Abstract
Non-ionic surfactant vesicles, simply known as niosomes are synthetic vesicles with potential technological applications. Niosomes have the same potential advantages of phospholipid vesicles (liposomes) of being able to accommodate both water soluble and lipid soluble drug molecules control their release and as such serve as versatile drug delivery devices of numerous applications. Additionally, niosomes can be considered as more economically, chemically, and occasionally physically stable alternatives to liposomes. Niosomes can be fabricated using simple methods of preparations and from widely used surfactants in pharmaceutical technology. Many reports have discussed niosomes in terms of physicochemical properties and their applications as drug delivery systems. In this report, a brief and simplified summary of different theories of self-assembly will be given. Furthermore manufacturing methods, physical characterization techniques, bilayer membrane additives, unconventional niosomes (discomes, proniosomes, elastic and polyhedral niosomes), their recent applications as drug delivery systems, limitations and directions for future research will be discussed.
Introduction
Niosomes
Niosomes are non-ionic surfactant-based vesicles. They were originally developed as an alternative controlled drug delivery system to liposomes, in order to overcome the problems associated with sterilization, large-scale production and stability (Azmin et al., Citation1985, Citation1986; El Maghraby & Williams, Citation2009).
The hydration of a film, comprising a mixture of a single or double-alkyl chain and cholesterol, leads to the formation of vesicular dispersion. These dispersions were termed niosomes (Baillie et al., Citation1985). Basically, these vesicles do not form spontaneously. Thermodynamically stable vesicles form only in the presence of proper mixtures of surfactants and a membrane stabilizing agent (cholesterol), at a temperature above the gel/liquid transition of the main lipid forming vesicles (Azmin et al., Citation1985, Citation1986; Sahin, 2007).
The first niosome formulations were developed and patented by L’Oreal in 1975 (Sahin, 2007). Niosomes were first utilized in drug delivery for anticancer drugs (Azmin et al., Citation1985, Citation1986). The developed niosome formulations were capable of altering the pharmacokinetic profile, organ distribution and metabolism of methotrexate in mice (Azmin et al., Citation1985, Citation1986). Niosomes are versatile in structure, morphology and size; they can entrap hydrophilic drugs in aqueous compartments or lipophilic drugs by partitioning of these molecules into bilayer domains ().
Furthermore, they can be formulated as unilamellar, oligolamellar or multilamellar vesicles (). Niosomes also possess good physical stability, are cost-effective, and are relatively straight forward for routine and large-scale production (Baillie et al., Citation1985; Uchegbu & Florence, Citation1995; Uchegbu & Vyas, Citation1998).
Figure 2. Major types of niosomes, MLV (multilamellar vesicles), OLV (oligolamellar vesicles) and ULV (unilamellar vesicles). Small circles (o) represent polar head group, sticks (–) represents apolar tails of single-chain surfactant molecules and a bilayer membrane represent a circular double surfactant molecules layer oriented in continuous tails-to-tails and polar heads lining the inner and outer circle.
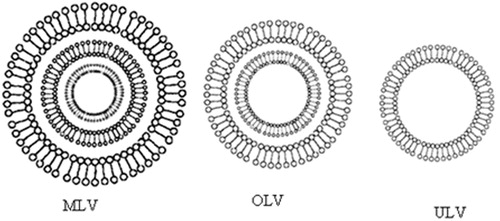
Self-assembly of amphiphilic molecules
The principle of opposing forces
Formerly, the self-assembly of amphiphilic (surfactants and polar lipid) molecules, composed of hydrophilic and lipophilic portions, in an aqueous environment into well-defined structures, such as micelles, open bilayers and vesicles, was solely ascribed to favorable hydrophobic and hydrophilic interactions in the aggregated phase (Tanford, Citation1973). The major forces derive from the hydrophobic interactions between the hydrocarbon tails, which induces the molecules to associate; and the hydrophilic nature of the head group, which imposes the opposite requirement, that they remain in contact with water (the theory of opposing forces) (Tanford, Citation1973). These two interactions compete giving rise to the idea of two “opposing forces” acting mainly in the interfacial region: one tends to decrease and the other tends to increase the interfacial area per molecule (the head group area) exposed to the aqueous phase. While this notion is true and can be accepted thermodynamically, it does not explain all the possible structures into which such molecules can aggregate and form. For instance, some form micelles (e.g. lysolecithin), bilayers (e.g. lecithin), while others form oinverted (reverse) micelless or precipitate out of solution (Israelachvili et al., Citation1980).
The principle of geometrical or packing properties
The role of molecular geometry or “packing” of amphiphiles (surfactants) was first described by (Israelachvili et al., Citation1976). Accordingly, an optimal polar head group area ao, optimal volume of the hydrocarbon chains v and a maximum effective length that the hydrocarbon chains can assume (critical chain length) lc will determine the self-assembly of the amphiphiles into organized aggregates ().
Figure 3. Schematic of a single-chain surfactant, ao = hydrophilic head group area, v = hydrophobic chain volume, lc = hydrophobic chain length.
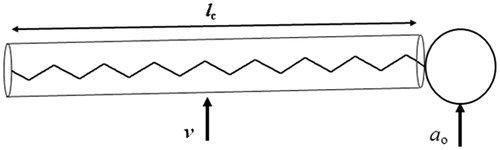
The value of the dimensionless “packing parameter” v/ao lc will determine whether a certain amphiphile or will form spherical micelles (v/ao lc < 1/3); non-spherical micelles (1/3 < v/ao lc < 1/2); bilayers (1/2 < v/ao lc < 1); an inverted micellar structure (v/ao lc > 1); or even precipitate out of solution (e.g. cholesterol), since cholesterol molecules possess a head-group too small to pack into organized structures (Israelachvili & Mitchell, Citation1975; Israelachvili et al., Citation1976; Israelachvili et al., Citation1980). A summary of how surfactant’s geometrical packing parameter affects their aggregation in aqueous medium is shown in .
Table 1. Geometrical characteristic of surfactants and proposed various surfactant aggregates. Bulky head groups’ surfactants form micelles, cone-shaped surfactants form inverted micelles and cylindrical-shaped surfactants form bilayers.
Apart from the selection of amphiphiles with proper geometrical properties, the formation of niosomes involves input of energy (physical agitation and heat), as closed vesicles do not form spontaneously (Baillie et al., Citation1985; Sahin, Citation2006).
In pharmaceutical research, the hydrophilic-lipophilic balance (HLB) system, which is a measure of the relative contributions of the hydrophilic and lipophilic regions of the surfactant molecules, is more commonly used as an indicator on potential niosomes formation. Surfactants possessing HLB values of 4 to 8 have been found to be favorable for the formation of niosomes (Girigoswami et al., Citation2006). On the other hand, surfactants of high HLB values (9.6–16.7) such as polysorbates (Tween®) tend to form micelles or open lamellar structures (Manosroi et al., Citation2003). Some surfactants such as sorbitan monooleate (Span 80, HLB of 4.3) cannot assemble into niosomes (on their own) due to their inadequate geometry, hence packing properties. The oleate moiety of this surfactant molecule has a double bond (with relatively high electron density) at the C9 which repels adjacent hydrocarbon chains resulting in the characteristic “kink” in the structure p (Yoshioka & Florence, Citation1994; Abdelkader et al., Citation2010).
Materials used in the manufacturing of niosomes
Niosome forming amphiphiles
Unlike naturally occurring phospholipids (the principal liposome-forming lipids) which have double alkyl chains, most widely used niosome forming amphiphiles are single chain, synthetic, non-ionic surfactants (Florence & Baillie, Citation1989; Uchegbu & Florence, Citation1995). Alkyl ethers, alkyl esters and alkyl amides, as well as fatty acid and amino acid compounds, can form vesicles (Uchegbu & Florence, Citation1995). Recently, niosomes comprising Pluronic L64 and P105 (copolymers of ethylene oxide and propylene oxides) have been prepared and used as a transdermal delivery system for sulfadiazine sodium (a model hydrophilic drug) (Muzzalupo et al., Citation2011). However, both alkyl ethers and alkyl esters have a wider application as niosomes drug delivery carriers, due to their commercial availability and low toxicity.
Alkyl ethers
Alkyl ethers can be divided broadly into two subclasses, based on their hydrophilic head group: alkyl ethers possessing head groups of glycerol subunits or larger sugar molecules, and alkyl ethers whose hydrophilic head groups consist of repeated ethylene oxide subunits (Uchegbu & Florence, Citation1995; Rajera et al., Citation2011).
Alkyl glycerol ethers, most notably hexadecyl diglycerol ether (C16G2), have been reported as niosome-forming surfactants and have been used in many drug delivery applications such as experimental cancer chemotherapy (Baillie et al., Citation1985; Uchegbu & Florence, Citation1995). These surfactants were used to alter the pharmacokinetics of methotrexate (Azmin et al., Citation1985) and doxorubicin (Uchegbu et al., Citation1996). Alkyl glycerol ethers were also used to encapsulate sodium stibogluconate to control leishmaniasis (Baillie et al., Citation1986).
The second group of alkyl ether surfactnats, in which the hydrophilic region consists of repeated oxyethylene units (Brij®), was used to encapsulate insulin for oral delivery, in order to prevent the inactivation of insulin by the gastric juice (Pardakhty et al., Citation2007). However, Brij 72- and Brij 52-based niosomes have been reported to show physical instability and technical problems due to rapid creaming and floating of aggregated vesicles rather than sedimentation during ultracentrifugation, respectively (Abdelkader et al., Citation2010; Abdelkader, Citation2012).
Alkyl esters
Alkyl esters, such as sorbitan fatty acid esters (Span®) and polyoxyethylene sorbitan fatty acid esters (Tween®), are widely used in cosmetics and foodstuffs, as well as oral, parenteral and topical pharmaceutical formulations. They are regarded as non-toxic and non-irritant materials (Lawrence, Citation2003). These surfactants have been utilized in manufacturing niosomes as a drug delivery system, including oral (Varshosaz et al., Citation2003), trandermal (Ibrahim et al., Citation2005; Balakrishnana et al., Citation2009; Muzzalupo et al., Citation2011) and ocular delivery (Aggarwal et al., Citation2004; Kaur et al., Citation2008; Abdelbary & El-gendy, Citation2008). Cell culture toxicity studies have shown that niosomes composed of ester-type surfactants are less toxic than ether-type ones. This could be attributed to the possible enzymatic degradation of ester bonds (Hofland et al., Citation1992).
Cholesterol (bilayer membrane stabilizer)
Some water-soluble surfactants, such as Tween 20 and Tween 61, can not form vesicles under normal conditions; these surfactants prefer to form micelles, in a manner identical to lyolecithin (Florence & Baillie, Citation1989; Manosroi et al., Citation2003). These surfactants might form vesicles when mixed with cholesterol. Cholesterol molecules operate cooperatively to form bilayer vesicles. Cholesterol is considered as lipid, but it is a comparatively rigid molecule and lacks the accommodating ability of an acyl-chained lipid. In particular, cholesterol does not form bilayers and its shape can be represented as an inverted cone () (Israelachvili et al., Citation1980). However, cholesterol has been called the “mortar” of bilayers because, by virtue of its molecular shape and solubility properties, it fills in empty spaces among the amphiphiles, thereby anchoring them more strongly into the bilayer structure.
Cholesterol in niosomes is reported to increase membrane stability, decrease the fluidity of the membrane and alter membrane permeability (Uchegbu & Florence, Citation1995; Uchegbu & Vyas, Citation1998; Abdelkader et al., Citation2010). Other excipients, such as dicetyl phosphate and stearyl amine, were used in niosomes formulation to impart negative and positive charges, respectively (Yoshioka & Florence, Citation1994; Abdelbary & El-gendy, Citation2008).
Bilayer membrane additives
Bilayer membrane additives can be defined as lipid excipients which are unable to form surfactant vesicles (liposomes and niosomes) by their own but can be accommodated by the bilayer domains (). Membrane additives have been found in surfactant vesicles basically for two reasons; either to alter the bio-distribution, or to improve the physical properties. Non-ionic surfactants can form niosomes in absence of other membrane additives under specified conditions. However, such niosomes have been found to be highly permeable and leaky to the solutes. Cholesterol is the most common membrane additive found in surfactant vesicles; it can significantly alter membrane permeability, fluidity, vesicle stability, entrapment efficiency and niosomes size and is widely known as a membrane stabilizer. Other membrane additives can be broadly classified into charge inducers and water-soluble surfactants. outlines structure and some physical properties for commonly used membrane additives used in niosome formulations (Abdelkader, Citation2012).
Figure 5. Hypothetical positions occupied by DCP (A), Solulan (B) and sodium cholate (C) in the bilayer membrane of Span 60 niosomes stabilised by cholesterol (Abdelkader et al., 2011).
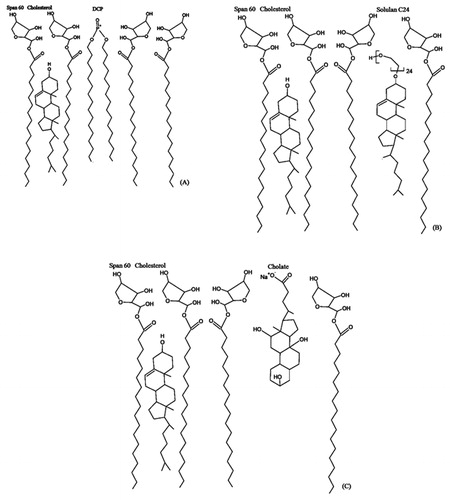
Table 2. Chemical structure, molecular weight, CMC and phase transition temperature of ionic and surfactant additives.
Charge inducers
Stearyl amine and dicetyl phosphate are positive and negative charge inducers respectively, and are frequently incorporated in the bilayer membranes. These charge inducers have been mainly utilized to improve the physical stability of the vesicular dispersions against aggregation (Uchegbu & Vyas, Citation1998); to prolong the half life of circulating surfactant vesicles in plasma (Maurer et al., Citation2007); and to prolong the ocular residence time of topically instilled ophthalmic surfactant vesicles (Kaur et al., Citation2004).
For example, incorporation of positively charged lipids such as stearyl amine is essential for successful delivery of negatively charged polynucleotides and efficient transfection into the cells (Maurer et al., Citation2007). Also, incorporation of dicetyl phosphate in niosomes significantly improved gentamicin sulfate entrapment efficiency in the prepared niosomes for ocular delivery compared with the neutral niosomal formulations (Abdelbary & El-gendy, Citation2008). Negatively charged niosomes have been also found most effective in prolonging gentamicin release rate (Abdelbary & El-gendy, Citation2008) and better permeation through excised bovine corneas (Abdelkader et al., Citation2011).
On the other hand, introduction of water soluble surfactants into vesicles’ bilayer membranes produces liposomes and niosomes with numerous applications.
Water soluble surfactants
Liposomes
The addition of surfactant molecules to vesicular dispersions of phospholipids leads to breakdown or solubilization of the liposomes and formation of a mixed micellar systems (Elsayed & Cevc, Citation2011). Such vesicle-to-micelle transition of phosphatidylcholine liposomes has been achieved by the addition of non-ionic surfactants of the polyoxyethylene cetyl ether class (Kim & Kim, Citation1991). In such a system lamellar mesophases and mixed micelles coexist. In another study, sodium cholate was found to induce a vesicle to micelle transition in cholate-phosphatidylcholine systems (Walter et al., Citation1991). On increasing the bile salt concentration, more multilamellar vesicles were detected. In addition, “open” vesicles, large (twenty to several hundred nanometres in diameter) bilayer sheets, and long (150–300 nm) flexible cylindrical vesicles were also detected (Walter et al., Citation1991).
Furthermore, new classes of liposomes, called Transferosomes®, have been developed upon mixing phospholipids with some water-soluble surfactants, such as sodium cholate and Tween 80. Transferosomes® have the same morphology as liposomes, but they have ultradeformable bilayer membranes. These non-conventional liposomes have been reported to penetrate intact skin when applied non-occlusively. To prepare these vesicles, surfactants such as sodium cholate and sodium deoxycholate have been incorporated in the vesicular membrane, in a concentration range from 20–50% mol/mol (Planas et al., Citation1992; Cevc et al., Citation1996; Elsayed & Cevc, Citation2011; Shamma & Elsayed, Citation2013).
Niosomes
Niosomes derived from non-ionic surfactants of low aqueous solubility are analogues of liposomes (Florence & Baillie, Citation1989; Arora et al., Citation2012). Niosomes are similar in many respects to liposomes, being prepared in the same way forming unilamellar or multilamellar structures which entrap lipid-soluble or water-soluble solutes (Uchegbu et al., Citation1992; Rajera et al., Citation2011). The incorporation of poly-24-oxyethylene cholesteryl ether (Solulan C24) into niosomes has been found to generate atypical niosomal structures such as polyhedral niosomes and discomes (Uchegbu & Florence, Citation1995; Uchegbu & Vyas, Citation1998; Abdelkader et al., Citation2012b).
Polyhedral niosomes
Polyhedral niosomes first obtained from surfactant systems are non-uniform spherical vesicles; they have between 4 to 12 straight edges of similar length (Uchegbu et al., Citation1997). Typically, the reported polyhedral niosomes are formed from mixtures of hexadecyl diglycerol ether (C16G2) and Solulan C24, and could also be formed from C16G2, Solulan C24 and minimum amount of cholesterol. These polyhedral vesicles have been found to be stable for at least 36 d and able to entrap and release slowly water-soluble markers, such as carboxyfluorescein, and nucleotides (Uchegbu & Florence, Citation1995; Uchegbu et al., Citation1997; Uchegbu & Vyas, Citation1998).
Luteinizing hormone releasing hormone (LHRH)-loaded polyhedral and spherical niosomes were prepared as a slow release depot system for intramuscular administration. Spherical conventional niosomes showed more stable membranes than polyhedral ones, suggesting that the conventional niosomes are more effective intramuscular depots (Arunothayanun et al., Citation1999).
Giant niosomes (discomes)
Large disc-like niosomes (discomes) of 11–60 µm in size () were formed upon incubation of the preformed conventional (spherical) niosome dispersion with various proportions of Solulan C24 in a shaking water bath at a temperature of 74 °C for 1 h (Uchegbu et al., Citation1992). Larger discomes were formed with higher Solulan C24 levels and vice versa. A C16G2: cholesterol molar ratio of 7:3 favors the formation of discomes. Increasing the cholesterol level in the vesicular dispersion above 30% mol/mol suppresses the production of discomes due to increasing the rigidity of niosomes (Uchegbu et al., Citation1992; Abdelkader et al., Citation2011).
Figure 6. Confocal laser scanning micrographs of discomes (left) and conventional spherical niosomes (right) loaded with carboxyfluorescein (Abdelkader et al., 2012b).
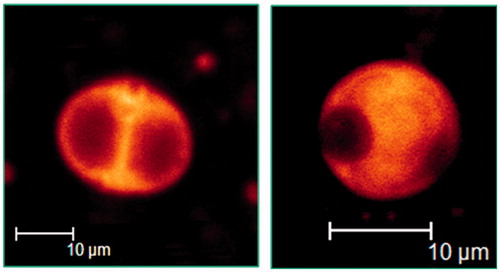
Discomes are thermoresponsive vesicles, which become leakier as the temperature increases from room temperature to 37 °C (Uchegbu & Vyas, Citation1998). Discomes have been proposed as a potential drug delivery system for ophthalmic administration (Uchegbu et al., Citation1992; Uchegbu & Vyas, Citation1998). They are capable of entrapping water-soluble drugs and their large faceted structure is likely to prevent their drainage and absorption into systemic circulation via the nasolacrimal duct. They have been investigated to control the ocular delivery of timolol maleate (Vyas et al., Citation1998). The prepared discomes showed a sustained drug release pattern and systemic absorption was minimized to a negligible level (Vyas et al., Citation1998). However, the formation of this system requires the incubation of the niosomal dispersion with Solulan C24 at 75 °C for at least 1 h. Such preparation conditions might affect the chemical stability of some thermolabile therapeutic agents when incubated at such high temperature. Recently, discomes encapsulating naltrexone hydrochloride, a promising agent for treatment of corneal healing disorders, were prepared under relatively milder conditions. The prepared discomes successfully controlled naltrexone permeation and enhanced its permeability through excised cow corneas (Abdelkader et al., Citation2011).
Vesicle preparation
Vesicle formation requires an input of energy, in the form of either gentle agitation or probe sonication. Usually, the surfactant/lipid mixtures are hydrated in the presence of the drug in an aqueous solution at a temperature above the gel/liquid transition temperature of the surfactant. The unentrapped drug can be separated by ultracentrifugation, low-pressure gel filtration chromatography or exhaustive dialysis.
Since vesicle size is an important determinant of drug biodistribution, it may be reduced by extrusion via nucleopore filters, microfluidization or high-pressure homogenization. The different methods used in surfactant/lipid hydration drug loading and niosome formation are summarized as follows.
The injection of an organic solution of surfactant/lipid into a hot aqueous solution of the drug to be encapsulated, which is heated above the boiling point of the organic solvent (ether injection) (Baillie et al., Citation1985).
The formation of a surfactant/lipid film by evaporation of an organic solution of surfactant/lipid. This lipid film is then hydrated with a solution of the drug (Azmin et al., Citation1985; Baillie et al., Citation1985). This method is commonly known as thin film hydration (TFH) or the conventional method. The niosomes generated by THF are multilamellar vesicles (MLV). The TFH method was previously described by (Bangham et al., Citation1965) for the preparation of liposomes.
The formation of a w/o emulsion from an organic solution of surfactant/lipid and an aqueous solution of the drug. The organic solvent is then slowly evaporated to leave niosomes dispersed in the aqueous phase. In some cases, a gel results which must be further hydrated to yield niosomes [reverse phase evaporation (REV)] (Szoka & Papahadjopoulos, Citation1978; Kiwada et al., Citation1985). The vesicles generated by the REV method are unilamellar or oligolamellar and are relatively more uniform in size compared with the TFH method (Szoka & Papahadjopoulos, Citation1978; Abdelkader et al., Citation2011).
The injection of melted surfactant/lipid into a highly agitated heated aqueous phase in which the drug is dissolved (Wallach & Philippot, Citation1993) or the addition of a warmed aqueous phase dissolving the drug to a mixture of melted lipids and a hydrophobic drug (Niemac et al., Citation1995). This method does not require the use of organic solvents, which are expensive, hazardous and difficult to completely remove.
The homogenization of a surfactant/lipid mixture, followed by bubbling of nitrogen gas through this mixture (Talsma et al., Citation1994). The bubbling unit consisted of a round bottom flask with three necks, positioned in a water bath to control temperature. A water-cooled reflux column was connected to the central neck of the flask to avoid loss of water during the experiment. A thermometer for temperature monitoring was positioned in the second neck. The third opening was used for the tubing. A continuous stream of gas bubbles was generated at the bottom of the flask by forcing nitrogen gas through a sintered glass filter with a surface area of 1 cm2 by a slight over pressure. Prior to the gas bubbling step, the lipid materials are added to the aqueous solution and then mixed for 30 min with a homogenizer. The prepared vesicle dispersion was in the size range of 0.2 µm to 0.5 µm. Omitting the homogenization step has been found not to affect the particle size; however, a longer “bubbling time” was required.
Supercritical carbon dioxide (scCO2) has been used as a more environmentally friendly alternative preparation medium for nisomes. The carbon dioxide gas can be in a fluid state (scCO2) under a temperature of 31.1 °C and pressure of 78.8 bars. The scCO2 has good solvating properties (Manosroi et al., Citation2008a). Niosomes composed of different molar ratios of Tween 61: cholesterol mixtures were successfully prepared using the scCO2 method and encapsulated glucose (a model water soluble agent). The scCO2 niosomes demonstrated higher drug entrapment efficiency than the niosomes prepared using the TFH method. However, The TFH niosomes showed superior stability and dispersibility compared with the scCO2 niosomes (Manosroi et al., Citation2008a).
Niosome size’s influence on in vivo performance
The particle size of niosomes can determine their behaviour in the body (Uchegbu & Vyas, Citation1998). It has been reported that particles smaller than 100 nm easily penetrate tumor tissues (Mayer et al., Citation1989). This is because these nano-particles can penetrate the discontinuous capillaries and fenestra of tumor tissues (Nakanoa et al., Citation2008). A size reduction step is occasionally incorporated into the niosome production procedure, subsequent to the initial hydration step. This is in order to improve the biodistribution of systemically injected niosomes and to target the administered niosomes at the affected area (Azmin et al., Citation1986; Uchegbu et al., Citation1995; Uchegbu & Vyas, Citation1998).
For ophthalmic niosome dispersions, the entrapped drug molecules are in the soluble molecular forms and they are either entrapped in the bilayer domains or encapsulated in the aqueous domains, depending on the drug’s physical properties (solubility and partition coefficient). Partitioning of the entrapped/encapsulated drug molecules throughout the bilayer membranes at the eye temperature could be the rate-controlling step for ocular drug absorption and subsequent ocular bioavailability. Niosome size of greater than 10 µm has been reported to be more suitable for ophthalmic drug delivery (Sahin, Citation2006). This allows them to encapsulate a considerable amount of therapeutic drug molecules, resist removal by lacrimation and prolong ocular residence time. Micro-size niosomes are not easily washed out by lacrimal fluid compared with nano-size ones(Uchegbu & Vyas, Citation1998).
Generally, size reduction (sonication or extrusion methods) adversely affects the entrapment efficiency of the active agent by vesicles. This could be ascribed to their small inner volume and drug leakage from small and intermediate-sized unilamellar vesicles (up to 100 nm) (Uchegbu & Florence, Citation1995, Uchegbu & Vyas, Citation1998; Zhigaltsev et al., Citation2001). A reduction in vesicle size may be achieved by a number of methods:
Probe sonication can produce niosomal vesicle sizes in the range of 100 to 140 nm (Azmin et al., Citation1985; Baillie et al., Citation1985). Although it is more effective than bath sonication, probe sonication suffers from high energy input to the solution, leading to a sharp local increase in the temperature of the solution and possible shedding of titanium particles into the sonicated dispersion. This necessitates an additional centrifugation step to remove the metallic particles from the niosomal dispersion.
Extrusion through polycarbonate (Nucleopore™) filters using liposome extruders.
A combination of sonication and filtration has been utilized to prepare doxorubicin niosomes in the 200 nm size range (Uchegbu et al., Citation1995).
High pressure homogenization also yields vesicles below 100 nm in diameter, although drug loading is ultimately sacrificed to achieve this small size (Uchegbu & Vyas, Citation1998).
Characterization of niosomes
Niosomes are versatile drug delivery devices and has numerous therapeutic applications. Hence, this versatility necessitates proper physicochemical characterisation techniques to suit the intended route of administration. summarizes different techniques used to characterize niosomes for bilayer formation, size, morphology, spreading ability, rigidity and skin/corneal penetrations.
Table 3. Overview of main characterization techniques for niosomes.
Non-conventional niosomes
Elastic niosomes
Over the past three decades, researchers in the field of liposomes have introduced many new class of liposomes. These have significant elasticity and ultradeformability, compared with conventional liposomes. These elastic or ultradeformable liposomes, Transferosomes®, demonstrated the ability to penetrate intact skin while carrying therapeutic concentrations of drugs (Cevc & Blume, Citation1992). They consist of phospholipids and an edge activator; an edge activator is often a single chain surfactant that can destabilize the lipid bilayers of the vesicles, and increase the deformability of the bilayers (Cevc et al., Citation1996). Parallel to this development, other classes of vesicles were produced; vesicles with ethanol (ethosomes) to provide flexibility were developed (Touitou et al., Citation1997). Ethosomes embodying a high concentration (20–45%) of ethanol demonstrated enhanced skin delivery and were reported to penetrate intact skin layers (Touitou et al., Citation2000). For more detail about these two delivery systems, the reader can refer to references (Elsayed et al., Citation2007; El Maghraby & Williams, Citation2009).
On the other hand there is very little published on unconventional niosomes; to our knowledge, only one study has reported on elastic niosomes (Manosroi et al., Citation2008b). Tween 61 and Span 60 niosomes entrapping diclofenac diethyammonium and embodying 0–25% ethanol have been developed. The prepared niosomes were called elastic niosomes. This is because the prepared niosomes registered deformability index values of 13.76, 3.44 times higher than conventional niosomes loaded and non-loaded with the drug respectively (Manosroi et al., Citation2008b).
Proniosomes
One approach to minimize the water content in liposomes and niosomes dispersions is to develop a vesicle preconcentrate, called proliposomes or proniosomes. Such preconcentrates contain all the vesicular components with ethanol and only traces of water. These systems are gel-like preparations that are believed to generate vesicles (in situ) when applied to the skin (El Maghraby & Williams, Citation2009; Ammar et al., Citation2011). Proniosomes are mainly composed of non-ionic surfactants, phospholipids, alcohols and water, with or without cholesterol (Mokhtar et al., Citation2008).
The effects of formulation composition, surfactant and alcohol type, and preparation method on the transdermal permeation profile of various proniosomes have been studied (Vora et al., Citation1998; Fang et al., Citation2001). The effects of non-ionic surfactants on the levonorgestrel permeation profile showed that the flux value was the highest for Span 80 and lowest for Span 60. No significant difference was observed in the skin permeation profile of formulations containing Span 40 and Span 60, due to their higher phase transition temperature, which is responsible for their lower permeability. Conversely, opposite effects in the case of estradiol were reported (Fang et al., Citation2001). Span 60 showed the highest flux on the estradiol permeation profile. Proniosomes from estradiol and levonorgestrel formulations differed in their content of cholesterol, type and content of alcohol. The effect of different alcohols on the drug permeation profile was found to be as follows: isopropanol > butanol > propanol > ethanol. Proniosomes could potentially evolve into a useful dosage form for transdermal drug delivery. This is due to their simple manufacturing scale-up and the ability of proniosomes to modulate drug delivery across the skin (El Maghraby & Williams, Citation2009; Ammar et al., Citation2011).
Niosomes as drug delivery systems
Over the past three decades, niosomes have been successfully utilized as a drug delivery system to solve some major biopharmaceutical problems. Poor bioavailability of slightly soluble drugs can be improved, as niosomes can accommodate hydrophobic molecules in their bilayer domains (Waraporn et al., Citation2006; Nasr et al., Citation2008). Niosomes can also minimize the side effects of some potent drugs by localizing the pharmacological effects to the target tissues (Baillie et al., Citation1985; Baillie et al., Citation1986; Vyas et al., Citation1998; Guinedi et al., Citation2005). Niosomes can serve as a non-toxic penetration enhancer for transdermal drug delivery (Schreier & Bouwstra, Citation1994; Solanki et al., Citation2010; Ammar et al., Citation2011). Moreover, niosomes can improve the chemical stability of photosensitive drugs by entrapping drug molecules in the aqueous internal milieu (Manconi et al., Citation2003, Abdelkader et al., Citation2012b). Below are discussed the most common applications of niosomes as drug delivery systems.
Anticancer delivery
Methotrexate-loaded niosomes were first utilized as a more stable and economic alternative drug delivery system to liposomes, in order to improve the therapeutic index of the anticancer drug by localizing the cytotoxic effects to target cells (Baillie et al., Citation1985). The anti-tumor activity of vincristine also increased in S-180 sarcoma and Ehrlich-ascites-bearing mice when encapsulated in niosomes (Parthasarathi et al., Citation1994). Moreover, Span 60 bleomycin niosomes increased the anti-tumor action of bleomycin in the abovementioned two tumor models (Naresh & Udupa, Citation1996). Cytotoxic side effects of doxorubicin decreased when encapsulated in C16G2 niosomes. Doxorubicin copolymer-loaded niosomes were not hemolytic in vitro at the doses selected for in vivo use. C16G2 niosomes also showed localized and sustained effects on liver and spleen (Uchegbu & Duncan, Citation1997). Furthermore, Span 40- and Span 60-based niosomal delivery systems for 5-flurouracil showed a sustained and higher local concentration of the drug in the liver and kidney of the rats, compared with the injectable drug solution (Namdeo & Jain, Citation1999). Recently, niosomes for the magnetic targeting of doxorubicin to a specific organ have successfully controlled drug release with no additional toxicity (Tavano et al., Citation2013).
Anti-infective delivery
Sodium stibogluconate is a drug used in the treatment of visceral leshmniasis (a protozoan infection of the reticuloendothelial system). The drug’s pharmacokinetics was equally altered by encapsulation into liposomes and niosomes (Hunter et al., Citation1988). It was found that niosomal sodium stibogluconate was significantly more active in reducing parasite burden than the free drug; the effect observed after multiple dosing suggested that niosomal formulations act as a depot within the liver.
Rifampicin, an anti-tuberculosis drug, encapsulated in Span 85 niosomes was found to accumulate in the lungs of mice (Jain & Vyas, Citation1995). Therefore, it can offer the possibility of improving anti-tuberculosis therapy (Jain & Vyas, Citation1995). Moreover, niosomes composed of Span 60: cholesterol: dicetyl phosphate at 4:2:1 molar ratio successfully encapsulated ribavirin (an antiviral agent used for treatment of hepatitis C). The concentration of ribavirin in the rat liver obtained from the ribavirin niosomes was 6-fold higher than that obtained from the ribavirin solution after an intraperitoneal injection of a single dose equivalent to 30 mg/kg of the drug. This demonstrates effective liver targeting properties for niosomes (Hashim et al., Citation2010).
Vaccine and macromolecule delivery
Span 60 niosomes loaded with tetanus toxoid (TT) were prepared by the REV method. The prepared niosomes were coated with a modified polysaccharide O-palmitoyl mannan (OPM), in order to protect the niosomes from enzymatic degradation in the gastrointestinal tract and to enhance their affinity towards the antigen presenting cells of Peyer’s patches. The results were compared with alum-adsorbed TT following oral and intramuscular administration, and it was observed that OPM-coated niosomes produced higher IgG levels compared with plain uncoated niosomes and alum-adsorbed TT upon oral administration. Oral niosomes also elicited a significant mucosal immune response (sIgA levels in mucosal secretions). The developed niosomes also elicited a combined serum IgG2a/IgG1 response suggesting that they were capable of eliciting both humoral and cellular response. The proposed system was simple, stable, and cost-effective and may be clinically acceptable. The study emphasized the potential of OPM-coated niosomes as an oral vaccine delivery carrier (Jain & Vyas, Citation2006). Moreover, niosomes loaded with insulin were prepared using the TFH method. The Span 60 niosomes protected insulin against proteolytic enzymes, and showed good stability in the presence of sodium desoxycholate (Varshosaz et al., Citation2003).
Transdermal delivery
Niosomes were studied as a transdermal drug delivery systems and their ability to enhance drug permeation and reduce skin irritation through the intact stratum corneum was also investigated (Reddy & Udupa, Citation1993; Schreier & Bouwstra, Citation1994; Van Hal et al., Citation1996; Ammar et al., Citation2011).
For instance, small niosomes (100 nm) have been visualized by freeze fracture electron microscopy technique between the first and second layer of human corneocytes 48 h after incubation with niosomes (Junginger et al., Citation1991). The penetration of this upper layer by niosomes appeared feasible (Junginger et al., Citation1991). However, no transport of niosomes took place across the whole skin layer (Junginger et al., Citation1991).
In vitro studies on the transdermal penetration of oestradiol using high-phase transition sucrose ester niosomes or C18EO7 niosomes and low phase transition poly-7-oxyethylene alkyl ether (C12EO7) niosomes revealed that the latter were better transdermal carriers (Van Hal et al., Citation1996). C12EO7 micelles were ineffective as drug carriers in this study. The higher flexibility of these bilayers is believed to be responsible for the improved transdermal penetration (Van Hal et al., Citation1996). Also, it was found that reducing the cholesterol content in niosomes increased the transdermal delivery of oestradiol. These can be attributed to increases in the flexability of the vesicles (Van Hal et al., Citation1996).
Niosomes were developed for the transdermal drug delivery of nimesulide (non-steroidal anti-inflammatory drug). The prepared niosomes were evaluated in vivo in rats using the carrageenan-induced rat paw edema method. This investigation conclusively demonstrated that the niosomes successfully prolonged the drug release into the skin and across the skin (Shahiwala & Misra, Citation2002).
Furthermore, permeation of ketorolac (a potent nonsteroidal anti-inflammatory drug) across excised rabbit skin from various proniosome gel formulations was investigated using Franz diffusion cells. The prepared proniosomes significantly improved drug permeation and reduced lag time (Ibrahim et al., Citation2005).
Ophthalmic delivery
Niosomes have been evaluated as ocular vehicles for a wide range of therapeutic classes such as anticholinergic (cyclopentolate), anti-glaucoa (acetazolamide and timolol maleate) and antibiotics (gentamicin) (Saettone et al., Citation1996; Vyas et al., Citation1998; Aggarwal et al., Citation2004; Abdelbary & El-gendy, Citation2008). Tween 20-based niosomes significantly improved the ocular bioavailability of cyclopentolate, with respect to the reference buffer drug solution and micellar solution, suggesting that it can be used as an efficient vehicle for ocular drug delivery (Saettone et al., Citation1996). Also, niosomes encapsulating timolol maleate (anti-gluacoa agent) were prepared using the reverse phase evaporation method and coated with 0.5% w/v chitosan solution. The timolol niosomes achieved a significantly higher (1.7 times) timolol concentration in the aqueous humor than that of the drug solution. This was attributed to better improvement of corneal penetration and prolonged precorneal residence time compared with the timolol aqueous solution (Kaur et al., Citation2010). Niosomes should have certain attributes in relation to their potential use as vehicles for ocular drug delivery. These include:
The size of the vesicle should be large enough to resist drainage by reflex tearing and eye blinking. For example, large multilamellar vesicles (MLV) were found to reside for a longer period on the ocular surface than small unilamellar liposomes. This was attributed to the ability of MLV to entrap a relatively higher amount of the drug and resist nasolacrimal drainage (Fitzgerald et al., Citation1987; Hathout et al., Citation2007). The same argument applies to niosomes. Ideally, it has been reported that niosome sizes > 10 µm are suitable for ocular delivery (Uchegbu & Vyas, Citation1998; Sahin, Citation2006).
The shape of the prepared niosomes should show some irregularities to fit properly into the cul-de-sac of the eye and lodge on the eye surface (Uchegbu et al., Citation1992; Uchegbu et al., Citation1997; Uchegbu & Vyas, Citation1998).
Ocular niosomes should ideally be thermo-responsive in order to release drug content in a controlled, yet timely manner before being flushed by blinking and nasolacrimal drainage (Uchegbu et al., Citation1997; Uchegbu & Vyas, Citation1998). Complete abolishment of gel/liquid transition of surfactant forming niosomes could produce niosomes with an extremely low release rate to water soluble drugs, which does not suit the short residence time of ocularly administered ophthalmic products.
The above mentioned criteria have typically been attributed to discomes and polyhedral niosomes. These are modified vesicular structures and are different from conventional spherical niosomes (Uchegbu & Florence, Citation1995; Uchegbu & Vyas, Citation1998; Azeem et al., Citation2009). Discomes containing a low cholesterol concentration less than 30% mol/mol were prepared by incubating the preformed spherical niosomes in cholesteryl poly-24-oxyethylene ether (Solulan C24) at 75 °C for 1 h. This resulted in the formation of large (11–60 µm) and multifaceted vesicular systems (Uchegbu et al., Citation1992). Since then, there has been only one report on discomes as an ocular delivery system for timolol maleate (Vyas et al., Citation1998). The prepared discomes have been found to entrap a relatively large quantity of timolol and improve the ocular bioavailability compared with a timolol maleate solution (Vyas et al., Citation1998). One possible reason for such scarcity is the need for a relatively high temperature during discome preparation which might affect the chemical stability of some thermo-labile therapeutic agents. On the other hand, spherical niosomes have been shown to possess more stable membranes than polyhedral ones due to the presence of cholesterol (Arunothayanun et al., Citation1999).
Toxicity of niosomes
There are limited research reports on the toxicity of niosomes. Different niosomal formulations were evaluated for inhibiting the cell proliferation of SV-40 human keratinocytes (Hofland et al., Citation1991). The effects of molecular structure of the surfactant and the incorporation of cholesterol on the cell proliferation were investigated. The surfactants were composed of different hydrocarbon chain lengths (12, 14, 17 and 18) and polyoxethylene chain lengths (3 and 7). Also, ether- and ester-type surfactants were examined. It was found that both the hydrocarbon chain length and the polyoxyethylene chain length had minor effects on cell proliferation. However, the bond (an ether or ester bond) by which the alkyl chain was linked to the polyoxyethylene head group had a strong effect on the cell proliferation. The concentration of the ether type-surfactants that inhibited cell proliferation by 50% was 10 times lower than for the ester-type surfactants. The cholesterol content of the bilayers has not been found to have an effect on cell proliferation. The low toxicity of ester-type surfactants compared with ether-type surfactants could be attributed to enzymatic degradation of ester bonds (Hofland et al., Citation1992).
In some instances, encapsulation of some drugs in niosomes reduces the toxicity. For example, niosomes encapsulating vincristine decreased the neurological symptoms, diarrhea and alopecia following intravenous administration of vincristine (Parthasarathi et al., Citation1994).
Ocular toxicity of niosomes has been recently assessed through studying conjunctival and corneal irritation potential of Span 60 niosomes, and Span 60 niosomes containing different concentrations of bilayer membrane additives such as dicetyl phosphate, sodium cholate and Solulan C24 using an acceptable in vitro conjunctival model (hen's egg chorioallantoic membranes) and excised bovine corneal opacity and permeability models. This study has revealed minimal ocular irritations indicating good ocular tolerability for niosomes (Abdelkader et al., Citation2012a).
Physical stability of niosomes
One of the most important features of niosomes compared with liposomes is their chemical stability. Niosomes are less vulnerable to chemical degradation or oxidation than phospholipids (Baillie et al., Citation1985; Zuidam et al., Citation2003). However, both liposomes and niosomes can equally show physical stability problems. Vesicle aggregation, bilayer fusion and drug leakage are the most common physical changes exhibited. Physically stable niosomal dispersions must exhibit a constant particle size and a constant level of entrapped drug over time.
It has been shown that niosomes are also more physically stable than liposomes (Mukherjee et al., Citation2007; Nasr et al., Citation2008). For example, aceclofenac Span 60 niosomes showed higher physical stability (in terms of particle aggregation and drug leakage) than liposomes when stored for three months at 4 °C (Nasr et al., Citation2008). Also, both reconstituted and lyophilized Span 20-based niosomes were more physically stable than liposomes when stored at 4 and 20 °C for 3 months (Mukherjee et al., Citation2007). Additionally, Tween 61 niosomes with 25% ethanol and Span 60 niosomes with 20% ethanol (elastic niosomes) exhibited no sedimentation, no layer separation and an unchanged particle size at 4, 27 and 45 °C within three months (Manosroi et al., Citation2008b).
It is worth mentioning that the main factors determining the stability of niosomes include surfactant/lipid type, original size and temperature of storage.
First, the choice of surfactant determines the fluidity of the bilayer membrane. It has been found the higher the gel-liquid transition temperature, the higher the drug-retentive properties of niosomes. This is because the bilayer membranes are always in a gel state at the storage conditions. The likelihood of carboxyfluorescein-loaded Span niosomes leaking was found to be in the following order: Span 60 > Span 40 > Span 20 > Span 80 (Yoshioka & Florence, Citation1994). Also, the incorporation of cholesterol (membrane stabilizer) in niosomes improves their physical stability (Uchegbu & Vyas, Citation1998).
Secondly, niosomes sized between 1 and 10 µm have been found to be more stable than those in the sub-micron range. Thermodynamically, smaller niosomes have higher surface free energy and tend to aggregate more than larger ones in order to lower the excess free energy (Moazeni et al., Citation2010). For example, niosomes composed of hexadecyl diglycerol: cholesterol: Solulan C24 at a 40:40:10 molar ratio with an original size of 70 nm were monitored for changes in size. The size of the niosomes increased 250-fold. This result demonstrated that the original size has a significant effect on the stability of niosomes (Uchegbu & Vyas, Citation1998).
Thirdly, the storage temperature for niosomes must be controlled, as a change in temperature often leads to a change in the nature of the surfactant bilayer membranes (Tanaka, Citation1990; Uchegbu & Vyas, Citation1998). In this context, Span 40 niosomes have been found to be more stable than liposomes at higher storage temperatures such as 25 and 37 °C since Span 40 has a higher transition temperature than phospholipids (Ning et al., Citation2006).
Limitations and future direction
The physical stability and sterilization of niosomes are the two major obstacles that hinder its utilization as potential drug delivery systems.
There are two approaches to improve the physical stability of lipid vesicles at ambient conditions. Dispersing liposomes in a viscous gel has been used either to reduce the rapid leakage of the encapsulated drug from liposomes (Meisnera & Mezeib, Citation1995) or to minimize the burst release effect observed with liposomes (Mehanna et al., Citation2009, Citation2010). This approach may be extrapolated to improve the physical stability of ocular niosomes. However, the drug release from such a system is likely to be complex, as the drug molecules have to release from the bilayer membranes and diffuse through the viscous gel. Furthermore, the improvement in the physical stability of the niosomes can be offset by reducing the ocular bioavailability of the administered drug. Additionally, a topical application of a viscous gel is less convenient than eye drops in terms of ocular administration and the adjustment of the dose.
In addition to the previous approach, converting the final liposomal/niosomal liquid dispersion to a powder form by lyophilization (freeze-drying) or spray-drying not only enhances the physical stability of the vesicles (Ingvarsson et al., Citation2011), but can also dramatically reduce the oxidative instability of oxidizable drug molecules, by minimising the formation of hydroxyl free radicals (Uchegbu & Vyas, Citation1998; Waterman et al., Citation2002).
These advantages can be offset by high production costs; long production time; and more complicated formulations containing cryoprotectants. The ready-to-use niosomal dispersions are the easiest and most convenient for producing and administering a dosage form. Accordingly, the direction of our future research on niosomes will be concerned with studying different strategies for improving the physical stability of niosomes.
Sterilization of lipid-based (niosomes/liposomes) products is a challenging endeavor. Heat sterilization is not recommended for lipid-based delivery systems (Zuidam et al., Citation1993). Dry heat and steam sterilization are unsuitable and destructive for lipid-based formulations with a gel/liquid transition temperature which is relatively lower than temperature involved in heat sterilization, since this may cause extensive drug leakage from the bilayer vesicles. Membrane filtration is also unsuitable for particulate delivery systems larger than the pore size (0.22 µm) of the bacterial filters. On the other hand, preparation under aseptic conditions, gas (ethylene oxide) and gamma irradiation could be useful, since minimal heat is generated during the sterilization process. Liposomes and niosomes are prepared in a sterile form by passing all organic lipid, buffer and drug solutions through bacterial filters; and then the final product is prepared under aseptic conditions (Hathout et al., Citation2007; Abdelbary & El-gendy, Citation2008). Gamma irradiation has high penetration power, so it can be applied to a packaged product and is applicable to heat-sensitive drugs. Many marketed pharmaceuticals are gamma radiation-sterilized, such as ophthalmic ointments, drops and injectables (Waterman et al., Citation2002). These sterilization methods could be of a potential value for producing sterile niosomes, and hence the effect of gamma irradiation on the physical stability of niosomes could be a potential research line for future work.
Declaration of interest
The authors report no conflicts of interest. The authors alone are responsible for the content and writing of this article.
References
- Abdelbary G, El-Gendy N. (2008). Niosome-encapsulated gentamicin for ophthalmic controlled delivery. AAPS Pharm Sci Tech 9:740–7
- Abdelkader H. (2012). Design and characterisation of niosomes for ocular delivery of naltrexone hydrochloride [PhD]. The University of Auckland
- Abdelkader H, Ismail S, Kamal A, Alany R. (2011). Design and evaluation of controlled-release niosomes and discomes for naltrexone hydrochloride ocular delivery. J Pharm Sci 100:1833–46
- Abdelkader H, Ismail S, Kamal A, et al. (2012a). Conjunctival and corneal tolerability assessment of ocular naltrexone niosomes and their ingredients on the hen’s egg chorioallantoic membrane and excised bovine cornea models. Int J Pharm 432:1–10
- Abdelkader H, Kamal H, Ismail S, Alany RG. (2010). Preparation of niosomes as an ocular delivery system for naltrexone hydrochloride: physicochem Characterization. Pharmazie 65:811–7
- Abdelkader H, Wu Z, Al-Kassas R, Alany R. (2012b). Niosomes and discomes for ocular delivery of naltrexone hydrochloride: morphological, rheological, spreading properties and photo-protective effects. Int J Pharm 433:142–8
- Aggarwal D, Garg A, Kaur IP. (2004). Development of a topical niosomal preparation of acetazolamide: preparation and evaluation. J Pharm Pharmacol 56:1509–17
- Ammar HO, Ghorab M, El-Nahhas SA, Higazy IM. (2011). Proniosomes as a carrier system for transdermal delivery of tenoxicam. Int J Pharm 405:142–52
- Arora S, Prashar B, Dhamija H, et al. (2012). Niosome: the unique vesicular drug carriers. J Drug Del Ther 2:96–101
- Arunothayanun P, Turton JA, Uchegbu IF, Florence AT. (1999). Preparation and in vitro/in vivo evaluation of luteinizing hormone releasing hormone (LHRH)-loaded polyhedral and spherical/tubular niosomes. J Pharm Sci 88:34–8
- Azeem A, Anwer K, Talegaonkar S. (2009). Niosomes in sustained and targeted drug delivery: some recent advances. J Drug Target 17:671–89
- Azmin MN, Florence AT, Handjani-Vila RM, et al. (1985). The effect of non-ionic surfactant vesicle (niosome) entrapment on the absorption and distribution of methotrexate in mice. J Pharm Pharmacol 37:237–42
- Azmin MN, Florence AT, Handjani-Vila RM, et al. (1986). The effect of niosome and polysorbate 80 on the metabolism and excretion of methotrexate in mouse. J Microencap 3:95–100
- Baillie AJ, Coombs GH, Dolan TF, Laurie J. (1986). Non-ionic surfactant vesicles, niosomes, as a delivery system for the anti-leshmanial drug, sodium stibogluconate. J Pharm Pharmacol 38:502–5
- Baillie AJ, Florence AT, Hume LR, et al. (1985). The preparation and properties of niosomes-non-ionic surfactant vesicles. J Pharm Pharmacol 37:863–8
- Balakrishnana P, Shanmugama S, Lee WS, et al. (2009). Formulation and in vitro assessment of minoxidil niosomes for enhanced skin delivery. Int J Pharm 377:1–8
- Bangham AD, Standish MM, Watkins JC. (1965). Diffusion of univalent ions across the lamellae of swollen phospholipids. J Mol Biol 13:238–52
- Cevc G, Blume G. (1992). Lipid vesicles penetrate into intact skin owing to the transdermal osmotic gradients and hydration force. Biochim Biophys Acta 1104:226–32
- Cevc G, Blume G, Schatzlein A, et al. (1996). The skin: a pathway for systemic treatment with patches and lipid-based agent carriers. Adv Drug Deliv Rev 18:349–78
- Egelhaaf RM, Epand RF, Maekawa S. (2003). The arrangement of cholesterol in membranes and binding of NAP-22. Chem Phys Lipids 122:33--9
- El Maghraby GM, Williams AC. (2009). Vesicular systems for delivering conventional small organic molecules and larger macromolecules to and through human skin. Expert Opin. Drug Deliv 6:149–63
- Elsayed M, Cevc G. (2011). The vesicle-to-micelle transformation of phospholipid–cholate mixed aggregates: a state of the art analysis including membrane curvature effects. Biochimica et Biophysica Acta 1808:140–53
- Elsayed MM, Abdallah OY, Naggar V, Khalafallah NM. (2007). Lipid vesicles for skin delivery of drugs: reviewing three decades of research. Int J Pharm 332:1–16
- Fang JY, Yu SY, Wu PC, et al. (2001). In vitro skin permeation of estradiol from various proniosome formulations. Int J Pharm 215:91–9
- Fitzgerald P, Hadgraft J, Kreuter J, Wilson CG. (1987). A gamma-scintigraphic evaluation of microparticulate ophthalmic delivery systems: liposomes and nanoparticles. Int J Pharm 40:81–4
- Florence AT, Baillie AJ. (1989). Non-ionic surfactant vesicles alternatives to liposomes in drug delivery? In: Prescott LE, Nimmo WS, eds. Novel drug delivery and its therapeutic application. San Francisco, CA: John Wiley & Sons Ltd, 281--96
- Girigoswami A, Das S, De S. (2006). Fluorescence and dynamic light scattering studies of niosomes-membrane mimetic systems. Spectrochimica Acta A 64:859–66
- Guinedi AS, Mortada ND, Mansour S, Hathout RM. (2005). Preparation and evaluation of reverse-phase evaporation and multilamellar niosomes as ophthalmic carriers of acetazolamide. Int J Pharm 306:71–82
- Hashim F, El-Ridy M, Nasr M, Abdallah Y. (2010). Preparation and characterization of niosomes containing ribavirin for liver targeting. Drug Delivery 17:282–7
- Hathout RM, Mansour S, Mortada ND, Guinedi AS. (2007). Liposomes as an ocular delivery system for acetazolamide: in vitro and in vivo studies. AAPS PharmSciTech 8:E1–12
- Hofland HE, Bouwstra JA, Verhoef JC, et al. (1992). Safety aspects of non-ionic surfactant vesicles – a toxicity study related to the physichochmical characteristics of non-ionic surfactants. J Pharm Pharmacol 44:287–94
- Hofland HEJ, Bouwstra JA, Ponec M, et al. (1991). Interactions of non-ionic surfactant vesicles with cultured keratinocytes and human skin in vitro: a survey of toxicological aspects and ultrastructural changes in stratum corneum. J Contr Rel 16:155–68
- Hunter CA, Dolan TF, Coombs GH, Baillie AJ. (1988). Vesicular system (niosome & liposome) for delivery of sodium stibogluconate in experimental murine visceral leishmaniasis. J Pharm Pharmacol 40:161–5
- Ibrahim AA, Bosela AA, Ahmed SM, Mahrous GM. (2005). Proniosomes as a drug carrier for transdermal delivery of ketorolac. Euro J Pharm Biopharm 59:485–90
- Ingvarsson PT, Yang M, Nielsen HM, et al. (2011). Stabilization of liposomes during drying. Expert Opinion Drug Delivery 8:375–88
- Israelachvili JN, Marcelja S, Horn RG. (1980). Physical principles of membrane organization. Quart Rev Biophys 13:121–200
- Israelachvili JN, Mitchell DJ. (1975). A model for the packing of lipids in bilayer membranes. Biochim Biophys Acta 389:13–9
- Israelachvili JN, Mitchell DJ, Ninham BW. (1976). Theory of self-assembly of hydrocarbon amphiphiles into micelles and bilayers. J Chem Soc Faraday Trans II 72:1525–68
- Jain CP, Vyas SP. (1995). Preparation and characterisation of niosomes containing rifampicin for lung targeting. J Microencap 12:401–7
- Jain S, Vyas SP. (2006). Mannosylated niosomes as adjuvant-carrier system for oral mucosal immunization. J Liposome Res 16:331–45
- Junginger HE, Hofland HEJ, Bouwstra JA. (1991). Liposomes and niosomes: interactions with human skin. Cosmet Toilet 106:45–50
- Kaur IP, Aggarwal D, Singh H. (2010). Improved ocular absorption kinetics of timolol maleate loaded into a bioadhesive niosomal delivery system. Graefe's Archive Clin Experiment Ophthalmol 248:1467–72
- Kaur IP, Garg A, Aggarwal D. (2004). Vesicular systems in ocular drug delivery: an overview. Int J Pharm 269:1–14
- Kaur IP, Rana C, Singh H. (2008). Development of effective ocular preparations of antifungal agents. J Ocul Pharmacol Ther 24:481–93
- Kim JG, Kim JD. (1991). Vesicle to micelle transitions of egg phosphatidylcholine liposomes induced by nonionic surfactants, poly(oxyethylene) cetyl ethers. J Biochem 110:436–42
- Kiwada H, Niimura H, Fujisaki Y, et al. (1985). Application of synthetic alkyl glycoside vesicles as drug carriers. I preparation and physical properties. Chem Pharm Bull 33:753–9
- Lawrence MJ. (2003). Sorbitan esters (sorbitan fatty acid esters). In: Rowe RC, Sheskey PL, Weller PL, eds. Handbook of pharmaceutical excipients. 4th edn. London, UK: Pharmaceutical Press
- Manconi M, Valenti D, Sinico C, et al. (2003). Niosomes as carriers for tretinoin II. Influence of vesicular incorporation on tretinoin photostability. Int J Pharm 260:261–72
- Manosroi A, Chutoprapat R, Abe M, Manosroi J. (2008a). Characteristics of niosomes prepared by supercritical carbon dioxide (scCO2) fluid. Int J Pharm 352:248–55
- Manosroi A, Jantrawuta P, Manosroi J. (2008b). Anti-inflammatory activity of gel containing novel elastic niosomes entrapped with diclofenac diethylammonium. Int J Pharm 360:156–63
- Manosroi A, Wongtrakul P, Manosroi J, et al. (2003). Characterization of vesicles prepared with various non-ionic surfactants mixed with cholesterol. Colloids Surf B Biointerfaces 30:129–38
- Maurer N, Zhigaltsev I, Cullis PR. (2007). Encapsulation of nucleic acid–based therapeutics. In: Gregoriadis G, ed. Liposome technology: entrapment of drugs and other materials into liposomes. 3rd edn. New York: Informa Healthcare, 149--68
- Mayer LD, Tai LC, Ko DS, et al. (1989). Influence of vesicle size, lipid composition, and drug-to-lipid ratio on the biological activity of liposomal doxorubicin in mice. Cancer Res 49:5922–30
- Mehanna MM, Elmaradny HA, Samaha MW. (2009). Ciprofloxacin liposomes as vesicular reservoirs for ocular delivery: formulation, optimization, and in vitro characterization. Drug Development Industrial Pharm 35:583–93
- Mehanna MM, Elmaradny HA, Samaha MW. (2010). Mucoadhesive liposomes as ocular delivery system: physical, microbiological and in vivo assessment. Drug Development Industrial Pharmacy 36:108–18
- Meisnera D, Mezeib M. (1995). Liposome ocular delivery systems. Adv Drug Delivery Rev 16:75–93
- Moazeni E, Gilani K, Sotoudegan F, et al. (2010). Formulation and in vitro evaluation of ciprofloxacin containing niosomes for pulmonary delivery. J Microencapsul 27:618–27
- Mokhtar M, Sammour OA, Hammad MA, Megrab NA. (2008). Effect of some formulation parameters on flurbiprofen encapsulation and release rates of niosomes prepared from proniosomes. Int J Pharm 361:104–11
- Mukherjee B, Patra B, Layek B, Mukherjee A. (2007). Sustained release of acyclovir from nano-liposomes and nano-niosomes: an in vitro study. Int J Nanomedicine 2:213–25
- Muzzalupo R, Tavano L, Cassano R, et al. (2011). A new approach for the evaluation of niosomes as effective transdermal drug delivery systems. Eur J Pharm Biopharm 79:28–35
- Nakanoa K, Tozuk Y, Yamamotob H, et al. (2008). A novel method for measuring rigidity of submicron-size liposomes with atomic force microscopy. Int J Pham 355:203–9
- Namdeo A, Jain NK. (1999). Niosomal delivery of 5-fluorouracil. J Microencapsul 16:731–40
- Naresh RAR, Udupa N. (1996). Niosome encapsulated bleomycin. STP Pharm Sci 6:61–71
- Nasr M, Mansour S, Mortada ND, Elshamy AA. (2008). Vesicular aceclofenac systems: a comparative study between liposomes and niosomes. J Microencapsul 25:499–512
- Niemac SM, Ramachandran C, Weiner N. (1995). Influence of non-ionic liposomal composition on topical delivery of peptide drugs into pilosebaceous units: an in-vivo study using hamster ear model. Pharm Res 12:1184–8
- Ning M, Guo Y, Pan H, et al. (2006). Preparation and characterization of EP-liposomes and Span 40-niosomes. Pharmazie 61:208–212
- Pardakhty A, Varshosaz J, Rouholamini A. (2007). In vitro study of polyoxyethylene alkyl ether niosomes for delivery of insulin. Int J Pharm 328:130–41
- Parthasarathi G, Udupa N, Umadevi P, Pillai GK. (1994). Niosome encapsulated of vincristine sulfate-improved anticancer activity with reduced toxicity in mice. J Drug Target 2:173–82
- Perrie Y, Mohammed AUR, Vangala A, McNeil S. (2007). Environmental scanning electron microscopy offers real-time morphological analysis of liposomes and niosomes. J Liposome Res 17:27--37
- Planas ME, Gonzalez P, Rodriguez L, et al. (1992). Noninvasive percutaneous induction of topical analgesia by a new type of drug carrier, and prolongation of local pain on sensitivity by anesthetic Liposomes. Anesth. Analg 75:615–21
- Rajera R, Nagpal K, Singh SK, Mishra DN. (2011). Niosomes: a controlled and novel drug delivery system. Biol Pharm Bull 34:945–53
- Reddy DN, Udupa N. (1993). Formulation and evaluation of oral and transdermal preparations of flurbiprofen and piroxicam incorporated with different carriers. Drug Dev Ind Pharm 19:843–52
- Saettone MF, Perini G, Carafa M, et al. (1996). Non-ionic surfactant vesicles as ophthalmic carriers for cyclopentolate. A preliminary evaluation. STP Pharma Sci 6:94–8
- Sahin NO. (2007). Niosomes as nanocarrier systems. In: Mozafari MR, ed. Nanomaterials and nanosystems for biomedical applications. New York: Springer, 67--81
- Schreier H, Bouwstra J. (1994). Liposomes and niosomes as topical drug carriers-dermal and transdermal drug-delivery. J Control Rel 30:1–15
- Shahiwala A, Misra A. (2002). Studies in topical application of niosomally entrapped nimesulide. J Pharm Pharmaceut Sci 5:220–5
- Shamma RN, Elsayed I. (2013). Transfersomal lyophilized gel of buspirone HCl: formulation, evaluation and statistical optimization. J Liposome Res 23:244–54
- Solanki AB, Parikh JR, Parikh RH, and Patel MR. (2010). Evaluation of different compositions of niosomes to optimize aceclofenac transdermal delivery. Asian Journal of Pharmaceutical Sciences 5:87–95
- Szoka F, Papahadjopoulos D. (1978). Procedure for preparation of liposomes with large internal aqueous space and high capture by reverse-phase evaporation. Proc Natl Ac Sci 75:4194–8
- Talsma H, Steenbergen MJV, Borchert JCH, Crommelin DJA. (1994). A novel technique for the one-step preparation of liposomes and non-ionic surfactant vesicles without the use of organic solvents. Liposome formation in a continuos gas stream: the bubble method. J Pharm Sci 83:276–80
- Tanaka M. (1990). Properties of aqueous vesicle dispersion formed with poly(oxyethylene) hydrogenated castor oil. J Am Oil Chem Soc 67:55–60
- Tanford C. (1973). The hydrophobic effect: formation of micelles and biological membranes. New York: John Wiley
- Tavano L, Vivacqua M, Carito V, et al. (2013). Doxorubicin loaded magneto-niosomes for targeted drug delivery. Colloids Surfaces B: Biointerfaces 102:803–7
- Touitou E, Alkabes M, Dayan N. (1997). Ethosomes: novel lipid vesicular system for enhanced delivery. Pharm Res S14:305–6
- Touitou E, Dayan N, Bergelson L, et al. (2000). Ethosomes—novel vesicular carriers for enhanced delivery: characterization and skin penetration properties. J Control Rel 65:403–18
- Uchegbu IF, Bouwstra JA, Florence AT. (1992). Large disk-shaped structures (Discomes) in non-ionic surfactant vesicles to micelle transitions. J Phys Chem 96:10548–53
- Uchegbu IF, Double JA, Turton JA, Florence AT. (1995). Distribution, metabolism and tumoricidal activity of doxorubicin administered in sorbitan monostearate (Span 60) niosomes in the mouse. Pharm Res 12:1019–24
- Uchegbu IF, Duncan R. (1997). Niosomes containing N-(2 hydroxypropyl)methacrylamide copolymer-doxorubicin (PK1): effect of method of preparation and choice of surfactant on niosome characteristics and a preliminary study of body distribution. Int J Pharm 155:7–17
- Uchegbu IF, Florence AT. (1995). Non-ionic surfactant vesicles (niosomes): physical and pharmaceutical chemistry. Adv Colloid Interface Sci 58:1–55
- Uchegbu IF, Gianasi ECF, Florence AT, Duncan R. (1996). Niosome encapsulation of a doxorubicin polymer conjugate. Eur J Pharm Sci 4:S28
- Uchegbu IF, Schatzlein AG, Vanlerrberghe G, et al. (1997). Polyhedral non-ionic surfactant vesicles. J Pharm Pharmacol 49:606–10
- Uchegbu IF, Vyas SP. (1998). Non-ionic surfactant based vesicles (niosomes) in drug delivery. Int J Pharm 172:33–70
- van Hal D, Vanrensen A, Devringer T, et al. (1996). Diffusion of estradiol from non-ionic surfactant vesicles through human stratum-corneum in vitro. STP Pharma Sci 6:72–8
- Varshosaz J, Pardakhty A, Hajhashemi V, Najafabadi AR. (2003). Development and physical characterization of sorbitan monoester niosomes for insulin oral delivery. Drug Deliv 10:251–62
- Vora B, Khopade A, Jain N. (1998). Proniosome based transdermal delivery of levonorgestrel for effective contraception. J Control Rel 54:149–65
- Vyas SP, Mysore N, Jaittley V, Venkatesan N. (1998). Discoidal niosome based controlled ocular delivery of Timolol maleate. Pharmazie 53:466–9
- Wallach DFH. Philippot JR. (1993). New type of lipid vesicle: novasome. In: Gregoriadis G, ed. Liposome Technology. Florida: CRC Press, 141--56
- Walter A, Vinson PK, Kaplun A, Talmon Y. (1991). Intermediate structures in the cholate-phosphatidylcholine vesiclemicelle transition. Biophs J 60:1315–25
- Waraporn S, Boonsri O, Nontima V. (2006). Preparation and characterization of propylthiouracil niosomes. J Liposome Res 16:391–401
- Waterman KC, Adami RC, Alsante KM, et al. (2002). Stabilization of pharmaceuticals to oxidative degradation. Pharm Dev Technol 7:1–32
- Yoshioka TBS, Florence AT. (1994). Preparation and properties of vesicles (niosomes) of sorbitan monoesters (Span 20, Span 40, Span 60 and Span 80) and a sorbitsan triester (Span 85). Int J Pharm 105:1–6
- Zhigaltsev IV, Kaplun AP, Kucheryanu VG, et al. (2001). Liposomes containing dopamine entrapped in response to transmembrane ammonium sulfate gradient as carrier systrem for dopamine delivery into the brain of Parkinsonian mice. J Liposome Res 11:55–71
- Zuidam NJ, Lee SS, Crommelin DJA. (1993). Sterilization of liposomes by heat treatment. Pharm Res 10:1591–6
- Zuidam NJ, Winden EV, Vrueh R, Crommelin DJA. (2003). Stability, storage, and sterilization of liposomes. In: Torchilin V, Weissig V, eds. Liposomes: a practical approach. 2nd edn. New York: Oxford University Press, 149--65