Abstract
Thymoquinone (2-isopropyl-5-methyl-1,4-benzoquinone) is a herbal-derived drug with potential chemopreventive and chemotherapeutic activity. However, thymoquinone suffers from high hydrophobicity causing poor solubility which limits its bioavailability and high lipophilicity causing poor formulation characteristics. Liposomes are versatile drug carriers that can be used to solve problems of drug solubility, instability, and bio-distribution. In this study, we were able to prepare thymoquinone-loaded liposomes (TQ-LP) and thymoquinone loaded in liposomes modified with Triton X-100 (XLP) with diameters of about 100 nm, and entrapment efficiency of more than 90% for TQ-LP and of 49.6% for XLP. The TQ-LP liposomes were effective in suppressing the proliferation of breast cancer cell lines MCF-7 and T47D, and at the same time exerting very low toxicity on normal periodontal ligament fibroblast. Altogether, this report describes the first successful encapsulation of thymoquinone into liposome; which maintains stability, improves bioavailability and maintains its anticancer activity.
Introduction
Cancer, being the second leading cause of death, is a major health concern both in developed and developing countries. A huge amount of research focused on finding novel and effective therapeutic agents for this disease. Unfortunately, most currently available drugs designed to kill tumor cells have severe side effects due to their general toxicity and lack of selectivity in targeting tumor cells. Due to these difficulties, there has been growing interest in naturally occurring phyto-chemical compounds with anticancer activity. Such compounds are relatively non-toxic, inexpensive and available in an ingestive form in most cases (Newman & Cragg, Citation2007).
Thymoquinone (2-isopropyl-5-methyl-1,4-benzoquinone) () is the main bioactive component of the volatile oil of the medical plant Nigella sativa (Black seed), and it has been shown to excrete anticancerous, antioxidant and anti-inflammatory activities both in vitro and in vivo (Worthen et al., Citation1998; Kaseb et al., Citation2007; Mansour et al., Citation2002; Chehl et al., Citation2009). Many previous reports have shown promising antitumor characteristics of thymoquinone (TQ) against different types of cancer cell lines in vitro, including human colorectal cancer cells (Gali-Muhtasib et al., Citation2004), myeloblastic leukemia cells (El-Mahdy et al., Citation2005), prostate cancer (Kaseb et al., Citation2007), pancreatic adenocarcinoma (Worthen et al., Citation1998) and ovarian and breast adenocarcinoma (Shoieb et al., Citation2003). The chemoprevention potential of TQ has been also recognized. For example, TQ inhibited the action of 20-methyl-cholanthrene induced fibrosarcoma in mice (Badary & Gamal El-Din, Citation2001), and benzo[a]pyrene induced for stomach tumorigenesis (Badary et al., Citation1999). However, despite all these promising pharmaceutical activities- especially as anticancer therapeutic- its application is hindered by various factors. Firstly: TQ’s hydrophobicity and hence its poor solubility in aqueous medium cause serious bioavailability issues. Secondly: its extreme lipophilicity which cause poor formulation characteristics. Thirdly: its thermal and light sensitive nature which limit the available suitable approaches that can be used to enhance its bioavailability including nano-formulations.
In order to increase its stability and solubility in aqueous medium -and hence its bioavailability- TQ can be loaded into a carrier capable of delivering it into the cells. In a previous study, Ravindran et al., (Citation2010) succeeded in loading TQ into a poly (lactide-co-glycolide) nanoparticle. Other attractive nanocarriers are liposomes. Liposomes are excellent candidates to act as a solubilizing agent and drug carrier for TQ to increase its bioavailability and uptake by cells.
In this study, we succeeded in TQ loading in a liposome system. TQ-loaded liposomes (TQ-LP) were then characterized and tested on various cell lines to see the effect of loading TQ into a liposome on its anticancer properties.
Materials and methods
Chemicals and reagents
Thymoquinone (2-isopropyl-5-methyl-1,4-benzoquinone) and Triton X-100 were purchased from Sigma (St. Louis, MO, USA). 1,2-dipalmitoyl-sn-glycero-3-phosphocholine (DPPC) was obtained from Avanti Polar Lipids, Inc. (Alabaster, Alabama, USA). Culture media Dulbecco’s Modified Eagle Medium (DMEM) and Dulbecco’s Modified Eagle Medium: Nutrient Mixture F-12 (DMEM-F12), fetal bovine serum (FBS), L- glutamine, penicillin and streptomycin were purchased from Invitrogen (Gibco, Invitrogen, USA). Deuterated chloroform (99.9% atom) and deuterated water (99.9% atom) were used for NMR analysis and were purchased from Aldrich (USA). All reagents and chemicals were used without further treatment.
Cell culture
Breast cancer cell lines (MCF-7 and T47D) were purchased from the American Type Culture Collection (ATCC, Manassas, VA, USA). The cells were grown in DMEM-F12 medium (Gibco, Invitrogen, USA) supplemented with 10% FBS, 100 U/mL penicillin, 100 µg/mL streptomycin and 2 mM glutamine at 37°C in a humidified atmosphere and 5% CO2.
Periodontal ligament fibroblasts cells isolation and culturing
Under sterile conditions in class ІІ microbiological safety cabinet, sample teeth, two fully erupted sound maxillary first molars extracted from an 11-year-old healthy man, was held with a sterile forceps and the middle third of the root surface was mechanically scraped with a number 15 scalpel to obtain samples of periodontal ligament (PDL) tissue. The PDL tissue was diced into small tissue explants of 1 mm3. Thereafter, these tissue explants were placed in 25 cm2 tissue culture flasks. The explants were incubated with DMEM culture medium supplemented with 10% FBS. The 10th–15th passage of periodontal ligament fibroblasts (PLF) cells were used in the experiment.
Liposomes preparation
Two types of liposomes were prepared, DPPC liposomes (LP) and DPPC-Triton X-100 liposomes (XLP). LP liposomes were prepared using the conventional thin-film hydration technique. Briefly, 50 mg of DPPC, 7 mg TQ were dissolved in 3 mL chloroform. Chloroform was then evaporated using IKA RV 05 Basic Rotary Evaporator (Germany) with room temperature water bath combined with vacuum of 30–150 mbar (VacuuBrand CVC2000, Germany), until a dried thin film was formed. The film was re-dissolved in chloroform again and the whole process of evaporation under vacuum was repeated until a very uniform and homogeneous thin film was obtained. Then the film was hydrated with 10 mL deionised water and left for 24 hours at room temperature for complete hydration. To enhance the encapsulation of the drug, the mixture was heated at ~50°C for 20–30 min with vigorous vortexing every 2–3 min.
XLP liposomes were made using the same method described above but using the following quantities: 50 mg DPPC, 2 µL Triton X-100 and 7 mg TQ, which were dissolved in chloroform, then the same steps were followed for LP preparation. These liposomes were prepared to compare their kinetic release of TQ with that of LP liposomes.
Free TQ was separated by extensive dialysis using Cellu•Sep® T1 (Membrane Filtration Products Inc. Seguin, Texas, USA) dialysis membrane (MWCO of 3500 Daltons) against 400 mL of sterile de-ionised distilled water. The dialysis buffer was changed twice.
The prepared liposomes’ size was controlled using both sonication and extrusion techniques. First, the liposomes were sonicated in a water bath sonicator for 30 min, then extruded 15–18 times through a 0.1 µm polycarbonate membrane (Whatman Nuclepore, Richmond, USA) using Avanti Mini-Extruder (Avanti Polar Lipids, Inc. Alabaster, Alabama, USA). The size and ξ-potential of the liposomes was measured using zetatrac (microtrac, Germany). Nuclear magnetic resonance (NMR) analysis was also performed to TQ, LP and TQ-LP using Bruker Avance III 500 mHz instrument (Bruker BioSpin, Switzerland).
Entrapment efficiency
Two methods were used to study the entrapment efficiency of TQ in liposomes using the dialysis solutions, destructed liposomes or both. The first one is UV-Vis spectrophotometry (Cary E1 (100)) using TQ’s λmax at 330 nm. The other technique is HPLC (Shimadzu, Japan), using a varian microsorb 100 C-18 column that was 25 cm long with 5 µm particle size. The HPLC experiments were performed with a mobile phase of acetonitrile:water (80:20) with a flow rate of 1.0 mL/min, λmax of 250 nm, at these conditions the retention time for TQ is 4.2 min. The liposomes were destructed using acetonitrile as follows: 800 µL of acetonitrile were added to 200 µL of the dialyzed liposome and then were transferred into Eppendorf tube, vortexed, sonicated just enough time to have a homogeneous mixture. Then were centrifuged (12,000 rpm for 10 min using high speed vacuum centrifuge SUPRA 30K (Hanil Science Industrial, Korea). Then the supernatant was taken for HPLC or spectrophotometric analysis. The same procedure was performed for empty liposomes to be used as the blank for these measurements.
Scanning electron microscope
A drop of extruded liposomes was dispersed on carbon adhesive tape applied on aluminium stub, then dried completely under fume hood. The dried liposomes were coated with platinum nanoparticles using Emitech K550X (England) sputter coater, and then the scanning electron microscope (SEM) images were obtained using Inspect™ F50 Scanning Electron Microscope (FEI, Netherlands) with voltage of 15 KV.
Transmission electron microscope
The liposomal vesicles were examined by transmission electron microscope (TEM) to characterize their microstructure. Briefly, small drop of liposomes was deposited on Formvar/carbon film coated copper grid of 300 mesh. The grid was then stained with 2% uranyl acetate. The TEM images were taken using Morgagni 268 transmission electron microscope (FEI, Netherlands) operating at 100 KV.
Differential scanning calorimetry
The differential scanning calorimetry (DSC) method was used to determine the change in liposome melting temperature due to the inclusion of TQ, Triton X-100 or both. Liposomes were analyzed on Mettler Toledo DSC823e and Netzsch DSC 204 F1 instrument (Germany) with heating rate of 5°C/min.
Cell proliferation assay
Breast cancer cell lines (T47D, MCF-7), and periodontal ligament fibroblasts cells (PLF) were seeded at a density of 6000 per well in 96-well plates in appropriate medium, allowed to grow for 24 hours, and then treated with increasing concentrations of free TQ and TQ-LP liposomes. Colorimetric celltiter 96 non-radioactive cell proliferation assay (Promega, Madison, USA) was used to detect live cells in each well as per manufacturer’s instructions. Briefly, 15 µL of dye solution (containing tetrazolium) were added on each well. The cells were incubated at 37°C and 5% CO2 for 4 hours. Then, 100 µL of the solubilized solution were added to solublize formazan. The absorbance was recorded at 570 nm with background subtraction at 690 nm, using colorimetric plate reader Sunrise-Basic TECAN (GmbH, Austria). The cellular proliferation was expressed as a percentage of cell viability of TQ treated cells or TQ-LP liposomes treated cells relative to untreated controls.
In vitro TQ release from TQ-LP liposomes
A 400 µL of liposome suspension sample was placed into the cellulose dialysis tubing (MCWO: 3500 Daltons) against 50 mL of sterile de-ionised distilled water in a covered beaker placed in a temperature-controlled water bath (37°C) and stirring rate of 30 rounds per minute. At various time intervals, 1 mL of the dialysis buffer was withdrawn from the beaker and the absorbance at 330 nm was measured to determine the quantity of the TQ released, 1 mL of fresh sterile, de-ionized water was added to the dialysis solution to maintain fixed volume.
Results
Preparation and characterization of TQ-LP liposome
In this study, the conventional thin-film hydration technique was used for the preparation of liposomes loaded with TQ. These liposomes were extruded and characterized using particle sizer, zeta potential, SEM, TEM and NMR. As shown in , SEM and TEM images for extruded TQ-LP. These liposomes have a mean diameter of 100 nm. These results agree well with those of zetatrac measurements of TQ-LP at room temperature were a mean diameter of 122 nm was obtained for extruded TQ-LP and ξ-potential of 22 mV. However, prior extrusion large liposomes were obtained that can be distinguished using optical microscope () and sizing data gave an average diameter of more than 400 nm.
Figure 2. Electron microscopic images for extruded TQ encapsulated liposomes; (A) SEM image (B) TEM image. The average diameter of liposomes is about 100 nm.
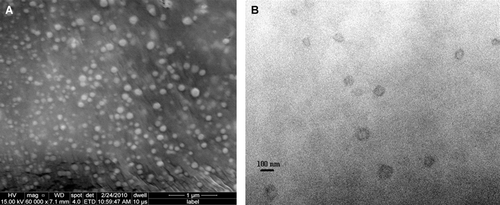
In order to determine the encapsulation efficiency of TQ in liposomes, the absorbance at TQ’s λmax was measured and calibration curves for TQ at that wavelength were prepared. However, the concentration of TQ in the dialysis buffer samples was very small, indicating a very high encapsulation efficiency of more than 90%. In order to ensure that our data were correct, samples of TQ-LP were destructed using acetonitrile (or Triton X-100) and these destructed liposomes were analyzed using HPLC equipped with a UV-Vis detector. Upon liposome destruction, TQ should be easily observed and measured. However, HPLC data showed the presence of very small amounts of free TQ. Also, in vitro release study of TQ-LP resulted in almost no TQ that were released. These findings contradicted with our previous data obtained from UV-Vis spectroscopy measurements of dialysis buffers. On the other hand, TQ-LP liposomes exhibited anti-proliferative activity in vitro on two cancer cell line (MCF-7 and T47D) close to those exhibited by free TQ and much higher than just empty liposomes as will be shown below. In order to better understand the situation, NMR spectroscopy was used to analyze TQ-LP system. Samples of DPPC and TQ were dissolved in deuterated chloroform (CDCl3). In addition, extruded and dried TQ-LP sample was also dissolved in CDCl3 to be compared with free TQ and free lipid. Of course CDCl3 was used since it is a very good solvent for TQ. The 1H-NMR spectra of DPPC and TQ are showed in and , respectively. Analysis of 1H-NMR of DPPC shows the presence of 11 signals assigned as follows: at 0.9 ppm (t, 2CH3); 1.3 ppm (br, nCH2 chains); 1.6 ppm (m, 2CH2); 2.3 ppm (q, 2CH2); 3.4 ppm (s, 3CH3 bonded to N+); 3.8 ppm (t, CH2 bonded to N+); 4.0 ppm (m, CH2 bonded to OCO-chain); 4.35 ppm (t, CH2 bonded to O-P); 4.15 ppm (dd) and 4.45 ppm (dd) (both for CH2 bonded to CH) and at 5.2 ppm (q, CH). The 1H-NMR spectrum of TQ-LP in CDCl3 (which will affect the integrity of the liposome) is shown in . A comparison between the spectrum of DPPC and that of TQ-LP showed the presence of an extra peak at 2.45 ppm (s, integration of 10) which is not found in the 1H-NMR spectra of neither DPPC nor free TQ in CDCl3. From the liposome preparation, almost equimolar amounts of TQ and lipid were used, so a comparison of the integration and assuming that TQ signals in the liposome experienced a shift we can assume that the peak at 2.45 ppm is originated from TQ aliphatic protons (chemical shift range is 1–2 ppm, ) will show almost a complete encapsulation of TQ in the liposome.
The incorporation of Triton X-100 in the liposomes’ formulation have decreased the melting point of DPPC liposomes by 2°C () and affect the transition phase behavior as detected by differential scanning calorimetry (DSC). This is expected since Triton X-100 increases the mobility of the bilayer. This also leads to a decrease in the encapsulation efficiency of TQ in XLP liposomes to a value of 49.6%.
TQ-LP liposomes exhibit anti-proliferative activity in vitro
MTT assays were applied to evaluate the cytotoxicity of TQ-LP liposomes, MTT assays were evaluated after a cell incubation period of 72 hours ( and ). TQ-LP liposomes caused cell death in a dose-dependent manner in breast cancer cell lines MCF-7 and T47D. The 50% effective dose (ED50) was about 75 µM and 200 µM in T47D and MCF-7, respectively. The ED50 for free TQ is 15 µM and 40 µM in T47D and MCF-7, respectively.
TQ-LP liposomes show low toxicity on normal fibroblast
To evaluate the toxicity of TQ-LP, MTT assays were used after the treatment of periodontal ligament fibroblasts cells (PLF). Very low toxicity of TQ-LP was detected as shown in , and the ED50 was about 350 µM compared with ED50 of 85 µM for free TQ.
In vitro release of TQ
As shown in , the in vitro release of TQ from TQ-LP can be described as negligible. This indicates that TQ is strongly attached to the lipid which can be explained by the strong lipophilicity of TQ. This can be seen clearly from NMR spectra for DPPC in and a physical mixture of DPPC and TQ in CDCl3 (). Although we have mixed DPPC with TQ physically and dissolved it in CDCl3, it caused the peak at 3.8 ppm (this peak is assigned to the CH2 bonded to N+) to split, indicating a strong interaction between TQ and DPPC.
Discussion
The liposomal targeting of tumor cells is a promising therapeutic strategy to increase the drug index of the targeted agents, while reducing the side effects. Because of the enhancement of permeability and retention effect (EPR), long circulating liposomes tend to accumulate in the tumor tissue, which is accompanied by sustained drug release in the tissue or engulfment of the liposomes by the tumor cells.
TQ is a potent anticancer drug, but its poor solubility in aqueous medium and relative toxicity affect its bioavailability and administration in human. In this study, we prepared TQ-LP liposome to enhance solubility, decrease toxicity and investigate their ability to inhibit the proliferation of breast cancer cells in vitro. DPPC phospholipids are well characterized and safe lipids that are commonly used in liposomes preparation; and we used it in this study as structural unit of the liposome design. TQ is a small non-polar molecule, so it accumulates in phospholipids bi-layer of DPPC liposome, and increase the hardness of the liposome membrane, which may decrease the uptake of liposome by the cell. The triton X-100 was added to see if it can give more flexibility to liposome membrane; and the DSC analysis showed the ability of triton X-100 to decrease the phase transition temperature of DPPC phospholipids by 2°C (), which makes the membrane more flexible. More interesting the addition of triton X-100 did not provoke an enhancement of drug release, suggesting that triton X-100 (in small ratios) maintain the integrity of liposome.
The anti-proliferative activity of prepared TQ-liposome was tested on breast cancer cells MCF-7 and T47D and compared with free TQ (). Although, the free TQ was more potent than TQ-LP, very low toxicity of TQ-LP was detected on periodontal ligament fibroblasts cells ().
This is the first study that describes the encapsulation of TQ in liposome. The new prepared liposome was highly stable, safe and has anti-proliferative activity on cancer cells in vitro.
Acknowledgments
We would like to thank Haifa Hattab, for her valuable help in sample preparation and TEM imaging. We also thank Wadah Faris for his valuable help in SEM imaging. All thanks are also given to Ayat Bozeya for her tremendous help in HPLC optimization and valuable discussions and suggestions.
Declaration of interest
The authors report no conflict of interest.
References
- Badary OA, Al-Shabanah OA, Nagi MN, Al-Rikabi AC, Elmazar MM. Inhibition of benzo(a)pyrene-induced forestomach carcinogenesis in mice by thymoquinone. Eur J Cancer Prev 1999;8:435–440.
- Badary OA, Gamal El-Din AM. Inhibitory effects of thymoquinone against 20-methylcholanthrene-induced fibrosarcoma tumorigenesis. Cancer Detect Prev 2001;25:362–368.
- Chehl N, Chipitsyna G, Gong Q, Yeo CJ, Arafat HA. Anti-inflammatory effects of the Nigella sativa seed extract, thymoquinone, in pancreatic cancer cells. HPB (Oxford) 2009;11:373–381.
- El-Mahdy MA, Zhu Q, Wang QE, Wani G, Wani AA. Thymoquinone induces apoptosis through activation of caspase-8 and mitochondrial events in p53-null myeloblastic leukemia HL-60 cells. Int J Cancer 2005;117:409–417.
- Gali-Muhtasib H, Diab-Assaf M, Boltze C, Al-Hmaira J, Hartig R, Roessner A et al. Thymoquinone extracted from black seed triggers apoptotic cell death in human colorectal cancer cells via a p53-dependent mechanism. Int J Oncol 2004;25:857–866.
- Kaseb AO, Chinnakannu K, Chen D, Sivanandam A, Tejwani S, Menon M et al. Androgen receptor and E2F-1 targeted thymoquinone therapy for hormone-refractory prostate cancer. Cancer Res 2007;67:7782–7788.
- Mansour MA, Nagi MN, El-Khatib AS, Al-Bekairi AM. Effects of thymoquinone on antioxidant enzyme activities, lipid peroxidation and DT-diaphorase in different tissues of mice: a possible mechanism of action. Cell Biochem Funct 2002;20:143–151.
- Newman DJ, Cragg GM. Natural products as sources of new drugs over the last 25 years. J Nat Prod 2007;70:461–477.
- Ravindran J, Nair HB, Sung B, Prasad S, Tekmal RR, Aggarwal BB. Thymoquinone poly(lactide-co-glycolide) nanoparticles exhibit enhanced antiproliferative, anti-inflammatory, and chemosensitization potential. Biochem Pharmacol 2010;79:1640–1647.
- Shoieb AM, Elgayyar M, Dudrick PS, Bell JL, Tithof PK. In vitro inhibition of growth and induction of apoptosis in cancer cell lines by thymoquinone. Int J Oncol 2003;22:107–113.
- Worthen DR, Ghosheh OA, Crooks PA. The in vitro anti-tumor activity of some crude and purified components of blackseed, Nigella sativa L. Anticancer Res 1998;18:1527–1532.