Abstract
Context: Gallic acid (GA) has well-documented antioxidant and CNS effects affecting glutathione, catalase and malonaldehyde levels in brain.
Objective: This study was designed to evaluate the anti-amnesic activity of pure GA in scopolamine (SC)-induced amnesic models and to enhance its effects using Tween 80®-coated nanoparticles.
Methods: GA-loaded chitosan nanoparticles (GANP) and corresponding Tween 80®-coated batch (cGANP) were formulated. Amnesia was induced by using SC (0.4 mg/kg, i.v.). GA, GANP, cGANP (dose equivalent to GA 10 mg/kg, i.p.) and positive control Piracetam (400 mg/kg, i.p.) were administered for successive 7 days to male Swiss albino mice. The in vivo pharmacodynamic study was performed using Morris water maze (MWM) and elevated plus maze (EPM) models; locomotor activity using photoactometer and brain acetyl cholinesterase (AChE) activity was also studied.
Key findings: GA-treated mice exhibited significant decrease in transfer latency in the EPM test; increase in time spent in target quadrant in MWM and reduced AChE activity. GA significantly reversed SC-induced amnesic activity. There was no significant change in locomotor activity of the mice by GA and its nanoparticle formulations. These effects were significantly increased by the administration of cGANP compared with pure GA administration but no significant change was observed for GANP.
Conclusion: GA possesses anti-amnesic activity by reversing the SC-induced amnesia which may be attributed to its anti-cholinesterase activity. Tween 80®-coated nanoparticle approach with improved brain targeting may serve as an effective approach to enhance its anti-amnesic effect.
Introduction
Gallic acid (GA) is a natural phenolic compound. Its chemical name is 3,4,5-trihydroxybenzoic acid. Its sources are gallnuts, sumac, witch hazel, grapes, oak bark and green tea. It possess antioxidant, antityrosinase, antimicrobial, anti-inflammatory, anticancer and neuroprotectant activities (Krogh et al., Citation2000; Shahrzad et al., Citation2001; Levites et al., Citation2002; Zhongbing et al., Citation2006; Bastianetto et al., Citation2011; Phiriyawirut & Phaechamud, Citation2012; Nagpal et al., Citation2012b). It possesses antibacterial property against Escherichia coli, Staphylococcus aureus, Pseudomonas aeruginosa and Klebsiella pneumonia (Rodrguez et al., Citation2007). It is well documented that regular consumption of polyphenols may attenuate the accumulation of Aβ peptides and thus neurodegeneration (Klein et al., 2001; Terry, Citation2001; Hardy & Seloke, Citation2002; Bastianetto & Quirion, Citation2010).
Free radicals and other reactive oxygen species (ROS) are generated continuously during normal physiological processes. They are degraded simultaneously by antioxidant defense mechanisms. Under pathological conditions, the ROS generation increases. The generation of ROS is one of the factors that are responsible for impaired cholinergic system that may cause memory disorders (Fukui et al., Citation2002). Because GA had in vivo anti-oxidant effects (Nagpal et al., Citation2012b), its anti-amnesic activity was hypothesized. The study was designed to evaluate the effect of GA in scopolamine (SC)-induced amnesia in mice.
The rationale of developing drug delivery systems is to improve the efficacy of a therapeutically active compound and minimize its toxic side effects. This can be achieved by increasing the amount and duration of drug in the vicinity of the target cells and minimizing its exposure to non-target cells (Nagpal et al., Citation2012b). Blood–brain barrier (BBB) is the major hurdle for delivery of CNS acting drugs. One of the strategies to overcome BBB and enhance the brain uptake of drug is Tween 80®-coated nanoparticulate drug delivery (Nagpal et al., Citation2012a,Citationb, Citation2013a,Citationb). The proposed mechanism is that when polysorbate-coated nanoparticles are administered, apolipoprotein E or B adsorb on the nanoparticle surface. The nanoparticles with covalently bound Apo E are taken up into the cerebral endothelium by an endocytotic mechanism followed by transcytosis across the endothelium with subsequent re-uptake by neurons into brain parenchyma (Zensi et al., Citation2009; Nagpal et al., Citation2013a). Thus, the present study was planned to assess the anti-amnesic activities of GA as well as its nanoparticles in mice by making use of exteroceptive (Morris water maze (MWM) and elevated plus maze (EPM) tests) and interoceptive model (SC-induced amnesia). These models simulate human dementia. Thereafter, anti-cholinesterase activity from brain homogenate was analyzed to study the effect of GA and its nanoparticles on the cholinergic system.
Materials
Chitosan was a gift sample from Central Institute of Fisheries Technology, Kochi, India. GA monohydrate was procured from Hi-Media, Mumbai, India. Other chemicals used during the study were of suitable analytical grade and were used as received.
Young Swiss male albino mice (25–30 g) were obtained from Disease Free Small Animal House, LLRUVAS, Hisar (Haryana), India. The animals were kept in groups of three, in plastic cages with soft bedding, under standard conditions of light and dark cycle and feed was provided ad libitum. All the experiments were carried out between 08:00 am and 04:00 pm. The experimental protocol was approved by Institutional Animals Ethics Committee (IAEC) and animal care was taken as per the guidelines of Committee for the Purpose of Control and Supervision of Experiments on Animals (CPCSEA), Ministry of Environment and Forests, Government of India (Registration no. 0436). Efforts were made throughout to minimize animal discomfort and to use minimum number of animals (n = 6) for statistical significance. All animals were acclimatized to laboratory environment for 7 days before testing.
Methods
Preparation and characterization of chitosan nanoparticles
The chitosan nanoparticles were prepared by the modified ionotropic gelation method (Nagpal et al., Citation2012a,Citationb). The chitosan and tri-polyphosphate pentasodium (TPP) with chitosan to TPP ratio 3:1 were combined electro-statically entrapping the drug (GA) followed by coating with Tween 80®. The uncoated batch was GANP and coated batch was cGANP. All experiments were carried out in triplicate and reported as mean ± SEM. The GANP and cGANP batches were characterized for particle size, size distribution and zeta potential (by dynamic light scattering analysis using ZetaSizer Nano ZS90 (Malvern Instruments Limited, UK)); percent drug entrapment efficiency (% DEE; using UV spectrophotometer (Cary 5000, Instrument version no. 1.12)); electron microscopic examination using transmission electron microscope (Morgagni 268 D, Fei Co., The Netherlands, operated at 60 KV); differential scanning calorimetry (Model No. Q10; TA Instruments Pvt. Ltd., New Castle, DE) and in vitro drug release study of formulated nanoparticles using the equilibrium dialysis membrane (Himedia, MWCO, molecular mass cutoff 12 000–14 000, pore size 2.4 nm) technique at 37 ± 1 °C (Nagpal et al., Citation2012b).
In vivo studies
Animals
Young Swiss male albino mice were housed separately in groups of six per cage (polycarbonate cage size: 29 × 22 × 14 cm) under laboratory conditions with alternating light and dark cycle of 12 h each with free access to food and water. The acclimatized animals were kept fasted 2 h before and 2 h after drug administration.
Drugs and treatment schedule
SC was used to induce amnesia in mice (Chauhan & Chaudhary, Citation2012). Piracetam (PT) was used as positive control (Khan et al., Citation2012). Blank nanoparticles coated with Tween 80® (cNP) were tested to evaluate the effect of polymer and Tween 80® coating on SC-induced amnesia. The cGANP batch and GANP batch were freshly prepared at the beginning of each experiment. The animals were divided in following groups having six mice in each group.
Groups for EPM
Group 1 (control group): Mice were administered normal saline (i.p.) for 7 successive days. Transfer latency (TL) was recorded 45 min after saline administration on sixth day (learning). After 24 h, TL (retention) was examined 45 min after saline treatment.
Group 2 (SC group): Mice in this group were administered normal saline (i.p.) for 6 successive days and TL was recorded on Day 6. After 24 h, SC (0.4 mg/kg, i.p.) was administered and TL was recorded 45 min after the administration of SC.
Group 3 (SC + cNP group): Mice in this group were administered the Tween 80®-coated NP without drug (dummy NP, (i.p.) equivalent to the amount used in Group 8) for 7 successive days. TL was noted 45 min after the administration of dummy nanoparticles on Day 6. After 24 h (Day 7), SC was injected (0.4 mg/kg, i.p.) 45 min after the administration of dummy NP and TL was recorded 45 min after the administration of SC.
Group 4 (GA group): Mice in this group were administered GA (10 mg/kg, i.p.) for 7 successive days. TL was noted 45 min after the administration of pure GA on Day 6. After 24 h (Day 7), TL was recorded 45 min after the administration of GA.
Group 5 (SC + PT): Mice in this group were administered PT (400 mg/kg, i.p.) for 7 successive days. TL was noted 45 min after the administration of PT on Day 6. After 24 h, SC was injected (0.4 mg/kg, i.p.) 45 min after the administration of PT and TL was recorded 45 min after the administration of SC.
Group 6 (SC + GA): Mice in this group were administered GA (10 mg/kg, i.p.) for 7 successive days. TL was noted 45 min after the administration of GA on Day 6. After 24 h, SC was injected (0.4 mg/kg, i.p.) 45 min after the administration of GA and TL was recorded 45 min after the administration of SC.
Group 7 (SC + GANP): Mice in this group were administered GANP (equivalent to 10 mg/kg, i.p.) for 7 successive days. TL was noted 45 min after the administration of GANP on Day 6. After 24 h, SC was injected (0.4 mg/kg, i.p.) 45 min after the administration of GANP and TL was recorded 45 min after the administration of SC.
Group 8 (SC + cGANP): Mice in this group were administered cGANP (equivalent to 10 mg/kg, i.p.) for 7 successive days. TL was noted 45 min after the administration of cGANP on Day 6. After 24 h, SC was injected (0.4 mg/kg, i.p.) 45 min after the administration of cGANP and TL was recorded 45 min after the administration of SC.
Groups for MWM
Groups 9–16: These were same as groups 1–8, except time spent in target quadrant (TSTQ) was measured in MWM.
Groups for biochemical estimations
Groups 17–24: The animals after being tested on MWM were sacrificed and brain acetyl-cholinesterase (AChE) activity was measured.
EPM test
The EPM test is used for the evaluation of learning and memory activity by measuring TL (Itoh et al., 1990; Ojha et al., Citation2010). The EPM (for mice) consisted of two open arms (16 cm × 5 cm) and two covered arms (16 cm × 5 cm × 15 cm) extended from a central platform (5 cm × 5 cm) and the maze was elevated to a height of 25 cm from the floor. On Day 6, each mouse was placed at the end of an open arm, facing away from the central platform. TL is the time taken by the animal to move from the open arm into one of the covered arms with all its four legs. For each animal, TL was recorded on Day 6. In case the animal did not enter into one of the covered arm within 90 s, it was pushed gently into one of the two covered arm and TL of 90 s was assigned. The mouse was then allowed to explore the maze for another 2 min and then returned to its home cage. The treatments (saline/SC/SC + cNP/GA/SC + PT/SC + GA/SC + GANP, SC + cGANP) were administered for 7 successive days. The retention of this learned-task (memory) was examined on Day 7 (as per study design).
MWM test
The MWM model has been used extensively to investigate spatial learning and memory in rodents (Ojha et al., Citation2010). The model uses the sensory information of the animal about its surroundings especially vision and proprioception to measure the spatial reference memory (Ojha et al., Citation2010). Animals after being used on EPM were kept on normal diet and water for 15 days (>>7 half life) as mean elimination half-life of GA is 1.19 ± 0.07 h (Shahrzad et al., Citation2001) and were then used for MWM. The procedure, technique and end point for testing memory were followed with slight modifications in the parameters described by Ojha et al. (Citation2010). Briefly, MWM (for mice) consisted of a circular pool (60 cm in diameter, 25 cm in height) filled to a depth of 20 cm with water (made opaque with non-toxic white-colored dye) maintained at 25 °C. The tank was divided into four equal quadrants (Q1, Q2, Q3, Q4) with the help of two threads, fixed at right angle to each other. A platform painted in white (top surface 6 cm × 6 cm) was placed inside the target quadrants (Q4 in the present investigation) of this pool was submerged 1 cm below the surface of water. The position of platform was kept unchanged throughout the training session. Each animal was subjected to four consecutive trials each day with a gap of 5 min for 4 consecutive days. During trial, they were allowed to escape on to the hidden platform and to remain there for 20 s. During this training session, the mouse was gently placed in the water between quadrants, facing the wall of pool with drop location changing for each trial, and allowed 120 s to locate submerged platform. In case the mouse failed to find the platform within 120 s, it was guided gently toward the platform and allowed to remain there for 20 s. Each animal was subjected to these training trials for 4 consecutive days (Days3–6), the starting position was changed with each exposure as described and target quadrant (Q4 in the present study) remained the same throughout the training period.
Day1 Q1 Q2 Q3 Q4
Day2 Q2 Q3 Q4 Q1
Day3 Q3 Q4 Q1 Q2
Day4 Q4 Q1 Q2 Q3
On Day 7, the dose was administered, and the TSTQ was noted. During the observation, the platform was removed and the animal was placed in any of the three quadrants and allowed to explore the target quadrant for 300 s. The mean time spent in the target quadrant (Q4) in search of the missing platform was noted as index of retrieval or memory. The position of the observer and other objects in the laboratory including relative location of water maze with respect to other objects in the laboratory was same throughout the study.
Measurement of locomotor activity
Alterations of locomotor activity and exploration are used to study specific processes, such as learning, memory reward, anxiety, and to evaluate the influence of drug on general motor activity. To study the effects of various treatments on locomotor activity, all the groups were tested for locomotor activities using Photoactometer (INCO, Ambala, India). The horizontal movement of the mice was recorded for 6 min after various treatments.
Biochemical estimation
Collection of brain samples
Immediately after behavioral study on MWM, the animals were sacrificed by cervical dislocation under mild anesthesia. The whole brain was carefully removed from the skull. For the preparation of brain homogenate, after weighing, the fresh whole brain was transferred to a glass homogenizer and subjected to homogenization in an ice bath after adding 10 volumes of phosphate buffer (pH 8, 0.1 M). The homogenate was centrifuged using refrigerated centrifuge (Remi Instruments, Mumbai, India) at 3000 rpm for 10 min at 4 °C. The cloudy supernatant liquid so obtained was used for the estimation of AChE activity.
Experiment
Brain AChE was estimated using the method as described by Ellman et al. (1961) and Ojha et al. (Citation2010). Brain homogenate (0.4 ml) was added to a test tube containing 2.6 ml of phosphate buffer. Dithiobisnitrobenzoic acid (DTNB) reagent (0.1 ml) was added to the above mixture and its absorbance was noted at 412 nm. Acetylcholine iodide solution (0.02 ml) was then added and after 15 min, the absorbance was noted again. Acetylcholine iodide is hydrolyzed to choline and acetate by AChE. The choline so produced reacts with DTNB reagent to produce a yellow color. The rate of formation of choline from acetylcholine iodide in the presence of tissue cholinesterase was measured using a spectrophotometer. The rate of color development is the measure of the AChE activity. Change in absorbance per minute of the sample was read at 412 nm and the rate of hydrolysis of substrate was calculated (Ojha et al., Citation2010).
Statistical analysis
All in vitro studies data are reported as mean ± SD (n = 3), and the in vivo studies data are reported as mean ± SEM (n = 6). The difference between the groups were tested using the analysis of variance (ANOVA) followed by Tukey's post hoc test for biochemical parameters at the level of p < 0.05. The difference greater than p < 0.05 was considered significant and was calculated using GraphPad Instat 3 (GraphPad Software, Inc. San Diego, CA).
Stability studies
To assess the stability of chitosan nanoparticles encapsulating GA (GANP and cGANP), a stability study was performed (Wilson et al., Citation2010). The samples were packed in borosilicate glass vials and sealed. The vials were stored at four different conditions, i.e. refrigerator (4 ± 1 °C); room temperature (25 ± 5 °C); 37 ± 1 °C (relative humidity, RH = 75%) and at 50 ± 5 °C for 3 months. Samples were observed after 1, 2 and 3 months for drug content as well as their physical appearance. Chemical stability was evaluated by Fourier transform–infrared (FT-IR) studies after 3 months of storage.
Results
Characterization of nanoparticles
The average particle size (hydrodynamic diameter) was observed to be 166.53 ± 4.48 nm and 104.40 ± 1.69 nm for GANP and cGANP, respectively. The zeta potential was 46.50 ± 2.09 mV and 39.67 ± 3.66 mV for GANP and cGANP, respectively. The mean percent DEE was found to be above 90% in both the batches. The in vitro release of nanoparticles study revealed greater release (85.32 ± 3.76%) from GANP batch as compared to cGANP batch (76.77 ± 3.89%) after initial burst release (Nagpal et al., Citation2012b).
In vivo studies
Effect of various treatments on TL of mice in EPM model
The effect of various treatments on TL of mice in EPM has been shown in . GA (10 mg/kg, i.p.) administered for 7 successive days significantly (p < 0.001) decreased TL of mice as compared to the saline-treated control group, showing significant memory enhancing activity of GA. In contrast, SC-treated mice showed a significant increase (p < 0.001) in TL (compared to both saline-treated and pure GA-treated groups) demonstrating that administration of SC induced marked memory impairment (amnesia). Similar decrease in TL was observed in the case of SC + cNP-treated group as compared to the saline-treated control group.
Figure 1. Effect of various treatments on transfer latency in elevated plus maze model. F (7, 40) = 40.834; p < 0.0001; n = 6 in each group. Values are expressed as mean ± SEM. Data was analyzed by one-way ANOVA followed by Tukey’s post hoc test. a = (p < 0.001) significant difference from saline-treated control group. b = (p < 0.001) significant difference from GA-treated group. c = (p < 0.001) significant difference from SC-treated group. d = (p < 0.05) significant difference from SC + GA–treated group. e = (p < 0.001) significant difference from SC + GANP–treated group.
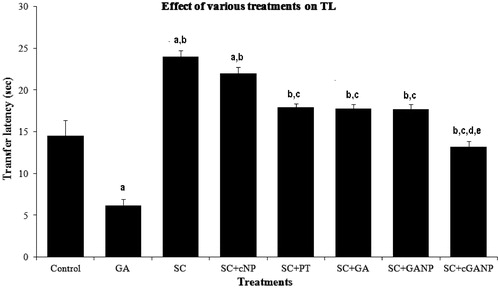
The treatment with PT, GA, GANP and cGANP significantly reversed the increase in TL induced by SC in mice as compared to SC per se. But the effect was more pronounced for cGANP dispersion treatment as compared to pure SC + GA, and SC + GANP-treated groups (p < 0.05).
Effect of various treatments on TSTQ of mice in MWM
The effect of various treatments on TSTQ of mice in MWM is depicted in . GA (10 mg/kg, i.p.) administered for 7 successive days significantly (p < 0.05) increased TSTQ of mice as compared to the saline-treated control group, showing significant memory enhancing activity of GA. In contrast, SC-treated mice showed a significant decrease (p < 0.001) in TSTQ indicating that administration of SC induced marked memory impairment. Decrease in TSTQ was observed in the case of SC + cNP-treated group compared to the saline-treated control group demonstrating the absence of effect of excipients used in formulation on TSTQ. The treatment with PT, GA, GANP and cGANP significantly reversed the SC-induced amnesia decrease in TSTQ as compared to SC per se. However, the reversal of SC-induced amnesic effect was significantly higher for cGANP-treated group as compared to both pure GA and GANP treatments.
Figure 2. Effect of various treatments on time spent in target quadrant in the Morris water maze model. F (7, 40) = 56.053; p < 0.0001; n = 6 in each group. Values are expressed as mean ± SEM. Data was analyzed by one-way ANOVA followed by Tukey's post hoc test. a = (p < 0.001) significant difference from saline-treated control group. b = (p < 0.001) significant difference from GA-treated group. c = (p < 0.001) significant difference from SC-treated group. d = (p < 0.05) significant difference from SC + GA–treated group. e = (p < 0.05) significant difference from SC + GANP–treated group.
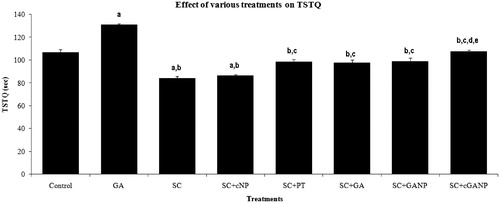
Locomotor activity
GA, SC, SC + cNP, SC + PT, SC + GA, SC + GANP and SC + cGANP administered for 7 successive days did not significantly influence the spontaneous locomotor activity in mice as compared to respective saline-treated groups ().
Biochemical estimations of AChE
The values obtained after the determination of brain AChE activity are shown in . GA (10 mg/kg, i.p.) administered for 7 successive days significantly (p < 0.001) decreased brain AChE of mice as compared to the saline-treated control group, showing significant memory-enhancing activity of GA. SC-treated mice showed a significant increase (p < 0.001) in AChE depicting marked memory impairment by the administration of SC. Decrease in TL was also observed in the case of SC + cNP-treated group as compared to the saline-treated control group indicating no effect of dummy Tween 80®-coated chitosan nanoparticles (excipients) on brain AChE. The treatment of SC-pretreated mice with PT, GA, GANP and cGANP resulted in significantly decreased brain AChE activity when compared to SC per se. But the effect was maximum for cGANP dispersion-treated group and was significantly different from SC + GA– (p < 0.01) and SC + GANP (p < 0.01)-treated groups.
Figure 4. Effect of various treatments on brain acetylcholine esterase. F (7, 40) = 150.060; p < 0.0001; n = 6 in each group. Values are expressed as mean ± SEM. Data was analyzed by one-way ANOVA followed by Tukey’s post hoc test. a = (p < 0.001) significant difference from saline-treated control group. b = (p < 0.001) significant difference from GA-treated group. c = (p < 0.001) significant difference from SC-treated group. d = (p < 0.01) significant difference from SC + GA–treated group. e = (p < 0.01) significant difference from SC + GANP–treated group.
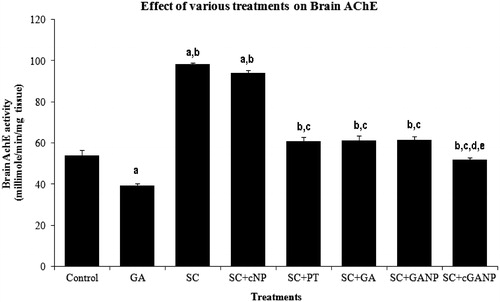
Stability studies
There were no significant change in percent drug content, physical appearance and IR spectra of the nanoparticles (RTNP and cRTNP), indicating that they were stable for 3 months stability study period at 4 ± 1 °C; 25 ± 5 °C; 37 ± 1 °C;RH 75% and 50 ± 5 °C ().
Table 1. Stability study data for GANP and cGANP.
Discussion
The aim of the present investigation was to explore the anti-amnesic activity of GA and to develop a novel, biocompatible and biodegradable Tween 80®-coated brain targeted delivery system for its targeted brain delivery. The selected polymer, chitosan, is completely biodegradable and biocompatible and formulation method for nanoparticles avoids the use of hazardous organic solvents (Nagpal et al., Citation2010). The formulated nanoparticles were in nanometer range and less than 200 nm for effective brain targeting with narrow size distribution (average polydispersity index below 0.3); in vitro drug release above 75% in 24 h (Nagpal et al., Citation2012b).
Learning and memory are two primary cognitive functions that award us the ability to acquire and assimilate information from our experiences (Liu et al., Citation2009). Learning is defined as the acquisition of any new information about the events occurring in surroundings and subsequent retrieval or retention of this information is known as memory (Okano et al., Citation2000). Memory is a complex function of brain and involves multiple neuronal pathways and neurotransmitters. The memory processes involve registration, consolidation and retrieval of information. Impairment of memory is an organic brain disorder defined as the loss of intellectual ability of sufficient severity to interfere either with occupational functioning, usual social activities or with relationship of a person in the absence of gross clouding of consciousness or motor involvement (Sharma et al., Citation2008). Decrease in cholinergic function level in brain (Zanardi et al., Citation2002), increase in oxidative stress (Zhu et al., Citation2007), hypercholesterolemia (Beach, Citation2008) and neuro-inflammations (Aisen, Citation2002) play an etiological role in memory deficits. Most of the currently used therapeutic interventions provide only symptomatic relief and do not halt the progression of memory deficits, and often such strategies are associated with side effects and thus are of limited use. Hence, there is need for new directions in the management of cognition disorders that not only provide relief from symptoms but also stop the progression of memory deficits.
The salient findings of this study indicated that treatment with GA and its nanoparticles for 7 successive days caused a significant improvement in the memory performance parameters, i.e. TL and TSTQ. Simultaneously a fall in brain AChE activity was observed. Anti-amnesic activities were assessed by SC-induced amnesic models. Decrease in TL and increase in TSTQ were taken as the index of enhanced memory in experimental animals (Morris, Citation1984).
SC is a centrally acting anti-muscarinic drug that has been extensively utilized to induce memory deficits in experimental animals. Pre-treatment with GA for 7 successive days prevented memory loss of mice in both amnesic and non-amnesic groups as compared to respective control animals. PT (400 mg/kg, i.p.) injected for 7 successive days significantly reversed the SC-induced amnesia in mice. This finding is confirmed by the earlier reports wherein repeated injections of PT had improved learning abilities and memory capacities of laboratory animals (Canonico et al., Citation1991; Bhattacharya et al., Citation1993; Ojha et al., Citation2010).
In the present investigation, pure GA administration for 7 successive days showed memory-enhancing activity in mice as evidenced by decrease in TL using EPM and increase in TSTQ using MWM. SC (0.4 mg/kg, i.p.) successfully induced amnesia in mice as shown by increase in TL in EPM and decrease in TSTQ in MWM. GA, GANP and cGANP reversed the amnesia induced by SC. But the effect was more pronounced in the case of cGANP dispersion ( and ), which may be attributed to efficient influx of GA by Tween 80®-coated nanoparticles. Such Tween 80®-coated nanoparticulate system has also improved the anti-depressant-like activity of GA (Nagpal et al., Citation2012b).
Acetylcholine is considered the most important neurotransmitter involved in the regulation of cognitive functions. Cognitive dysfunction has been shown to be associated with impaired cholinergic transmission and the facilitation of central cholinergic transmission with improved memory. Moreover, selective loss of cholinergic neurons in certain brain parts appeared to be a characteristic feature of senile dementia (Watanabe et al., Citation2009). AChE degrades acetylcholine. Excessive AChE activity may lead to deficiency of acetylcholine and memory impairment. Thus, facilitation of cholinergic pathways can be an important strategy in improving learning and memory. Administration of GA, GANP and cGANP may also prove to be beneficial for reversing SC-induced amnesia, owing to the AChE inhibitory property shown by GA, GANP and cGANP in the present study.
In the present investigation, an extremely significant decrease in brain AChE activity has been observed after the administration of GA as compared to the saline-treated control group for 7 successive days. This decrease in AChE activity would lead to increase in brain levels of acetylcholine. This result suggests that one of the reasons of the memory-enhancing effect may be due to lowering of AChE activity. The administration of SC significantly reduced brain AChE as compared to the saline-treated control group indicating the amnesic effect of SC. This decrease in AChE was reversed by the administration of positive control (PT), pure GA, GANP as well as cGANP as compared to the SC-treated group. No significant difference was observed in the performance of pure GA and GANP in terms of TL, TSTQ and brain AChE activity. cGANP-treated mice showed minimum brain AChE activity, minimum TL in EPM and maximum TSTQ in MWM. Thus, anti-amnesic effect of GA was significantly improved by the administration of Tween 80®-coated nanoparticles encapsulating GA (cGANP).
Conclusion
GA reversed the SC-induced amnesia in mice which may be attributed to its antioxidant properties and improved cholinergic functions. The increase in this effect by the administration of Tween 80®-coated nanoparticles may be due to increased uptake of GA through Tween 80®-coated nanoparticulate drug delivery system. No significant change in pharmacodynamic as well as biochemical parameter was observed in the case of GANP without coating.
Declaration of interest
The authors are grateful to Haryana State Counseling society for providing CV Raman Scholarship as financial assistance to Ms Kalpana Nagpal. There is no conflict of interest among the authors.
References
- Aisen PS. (2002). The potential of anti-inflammatory drugs for the treatment of Alzheimer’s disease. Lancet Neurol 1:279–84
- Bastianetto S, Dumont Y, Quirion R. (2011). Catechins and resveratrol as protective polyphenols against beta-amyloid-induced toxicity: possible significance to Alzheimer’s disease. In: Ramassamy C, Bastianetto S, eds. Recent advances on nutrition and the prevention of Alzheimer’s disease. Trivandrum, Kerala, India: Transworld Research Network Inc., 145–54
- Bastianetto S, Quirion R. (2010). Heme oxygenase 1: another possible target to explain the neuroprotective action of resveratrol, a multifaceted nutrient-based molecule. Exp Neurol 225:237–9
- Beach T. (2008). Physiologic origins of age-related b-amyloid deposition. Neurodegener Dis 5:143–5
- Bhattacharya SK, Upadhyay SN, Jaiswal AK. (1993). Effect of piracetam on electroshock induced amnesia and decrease in brain acetylcholine in rats. Indian J Exp Biol 31:822–4
- Canonico PL, Aronica E, Aleppo G, et al. (1991). Repeated injections of piracetam improve spatial learning and increase the stimulation of inositol phospholipid hydrolysis by excitatory amino acids in aged rats. Funct Neurol 6:107–11
- Chauhan B, Chaudhary AK. (2012). Memory enhancing activity of methanolic extract of Pterocarpus marsupium Roxb. Phytopharmacology 2:72–80
- Ellman GL, Courtney KD, Andres V Jr, Feather-Stone RM. (1961). A new and rapid colorimetric determination of acetylcholinesterase activity. Biochem Pharmacol 7:88–95
- Fukui K, Omoi N-O, Hayasaka T, et al. (2002). Cognitive impairment of rats caused by oxidative stress and aging, and its prevention by vitamin E. Ann N Y Acad Sci 959:275–84
- Hardy J, Seloke DJ. (2002). The amyloid hypothesis of Alzheimer’s disease: progress and problems on the road to therapeutics. Science 297:353–6
- Itoh J, Nabeshima T, Kameyama T. (1990). Utility of an elevated plus-maze for the evaluation of memory in mice: effects of nootropics, SC and electroconvulsive shock. Psychopharmacology (Berl) 101:27–33
- Khan D, Mubashir M, Une HD, et al. (2012). Nootropic activity of n-butanolic fraction of methanolic extract of leaves of Ziziphus mauritiana Lam. in mice. Der Pharmacia Sinica 3:193–98
- Klein WL, Krafft GA, Finch CE. (2001). Targeting small Abeta oligomers: the solution to an Alzheimer's disease conundrum? Trends Neurosci 24:219–24
- Krogh R, Yunes RA, Andricopulo AD. (2000). Structure–activity relationships for the analgesic activity of gallic acid derivatives. Farmaco 55:730–5
- Levites Y, Amit T, Youdim MB, Mandel S. (2002). Involvement of protein kinase C activation and cell survival/cell cycle genes in green tea polyphenol (–)-epigallocatechin 3-gallate neuroprotective action. J Biol Chem 277:30574–80
- Liu L, van Groen T, Kadish I, Tollefsbol TO. (2009). DNA methylation impacts on learning and memory in aging. Neurobiol Aging 30:549–60
- Morris R. (1984). Developments of a water-maze procedure for studying spatial learning in the rat. J Neurosci Methods 11:47–60
- Nagpal K, Singh SK, Mishra DN. (2010). Chitosan nanoparticles: a promising system in novel drug delivery. Chem Pharm Bull (Tokyo) 58:1423–30
- Nagpal K, Singh SK, Mishra DN. (2012a). Evaluation of anti-anxiety activity of nanoparticle mediated delivery of minocycline HCl. Inventi Rapid: NDDS 2012:1–4
- Nagpal K, Singh SK, Mishra DN. (2012b). Nanoparticle mediated brain targeted delivery of gallic acid: in vivo behavioral and biochemical studies for improved antioxidant and antidepressant-like activity. Drug Deliv 19:378–91
- Nagpal K, Singh SK, Mishra DN. (2013a). Drug targeting to brain: a systematic approach to study the factors, parameters and approaches for prediction of permeability of drugs across BBB. Expert Opin Drug Deliv (in press). DOI: 10.1517/17425247.2013.762354
- Nagpal K, Singh SK, Mishra DN. (2013b). Formulation, optimization, in vivo pharmacokinetic, behavioral and biochemical estimations of minocycline loaded chitosan nanoparticles for enhanced brain uptake. Chem Pharm Bull 6l:l–16
- Ojha R, Sahu AN, Muruganandam AV, et al. (2010). Asparagus recemosus enhances memory and protects against amnesia in rodent models. Brain Cogn 74:1–9
- Okano H, Hirano T, Balaban E. (2000). Learning and memory. Proc Natl Acad Sci USA 97:12403–4
- Phiriyawirut M, Phaechamud T. (2012). Gallic acid-loaded cellulose acetate electrospun nanofibers: thermal properties, mechanical properties, and drug release behavior. Open J Polym Chem 2:21–9
- Rodrguez VMJ, Alberto MR, de Nadra MMC. (2007). Antibacterial effect of phenolic compounds from different wines. Food Control 18:93–101
- Shahrzad S, Aoyagi K, Winter A, et al. (2001). Pharmacokinetics of gallic acid and its relative bioavailability from tea in healthy humans. J Nutr 131:1207–10
- Sharma B, Singh N, Singh M. (2008). Modulation of celecoxib- and streptozotocin-induced experimental dementia of Alzheimer's disease by pitavastatin and donepezil. J Psychopharmacol 22:162–71
- Terry RD. (2001). An honorable compromise regarding amyloid in Alzheimer disease. Ann Neurol 49:684
- Watanabe T, Yamagata N, Takasaki K, et al. (2009). Decreased acetylcholine release is correlated to memory impairment in the Tg2576 transgenic mouse model of Alzheimer’s disease. Brain Res 1249:222–8
- Wilson B, Samanta MK, Santhi K, et al. (2010). Chitosan NPs as a new delivery system for the anti-Alzheimer drug tacrine. Nanomed: Nanotech Bio Med 6:144–52
- Zanardi A, Leo G, Biagini G, Zoli M. (2002). Nicotine and neurodegeneration in ageing. Toxicol Lett 127:207–15
- Zensi A, Begley D, Pontikis C, et al. (2009). Albumin nanoparticles targeted with Apo E enter the CNS by transcytosis and are delivered to neurons. J Control Release 137:78–86
- Zhongbing L, Guangjun N, Belton PS, et al. (2006). Structure–activity relationship analysis of anti-oxidant ability and neuroprotective effect of gallic acid derivatives. Neurochem Int 48:263–74
- Zhu X, Smith MA, Honda K, et al. (2007). Vascular oxidative stress in Alzheimer disease. J Neurol Sci 257:240–6