Abstract
High drug loading is one of the important issues in the drug delivery research, especially the drug delivery system by oral administration. If high drug loading carriers are utilized the times of drug intake could be significantly reduced. Accordingly in this study, ordered mesoporous SBA-15 modified with (3-aminopropyl) triethoxysilane (APTES) was used as a carrier for nonsteroidal anti-inflammatory drug and optimization of the loading process was done. SBA-15 silica material with rope-like morphology was synthesized and modified by post-synthesis method with APTES. The synthesized SBA-15 and modified SBA-15 were characterized by XRD, SEM, thermal analysis and FT-IR spectroscopy. Loading optimization experiments were performed by changing the factors affecting the drug loading, such as temperature, time, stirring rate, Ibuprofen/SBA-15 ratio. The results of drug delivery experiments showed that the surface modification of SBA-15 with amino groups significantly increases the drug loading and decreases the drug delivery rate.
Introduction
Over the past three decades, there has been rapid growth in the area of drug delivery which is due to the underlying principle that drug delivery technology can bring both commercial and therapeutic values to health care products (Song et al., Citation2005).
Controlled drug release systems consist of matrix carriers which the drug is released under appropriate conditions. Carriers must satisfy several perquisites, such as (Yang et al., Citation2008) excellent biocompatibility (Wang, Citation2009), high loading/encapsulation amount of the drug (Slowing et al., Citation2008), zero premature release of drug molecules (Vallet-Regi et al., Citation2001), cell type or tissue specificity and site directing ability, and (Gonzalez et al., Citation2009) proper controlled release rate of drug molecules to achieve an effective local concentration, to serve as efficient drug release systems (Slowing et al., Citation2008).
Mesoporous silica nanoparticles (MSNs) have aroused special attention in the biomedical research field due to its great advantages in many aspects such as well biocompatible, unique properties of tunable pore size and structure, large surface areas and pore volumes, controllable morphology and modifiable surfaces, high chemical and thermal stabilities (Vallet-Regi et al., Citation2001; Qu et al., Citation2006; Urata et al., Citation2011; Yang et al., Citation2011; Wu & Yamauchi, Citation2012). Especially, they have a supremely potential application in drug delivery systems, for example, carriers for various guest molecules with therapeutic efficacy (e.g. anticancer drugs (Slowing et al., Citation2007), proteins (Lu et al., Citation2007), genes (Radu et al., Citation2004a; Torney et al., Citation2007), antibiotics and nonsteroidal anti-inflammatory drugs (Vallet-Regi et al., Citation2007)).
The discovery of triblock copolymer template SBA-15 with large, controlled pore size and highly ordered hexagonal topology has opened the way to do intriguing experiments inside the resulting channel structures (Zhao et al., Citation1998). By anchoring various functional groups on the internal surfaces, the sorption capacity and behavior of SBA-15 could be substantially altered. These properties of SBA-15 have led to some intriguing applications, such as selective adsorption of heavy or noble metal (Liu et al., Citation2000; Kang et al., Citation2004), enzyme immobilization for biocatalysis (Deere et al., 2003; Lei et al., Citation2004; Vinu et al., Citation2004) and immobilization of large chelating groups (Corriu et al., Citation2002).
Considering its potential application in the area of drug delivery, there have been attempts recently to apply pure SBA-15 as a drug host (Doadrio et al., Citation2004; Xue & Shi, Citation2004). However, for pure SBA-15, there exist only silanol groups on the channel walls, and these silanol groups simply form weak intermolecular hydrogen bonds with drugs; hence, they are not strong enough to hold drugs and allow them to be released in a sustained manner. The need to synthesize suitable carriers to have specific host–guest interactions with drugs led us to introduce functional groups on the surface of SBA-15 (Song et al., Citation2005).
SBA-15 and its functionalized products are well suited for drug delivery systems for many drugs, such as Ibuprofen (Song et al., Citation2005; Tang et al., Citation2006; Limnell et al., Citation2007; Yang et al., Citation2009; Xu et al., Citation2009) and some other drugs (Yang et al., Citation2005; Xu et al., Citation2008; Yu & Zhai, Citation2009). Various functional groups are introduced including amino alkyl (Huh et al., Citation2003, Citation2005; Radu et al., Citation2005), cyan alkyl (Huh et al., Citation2003, Citation2005; Radu et al., Citation2005), epoxy alkyl (Radu et al., Citation2004b), metal-amino alkyl (Yu & Zhai, Citation2009), etc. Various functionalized SBA-15 materials and model drugs can form the host–guest materials by surface interaction including ionic bond reaction and hydrogen bonding effect (Yang et al., Citation2009). These surface interactions could achieve a slow-release effect to tackle the problem of drug burst release. Meanwhile, the issue of site-selective is solved excellently (Xu et al., Citation2010).
In this article, Ibuprofen was selected as a model drug for loading and release studies. It is a well-known, nonsteroidal anti-inflammatory drug and has been widely used for the treatment of inflammation, pain or rheumatism. But this drug has a short biological half-life (2 h) (Rainsford, Citation1999), which makes it a suitable candidate for sustained or controlled drug delivery. Amine-functionalized SBA-15 was applied as a carrier for adsorption of Ibuprofen with acidic character. This study is a part of a comparative research on drug delivery systems using silica carriers. The factors affecting the drug loading, such as pH, temperature and drug concentration as well as the release behavior, were investigated.
Experimental
Materials
Poly (ethylene glycol)-block-poly (propylene glycol)-block-poly (ethylene glycol) [Pluronic P123, [(EO)20(PO)70(EO)20]] was purchased from Aldrich (Germany). Tetraethyl orthosilicate (TEOS) and (3-aminopropyl)triethoxysilane (APTES), toluene, n-hexane, ethanol and dichloromethane were purchased from Merck (Germany). Ibuprofen was purchased from DarouPakhsh Co., Iran. Toluene and n-hexane were refluxed over sodium with benzophenone as an indicator and distilled under nitrogen atmosphere before use. Dichloromethane was dried by distilling over P2O5 under nitrogen atmosphere. Distilled water was used during the experiments.
Synthesis of SBA-15
SBA-15 material was prepared according to the procedure reported by Zhao et al. (Zhao et al., Citation1998), using Pluronic 123 as a structure-directing agent and TEOS as silica source. In a typical preparation, 4.0 g of Pluronic was dissolved in 30.0 g of water and 120.0 g of 2 M HCl solution with stirring at 35 °C. Then 8.5 g of TEOS was added into the solution. After 20 h the mixture was aged at 80 °C over night under static conditions. The solid product was filtered off, washed and air-dried at room temperature. The resultant sample is denoted as SBA-15-SY.
Then, the template was removed from the as-made mesoporous material by soxhlet extraction with ethanol (SBA-15-S) or calcination at 550 °C for 6 h (SBA-15). Synthesis methods are shown in .
Surface functionalization
To functionalization of SBA-15, 3.0 g of silica was placed in a 100-mL round bottom flask with 50 mL of dry toluene and 2.4 g of 3-aminopropyltriethoxysilane (3-APS). The mixture was stirred and refluxed at 110 °C for 48 h under the protection of nitrogen. The white 3-aminopropyl functional silica washed with toluene, n-hexane and dichloromethane, respectively, and dried at 25 °C for 12 h. This material is denoted as SBA-15-NH2. Surface functionalization of the sample is shown schematically in .
Drug loading
The loading of Ibuprofen was achieved by liquid-phase grafting method. To optimize the drug loading, certain amounts of mesoporous material were saturated with n-hexane solution containing 30 mg/cm3 of Ibuprofen at different temperatures, times, Ibuprofen/silica ratio and stirring rates. Finally, Ibuprofen-loaded samples were centrifuged (750 rpm, 5 min); the residue was washed with n-hexane and oven-dried for at least 3 h at 80 °C. The percent of drug loading was achieved by Equation (1):
where m1 is the initial weight of SBA-15, m2 is the weight of drug loaded SBA-15 and m3 is the initial weight of drug in the solution.
In vitro release measurements
To study the release of Ibuprofen from SBA-15, the loaded powders were immersed in simulated body fluid (SBF) of pH = 7.2–7.4, under magnetic stirring in an interpenetrating membrane. Samples (2 mL) were taken at given interval times while 2 mL of the fresh buffer was added to replace the extracted volume. The samples were analyzed by UV spectrophotometer at 224 nm. The concentration of released drug in SBF at each interval is evaluated by Equation (2);
where Cn, CN, V1 and Vt are the actual concentration of drug, the apparent concentration of drug, the volume of the sample taken in each time period and the total volume of the release medium, respectively. The cumulative release (CR %) is determined by the Equation (3);
Physicochemical characterization
X-ray diffraction data were obtained with D/max-3BX diffractometer using Cu-kα radiation of wavelength 0.154 nm. Nitrogen adsorption–desorption isotherms were obtained at 77 K using an OMNISORP (TM) 100CX VER 1G adsorption apparatus. Samples were outgassed at 473 K for at least 8 h in vacuum prior to measurements. UV-Vis spectroscopy measurements were done by Shimadzu UV-1650PC UV-Vis spectrophotometer. The FT-IR spectra of the samples were recorded with FT-IR (Perkin-Elmer, 580 B) in the range between 400 and 4000 cm−1 at room temperature from KBr pellets. The morphologies were recorded using a Scanning Electron Microscope (Cambridge S-360). The thermogravimetric measurements were performed by SDT 2960 instrument with a heating rate of 10 °C/min in air flow.
Results and discussion
Scanning electron microscopy
Scanning electron microscopy (SEM) image () reveals that the calcined SBA-15 sample consists of many rope-like domains with relatively uniform sizes of ∼1 µm. As shown in ), when we used soxhlet extraction method for template removal, the rope-like SBA-15 forms ordered arrays. But by calcinations, the SBA-15 particles set in arbitrary directions. By comparing ), it can be concluded that the soxhlet method can increase the agglomeration of the particles. So, we calcined the sample to reduce the agglomeration and complete template removal.
N2 adsorption–desorption isotherm
From ), it can be observed that the nitrogen adsorption/desorption isotherms of the SBA-15 were typical type IV isotherms according to the IUPAC classification, characteristic for mesoporous materials. Additionally, the size distribution is narrow and about 3.6 nm. summarizes the textural properties of the SBA-15 support derived from nitrogen adsorption-desorption isotherm plot and pore size distribution curve.
Figure 4. (A) N2 adsorption–desorption isotherms and (B) corresponding BJH pore size distribution curves of pure SBA-15.
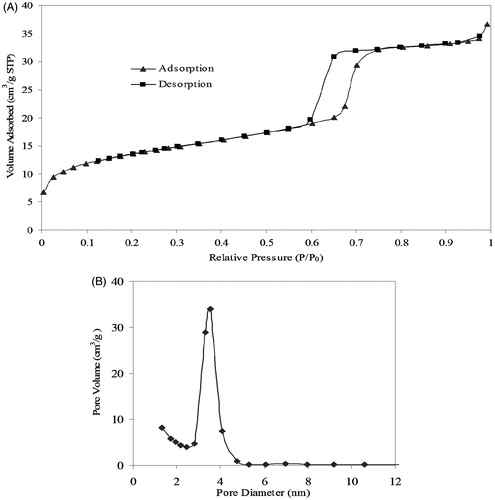
Table 1. Structural parameters of SBA-15.
X-ray diffraction
represents the XRD diffraction pattern within the wide angle ranges. The XRD patterns of SBA-15 exhibits four well-resolved peaks. The peaks can be indexed as (100), (110), (200) and (210) and associated with hexagonal symmetry (Lu et al., Citation2004). An XRD pattern indicates that the SBA-15 has an ordered hexagonal pore arrays. The intense (100) peak is associated with a d spacing of 87 Å, corresponding to a large unit cell parameter (a = 90 Å) for pure silica SBA-15 (Alexa et al., Citation2012).
FTIR spectra
shows the FTIR spectra of the uncalcined SBA-15 (SY), calcined SBA-15 and SBA-15-NH2 carriers before Ibuprofen loading. For all samples, the typical Si–O–Si bands around 1070–1220 cm−1 associated with the formation of a condensed silica network are present (Wang et al., Citation2012). The broad peak at 3400 cm−1 is attributed to the O–H stretching vibration mode. In uncalcined sample SBA-15 (SY), the peaks at 2850–3000 cm−1 and 1465 cm−1 are related to the C–H stretching and CH2 bending of Pluronic 123, respectively, which are not significantly observed in the calcined sample.
For SBA-15-NH2, C–H stretching bands of propyl groups can be observed at 2897 cm−1 and 2980 cm−1 (Kim et al., Citation2006; Xu et al., Citation2012). The band at 1540 cm−1, which is attributed to N–H stretching vibrations of aminopropyl anchored on the surface of mesoporous support, can be found in the FTIR spectra of SBA-15-NH2 but not in pure SBA-15. These characteristic bands confirm that the aminopropyl groups of APTES were introduced into SBA-15 (Lu et al., Citation2004).
Thermal gravimetric and differential thermal analysis
According to , the weight loss for all samples occurred in the temperature between 250 °C and 650 °C arose from the removal of the template molecules connected to the SBA-15 surface. The TGA curve of SBA-15 (SY) shows total weight loss of 54 wt%.
Figure 7. TGA curves of as synthesized, calcined, Soxhlet (2 days), Soxhelt (3 days) SBA-15 (A). DTA and TGA analysis of SBA-15-NH2 (B).
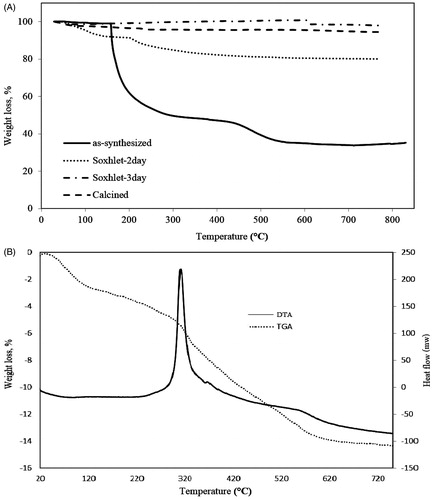
The TGA curve of SBA-15-S () indicates that the removal of the template cannot be completed after two days, but when the time of soxhlet extraction increased to three days, most of the organic materials were removed and the curve of TGA is resembled to the calcined SBA-15. Accordingly for these samples, weight loss is attributed to removal of adsorbed water molecules.
As shown in , aminopropyl group content of SBA-15-NH2 was determined by the weight loss measurement of the sample. The maximum weight loss occurred in the range of 250–700 °C, which is related to the gradual exit of the amine groups. The total weight loss for the SBA-15-NH2 is 14.25%. Furthermore, thermal analysis revealed that, for 1 g of SBA-15, 2.3 mmol APTES had been grafted.
Drug loading optimization
SBA-15-NH2 was saturated with n-hexane solution containing Ibuprofen. Drug loadings (%) were optimized at different temperatures, times and drug/silica gel ratios. According to , it is obvious that the soaking time is an important factor on drug loading efficiency. DL% increased with increasing time of soaking from 5 to 35 h. The loading appeared to reach a constant of 43 wt% after sufficient loading time (35 h). After 35 h, the surface of the SBA-15 was saturated with drug. So, all the subsequent experiments were performed at 35 h.
Figure 8. Effect of soaking time on drug loading efficiency while the other conditions were maintained (25 °C, n-hexane solvent).
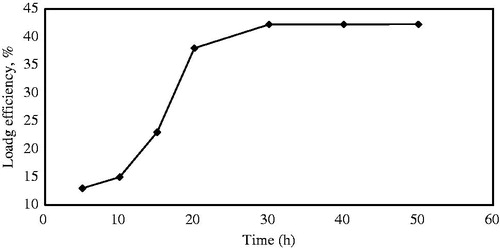
The drug loading efficiency was also influenced by the temperature of the loading medium. revealed that the loading was increased with increasing the temperature from 20 °C to 40 °C. This effect is attributed to an increase in the motion of drugs as well as the motion of the SBA-15 in such a way that interaction between the amine group of modified SBA-15 and the carboxyl group of drug are enhanced. The mild preparation process does not have any thermal/chemical destructive influence on the drug loading and the highest loading efficiency is achieved under this condition. Therefore, the following experiments were performed at 40 °C.
Figure 9. Effect of temperatures on loading efficiencies while the other conditions were maintained (35 h, n-hexane solvent).
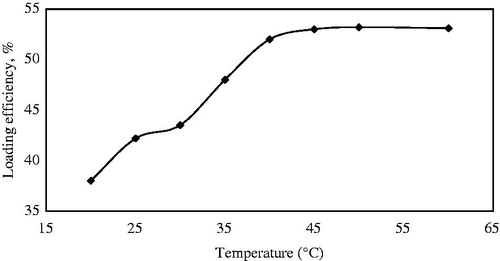
Different drug to SBA-15 ratios were selected to find the optimum drug used for loading conducted at 35 h and 40 °C. According to the results plotted in , the highest drug loading efficiency, that is, 50% was achieved using a drug loading of Ibuprofen/SBA-15 ratio of 50:100 (w/w).
Figure 10. The effect of Ibuprofen/SBA-15 ratio on loading efficiency while the other conditions were maintained (35 h, 40 °C, n-hexane solvent).
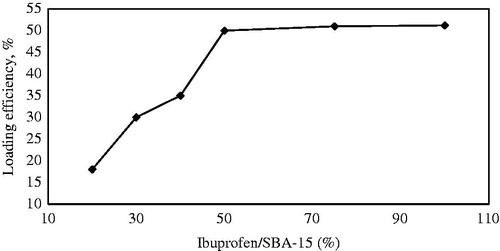
Accordingly, the loading was achieved at 40 °C, 35 h, with the stirring rate of 100 rpm and drug/SBA-15 ratio of 50:100. To show the effect of modifying procedures on drug loading, the drug-loading efficiencies were calculated in optimized conditions for different samples.
The results shown in indicate that the calcination and modifying procedure can enhance the drug loading percent. Hydrogen-bonded interaction between hydroxyl groups of SBA-15 and carboxyl groups in Ibuprofen plays an important role in the loading process. The pure SBA-15 material has only silanol groups on the surface, which simply form weak intermolecular hydrogen bonds with biological molecules/drugs. Hence, they are not strong enough to hold the drugs and allow them to release in a sustainable manner. Functionalization of mesoporous materials with amino groups has profound impact in both adsorption and drug delivery purpose. In our study, Ibuprofen was loaded on amino-functionalized SBA-15 for drug delivery purpose. Aminopropyl functional groups can improve the drug loading of SBA-15 by increasing the organophilic properties of the parent SBA-15 materials. As shown in , hydrogen-bonded interaction between amino groups of SBA-15-NH2 and carboxyl groups of Ibuprofen highly increased the drug loading percent to 51%. According to , in as-synthesized SBA-15, presence of the template in the mesoporouse structure resulted in lower drug loading efficiency.
Table 2. Drug loading efficiencies (%) of the synthesized samples.
In vitro release studies
The Ibuprofen release from the modified SBA-15 was studied in different pH. Hydrogen bonding between Ibuprofen and the parent mesoporous silica materials is relatively weak, so the mass transfer of Ibuprofen molecules through the channels is controlled by diffusion (Szegedi et al., Citation2011). The concentration of Ibuprofen released from SBA-15 in SBF medium as a function of pH was determined by UV-Vis spectroscopy by monitoring the changes in absorbance at wavelength of 264 nm at different pH. As shown in , the release profile is very low in acidic environment such as the stomach. But in the pH between 7.2 and 7.4, cumulative release of Ibuprofen reaches to a maximum 90%. So, the SBA-15/drug system is useful as an oral drug delivery system.
Influence of the carrier type on drug delivery
As shown in , in SBA-15 (SY) 40% of the drug released after 8 h. In the as-synthesized SBA-15, existence of a lot of organic compounds can lock the drug at the pores and inhibit the release of Ibuprofen fromSBA-15. The cumulative release profile of the SBA-15 indicates the burst release at the first 1 h. This phenomenon is as a result of that the template removal during calcination process can cause higher rate of release in SBA-15 at the first times. The presence of the template can inhibit the burst release so unmodified SBA-15 is not an appropriate carrier for sustained drug delivery. In the modified SBA-15, drug delivery happened over a long period of time (∼500 min) with a relatively constant rate.
Figure 13. Cumulative release (CR) % of Ibuprofen from (A) SBA-15 (SY), SBA-15 and SBA-15-NH2 untile 8 h, (B) SBA-15-NH2 at 260 h.
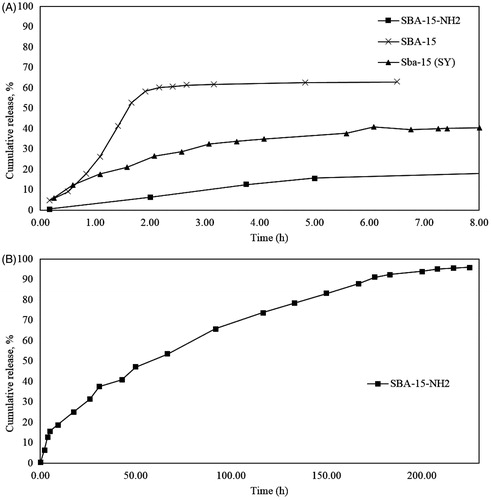
Accordingly, by comparing the drug delivery rate of pure SBA-15 and amine-modified one, it is obvious by functionalization of SBA-15 not only the amount of drug loading has been enhanced but also the drug delivery has been continued until the 95% of the loaded drug is released. Additionally, by comparing to SBA-15 (SY) and SBA-15, the burst release did not occur and it took a long time to release the Ibuprofen from SBA-15-NH2. This is due to interaction between Ibuprofen (COOH) and the functional groups of the amino-modified SBA-15 samples, which is stronger than that between silanol groups of the parent silica and Ibuprofen. This can explain the slower release rates of Ibuprofen from SBA-15-NH2 samples in comparison to the unmodified SBA-15.
Conclusion
Mesoporous SBA-15 has been synthesized and functionalized by APTES successfully. Then the loading efficiency optimized by changing the loading conditions such as time, temperature, drug to SBA-15 ratio. The best loading efficiency was achieved at 40 °C, 35 h, with the stirring rate of 100 rpm and drug to the SBA-15 ratio of 50%. Adsorption of Ibuprofen occurs with much better yields on the modified surface. Furthermore, the results showed that the Ibuprofen release can be controlled by the synthesis procedure and surface functionalization of the mesoporous silica. By functionalization of SBA-15 with amino groups the release rate becomes slower.
Declaration of interest
The authors report no conflicts of interest. The authors alone are responsible for the content and writing of this article.
References
- Alexa IF, Ignat M, Popovici RF, et al. (2012). In vitro controlled release of antihypertensive drugs intercalated into unmodified SBA-15 and MgO modified SBA-15 matrices. Int J Pharm 436:111–19
- Corriu RJP, Mehdi A, Reye C, Thieuleux C. (2002). Mesoporous hybrid materials containing functional organic groups inside both the framework and the channel pores. Chem Commun 13:1382–3
- Deere J, Magner E, Wall JG, Hodnett BK. (2003). Adsorption and activity of proteins onto mesoporous silica. Catal Lett 85:19–23
- Doadrio AL, Sousa EM, Doadrio JC, et al. (2004). Mesoporous SBA-15 HPLC evaluation for controlled gentamicin drug delivery. J Control Release 97:125–32
- Gonzalez B, Colilla M, Lopez de Laorden C, Vallet-Regi M. (2009). A novel synthetic strategy for covalently bonding dendrimers to ordered mesoporoussilica: potential drug delivery applications. Mater Chem 19:9012–24
- Huh S, Chen H-T, Wiench JW, et al. (2005). Cooperative catalysis by general acid and base bifunctionalized mesoporous silica nanospheres. Angew Chem Int Ed Engl 44:1826–30
- Huh S, Wiench JW, Yoo J-C, et al. (2003). Organic functionalization and morphology control of mesoporoussilicas via a co-condensation synthesis method. Chem Mater 15:4247–56
- Kang T, Park Y, Yi J. (2004). Highly selective adsorption of Pt2+ and Pd2+ using thiol-functionalized mesoporous silica. Ind Eng Chem Res 43:1478–84
- Kim H, Jung JC, Kim P, et al. (2006). Preparation of H3PMo12O40 catalyst immobilized on surface modified mesostructured cellular foam (SM-MCF) silica and its application to the ethanol conversion reaction. Mol Catal A: Chem 259:150–5
- Lei J, Fan J, Yu CZ, et al. (2004). Immobilization of enzymes in mesoporous materials: controlling the entrance to nanospace. Microporous Mesoporous Mater 73:121–8
- Limnell T, Riikonen J, Salonen J, et al. (2007). Surface chemistry and pore size affect carrier properties of mesoporous silicon microparticles. Int J Pharm 343:141–7
- Liu AM, Hidajat K, Kawi S, Zhao DY. (2000). A new class of hybrid mesoporous materials with functionalized organic monolayers for selective adsorption of heavy metal ions. Chem Commun 13:1145–6
- Lu J, Liong M, Zink JI, Tamanoi F. (2007). Mesoporous silica nanoparticles as a delivery system for hydrophobic anticancer drugs. Small 3:1341–6
- Lu Q, Gao F, Komarneni S, Mallouk TE. (2004). Ordered SBA-15 nano rod arrays inside a porous alumina membrane. Am Chem Soc 126:8650–1
- Qu FY, Zhu GS, Huang SY, et al. (2006). Effective controlled release of captopril by silylation of mesoporous MCM-41. Chem Phys Chem 7:400–6
- Radu DR, Lai C-Y, Huang J, et al. (2005). Fine-tuning the degree of organic functionalization of mesoporous silica nanosphere materials via an interfacially designed co-condensation method. Chem Commun 10:1264–6
- Radu DR, Lai CY, Jeftinija K, et al. (2004a). Capped mesoporous silica nanosphere-based gene transfection reagent. Am Chem Soc 126:13216–17
- Radu DR, Lai C-Y, Wiench JW, et al. (2004b). Gate keeping layer effect: a poly(lactic acid)-coated mesoporous silica nanosphere-based fluorescence probe for detection of amino-containing neurotransmitters. Am Chem Soc 126:1640–1
- Rainsford KD. (1999). Ibuprofen: a critical bibliographic review. London: Taylor & Francis
- Slowing II, Trewyn BG, Lin VSY. (2007). Nanoparticles for intracellular delivery of membrane-impermeable proteins. Am Chem Soc 129:8845–6
- Slowing II, Vivero-Escoto JL, Wu CW, Lin VSY. (2008). Mesoporous silica nanoparticles as controlled release drug delivery and gene transfection carriers. Adv Drug Delivery Rev 60:1278–88
- Song S-W, Hidajat K, Kawi S. (2005). Functionalized SBA-15 materials as carriers for controlled drug delivery: influence of surface properties on matrix-drug interactions. Langmuir 21:9568–75
- Szegedi A, Popova M, Goshev I, Mihály J. (2011). Effect of amine functionalization of spherical MCM-41 and SBA-15 on controlled drug release. Sol State Chem 184:1201–7
- Tang Q, Xu Y, Wu D, et al. (2006). Studies on a new carrier of trimethylsilyl-modified mesoporous material for controlled drug delivery. J Control Release 114:41–6
- Torney F, Trewyn BG, Lin VSY, Wang K. (2007). Mesoporous silica nanoparticles deliver DNA and chemicals into plants. Nat Nanotechnol 2:295–300
- Urata C, Yamada H, Wakabayashi R, et al. (2011). Aqueous colloidal mesoporous nanoparticles with ethenylene-bridged silsesquioxane frameworks. Am Chem Soc 133:8102–5
- Vallet-Regi M, Balas F, Arcos D. (2007). Mesoporous materials for drug delivery. Angew Chem Int Ed Engl 46:7548–58
- Vallet-Regi M, Ramila A, Del Real RP, Pérez-Pariente J. (2001). A new property of MCM-41: drug delivery system. Chem Mater 13:308–11
- Vinu A, Murugesan V, Hartmann M. (2004). Adsorption of lysozyme over mesoporous molecular sieves MCM-41 and SBA-15: influence of pH and aluminum incorporation. J Phys Chem B 108:7323–30
- Wang H, Gao X, Wang Y, et al. (2012). Effect of amine functionalization of SBA-15 on controlled baicalin drug release. Ceram Int 38:6931–5
- Wang SB. (2009). Ordered mesoporous materials for drug delivery. Microporous Mesoporous Mater 117:1–9
- Wu KCW, Yamauchi Y. (2012). Controlling physical features of mesoporous silica nanoparticles (MSNs) for emerging applications. Mater Chem 22:1251–6
- Xu W, Gao Q, Xu Y, et al. (2008). Controlled drug release from bifunctionalized mesoporous silica. J Solid State Chem 181:2837–44
- Xu W, Gao Q, Xu Y, et al. (2009). Controllable release of Ibuprofen from size-adjustable and surface hydrophobic mesoporous silica spheres. Powder Technol 191:13–20
- Xu Y, Wang C, Zhou G, et al. (2012). Improving the controlled release of water-insoluble emodin from amino-functionalized mesoporous silica. Appl Surf Sci 258:6366–72
- Xu Zh, Ji Y, Guan M, et al. (2010). Preparation and characterization of L-Leucine-modified amphiproticbifunctionalmesoporous SBA-15 molecular sieve as a drug carrier for ribavirin. Appl Surf Sci 256:3160–5
- Xue JM, Shi M. (2004). PLGA/mesoporous silica hybrid structure for controlled drug release. J Control Release 98:209–17
- Yang PP, Quan ZW, Lu LL, et al. (2008). Luminescence functionalization of mesoporous silica with different morphologies and applications as drug delivery systems. Biomaterials 29:692–702
- Yang Q, Wang S, Fan P, et al. (2005). pH-responsive carrier system based on carboxylic acid modified mesoporous silica and polyelectrolyte for drug delivery. Chem Mater 17:5999–6003
- Yang Y, Jia Y, Gao L, et al. (2011). Fabrication of autofluorescent protein coated mesoporous silica nanoparticles for biological application. Chem Commun 47:12167–9
- Yang Y-J, Tao X, Hou Q, Chen JF. (2009). Fluorescent mesoporous silica nanotubes incorporating CdS quantum dots for controlled release of Ibuprofen. Acta Biomater 5:3488–96
- Yu H, Zhai Q-Zh. (2009). Mesoporous SBA-15 molecular sieve as a carrier for controlled release of nimodipine. Micropor Mesopor Mater 123:298–305
- Zhao D, Feng J, Huo Q, et al. (1998). Triblock copolymer syntheses of mesoporous silica with periodic 50 to 300 angstrom pores. Science 279:548–52