLGA microspheres (A) Lnxc loaded microspheres(freeze drying without mannitol), (B) Lnxc loaded microspheres (freeze drying with mannitol), (C) physical mixture(with mannitol), (D) physical mixture(without mannitol), (E) mannitol, (F) PLGA, (G) gelatin, (H) Lnxc.
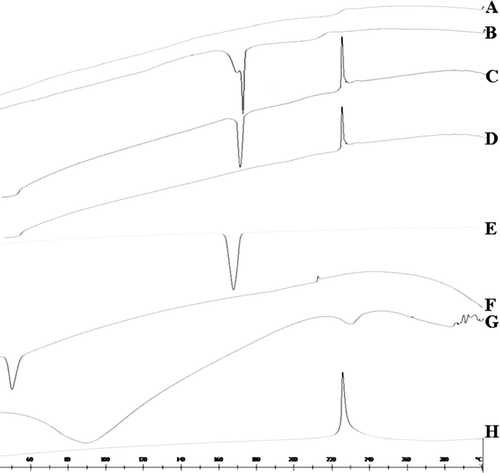
Lnxc release from Lnxc-loaded PLGA microsphere
The in vitro release curves of Lnxc-PLGA-MS were shown in . While the Lnxc solution released 98% of the drugs in the first 5 h, the Lnxc-PLGA-MS released only 6%. The cumulative amount of Lnxc released in 32 days was over 80% and release rates of microspheres slowed down thereafter.
Table 1. The regression equation of in vitro release of Lnxc and Lnxc-PLGA-MS
Release kinetics
In vitro release has been recognized as an important element in drug development. Under certain conditions it can be used as a surrogate for the assessment of bioequivalence. Several theories/kinetics models describe drug dissolution from immediate and modified release dosage forms(CitationPaulo Costa 2001). The model that fits the Lnxc-PLGA-MS release curves best was the Weibull model, with a good correlation coefficient (r > 0.99) (Tab. 1). It revealed that the method used in our study could fabricate microspheres that have controlled ability on the drug release.
The in vitro release behavior of Lnxc-PLGA-MS investigated in pH7.4 phosphate buffer saline containing 1% Tween-80 could be described by Weibull equation and could be expressed by the following equation: lg[-ln(1-Q)] = 0.6517lgt-1.5349, r = 0.996. The in vitro release profiles of Lnxc solution could be described by First-order kinetics equation and could be expressed by the following equation: ln(100-Q)=- t+100, r = 0.9999.
Determination of targeting efficiency
Joint cavity leakage in rabbits after intra-articular injection administration
A linear relationship between Lnxc concentrations in plasma and ratios of peak areas of Lnxc over the internal standard was obtained in the concentration range of 5-400ng/mL (r = 0.9990) by the HPLC assay. The plasma Lnxc concentrations after a single intra-articular injection of either Lnxc-PLGA-MS or free Lnxc are shown in .
Figure 5. Mean plasma concentration of Lnxc after intra-articular injection of Lnxc-Soland Lnxc-PLGA-MS in rabbits (n = 5).
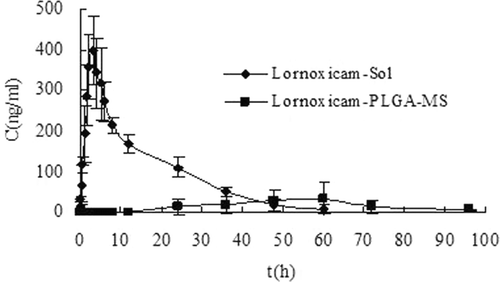
The dose of free Lnxc-Sol was calculated based on the actual loading of the microspheres so that the doses of Lnxc for both groups were the same. The clearance of free Lnxc from knee joint of the rabbits was rapid. The plasma Lnxc concentration increased 5 min after the injection and plasma concentrations reached a maximum 3 h after the injection (tmax of 3 h and cmax of 400.79ng/mL). The plasma concentrations gradually declined and were below the limit of quantitation of the assay (5ng/mL) 60h after the injection. On the other hand, the plasma Lnxc concentrations of those rabbits injected with Lnxc-PLGA-MS can not be found until at 24 h. Plasma concentrations reached a maximum 60 h after the injection (tmax of 60 h and cmax of 31.61ng/mL) and declined a minimum 96 h.
Table 2. The pharmacokinetic parameters of Lnxc after intra-articular injection of Lnxc-Sol and Lnxc-PLGA-MS in rabbits (n = 5)
The pharmacokinetic parameters are summarized in Tab. 2. The area under the curve (AUC) of solution group is 4.02 times more than the microspheres group, indicated more drugs leaked to the systemic cycle of rabbits from the joint cavity in the solution group. After intra-articular injection to rabbits, drug concentration in plasma maintained at a relatively low level in microspheres group. The results showed that Lnxc-loaded microspheres may retain the drug in the joint by reducing clearance from the joint into the blood.
Drug retention in the synovial fluid in rats
To evaluate whether drug loaded microspheres were retained in the joint, synovial fluid of rats were collected for analysis. The concentrations of Lnxc at different time points after intra-articular injections of either Lnxc-Sol or Lnxc-PLGA-MS were given in .
Figure 6. The distribution of Lnxc in synovial fluid of rats at different time points after intra-articular injection of Lnxc-Sol and Lnxc-PLGA-MS (n = 5).
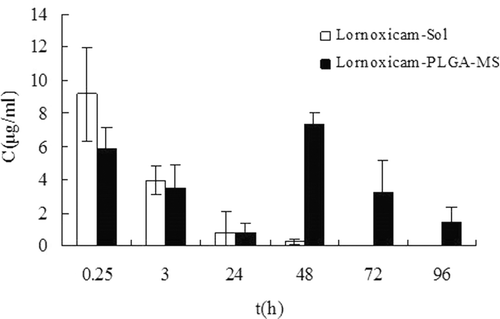
At 0.25h following the intra-articular injection, the concentration of Lnxc in rats synovial fluid after injection with Lnxc microspheres was higher than 5.870μg/ml which was due to the initial burst release. After injection with Lnxc solution, the concentration of Lnxc in the synovial fluid decreased quickly to be lower at 48h than the limit of quantitation while that of rats injected with Lnxc-PLGA-MS reached maximum (7.307μg/ml), indicating the prolonged retention time in the joint cavity.
There are three main pathways for drugs and small solutes to exchange between plasma and synovial membrane. 1) Small molecules may be taken up by the cells in the synovium, pass through the synovial tissue into the capillaries and then into the systemic circulation, or pass into the lymphatic system. 2) Colloidal substances greater than 10 nm do not gain access to the blood stream but they do reach regional lymph nodes. 3) Particles greater than 100 nm and less than 5 μm are phagocytozed by macrophages and carried to subsynovial tissue(CitationLiang et al. 2005). It is likely that Lnxc-PLGA-MS remained trapped in the joint cavity and less leakage to the joint may also be due to the microsheres size above 5 μm (∼7.47μm). The present study showed that there was a reductive leakage of Lnxc from the joint cavity following intra-articularly injection of Lnxc-loaded PLGA microspehres. This type of system can be used to retain molecules within the joint for a prolonged period of time.
Conclusion
To achieve long-term drug exposure, different established formulations such as suspensions and hydrogels, and also novel approaches such as lipid based formulations and nano- or microparticles are currently in development(CitationGerwin et al. 2006). Intra-articular injection of Lnxc-PLGA-MS is a potential treatment of OA. Comparing the joint cavity leakage and drug retention in the synovial fluid of Lnxc-PLGA-MS group with that of control group, the present study demonstrated that intra-articular administration of Lnxc-PLGA-MS enhances drug targeting in joint cavity due to the prolonged stagnation time of drug in the joint. PLGA microspheres could be effective in preventing drug leakage induced by the clearance of small drug molecule and prolonging the retention time in the joint cavity. From the clinical point of view, more studies will be performed to to examine therapeutic efficacy of Lnxc-loaded PLGA micropsheres in a mouse model of antigen-induced arthritis, and determine whether the micropsheres have a potential clinical application.
Acknowledgements
Declaration of interest
The authors report no conflicts of interest. The authors alone are responsible for the content and writing of the paper.
References
- A. Kishida, JBD, Yoshioka, S., Aso, Y., Takeda, Y. (1990). Some Determinants of Morphology and Release Rate from Poly(L)Lactic Acid Microspheres. Journal of Controlled Release.13, 83–89.
- Ayan E, Kaplan MB, Koksela O, Tamer L, Karabacak T, Ayaz LOzdulger A (2008). Efficiency of Lornoxicam in Lung and Trachea Injury Caused by Peroxynitrite. Pulmonary Pharmacology and Therapeutics.21, 201–207.
- Berg J, Fellier H, Christoph T, Grarup JStimmeder D (1999). The Analgesic Nsaid Lornoxicam Inhibits Cyclooxygenase (Cox)-1/-2, Inducible Nitric Oxide Synthase (Inos), and the Formation of Interleukin (Il)-6 in Vitro. Inflammation Research.48, 369–379.
- Betre H, Liu W, Zalutsky MR, Chilkoti A, Kraus VBSetton LA (2006). A Thermally Responsive Biopolymer for Intra-Articular Drug Delivery. J Control Release.115, 175–182.
- Bigi A, Cojazzi G, Panzavolta S, Rubini KRoveri N (2001). Mechanical and Thermal Properties of Gelatin Films at Different Degrees of Glutaraldehyde Crosslinking. Biomaterials.22, 763–768.
- Ding Yue DH, Shangli Liu Ruofan, Ma (2004). The Efficacy and Safety of Lornoxicam in Treatment of Rheumatoid Arthritis and Osteoarthritis. Chinese journal of new drugs.13, 562–564.
- Draye JP, Delaey B, Van de Voorde A, Van Den Bulcke A, De Reu BSchacht E (1998). In Vitro and in Vivo Biocompatibility of Dextran Dialdehyde Cross-Linked Gelatin Hydrogel Films. Biomaterials.19, 1677–1687.
- Freitas S, Merkle HPGander B (2005). Microencapsulation by Solvent Extraction/Evaporation: Reviewing the State of the Art of Microsphere Preparation Process Technology. J Control Release.102, 313–332.
- Gerwin N, Hops CLucke A (2006). Intraarticular Drug Delivery in Osteoarthritis. Adv Drug Deliv Rev.58, 226–242.
- Gerwin N, Hops CLucke A (2006). Intraarticular Drug Delivery in Osteoarthritis. Advanced Drug Delivery Reviews.58, 226–242.
- Guo L, Liu G, Hong RYLi HZ (2010). Preparation and Characterization of Chitosan Poly(Acrylic Acid) Magnetic Microspheres. Mar Drugs.8, 2212–2222.
- H S (1997). Microencapsulation Techniques Using Ethyl Acetate as a Dispersed Solvent: Effects of Its Extraction Rate on the Characteristics of Plga Microspheres. Journal of Controlled Release.47, 233–245.
- Horisawa E, Hirota T, Kawazoe S, Yamada J, Yamamoto H, Takeuchi HKawashima Y (2002). Prolonged Anti-Inflammatory Action of Dl-Lactide/Glycolide Copolymer Nanospheres Containing Betamethasone Sodium Phosphate for an Intra-Articular Delivery System in Antigen-Induced Arthritic Rabbit. Pharm Res.19, 403–410.
- Hunter DJ, McDougall JJKeefe FJ (2008). The Symptoms of Osteoarthritis and the Genesis of Pain. Rheumatic Diseases Clinics of North America.34, 623–643.
- Inoue A, Takahashi KA, Arai Y, Tonomura H, Sakao K, Saito M, Fujioka M, Fujiwara H, Tabata YKubo T (2006). The Therapeutic Effects of Basic Fibroblast Growth Factor Contained in Gelatin Hydrogel Microspheres on Experimental Osteoarthritis in the Rabbit Knee. Arthritis and Rheumatism.54, 264–270.
- Liang LS, Jackson J, Min W, Risovic V, Wasan KMBurt HM (2004). Methotrexate Loaded Poly(L-Lactic Acid) Microspheres for Intra-Articular Delivery of Methotrexate to the Joint. J Pharm Sci.93, 943–956.
- Liang LS, Wong WBurt HM (2005). Pharmacokinetic Study of Methotrexate Following Intra-Articular Injection of Methotrexate Loaded Poly(L-Lactic Acid) Microspheres in Rabbits. J Pharm Sci.94, 1204–1215.
- M. Fransen, SM, Hernandez-Molina, G., Reichenbach, S. (2010). Does Land-Based Exercise Reduce Pain and Disability Associated with Hip Osteoarthritis? A Meta-Analysis of Randomized Controlled Trials. Osteoarthritis and Cartilage.18, 613–620.
- McGinity JWO’, Donnell PB (1997). Preparation of Microspheres by the Solvent Evaporation Technique. Adv Drug Deliv Rev.28, 25–42.
- Morita T, Sakamura Y, Horikiri Y, Suzuki TYoshino H (2000). Protein Encapsulation into Biodegradable Microspheres by a Novel S/O/W Emulsion Method Using Poly(Ethylene Glycol) as a Protein Micronization Adjuvant. Journal of Controlled Release.69, 435–444.
- Patil KR, Rane VP, Sangshetti JNShinde DB (2009). Stability-Indicating Lc Method for Analysis of Lornoxicam in the Dosage Form. Chromatographia.69, 1001–1005.
- Patil S, Babbar A, Mathur R, Mishra ASawant K (2010). Mucoadhesive Chitosan Microspheres of Carvedilol for Nasal Administration. J Drug Target.18, 321–331.
- Paulo Costa JMSL (2001). Modeling and Comparison of Dissolution Profiles. European Journal of Pharmaceutical Sciences.13 123–133.
- Pruss TP, Stroissnig H, Radhofer-Welte S, Wendtlandt W, Mehdi N, Takacs FFellier H (1990). Overview of the Pharmacological Properties, Pharmacokinetics and Animal Safety Assessment of Lornoxicam. Postgraduate Medical Journal.66 Suppl 4, S18–21
- Lawrence Reva C., Helmick, Charles G., Arnett, Frank C., (1998). Estimates of the Prevalence of Arthritis and Selected Musculoskeletal Disorders in the United States. Arthritis & Rheumatism.41, 778–799.
- Sakakibara Y, Nishimura K, Tambara K, Yamamoto M, Lu F, Tabata YKomeda M (2002). Prevascularization with Gelatin Microspheres Containing Basic Fibroblast Growth Factor Enhances the Benefits of Cardiomyocyte Transplantation. Journal of Thoracic and Cardiovascular Surgery.124, 50–56.
- Saricaoglu F, Dal D, Atilla P, Iskit AB, Tarhan O, Asan EAypar U (2008). Effect of Intraarticular Injection of Lornoxicam on the Articular Cartilage & Synovium in Rat. Indian Journal of Medical Research.127, 362–365.
- Sayre EC, Li LC, Kopec JA, Esdaile JM, Bar SCibere J (2010). The Effect of Disease Site (Knee, Hip, Hand, Foot, Lower Back or Neck) on Employment Reduction Due to Osteoarthritis. Plos One.5,.
- Sen S, Doger FK, Aydin ON, Karul ADost T (2006). The Protective Effect of Nitroglycerin on Gastrointestinal and Renal Side Effects of Lornoxicam in Rats. Eur J Pharmacol.541, 191–197.
- Shive MSAnderson JM (1997). Biodegradation and Biocompatibility of Pla and Plga Microspheres. Adv Drug Deliv Rev.28, 5–24.
- Surh J, Decker EAMcClements DJ (2006). Properties and Stability of Oil-in-Water Emulsions Stabilized by Fish Gelatin. Food Hydrocolloids.20, 596–606.
- Tabata Y, Hijikata S, Muniruzzaman MIkada Y (1999). Neovascularization Effect of Biodegradable Gelatin Microspheres Incorporating Basic Fibroblast Growth Factor. J Biomater Sci Polym Ed.10, 79–94.
- Tabata YIkada Y (1989). Synthesis of Gelatin Microspheres Containing Interferon. Pharm Res.6, 422–427.
- Tamber H, Johansen P, Merkle HPGander B (2005). Formulation Aspects of Biodegradable Polymeric Microspheres for Antigen Delivery. Adv Drug Deliv Rev.57, 357–376.
- Tsung MJBurgess DJ (2001). Preparation and Characterization of Gelatin Surface Modified Plga Microspheres. AAPS PharmSci.3, 14–24.
- Tuncay M, Calis S, Kas HS, Ercan MT, Peksoy IHincal AA (2000). In Vitro and in Vivo Evaluation of Diclofenac Sodium Loaded Albumin Microspheres. J Microencapsul.17, 145–155.
- Zhang hu Zx Li, huifan (2006). The Effects of Lornoxicam on Chronic Arthritis in Rat. yunnan medicine.27, 420–422.