Abstract
Even though the therapeutic efficacy of numerous antimicrobial drugs has been well established, inefficient delivery can result in an inadequate therapeutic index. Gold nanoparticles have unique physicochemical properties such as large surface area to mass ratio and functionalizable structure. These properties can be applied to facilitate the administration of antimicrobial drugs, thereby overcoming some of the limitations in traditional antimicrobial therapeutics. In this study, gold nanospheres were used as a drug carrier system for gentamicin delivery to Staphylococcal infected foci. Conjugation of gentamicin with gold nanospheres was performed in HEPES buffer. The attachment of gentamicin to gold nanospheres was confirmed by UV/Vis spectroscopy. The HPLC and atomic absorption spectrometer analyses showed that 347 gentamicin molecules were attached to each gold nanosphere. Minimum inhibitory concentration and minimum bactericidal concentration studies showed the enhanced antibacterial effect of gentamicin-gold nanospheres complex in comparison with free gentamicin. The biodistribution study showed the localization of the complex at the site of Staphylococcal infection foci with high sensitivity in mouse model.
Introduction
Bacterial infections continue to be one of the major causes of morbidity and mortality. Staphylococcal infections pose an emerging problem with major economic impact (Rubin et al., Citation1999; Brin et al., Citation2008). The antimicrobial activity of antibiotics against microbes is also severely limited by poor membrane transport ability (Kim & Jones, Citation2004). Thus there is a need of delivery vehicles that allow localized and controlled delivery of antibiotics for preventing microbial infections. Moreover, during infection the bacteria are localized intracellularly making the treatment difficult by antibiotics. On the other hand, there are some difficulties to administer many antimicrobial drugs because of their low water solubility, cytotoxicity to healthy tissues and rapid degradation and clearance in the blood stream (Dini et al., Citation2003; Wang et al., Citation2005).
Many studies have previously demonstrated that nanoparticles such as liposomes, polymeric nanoparticles, solid lipid nanoparticles, dendrimers and particularly metal nanoparticles were able to overcome these issues and facilitate antimicrobial delivery to microbial infection sites (Coates et al., Citation2002; Zhang et al., Citation2010). Gold nanoparticles (GNPs) are used in diagnosis and therapeutics due to their unique properties of small size, large surface area to volume ratio, high reactivity to the living cells, stability over high temperatures and translocation into the cells (El-Ansary & Al-Daihan, Citation2009). Nanoparticles have been found useful in the development of systemic, oral, pulmonary, transdermal and other administration routes to study drug targeting, the enhancement of drug bioavailability and protection of drug bioactivity and stability (Giljohann et al., Citation2010). However, due to their “nano” size, their entry is easily facilitated into various cells posing one of the greatest difficulties in using these nanoparticles for targeted delivery to specific tissues. To obviate this problem, researchers have been conjugated these nanoparticles with various biomolecules and ligands to develop strategies for targeted delivery (Delehanty et al., Citation2010; Petros & Desimone, Citation2010).
The efficacy of GNPs conjugated to several antibiotics has also been the subject of some studies by Grace & Pandian (Citation2007) and Saha et al. (Citation2007) They found that GNPs conjugates were more efficient in inhibiting the growth of Gram-positive and Gram-negative bacteria in comparison with the same dosage of antibiotics used alone. Their results suggest that GNPs can act as an effective drug carrier in a drug delivery system (Grace & Pandian, Citation2007; Selvaraj & Alagar, Citation2007; Pissuwan et al., Citation2010).
Gentamicin sulfate is an aminoglycoside with a wide spectrum of antibacterial activity though important side effects mostly related to nephrotoxicity and ototoxicity restrict its use. Gentamicin is used in the treatment of serious microbial infections. It is a highly soluble drug, but does not cross cell membranes efficiently (Tulkens, Citation1986; Gargani & Pacetti, Citation1998). Gentamicin has three NH2 groups and has strong affinity for binding to GNPs ().
Each GNP surrounded by a number of drug moieties acts as a single group against the microbial organisms (Gargani & Pacetti, Citation1998). The greater antibacterial effect of the GNPs conjugates has been attributed to their ability to bind to and/or penetrate the cell wall and, in doing so they are able to deliver a large number of antibiotic molecules into a highly localized volume (Duncan et al., Citation2010). The current study focuses on conjugation of gentamicin with gold nanospheres and its potential as a drug delivery system.
Materials and methods
Materials
GNPs in the size range of 10–12 nm with spherical shape and gentamicin sulfate were purchased from Sigma Aldrich, St. Louis, MO. HEPES buffer and nutrient agar media were purchased from Merck, Frankfurt, Germany. BALB/c mice (6–7 weeks old and 20–25 g weight) were purchased from Razi Vaccine and Serum Research Institute, Karaj, Iran. Staphylococcus aureus strain ATCC 29213 was obtained from microbial bank of Institut Pasteur, Tehran, Iran.
Conjugation of gentamicin to gold nanospheres
The conjugation of gentamicin molecules to gold nanospheres was carried out according to the method described by Xiaohua Huang (EI-Sayed et al., Citation2006). Basically, the gold nanospheres were diluted in 20 mM HEPES (4-(2-Hydroxyethyl)-1-piperazine-ethanesulfonic acid) buffer to a final concentration with optical density (OD) of 0.8 at 520 nm. Forty microliters of gentamicin (6 mg/ml) were added to 960 µl of the same HEPES buffer (pH: 6) to form 1 ml dilute solution. Then, 10 ml of the prepared gold solution was added dropwise to the dilute solution while being stirred. After stirring for 5 min, the solution was left to react for 20 min. The final solution was centrifuged at 6000 rpm for 30 min and gentamicin-gold nanospheres pellet was redispersed in PBS buffer (pH 7.4) and stored at 4°C.
Characterization of the conjugate
In the first step, the change in the color of gold nanospheres solution, from wine red to purple blue, was monitored after conjugation reaction with gentamicin. Then, UV-Vis absorption spectroscopic measurements were recorded on a single beam UV-Vis spectrometer (Cary 100, Varian, CA) in wavelengths from 200 to 800 nm at the resolution of 1 nm. It was known that gold nanospheres and gentamicin possess the characteristic surface plasmon absorption peaks at 520 and 245 nm, respectively. The broadening and red-shifting of the plasmon absorption peak is expected if the conjugation occurs (Grace & Pandian, Citation2007).
Determination of the number of antibiotic molecules attached per each nanosphere
The number of antibiotics attached per each nanosphere was calculated by dividing the concentration of grafted antibiotics by the number of nanospheres in the solution. The concentration of gentamicin and gold nanospheres at the conjugate was measured by HPLC and atomic absorption spectroscopy, respectively (Hosta-Rigau et al., Citation2010).
Gentamicin concentration
The concentration of grafted gentamicin was determined by interpolation into a calibration curve of the area of the HPLC peak of the solution resulting after centrifugation with an Amicon filter (model 2478, Waters, Arcade, NY) of a known volume of the conjugate at 10 000 rpm for 10 min. Once the gold nanospheres pellet was removed, the free gentamicin was injected into the HPLC system (Hosta-Rigau et al., Citation2010).
Gold nanospheres concentration
The concentration of gold nanospheres was determined by ashing the samples with addition of sulfuric acid at 600–630°C. Then, the ash was dissolved in a mixture of concentrated hydrochloric and nitric acids. The solution was dried by evaporation, then a necessary amount of 0.5 N hydrochloric acid was added and the prepared sample was monitored for gold by an atomic absorption spectrometer (model AA240FS, VARIAN, CA). The concentration of gold nanospheres was determined in comparison with the standard curves (Burygin et al., Citation2009).
Antibacterial activity of gentamicin-gold nanospheres complex
The antibacterial effect of gentamicin-gold nanospheres complex against S. aureus was investigated by the liquid broth dilution method (Burygin et al., Citation2009; Fayaz et al., Citation2011). The cultures were inoculated from fresh colonies on agar plates into a 100 ml Muller Hinton culture medium. The growth of the cultures was allowed until the OD reached 0.2 at 600 nm (OD of 0.2 corresponds to a concentration of 108 CFU/ml of the medium). Subsequently, 2 × 108 CFU culture from the solution was added to the 100 ml of Muller Hinton medium supplemented with different concentrations of GNPs, gentamicin and gentamicin-gold nanospheres complex, whereas medium without GNPs, gentamicin or gentamicin-gold nanospheres complex was used as control. The minimum inhibitory concentration (MIC) and minimum bactericidal concentration (MBC) were determined by measuring the growth rate using OD at 600 nm in regular time intervals.
Biodistribution study
The in vivo behavior of gentamicin-gold nanospheres complex was evaluated in four groups of five BALB/c mice infected with Staphylococcal infection. Firstly, S. aureus was cultured in aerobic condition in nutrient agar medium for 16 h at 37°C. Then, a turbid suspension, containing 2 × 108 CFU/ml of viable S. aureus in 0.4 ml of saline, was injected intramuscularly to the left lateral thigh muscles of the BALB/c mice. The right leg muscles were considered as controls. The gentamicin-gold nanospheres complex was injected via the tail vein. At 10, 20, 30 and 60 min post-injection, each group of mice was sacrificed separately by CO2 gas. The liver, heart, kidneys, large and small intestines, stomach, blood and normal and infected thigh muscles were dissected and weighted. The atomic absorption spectroscopy analysis was performed for the quantitative measurement of the conjugate distribution at ultrastructural anatomical resolution in the infected BALB/c mice (Hainfeld et al., Citation2006). The results were calculated as a percentage of injected doses per gram of tissue (%ID/g).
Results
UV-Vis spectroscopy
The change in color of gold nanospheres solution from wine red to purple blue after conjugation reaction with gentamicin initially approved the production of gentamicin-gold nanospheres complex ().
With the addition of gold nanospheres, the intensity of the absorption band at 520 and 245 nm (pertaining to gold nanospheres and gentamicin moiety, respectively) decreased and was accompanied by a new peak emerging at 640 nm that approved the production of gentamicin-gold nanospheres complex ().
Figure 3. UV-Vis spectra of gentamicin, gold nanospheres and their respective conjugates. A shift in the maximum is representative for the production of gentamicin-gold nanospheres complex.
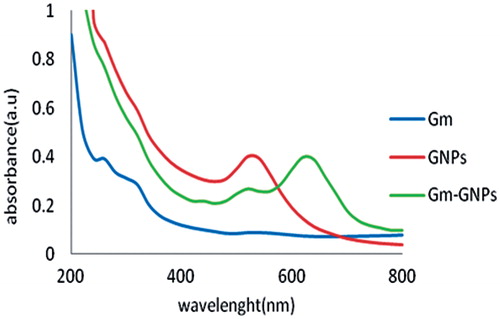
Mechanism of gold nanospheres binding with gentamicin is illustrated in . Gold nanospheres were capped with citrate and the surface of nanoparticles was negatively charged. Gentamicin has three NH2 groups and has strong affinity for binding to gold nanospheres. Gold nanospheres react with gentamicin molecule by ionic interaction between amino group of gentamicin and negative surface charge of gold nanospheres.
Determination of the number of antibiotic molecules attached to each nanosphere
The concentration of gold nanospheres at the conjugate measured by atomic absorption spectroscopy was 81.14 ± 4.2% ().
Table 1. Results of atomic absorption analysis.
HPLC analysis determined that approximately 73.12 ± 2.1% of gentamicin molecules were attached to the gold nanospheres ().
Finally, the calculations indicated that 347 gentamicin molecules were attached to each gold nanosphere.
MIC and MBC of gentamicin-gold nanospheres complex
The results of MIC and MBC of gentamicin-gold nanospheres complex and free gentamicin are shown in . Free GNPs did not show any antimicrobial activity. These findings showed the enhanced antibacterial effect of conjugate in comparison with free gentamicin.
Table 2. The MIC and MBC of gentamicin and a gentamicin-gold nanospheres complex against S. aureus.
Biodistribution study
Tissue distribution of gentamicin-gold nanospheres complex at 10, 20, 30 and 60 min post-injection in the infected BALB/c mice is shown in . The results showed more accumulation of gentamicin-gold nanospheres complex in the site of infection in all the measured times with high sensitivity in comparison with control leg.
Discussion
In this study, gentamicin binding to gold nanospheres was performed by direct method according to the protocol described by Huang et al. with some modifications. The method was simple, rapid with high efficiency. We chose GNPs with spherical shape and 10–12 nm diameter, because they possess a widespread organ distribution which is very relevant for future drug delivery applications (EI-Sayed et al., Citation2006; Hosta-Rigau et al., Citation2010). The conjugation was confirmed using a UV-Vis spectroscopy technique and observation of change in the colloid color from red to purple blue (Grace & Pandian, Citation2007). The results of HPLC and atomic absorption spectroscopy showed a ratio of around 347 gentamicin molecules attached per each gold nanosphere (Hosta-Rigau et al., Citation2010). This result is good in regard to small size of nanoparticles and the spherical shape of our GNPs. The result of liquid broth dilution test showed the enhancement of antibacterial effect of gentamicin conjugated with gold nanospheres in comparison with the free gentamicin. Gold nanospheres can be considered as extraordinary molecular carriers for the targeting, intracellular trafficking and delivery of a huge array of antibiotic molecules to the infection foci (Burygin et al., Citation2009; Fayaz et al., Citation2011). The biodistribution of gentamicin-gold nanospheres complex has also been attributed in this study on BALB/c mice (Hainfeld et al., Citation2006). The results showed the considerable accumulation of gentamicin-gold nanospheres complex in the site of infection in comparison with uninfected muscle in all measured time intervals especially after 30 min post-injection. Gentamicin-gold nanospheres complex was able to achieve good discrimination between normal and infection muscle in mice. This gold retention may be useful for enhanced infection detection and possibly for therapy due to the improved infection: non-infection ratio a few minutes after the injection of the conjugate.
Conclusion
The results suggest that gold nanospheres can act as an effective drug carrier to transport gentamicin to Staphylococcal infected foci.
References
- Brin YS, Golenser J, Mizrahi B, et al (2008). Treatment of osteomyelitis in rats by injection of degradable polymer releasing gentamicin. J Control Release 131:121–7
- Burygin GL, Khlebtsov BN, Shantrokha AN, et al (2009). On the enhanced antibacterial activity of antibiotics mixed with gold nanoparticles. Nanoscale Res Lett 4:794–801
- Coates A, Hu Y, Bax R, Page C, (2002). The future challenges facing the development of new antimicrobial drugs. Nat Rev Drug Discov 1:895–910
- Delehanty JB, Boeneman K, Bradburne CE, et al (2010). Peptides for specific intracellular delivery and targeting of nanoparticles: implications for developing nanoparticle-mediated drug delivery. Medintz Ther Deliv 1:411–33
- Dini E, Alexandridou S, Kiparissides C. (2003). Synthesis and characterization of cross-linked chitosan microspheres for drug delivery applications. J Microencapsul 3:375–85
- Duncan B, Kim C, Rotello VM. (2010). Gold nanoparticle platforms as drug and biomacromolecule delivery systems. J. Control Release 148:122–7
- El-Ansary A, Al-Daihan S. (2009). On the toxicity of therapeutically used nanoparticles: an overview. J Toxicol 2009:1–9
- EI-Sayed IH, Huang X, EI-Sayed MA. (2006). Selective laser photo-thermal therapy of carcinoma using anti-EGFR antibody conjugated gold nanoparticles. Cancer Lett 230:129–35
- Fayaz AM, Girilal M, Mahdy SA, et al (2011). Vancomycin bound biogenic gold nanoparticles: a different perspective for development of anti VRSA agents. Process Biochem 46:636–41
- Gargani G, Pacetti AM. (1998). Sensitivity of 115 strains of the genus Brucella to some antibiotics (cephalosporins, ureidopenicillins and aminoglycosides). Chemioterapia 5:7–13
- Giljohann DA, Seferos DS, Daniel WL, et al (2010). Gold nanoparticles for biology and medicine. Angew Chem Int Ed 49:3280–94
- Grace NA, Pandian K. (2007). Antibacterial efficacy of aminoglycosidic antibiotics protected gold nanoparticles—A brief study. Colloids Surf A Physicochem Eng Asp 297:63–70
- Hainfeld JF, Slatkin DN, Focella TM, Smilowitz HM. (2006). Gold nanoparticles: a new X-ray contrast agent. Br J Radiol 79:248–53
- Hosta-Rigau L, Olmedo I, Arbiol J, et al (2010). Multifunctionalized gold nanoparticles with peptides targeted to gastrin-releasing peptide receptor of a tumor cell line. Bioconjugate Chem 21:1070–8
- Kim HJ, Jones MN. (2004). The delivery of benzyl penicillin to Staphylococcus aureus biofilms by use of liposomes. J. Liposome Res 14:123–39
- Petros RA, DeSimone JM. (2010). Strategies in the design of nanoparticles for therapeutic applications. Nat Rev Drug Discovery 9:615–27
- Pissuwan D, Cortie CH, Valenzuela SM, Cortie MB. (2010). Functionalised gold nanoparticles for controlling pathogenic bacteria. Trends Biotechnol 28:207–13
- Rubin RJ, Harrington CA, Poon A. (1999). The economic impact of S. aureus infection in NY City hospitals. Emerg Infect Dis 5:9–17
- Saha B, Bhattacharya J, Mukherjee A, et al (2007). In vitro structural and functional evaluation of gold nanoparticles conjugated antibiotics. Nanoscale Res Lett 2:614–22
- Selvaraj V, Alagar M. (2007). Analytical detection and biological assay of antileukemic drug 5-fluorouracil using gold nanoparticles as probe. Int J Pharm 337:275–81
- Tulkens PM. (1986). Experimental studies on nephrotoxicity of aminoglycosides at low doses. Mechanisms and perspectives. Am J Med 80:105–14
- Wang LY, Ma GH, Zhi-Guo S. (2005). Preparation of uniform sized chitosan microspheres by membrane emulsification technique and application as a carrier of protein drug. J Control Release 106:62–75
- Zhang L, Pornpattananangkul D, Hu MJ, Huang M. (2010). Development of nanoparticles for antimicrobial drug delivery. Curr Med Chem 17:585–94