Abstract
Objective: This study aims at testing the hypothesis that reversed phase evaporation liposomes (REVs) are suitable for systemic delivery of an anti-osteporotic drug (risedronate sodium (RS)) via pulmonary nebulization.
Materials and methods: RS REVs were prepared using phospholipids and cholesterol with or without stearylamine, and were characterized for morphology, entrapment efficiency (EE%), in vitro release, particle size and aerosolization behavior from an actively vibrating mesh nebulizer. RS accumulation in rat bones following intra-tracheal administration of the selected formulation was assessed using a radiolabelling-based technique, and histological examination of rat lung tissue was performed to assess its safety.
Results: The EE% of RS REVs ranged from 8.8% to 58.96% depending on cholesterol molar ratio, phospholipid type and presence of stearylamine. RS REVs’ particle size ranged from 2.15 to 3.61 µm and were spherical and moderately polydisperse. Nebulization of the selected formulation showed an aerosol output of 85%, a fine particle fraction of 70.75% and a predicted alveolar deposition of 30.39%. The amount of radiolabelled RS deposited in rat bones after pulmonary administration was 20%, while being considerably safe on lung tissues.
Conclusion: Cationic REVs is a promising carrier for systemic delivery of RS for treatment of bone resorptive diseases.
Introduction
Pulmonary administration of drugs for systemic delivery represents a significant opportunity for many drug classes, in particular, for those with poor oral bioavailability. Compared to oral delivery, this route of administration eliminates the potential for poor absorption and/or high metabolism in the gastrointestinal tract and liver, and compared to injection therapy, it is not associated with pain and as a consequence, this should improve patient comfort and compliance, leading to enhanced treatment outcome (Liu et al., Citation2008). Among those drug classes is the popular “bisphosphonates” (BPs). Several studies suggest that the simplified dosage regimens of BPs together with the reduced frequency of their administration are important factors for improving adherence to therapy and avoiding the reduction of anti-fracture efficacy (Miller, Citation2005). Currently, dosing guidelines oblige the patients to take BPs in fasting conditions and to maintain an upright posture for at least 30 min after oral administration. These guidelines are recommended to reduce the gastro-esophageal injuries induced by this class. However, the application of the aforementioned guidelines is not always sufficient to prevent side effects, in particular, when BPs are administered with relatively high frequency (Blumentals et al., Citation2009). In addition, the high polarity and hydrophilicity of this class of drugs cause their extremely low bioavailability levels (less than 1% upon their oral intake) (Ezra & Golomb, Citation2000). Slow release of BPs is also required since it was reported that rapid injection of BPs can lead to renal failure due to the formation of complexes with calcium in the blood, which are then held back in the kidney (Ezra & Golomb, Citation2000).
In light of the above, a sustained release carrier-based delivery system for BPs, delivered through a route of administration other than the oral route, such as the pulmonary route presented here in the current work, would be an advantage, to achieve better and sustained delivery of RS to bones and thereby reduce the side effects of the drug. The liposomal and polymeric systems have been reported as the most common drug carriers for pulmonary delivery. In the current work, liposomal systems were chosen owing to their inherent biocompatibility; being based on endogenous phospholipids (Gaspar et al., Citation2010). Furthermore, liposomal formulations were also reported to exhibit a satisfactory pulmonary aerosolization behavior when combined with the appropriate nebulizer type such as active vibrating mesh nebulizers (Gaspar et al., Citation2010). Risedronate sodium (RS) was chosen as a representative drug of BPs, owing to its reported high anti-resorptive action (Dunford et al., Citation2001, Citation2008). Therefore, the aim of the current work was to investigate the potential of using nebulizable liposomal carrier system for RS to achieve systemic delivery through the pulmonary route.
Materials and methods
Materials
RS was a gift from SPIC Pharma Company, Chennai, India. Dipalmitoylglycerophosphatidylcholine (DPPC) was kindly gifted by Corden Pharma, Liestal, Switzerland. l-α-Phosphatidyl choline, type X-E: from dried egg yolk (PC), cholesterol (CH), stearylamine (SA), phosphate buffered saline tablets (PBS), sodium pentobarbital and chloramine-T were purchased from Sigma Chemical Co., St. Louis, MO. Uranyl acetate -2- hydrate was purchased from Allied Signal, Riedel-dahaen, Germany. Spectra/Por dialysis membrane, 12 000–14 000 molecular weight cut off was purchased from Spectrum Laboratories Inc., Canada. K125I was purchased from H1121 Budapest, Konkoly-Thege Miklòs ùt of radionuclidic purity >99%. All other chemicals were obtained as reagent-grade products.
Preparation of RS liposomes using reversed phase evaporation technique
Risedronate-loaded liposomes were prepared using the reversed phase evaporation technique (REV) (Hathout et al., Citation2007). The lipid components were dissolved in chloroform: methanol mixture (2:1, v/v), followed by evaporation of the organic solvent mixture using a rotary evaporator (Buchi, R-114, Switzerland) at 60 °C, allowing the formation of a thin lipid film. The film was re-dissolved in 12 ml diethyl ether, followed by the addition of 6 ml phosphate buffered saline pH 7.4 containing 25 mg RS. The system was sonicated for 2 min (Crest Ultrasonics, model 275 T, Trenton, NJ), swirled by hand, and re-sonicated again for another 2 min. The formed emulsion was then placed on the rotary evaporator and the organic solvent was removed under reduced pressure to fully remove the organic solvent. The resultant liposomal suspension was left to equilibrate at room temperature, followed by the addition of 4 ml phosphate buffered saline, and storage at 4 °C for overnight maturation. The composition of the prepared liposomal formulations is demonstrated in .
Table 1. Composition and properties of different RS REVs.
Characterization of the prepared RS REVs
Determination of RS entrapment efficiency in REV liposomes
The free RS was separated from the liposomal entrapped counterpart by centrifugation at 15 000 rpm for 1 h at 4 °C. The supernatant was analyzed for the un-entrapped RS using HPLC method as reported by Jia et al. (Citation2006), using Agilent Eclipse column XDB-C18 (5 µm, 4.6 × 150 mm). The mobile phase consisted of buffer (5 mM tetrabutylammonium bromide as ion-pair reagent, 11 mM sodium phosphate and 1.5 mM EDTA-2Na, adjusted to pH 6.75) – methanol in a ratio of 88:12, pumped at a flow rate of 1 ml/min. Samples of 100 µl were injected and the detection wavelength was 262 nm. Entrapment efficiency (EE%) was calculated according to the following equation (Equation (1)):
Determination of the morphology of RS REVs using transmission electron microscopy (TEM)
The selected RS REV formulation was negatively stained by placing a drop of liposomal suspension on a carbon – coated grid, followed by removal of the excess liquid using a filter paper (Nasr et al., Citation2008). A drop of 2% (w/v) uranil acetate aqueous solution was placed on the grid and examined by transmission electron microscope (JEM-100 S, Joel, Tokyo, Japan).
Particle size analysis
The volume mean diameter size of RS REVs was determined using laser diffraction (Malvern Mastersizer, Worcestershire, UK). The SPAN index, indicating the polydispersity of the liposomal formulations was calculated according to the following equation:
where D0.9, D0.1 and D0.5 represent the particles diameters determined at the 90th, 10th and 50th percentile, respectively.
In vitro RS release from REVs
The in vitro release of RS was tested using a franz diffusion cell (Variomag, Telesystem, H + P Labortechnik, Germany) with a diffusion area of 1.77 cm2 fitted with a cellulose acetate membrane (Nasr et al., Citation2013). Soaking of the cellophane membrane in distilled water was done in advance for 4 h. The receptor compartment consisted of 7.5 ml phosphate buffered saline pH 7.4 at 37 °C, which was constantly stirred at 150 rpm with a small magnetic bar. An amount of RS REVs equivalent to 1 mg RS were placed on the membrane and dispersed in 200 µl PBS in the donor compartment. At selected time intervals (0.5, 1, 2, 3, 4, 6, 8 and 24 h), an aliquot of 500 µl of the buffer were sampled from the receptor compartment, with the consequent replacement with an equal volume of fresh receptor solution. The release of 1 mg RS solution was performed for comparison. The quantity of RS released was determined using UV spectrophotometry at 262 nm. The in vitro drug release data were fitted using zero, first, Higuchi diffusion. Moreover, fitting using Korsmeyer–Peppas equation was performed as well according to the following equation:
where Mt and M∞ corresponds to the amount of drug released at time t and ∞, respectively, k is the kinetic constant, t is the release time and n is the diffusional component for drug release.
Determination of nebulization behavior using laser diffraction
The aerosol droplet size of the selected RS REV formulation was determined using laser diffraction, owing to the reported correlation of the in vitro results with the in vivo lung deposition (Clark, Citation1995). The size of the aerosol droplets of the selected liposomal formulation was determined using a Malvern Spraytec laser diffraction size analyzer (Malvern Instruments, Worcestershire, UK). Three milliliters of RS REV selected formulation were loaded into an active vibrating mesh nebulizer (Aeroneb Pro, Aerogen, Ireland), which was consequently placed perpendicular to the laser lens line of the instrument at a distance of 3 cm from the laser beam (Nasr et al., Citation2012). A vacuum of 60 l/min was applied (Model HCP5, Copley Instruments, Nottingham, UK), to draw the aerosol through the beam for particle size analysis, and the volume median diameter (VMD) were recorded from the charts. The size distribution (represented by SPAN index) was calculated as previously stated in Equation (2). The aerosol output, fine particle fraction (FPF) and the predicted alveolar deposition were calculated using the following equations, respectively:
In vivo determination of bone deposition of RS REV selected formulation using radiolabeling technique
Radio-iodination labeling technique using K125I
RS was dissolved in double distilled water at a concentration of (50 mg/2 ml) and the pH was adjusted to 9 using phosphate buffer in a screw capped reaction vial. Chloramine-T solution which was freshly prepared (50 µg/5µl) was added to RS solution followed by the incorporation of 3.5 mCi K125I at 25 °C. After 1 h, the reaction was quenched through the reduction of remaining chloramine-T using (150 µg/5 µl) Na2S2O5 (El Ghany et al., Citation2005). The oxidation by chloramine-T proceeds according to the following equation (Equation (7)) (El Ghany et al., Citation2005). The labeled compound was investigated using both electrophoretic and thin layer chromatographic analysis.
Calculating the reaction yield using electrophoretic and thin layer chromatographic analysis
Electrophoresis was performed using cellulose acetate strips of 45 cm in length (E.C. 3000p-series 90, St. Petersburg, FL). Cathode poles, anode poles, and application points were indicated on the strips and moistened with buffer solution (0.05 M phosphate buffer pH 7). The strips were introduced in the electrophoresis chamber after the reaction mixture samples were set on the strips (10 µl) and applied at a distance of 10 cm from the cathode. A current of 300 V was applied for 1.5 h (El Ghany et al., Citation2005). The developed strips were dried and cut into 1 cm segments, followed by consequent counting by a well-type NaI scintillation counter. The radiochemical yield was calculated from the ratio of the radioactivity of the labeled product to the total radioactivity according to the following equation:
In addition, the radiochemical yield of 125I-RS was confirmed using thin layer chromatography (TLC), in which silica gel 60 supported on aluminum sheets (20 × 2 cm2) were activated by heating at 110 °C for 2 h. Volumes of 5 µl were placed 2 cm apart from the base of TLC, then developed using mobile phase chloroform:ethanol:ammonia (90:10:0.5) with ascending technique. The strips were dried under inert N2 and cut into 1 cm segments then assayed for their radioactivity. The radiochemical yield of I125 labeled RS was determined as stated in Equation (7) and the retention factor was calculated according to the following equation:
In vivo determination of the amount of bone deposited 125I-RS
Quantitative animal biodistribution studies involve the administration of a radionuclide-labeled drug to an animal, most commonly a small rodent such as the rat or mouse. After a prescribed time interval has elapsed, the animal is sacrificed for tissue sampling and target organs are weighed and counted in a gamma counting device. The sample masses and counts, after correction for background and decay, are used to determine the biodistribution of the drug (Maddalena, Citation1983). Small rodents, such as rats are commonly used as in vivo whole animal models for pulmonary route, since relatively small amounts of test material are required for their pulmonary dosing (Sakagami, Citation2006).
The bone deposition experiment was carried out on two groups of male albino rats of six rats each, weighing 250–300 g. The animals were fed a normal diet and water, and kept in a constant temperature environment of 25 °C. Rats were anesthetized using intra-peritonial injection of sodium pentobarbital (32 mg/kg), followed by intra-tracheal instillation of the selected 125I-RS REV formulation (L7) equivalent to a dose of 1 mg 125I-RS (group I) or intravenously injected an amount equivalent to 1 mg 125I-RS solution in the tail vein (group II). In case of group I, an orotracheal tube was pushed against the soft plate to enter the trachea following the vocal cord. When definite bumping against a tracheal cartilage ring was detected, the tube was inserted almost to the bottom of the trachea. The procedure was validated in our laboratory for the reproducibility of the delivery of the test material to the lung. Both groups were sacrificed 24 h post dosing using diethyl ether, with samples of fresh blood taken immediately before killing the rats. The bones and thyroid glands were dissected and placed in pre-weighed counting vials, the radioactivity was counted using the scintillation counter, after correction of background activity. Radioactivity was expressed as a percent of the inhaled or injected activity, in which the percentage of injected/instilled dose (% I.D.) was calculated according to the following equation:
where C2 is the sample counts per unit time, W2 is the organ mass (g), W1 is sample mass (g), S is the counts per unit time of the injected/instilled dose. C2 and S are expressed as counts per minute. Bone and blood were assumed to be 12% and 7% of the total body weight, respectively (Maddalena, Citation1983; Lammers et al., Citation2005; Sakagami, Citation2006).
The animal procedures conducted in the current study were approved by the Ethical Committee of the Faculty of Pharmacy Ain Shams University, in accordance with the guidelines of the Experiments and Advanced Pharmaceutical Research Unit (EAPRU), and were complying with the principles of laboratory animal care.
Histopathology of lung tissue
In order to detect the pathological changes that may occur to the lungs due to administration of RS REVs, histological staining of the lungs was performed. Autopsy lung samples were taken 24 h post dosing from two different groups of rats, group I was administered phosphate buffered saline intra-tracheally as a negative control, and group II was administered the selected RS REV formulation (L7) in an amount equivalent to 1 mg. Autopsy samples were fixed in 10% formol saline for 24 h, followed by washing and dehydration using alcohol. Specimens were cleared in xylene and embedded in paraffin at 56 °C in hot air oven for 24 h, followed by sectioning as paraffin bees wax tissue blocks at 4 μm by slidge microtome (Rotary Leica RM2245, USA). The specimens were deparaffinized and stained by hematoxylin and eosin stains for histopathological examination using light microscopy (Axiostar plus, Zeiss, New York, NY).
Statistical analysis
Results were expressed as the mean ± standard deviation in triplicate. One-way analysis of variance (ANOVA) followed by Tukey–Kramer test were performed using GraphPad InStat® software (Graphpad instat, La Jolla, CA) to check the significance of differences, in which a statistically significant difference was reported if the p-value was less than or equal to 0.05.
Results
Determination of RS EE into REVs
The EE values for different RS REVs are reported in . As obvious from the results, the EE% ranged from 8.8% to 58.96%, depending on the molar ratio of the phospholipid type and cholesterol, and whether or not stearylamine has been included. In case of REVs prepared using egg phosphatidylcholine, the increase in cholesterol molar ratio from 7:4 to 7:6 caused a significant increase in EE% of RS in case of the neutral REVs (from 8.8% to 37.51%), and an insignificant change in EE% in cationic REVs (p < 0.05). On the other hand, in case of REVs prepared using DPPC, the increase in cholesterol molar ratio caused a significant decrease in RS EE% whether the liposomes were neutral or cationic (p < 0.05). The presence of stearylamine significantly increased the EE% of RS in REVs prepared using either egg phosphatidylcholine or DPPC (p < 0.05).
Transmission electron microscopy
demonstrate the morphology of the selected RS REVs formula L7 using TEM at 5000× and 50 000×, respectively. As obvious, liposomes showed regular unilamellar spherical shape.
Particle size analysis
As evident in , the particle size of the prepared RS REVs were within the range of 2.15–3.61 µm, with a SPAN index ranging from 0.98 to 1.63 indicating moderate and acceptable polydispersity.
In vitro drug release from REVs
As demonstrated in , the cumulative amount of RS released from REVs ranged from 18.76 to 42.13%, and was sustained till 24 h. The increase in cholesterol significantly increased the cumulative amount released after 24 h in case of egg phosphatidylcholine REVs. Nevertheless, its presence caused the contrary in case of DPPC REVs (p < 0.05).
Figure 2. Cumulative amount of RS released from REVs prepared using (a) l-α-phosphatidylcholine, (b) DPPC.
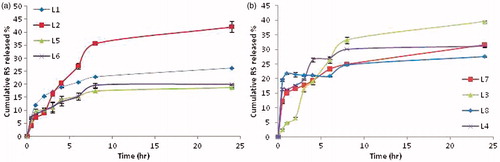
Cationic REVs exhibited significantly lower amount released than their neutral counterparts which concurs with the EE% results (p < 0.05). The cumulative amount released of RS after 24 h from DPPC REVs was significantly lower than those compared to the amount released from the egg PC counterpart (p < 0.05), with occurrence of a relatively more burst effect for RS associated with DPPC REVs compared to egg PC REVs.
Upon kinetic fitting of release data to first order, zero order, Higuchi diffusion models, all formulae were shown to exhibit a diffusion controlled release. Upon further fitting to Korsmeyer–Peppas equation, formulae L1-L3 exhibited anomalous diffusion pattern, while formulae L4-L8 exhibited Fickian diffusion pattern (Supplementary data).
Nebulization experiment
The aerosolization behavior of the selected formulation L7 was tested using an actively vibrating mesh nebulizer. The aerosol output was found to be 85% ± 0.23, and the VMD of the aerosol droplets was found to be 3.69 µm ± 0.19. The FPF% and predicted alveolar deposition percentage were calculated to be 70.75% ± 1.98 and 30.39 ± 5.04, respectively.
Pulmonary absorption and bone deposition of RS
The radiolabeling technique attempted in the current work allowed the detection and calculation of the amount of RS in the bones, blood and thyroid gland. The percentages of RS deposited in the thyroid gland, bones and blood after intra-tracheal administration of formula L7 were calculated to be 0.02% ± 0.02, 20.88% ± 0.05 and 14.52% ± 1.10, respectively.
Histology
As evident in , the stained rat lung tissues showed no histopathological changes after 24 h of administration of RS REVs formula L7 compared to the PBS control. There was no cellular infiltration or tissue fibrosis, with alveolar walls being intact and displaying normal morphology in both groups.
Discussion
The current work tested the hypothesis that an anti-osteoporotic drug (RS) can be delivered through an alternative route of administration to oral route to achieve systemic bioavailability, and consequently, bone deposition. As described in the work, RS liposomes were prepared and characterized for the potential of pulmonary delivery of RS.
The EE% of RS in REVs was found to be influenced by two major factors; the stearylamine presence as well as an interaction factor between phospholipid type and cholesterol molar ratio. Stearylamine exhibited an electrostatic attraction with the drug anion, accounting for the higher EE of cationic REVs when compared to neutral REVs, where such interaction would not be possible (Hathout et al., Citation2007; Bai et al., Citation2009). In fact, as obvious from the results, the inclusion of the positive charge inducer was the most prominent factor dictating the significant increase in EE% of RS in REVs (p < 0.05). Since the increase in the molar ratio of cholesterol elevated the EE% when using egg phosphatidylcholine and decreased the EE% when using DPPC, it was obvious that the interaction between cholesterol and the phospholipid type, rather than the amount of cholesterol or the phospholipid type on their own, affected the EE% of RS in REVs. Cholesterol is known to form different associations with phospholipids in different stochiometric ratios, this association may either be caused by the hydrogen bond between the beta-OH of cholesterol and the glycerol ester oxygen of the phospholipid molecule or to Van der Waal forces in the hydrocarbon region of the bilayers (Presti & Chan, Citation1982; Presti et al., Citation1982). It is also worth noting that the significant higher EE% of the formula L2 compared to the formula L1 may be attributed to the relatively larger particle size associated with formula L2 allowing for more internal aqueous core volume that represents the host place for a hydrophilic drug such as RS in the prepared REVs. In the current case, increasing the cholesterol molar ratio while using the saturated DPPC, which is known to form rigid membranes, caused a displacement of RS sodium, owing to the increased disorder of the acyl chains leading to the decreased EE%, while increasing the cholesterol molar ratio with egg phosphatidylcholine, which is known to form rather fluid membranes enhanced the rigidity and the order of the REVs leading to an increase in the EE% of RS. The highest EE% was obtained with formula L7 which was prepared using DPPC, cholesterol and stearylamine at a molar ratio of 7:4:1, and hence, it was selected for further studies.
The significantly lower cumulative amount released of RS from DPPC REVs compared to egg PC REVs may be ascribed to the difference of the fluidity of the liposomal membranes when placed at a temperature of 37 °C. This temperature is above the transition temperature of the former lipid (the fluid egg PC), and below the transition temperature of the latter one (the synthetic DPPC). The higher amount of cholesterol (a hydrophobic moiety that increases the partitioning of a hydrophilic drug viz. RS outside the liposomes) together with the high fluidity associated with the egg PC REVs caused the significant increase in the release of RS from formula L2.
The relatively higher burst effect for RS, which is associated with DPPC REVs compared to egg PC REVs might be ascribed to one of two reasons; firstly, the used egg PC contains oleic acid and linoleic acid fatty acid residues (Sigma-Aldrich Product Information Sheet), and it has been proven that unsaturation increases the surfactants HLB and polarity (Hathout et al., Citation2010; Vrignaud et al., Citation2012), a case that would allow more affinity for the investigated drug and the subsequent retardation of its release. Secondly, the decrease in water permeability in lipid-based membranes was also correlated with the area occupied by the lipid used (Mathai et al., Citation2008); DPPC was reported to have smaller lipid area than EPC (Petrache et al., Citation1998), further leading to increased water permeability in its liposomal-based membranes compared to the EPC-based counterparts leading to the relative DPPC-associated burst effect that is observed during release.
Cationic REVs exhibited significantly lower amount of RS released than their neutral counterparts which concurred with the EE% results owing to the electrostatic attraction between the negatively charged RS and the positively charged stearylamine (Nasr et al., Citation2008).
From the EE% and release experiments, formula L7 was selected for nebulization and in vivo studies. The Aeroneb Pro nebulizer (actively vibrating mesh nebulizer) was chosen for nebulization of our liposomal formulation since it was previously reported that this nebulizer is particularly suited for the pulmonary delivery of liposomal formulations (Gaspar et al., Citation2010). The aerosol output of formula L7 was found to be considerably high and the VMD of the aerosol droplets was found to be less than 5 µm, which suggests the deep lung deposition potential of RS REVs aerosol. The FPF%, representing the fraction of the aerosol expected to reach the deep lung was also high (70.75%), and half of which was predicted to deposit in the alveoli, allowing the systemic absorption of RS.
In order to directly measure the amount of RS deposited in the bones to exhibit the antiresorptive action, a radiolabelling technique was developed based on the fact that RS is known to selectively bind to the hydroxyapatite crystals in the bones, accounting for its high osteotropic and anti-resorptive nature. Optimization of the radiolabelling technique was done in order to achieve a high reaction yield (>90%). The utilized chloramine T concentration was the optimum concentration to be used, since below this concentration, the chloramine T was insufficient to oxidize all iodide ions to iodonuim ions, and free iodide was detected majorly in the reaction mixture.
The percentages of RS deposited in the three key organs (thyroid gland, bones and blood) after intra-tracheal administration of formula L7 were calculated to be 0.02% ± 0.02, 20.88% ± 0.05 and 14.52% ± 1.10, respectively, which correlated with the cumulative amount of RS released in vitro after 24 h (31.65% ± 0.66). It has been demonstrated in several studies that if 125I was found in the free form, it would be concentrated in the thyroid gland in about 30% concentration (El Ghany et al., Citation2005). Therefore, the low values of radioactivity detected in the thyroid gland suggest the in vivo stability of radiolabeled RS. The presence of residual activity in the blood after intra-tracheal instillation by 24 h of formula L7 may be ascribed to the sustained release nature of the administered formula or to the binding of the labeled RS to the plasma proteins, owing to its complete ionization at the physiological pH (Milner et al., Citation2004). It is still unclear whether BPs bind directly to albumin or to calcium, which in turn binds to albumin. The amount of RS deposited in the bone after 24 h of administration of liposomal formula L7, being 20.88%, was found to be significantly higher (p < 0.05) than the amount deposited from RS after 24 h when administered via a polymeric delivery system (PLGA porous microspheres) in a similar work conducted in our lab (Nasr et al., Citation2011). This clearly demonstrates the superiority of REVs for systemic delivery of RS through the pulmonary route.
Conclusions
The current study is considered the first to demonstrate that a drug belonging to the bisphosphonates class (risderonate sodium) can be successfully delivered to the systemic circulation and deposited in the bones after pulmonary administration in a liposomal form.
Declaration of interest
The authors declare no conflicts of interest.
Supplementary Material
Download PDF (7.9 KB)Acknowledgements
The authors would like to thank Corden Pharma, Switzerland and SPIC pharma, India for their kind supply of DPPC and RS, respectively.
References
- Bai S, Gupta V, Ahsan F. (2009). Cationic liposomes as carriers for aerosolized formulations of an anionic drug: safety and efficacy study. Eur J Pharm Sci 38:165–71
- Blumentals WA, Harris ST, Cole RE, et al. (2009). Risk of severe gastrointestinal events in women treated with monthly ibandronate or weekly alendronate and risedronate. Ann Pharmacother 43:577–85
- Clark AR. (1995). The use of laser diffraction for the evaluation of the aerosol clouds generated by medical nebulizers. Int J Pharm 115:69–78
- Dunford JE, Kwaasi AA, Rogers MJ, et al. (2008). Structure-activity relationships among the nitrogen containing bisphosphonates in clinical use and other analogues: time-dependent inhibition of human farnesyl pyrophosphate synthase. J Med Chem 51:2187–95
- Dunford JE, Thompson K, Coxon FP, et al. (2001). Structure-activity relationships for inhibition of farnesyl diphosphate synthase in vitro and inhibition of bone resportion in vivo by nitrogen-containing bisphosphonates. J Pharmacol Exp Ther 296:235–42
- El-Ghany EA, Amine AM, EL-Sayed AS, et al. (2005). Radiochemical and biological characteristics of radioactive iodine labeled indomethacin for imaging of inflammation. J Radioanal Nucl Chem 266:117–24
- Ezra A, Golomb G. (2000). Administration routes and delivery systems of bisphosphonates for the treatment of bone resorption. Adv Drug Deliv Rev 42:175–95
- Gaspar MM, Gobbo O, Ehrhardt C. (2010). Generation of liposome aerosols with the Aeroneb Pro and the AeroProbe nebulizers. J Liposome Res 20:55–61
- Hathout RM, Mansour S, Mortada ND, Guinedi AS. (2007). Liposomes as an ocular delivery system for acetazolamide: in vitro and in vivo studies. AAPS PharmSciTech 8:1
- Hathout RM, Woodman TJ, Mansour S, et al. (2010). Microemulsion formulations for the transdermal delivery of testosterone. Eur J Pharm Sci 40:188–96
- Jia HJ, Li W, Zhao K. (2006). Determination of risedronate in rat plasma samples by ion-pair high-performance liquid chromatography with UV detector. Analytica Chimica Acta 562:171–5
- Lammers T, Kühnlein R, Kissel M, et al. (2005). Effect of physicochemical modification on the biodistribution and tumor accumulation of HPMA copolymers. J Contr Rel 110:103–18
- Liu X-B, Ye J-X, Quan L-H, et al. (2008). Pulmonary delivery of scutellarin solution and mucoadhesive particles in rats. Eur J Pharm Biopharm 70:845–52
- Maddalena DJ. (1983). TISCON, a basic computer program for the calculation of the biodistribution of radionuclide-labelled drugs in rats and mice. Lucas Heights, Australia: Australian Atomic Energy Commission
- Mathai JC, Trsitram-Nagle S, Nagle JF, Zeidel ML. (2008). Structural determinants of water permeability through the lipid membrane. J Gen Physiol 131:69–76
- Miller PD. (2005). Optimizing the management of postmenopausal osteoporosis with bisphosphonates: the emerging role of intermittent therapy. Clin Ther 27:361–76
- Milner RJ, Farese J, Henry CJ, et al. (2004). Bisphosphonates and cancer. J Vet Intern Med 18:597–604
- Nasr M, Awad GAS, Mansour S, et al. (2011). Different modalities of NaCl osmogen in biodegradable microspheres for bone deposition of risedronate sodium by alveolar targeting. Eur J Pharm Biopharm 79:601–11
- Nasr M, Awad GAS, Mansour S, et al. (2013). Hydrophilic versus hydrophobic porogens for engineering of poly(lactide-co-glycolide) microparticles containing risedronate sodium. Pharm Dev Technol 18:1078–88
- Nasr M, Mansour S, Mortada ND, Elshamy AA. (2008). Vesicular aceclofenac systems: a comparative study between liposomes and niosomes. J Microencapsulation 25:499–512
- Nasr M, Nawaz S, Elhissi A. (2012). Amphotericin B lipid nanoemulsion aerosols for targeting peripheral respiratory airways via nebulization. Int J Pharm 436:611–16
- Petrache HI, Tristram-Nagle S, Nagle JF. (1998). Fluid phase structure of EPC and DMPC bilayers. Chem Phys Lipids 95:83–94
- Presti FT, Chan SI. (1982). Cholesterol-phospholipid interaction in membranes. 1. Cholestane spin-label studies of the phase behavior of cholesterol-phospholipid liposomes. Biochemistry 21:3821–30
- Presti FT, Pace RJ, Chan SI. (1982). Cholesterol-phospholipid interaction in membranes. 2. Stoichiometry and molecular packing of cholesterol-rich domains. Biochemistry 21:3831–5
- Sakagami M. (2006). In vivo. in vitro and ex vivo models to assess pulmonary absorption and disposition of inhaled therapeutics for systemic delivery. Adv Drug Deliv Rev 58:1030–60
- Vrignaud S, Hureaux J, Wack S, et al. (2012). Design, optimization and in vitro evaluation of reverse-micelle loaded lipid nanocarriers containing erlotinib hydrochloride. Int J Pharm 436:194–200