Abstract
Herbal drugs have been used for thousands of years in the east and have had a recent resurgence in popularity among consumers in the west. However, most of herbal drug are poorly soluble and have hydrophobic properties and poor distribution, leading to reduced bioavailability and hence decreased treatment efficacy, requiring repeated administration or increased dose. In the past few decades, considerable attention has been focused on the development of self-emulsifying drug delivery system (SEDDS) for herbal drugs. SEDDS is isotropic and thermodynamically stable solutions consisting of oil, surfactant, co-surfactant and drug that can spontaneously form oil-in-water micro/nanoemulsion when mixed with water under gentle stirring. The formulation can be a viable alternative to classical formulations to take advantage of their lipophilic nature and to solve their problems of poor solubility, poor bioavailability, low oral absorption and instability. The mechanism of self-emulsification, solubility studies, construction of phase diagram, optimization and characterization of herbal drugs-loaded SEDDS formulation and in situ absorption evaluation of herbal drugs in rat intestine are presented in our article.
Introduction
As it described in , herbs are generally defined as any form of plants or plant products, including leaves, stems, flowers, roots and seeds (Goldman, Citation2001; Bent & Ko, Citation2004; Bent, Citation2008). Herbal drugs have been used for thousands of years in the east and have had a recent resurgence in popularity among consumers in the west (Eisenberg et al., Citation1998; Su & Li, Citation2011). Nowadays, 80% of the world’s population uses medicines, which are directly or indirectly derived from plants. Worldwide, such medicines make up a 25% share of the pharmaceutical arsenal (Bhattaram et al., Citation2002).
Extraction involves boiling or percolating the herb in water, alcohol or other solvents to release biologically active constituents of the plant. These liquid extracts may then be heated or dried to create more concentrated liquids, pastes or powders. Both the raw herb and the extract contain complicated mixtures of organic chemicals, which may include fatty acids, sterols, alkaloids, flavonoids, glycosides, saponins, tannins and terpenes (Bent & Ko, Citation2004). However, most of herbal active constituents are poorly soluble and have hydrophobic properties and poor distribution, leading to reduced bioavailability and hence decreased treatment efficacy, requiring repeated administration or increased dose. In the past few decades, considerable attention has been focused on the development of novel drug delivery system for herbal drugs.
In recently research, developing nanosized forms [polymeric nanoparticles (Yen et al., Citation2009; Cui et al., Citation2013), nanocapsules (Sierant et al., Citation2013), liposomes (Zhou et al., Citation2007; Khan et al., Citation2013), solid lipid nanoparticles (Li et al., Citation2011a) and nanoemulsions (Chen et al., Citation2004; Wei et al., Citation2012) have a number of advantages for herbal drugs, including enhancement of solubility and bioavailability, protection from toxicity, enhancement of pharmacological activity, enhancement of stability, improving tissue macrophages distribution, sustained delivery, protection from physical and chemical degradation (Khan et al., Citation2013). Thus the nanosized novel drug delivery systems of herbal drugs have a potential future for enhancing the activity and overcoming problems associated with herbal drugs (Cai et al., Citation2007; Ajazuddin & Saraf, Citation2010; Ma et al., Citation2012).
SEDDS has arisen as a potential carrier system for improving the bioavailability of poorly absorbed plant extracts/actives, such as mangiferin (Xuan et al., Citation2012), tanshinones (Wang et al., Citation2010), patchoulic alcohol (You et al., Citation2010) and lutin (Yoo et al., Citation2010). The Herbal drugs-loaded SM/NEDDS formulations are presented in . This formulation can be a viable alternative to classical formulations to take advantage of their lipophilic nature and to solve their problems of poor solubility, poor bioavailability, low oral absorption and instability. Several novel drug delivery systems for herbal medicine curcumin were investigated in our laboratory (Zhang et al., Citation2011, Citation2012; Gao et al., Citation2012; Wang et al., Citation2012; Zhao et al., Citation2012, Citation2013). By comparing the herbal drug-loaded systems, the SEDDS were considered to have remarkable advantages (the technology is simple and easy to direct production, higher drug loading, easy to storage in liquid and solid dosage forms) over the other formulations in deliverying herbal medicine. For example, the drug loading of curcumin in SMEDDS was 6.73 ± 0.92% (Zhang et al., Citation2012), was higher than that in liposome (2.33%) (Chen et al., Citation2012a), triblock copolymeric micelles (6.4 ± 0.02 %) (Song et al., Citation2011) and gelatin microspheres (6.15 ± 0.44%) (Cao et al., Citation2011). As it described in , SEDDS is an isotropic and thermodynamically stable solution consisting of oil, surfactant, co-surfactant and drug that can spontaneously form oil-in-water micro/nanoemulsion when mixed with water under gentle stirring. Owing to its miniscule globule size, the micro/nanoemulsified drug can easily be absorbed through lymphatic pathways, bypassing the hepatic first-pass effect (Cho & Park, Citation2013; Jakki et al., Citation2013). The pathway of herbal drug transport from SEDDS is presented in . “SEDDS” is a broad term, typically producing emulsions with a droplet size ranging from a few nanometers to several microns. “Self-microemulsifying drug delivery systems” (SMEDDS) indicates the formulations forming transparent microemulsions with oil droplets ranging between 100 and 250 nm. “Self-nanoemulsifying drug delivery systems” (SNEDDS) is a recent term construing the globule size range <100 nm (Kohli et al., Citation2010).
Figure 2. Schematic flowchart on the general strategy of formulating self-emulsifying systems and their subsequent conversion to micro/nano emulsions.
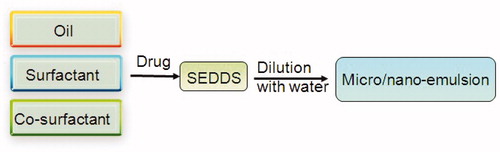
Table 1. Herbal drugs-loaded SM/NEDDS formulation.
Compared to conventional metastable emulsions, S(M/N)EDDS is a thermodynamically stable formulation with high solubilization capacity for lipophilic drugs, and also can be filled directly into soft or hard gelatin capsules for convenient oral administration. At present, there are four drug products, Sandimmune® and Sandimmun Neoral® (Cyclosporine A, Novartis Pharmaceuticals Corporation, Basel, Switzerland), Norvir® (Ritonavir, Abbott Laboratories, Abbott Park, IL), and Fortovase® (Saquinavir, Hoffmann-La Roche ltd., Basel, Switzerland) on the pharmaceutical market, the active compounds of which have been formulated into specific SEDDS (Gursoy & Benita, Citation2004).
After the investigation of two herbal drugs (curcumin and apigenin) loaded SMEDDS (Zhang et al., Citation2012; Zhao et al., Citation2013), we were interested in this new drug delivery system for tremendously increasing the solubility of herbal drugs. Therefore, we review the application of SEDDS in herbal drugs in recent years to provide some basic ideas to the readers which are interesting in herbal drug-loaded SEDDS.
Just like the chemical drugs-loaded SEDDS, herbal drug-loaded SEDDS are also important in the investigation of SEDDS. The SEDDS had offered a great opportunity and hope in improving the solubility in water and in vivo bioavailability of herbal drugs. Therefore, our manuscript reviewed literatures on herbal drug-loaded SEDDS which were published in the past few years. In our manuscript, we emphasized the SEDDS had a very good prospect in pharmaceutics for these lipophilic herbal drugs by presenting the mechanism of self-emulsification, preparation, construction of phase diagram, in vivo and in vitro assessment of SEDDS.
Composition of SEDDS
Self-emulsification has been shown to be specific to: the nature of the oil/surfactant pair; the surfactant concentration and oil/surfactant ratio and the temperature at which self-emulsification occurs. In support of these facts, it has also been demonstrated that only very specific pharmaceutical excipient combinations could lead to efficient self-emulsifying systems.
Oils
The oil represents one of the most important excipients in the SEDDS formulation not only because it can solubilize marked amounts of the lipophilic herbal drug or facilitate self-emulsification but also and mainly because it can increase the fraction of herbal drug transported via the intestinal lymphatic system, thereby increasing absorption from the gastrointestinal (GI) tract. Both long and medium chain triglyceride oils with different degrees of saturation have been used for the design of self-emulsifying formulations. Unmodified medium-chain triglycerides (MCT) provide the most “natural” basis for lipid vehicles. MCTs are preferred in the earlier self-emulsifying formulations (Charman et al., Citation1992) because of higher fluidity, better solubility properties and self-emulsification ability. However, they are not frequently preferred in this regard owing to their poor ability to dissolve large amounts of lipophilic herbal drugs. Modified long- and medium-chain triglyceride oils, with varying degrees of saturation or hydrolysis, have been used widely for the design of herbal drugs-loaded SEDDS (Mekjaruskul et al., Citation2013). Natural oils (such as castor oil, corn oil, olive oil, soybean oil and peanut oil) are also usually used to enhance the solubility of herbal drugs. However, their ability to solubilize herbal drugs cannot catch up with some medical liquid glycerin series (Labrafac™ PG, Maisine™ 35-1, Labrafac™, Lipophile WL 1349 and capryol™ 90), which can greatly improve the solubility of herbal drugs. For example, in the solubility studies of curcumin, Capryol™ 90 and Labrafac™ PG afforded higher solubility of curcumin (42.34 ± 0.27 mg/ml and 20.24 ± 0.15 mg/ml, respectively) compared with corn oil (7.58 ± 0.09 mg/ml) (Setthacheewakul et al., Citation2010). The type of oil affect the phase behavior of SEDDS. In the investigation of Warisnoicharoen et al. (Citation2000), it indicated that the larger molecular volume oils (soybean oil, Miglyol 812 and ethyl oleate) were solubilized to a lower extent than the smaller molecular volume oils (namely, ethyl butyrate and ethyl caprylate).
Surfactants
Nonionic surfactants were used to develop the SMEDDS formulation as they are less toxic compared to ionic surfactants, more compatible with biological systems and less affected by pH and ionic strength. The selection of surfactants for using in a SEDDS formulation is based on their emulsification efficiency rather than their ability to solubilize the drug. The efficiency of self-emulsification is related to the hydrophilic–lipophilic balance (HLB) value of the surfactant (Thi et al., Citation2009). Generally, surfactants with HLB 12–15 are regarded as being of good efficiency. Considering the safety and biocompatibility of the excipient, several nonionic surfactants, such as Cremophor EL (HLB 12–14), Cremophor RH40 (HLB 12–14), Tween 80 (HLB 15) and Labrasol (HLB 14) are commonly used in herbal drug-loaded SEDDS (Cui et al., Citation2005; Xi et al., Citation2009; Chen et al., Citation2012b; Zhao et al., Citation2013).
Co-surfactants
The addition of co-surfactant aids in the formation of fine droplets, increasing the solubility of hydrophobic drugs and improves the stability of the micro/naoemulsion through the reduction of the interfacial tension. Organic solvents, suitable for oral administration (1,2-propylene glycol, polyethylene glycol (PEG) and Transcutol HP) may help to dissolve large amounts of either the hydrophilic surfactant or the drug in the lipid base(Cui et al., Citation2009; Xie et al., Citation2009; Zhang et al., Citation2012). It is important to note that, alcohol and other volatile co-surfactants have the disadvantage of evaporating into the shell of soft or hard gelatin capsules, leading to precipitation of drug.
Mechanism of self-emulsification
Formulation of interfacial film-liquid crystal
In earlier work, it was suggested that the ease of emulsification could be associated with the ease by which water penetrates into the various liquid crystal (LC) or gel phases formed on the surface of the droplet (Groves et al., Citation1974; Rang & Miller, Citation1999). According to the research, the addition of a binary mixture (oil/non-ionic surfactant) to water results in interface formation between the oil and aqueous-continuous phases, followed by the solubilization of water within the oil phase owing to aqueous penetration through the interface. This will occur until the solubilization limit is reached close to the interface. Further aqueous penetration will result in the formation of the dispersed LC phase. As the aqueous penetration proceeds, eventually all material close to the interface will be LC, the actual amount depending on the surfactant concentration in the binary mixture. Once formed, rapid penetration of water into the aqueous cores, aided by the gentle agitation of the self-emulsification process, causes interface disruption and droplet formation. The high stability of these self-emulsified systems to coalescence is considered to be due to the LC interface surrounding the oil droplets.
Thermodynamics theory
The mechanism, by virtue of which self-emulsification tends to occur has not yet been thoroughly elucidated. Nevertheless, it has been suggested that self-emulsification occurs when the entropy change that favors dispersion is greater than the energy required to increase the surface area of the dispersion (Gershanik & Benita, Citation2000; Nazzal et al., Citation2002). In addition, the free energy of a conventional emulsion formation is a direct function of the energy required to create a new surface between the oil and water phases. The thermodynamic relationship for the net energy change is described by Equation (Equation1(1) ).
(1)
where ΔG is the free energy associated with the process (ignoring the free energy of mixing), N is the number of droplets, r is the radius of droplets and the σ represents the interfacial energy. With time, the two phases of the emulsion will tend to separate, in order to reduce the interfacial area, and subsequently, the free energy of the systems. Therefore, the emulsions resulting from aqueous dilution are stabilized by conventional emulsifying agents, which form a monolayer around the emulsion droplets, and hence, reduce the interfacial energy, as well as providing a barrier to coalescence.
Solubility studies
The self-emulsifying formulations consisting of oil, surfactants, co-surfactants and the herbal drug should form a clear and monophasic liquid at ambient temperature when introduced into an aqueous phase, and should have good solvent properties to allow presentation of the herbal drug in solution (Shahnaz et al., Citation2011). In a SEDDS formulation, the drug loading is determined by the solubility of the herbal drug in the oil and surfactant phases of the system (Chen et al., Citation2012b; Huang et al., Citation2013). Therefore, identification of oil, surfactant/co-surfactant that has maximum solubilization potential for the herbal drug under investigation is important to determine optimum drug loading (Ma et al., Citation2012; Huang et al., Citation2013; Mekjaruskul et al., Citation2013). To test solubility of herbal drug in single component, the solubility studies are usually carried out as the following steps: “An excess amount of herbal drug was introduced to a certain volume or weight of each excipient and the mixture was shaken in an selected vehicle vial at 37 °C for 48 h (or 72 h). After equilibrium was achieved, the mixture was centrifuged using a centrifuge. The concentration of the herbal drug was then determined by HPLC analysis”. The vehicles provided higher solubility of the herbal drugs are selected for further investigations for formulating the SEDDS (Shao et al., Citation2010).
Application of construction of phase diagram in herbal medicine
Phase diagrams are constructed to obtain the optimum concentrations of oil, surfactant and co-surfactant, as well as getting the proportion of components that can result in maximum micro/nanoemulsion existence area. SEDDS form fine oil–water micro/nanoemulsions with only gentle agitation, upon its introduction into aqueous media. Since the free energy required to form an emulsion is very low, the formation is thermodynamically spontaneous. Surfactants form a layer around the emulsion droplets and reduce the interfacial energy as well as providing a mechanical barrier to coalescence. The visual test is measured the apparent spontaneity of emulsion formation. The series of SEDDS were prepared and their self-emulsifying properties were observed visually (Kang et al., Citation2004). The vehicles (oils, surfactants and co-surfactants) provided higher solubility of the herbal drugs were selected for constructing the phase diagram without drugs (blank phase diagram). Pseudo-ternary phase diagrams and ternary phase diagrams are used in the investigation of blank SEDDS which are shown in (Li et al., Citation2011b; Zhang et al., Citation2012).
Figure 4. Ternary phase diagrams (A and B) and pseudo-ternary phase diagrams (C) used in self-emulsifying drug delivery system.
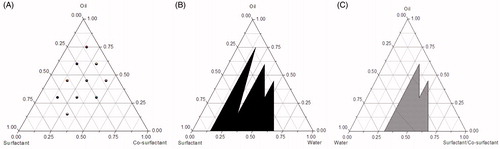
The pseudo-ternary phase diagrams are constructed by titration of homogenous liquid mixtures of oil, surfactant and co-surfactant with water at room temperature (Djordjevic et al., Citation2004; Subramanian et al., Citation2004; Desai et al., Citation2012). Pseudo-ternary phase diagrams of oil, surfactant/co-surfactant and water were usually developed using the water titration method (Beg et al., Citation2013; Singh et al., Citation2013). The surfactant and co-surfactant were weighed precisely and mixed at mass ratios of 9:1, 8:2, 7:3, 6:4, 5:5, 4:6, 3:7, 2:8 and 1:9. For each pseudo-ternary phase diagram at a specific surfactant/co-surfactant weight ratio, the oily mixtures of oil, surfactant and co-surfactant were prepared with the weight ratio of oil to the mixture of surfactant and co-surfactant at 9:1, 8:2, 7:3, 6:4, 5:5, 4:6, 3:7, 2:8 and 1:9, respectively. The mixture was homogeneously mixed using a vortex mixer. Water was then added drop-by-drop using a dropper into each oily mixture. During the titration, samples were stirred vigorously for a sufficient length of time for homogenization and visually monitored against a dark background by illuminating the samples with white light. The concentration of water at which turbidity-to-transparency and transparency-to-turbidity transition occurred was derived from the weight measurements. These values were then used to determine the boundaries of the micro/nanoemulsion regions corresponding to the selected optimum ratios of combination vehicles for developing SEDDS. In a current study of persimmon leaf extract (PLE) loaded SNEDDS, pseudo-ternary phase diagrams were constructed by Micro Plate Dilution (PDMPD) method, based on the water titration method, was a novel for phase diagram construction (Li et al., Citation2011b). In brief, the mixtures of oil, surfactant and co-surfactant at certain weight ratios were gradually diluted with water in a microtitre plate (96 wells, 350 μl volumes each). The microtitre plates were filled by pipette in accordance with the filling scheme: the mixture was added starting from A1 with 200 μl, A2 with 190 μl, continuing to B8 with 10 μl, decreasing 10 μl each well and the water phase was then added from A2 with 10 μl, A3 with 20 μl, continuing to B9 with 200 μl, increasing 10 μl each well. The wells C1–D9 were filled with the next batch using the same procedure. The plates were then sealed with their associated lids and eddied for 10 min. The turbidity of each well by Multiskan Ascent at 630 nm was detected to provide basis for drawing pseudo-ternary phase diagrams.
The ternary phase diagrams were constructed by oil, surfactant and co-surfactant at room temperature (Shah et al., Citation2013). A series of self-emulsifying systems were prepared with various weight percentages of oil, surfactant and co-surfactant as follows: (oil:surfactant:co-surfactant = 0.15:0.15:0.70, 0.15:0.30:0.55,…,0.75:0.15:0.1). The mixture (1 g) was introduced into 100 ml of distilled water in a beaker at room temperature and the contents were mixed gently with a magnetic stir bar (Basalious et al., Citation2010). The tendency to emulsify spontaneously and the progress of emulsion droplets spread were visually assessed using the grading system (Shafiq et al., Citation2007; Zhao et al., Citation2010). If a clear or slightly bluish micro/nanoemulsion is rapidly formed within 1 min will be labeled as “A”, if a bright white emulsion is formed within 2 min, the region will be labeled as “B”. However, if a dull, grayish white emulsion with a slightly oil appearance (i.e. a formulation exhibiting either poor or minimal emulsification with large oil droplets floating on the surface) is formed slowly (i.e. longer than 2 min), the composition for this formulation will be labeled as“C” in the phase diagram. Both A and B are considered to meet the criterion for self-emulsification. Phase diagrams were constructed by identifying the A and B self-micro/nanoemulsifying region using the software of Origin 7.5.
Optimization of herbal drug-loaded SEDDS
Simplex lattice method was used to optimize the formulation of herbal drug-loaded SEDDS (Zhang et al., Citation2012; Zhao et al., Citation2013). This design is extremely useful in preliminary studies where the aim is to identify formulation variables that can be used in further investigation (Subramanian et al., Citation2004; Zhu et al., Citation2008). In this design, the total concentration of oil, surfactant and co-surfactant in the formulation was kept constant while the ratio of the three was varied. The simplex lattice experiment design for a three-component system was represented by an equilateral triangle in two-dimensional space. As shown in , seven batches of SEDDS were prepared as follows: three vertexes (A, B, C), each of them represented a formulation containing the maximum amount of one component, with the other two components at minimum; three halfway points between vertices (AB, BC, AC), which showed a formulation containing the average of the minimum and maximum amounts of the two ingredients represented by two vertices; the center point (ABC), which represented a formulation containing one-third of each ingredient. In the investigation of Cui et al. (Citation2009), simplex lattice method was used to optimize the formulation of curcumin-loaded SMEDDS. The concentrations of surfactant (X1), co-surfactant (X2) and oil (X3) were chosen as the independent variables. The solubility of curcumin in SMEDDS (Y1) and the mean particle size (Y2) of formed microemulsion by diluting SMEDDS with distil water were taken as responses, respectively. The equation for simplex lattice model was described as follows:
(2)
The responses for seven formulations were used to fit an equation for simplex lattice model (Patel et al., Citation2007) which then can predict the properties of all possible formulations. Graphs of these properties in the form of contour plots were constructed with Matlab software. With the aid of Matlab software, the model equation was developed to be the best explanation for the relationship between the solubility and particle size characteristics. Based on the two equations (Y1 and Y2), contour plots of solubility and mean particle size were constructed when oil phase was set as an appropriate value. The common area between Y1 and Y2 which was higher in particle size and solubility was the best choosing area for the concentrations for surfactant and co-surfactant. Then the formulation with higher solubility and appropriate particle size was confirmed.
Central composite design (CCD) was also used to optimize the formulation of baicalein-loaded SMEDDS (Liu et al., Citation2012). Based on the preliminary experiments, two formulation parameters, the oil percentage (X1) and Sur/Cos ratio (X2), were identified as key factors responsible for the properties of SMEDDS. Droplet size (Y1) and equilibrium solubility (Y2) are selected as the responses because they are generally considered as significant factors for assessing the qualities of SMEDDS. A two-factor, four-level CCD was undertaken to investigate the main effects and the interactions of the two factors on the two responses.
The data obtained for the two responses were fitted to classical second-order polynomial models, which were represented as follows:
(3)
where Y corresponds to predicted response, X1 and X2 correspond to the studied factors, b0 is an intercept and b1–b5 are regression coefficient.
In the investigation of Liu et al. (Citation2012), a central composite design was used to investigate the influence of factors (oil percentage (X1) and surfactant to co-surfactant ratio [Sur/Co-s ratio) (X2)] on the responses including droplet size (Y1), polydispersity (Y2), equilibrium solubility (Y3) and in situ intestine absorption rate (Y4). A two-factor, five-level CCD was undertaken to investigate the main effects and the interactions of the two factors on the four responses. The data obtained for the four responses in each trial were fitted to classical second-order polynomial model and third-order quadratic model. The mathematical models were expressed as follows:
The second-order polynomial model was same as Equation (Equation3(3) ).
The third-order quadratic model was described as Equation (Equation4(4) ).
(4)
Data were analyzed by nonlinear estimation using STATISTICA 6.0 software. F-test was used to evaluate lack of fit within each model and identify the fitting model. Response surface delineation was performed according to the fitting model. Graphs of surface responses were plotted with the response variation against the two factors. Further optimization was carried out by the following desirability function.
In vitro assessment of herbal drug-loaded SEDDS
The main means of self-emulsification assessment is visual evaluation. The various ways to characterize SEDDS are listed as bellows.
Morphology
The morphology of herbal drug-loaded SEDDS was observed using a transmission electron microscope (TEM). SEDDS was diluted with distilled water and mixed by slightly shaking. One drop of diluted samples was deposited on a film-coated copper grid and then stained with one drop of 2% aqueous solution of phosphotungstic acid (PTA), allowing to dry before observation under the electron microscope (Setthacheewakul et al., Citation2010).
Equilibrium solubility
SEDDS formulations should have good solvent properties to allow presentation of drug in solution. Both oil percentage and Sur/Cos ratio influence the solubility of drug in SEDDS to some extent (Shahba et al., Citation2012). The equilibrium solubility of oridonin was determined as the following method: the blank formulation (absence of drug) was prepared with the composition of SMEDDS (Zhang et al., Citation2008). Excess oridonin was added to each blank SMEDDS and the mixtures were stirred for 48 h at 37 °C in a thermostatically controlled water bath until they reached equilibrium. The samples were centrifuged at 2500 × g for 20 min. The supernatant was filtered through a membrane filter (0.45 μm). The obtained filtrate was diluted with methanol. The concentration of oridonin was determined by HPLC. The result showed that the effect of oil percentage on the oridonin solubility in the formulation was highly significant, whereas Sur/Cos ratio played a less role.
Droplet size measurement
Droplet size is an important parameter in the development of SEDDS as it influences the rate of drug release as well as their in vivo stability. Smaller droplets have a greater surface area, which subsequently increases the rate of drug release (Lee et al., Citation2009; Ofokansi et al., Citation2009). In addition, most of herbal drug-loaded SEDDS were found to be transparent after dilution with water. Photon correlation spectroscopy, microscopic techniques or a Coulter Nanosizer are mainly used for the determination of the emulsion droplet size. The small droplet size may be due to the increased surfactant concentration, which forms a closed packed film of surfactant at the interface, thereby stabilizing the dispersed droplets (Venkatesh et al., Citation2010).
The interaction of surfactant and co-surfactant played an important role in droplet size of SEDDS. Li et al. (Citation2011b) concluded that the droplet size of persimmon leaf extract was related to the ratios of surfactant and co-surfactant. The droplet size had an enlarging trend on the whole when the ratio of co-surfactant was increased.
The effect of drug loading on particle size in distilled water is presented in the investigation of Shen & Zhong (Citation2006). The mean size increased slightly with increased daidzein loading concentration from 1% to 3%. When drug loading further increased, particle size increased dramatically, even to as high as 100 nm or over, which was beyond the range of colloidal system and no more presented the properties of microemulsion. It could be thought that undissolved drug in the formulation affected the mean droplet size to increase. In another study of curcumin-loaded folate modified self-microemulsion drug delivery system (FSMEDDS), the droplets size was significantly increased with the incorporation of F-PEG-CHEMS in the system (p < 0.05). The long PEG-spacer in F-PEG-CHEMS dispersing into the emulsifying membrane layer (composed of surfactant and co-surfactant) might lead to the increase of the droplet size. It was observed that the addition of curcumin in the SMEDDS and FSMEDDS increased significantly the size of the droplet obtained after diluting with distilled water (p < 0.05) (Zhang et al., Citation2012).
The effect of dilution on droplet size in distilled water was measured by Zhang et al. (Citation2008). When the dilution time was 1000-fold, the droplet size seemed to be unchanged, which revealed that the microemulsion formed upon dilution was as large as 1000 times capable of keeping oridonin solubilized.
Zeta potential
The stability of emulsion is directly related to the charge present on mobile surface, which is termed as zeta potential (Singh et al., Citation2009; Xiao et al., Citation2013). Zeta potential is an important parameter, indicating the electrostatic repulsion and congregation of the oily droplets (Goyal et al., Citation2012; Gursoy & Cevik, Citation2013). The electrostatic forces of the emulsion droplets are vital for assessing the stability of the systems. High electrostatic repulsive forces between the droplets can prevent coalescence of the fine emulsion droplets into large oily globules, whereas low electrostatic repulsive forces can result in phase separation (Nekkanti et al., Citation2010). The addition of herbal drug into SEDDS may change the zeta potential due to the structures of drugs and SEDDS. As shown in the study of Zhang et al. (Citation2012), the absolute zeta potential value of curcumin-loaded SMEDDS was significantly higher than that of the blank SMEDDS (p < 0.05). The possible reason was that the formation of intermolecular hydrogen bonds among the hydroxyl groups in curcumin and some relative groups containing oxygen or nitrogen atoms in surfactant, co-surfactant and oil produced the change in the surface electrostatic double layer of droplets.
In situ absorption evaluation of herbal drug in rat intestine
Intestinal absorption has been recognized as a critical factor affecting the bioavailability of oral delivery of herbal drugs. Intestinal absorption is affected by many factors including the physicochemical property of the herbal drug, the absorption mechanism and the need for absorption enhances (Li et al., Citation2006). It is clear that the accurate intestinal permeability for herbal drug is difficult to directly study in human. It is also unrealistic to predict the ability of a drug molecule to cross the intestinal barrier from simple physicochemical measurements such as pKa, molecular size and partition coefficient. Therefore a number of in vitro and in situ experimental models have been developed which determine the intestinal absorptive potential of a drug and the mechanism of absorption. Among these methods single-pass intestinal perfusion (SPIP) and intestinal recirculating perfusion are the most frequently used techniques which provide conditions closer to what is faced following oral administration (Grassi & Cadelli, Citation2001). The single-pass perfusion technique facilitates the study of rapid absorption and is suitable for drugs that are rapidly absorbed. In the recirculating perfusion model, the probability of absorption is considerably increased because of the longer retention time of the solution in the intestine (Grassi & Cadelli, Citation2001; Luo et al., Citation2013). Thus, it is recommended that drugs which are absorbed comparatively slowly as shown by single-pass perfusion techniques are reexamined using recirculating perfusion to magnify the concentration changes (Zhou et al., Citation2008; Luo et al., Citation2013; Singh et al., Citation2013).
Intestinal recirculating perfusion
Male adult rats weighing 200 ∼ 250 g were usually used for intestinal recirculating perfusion studies. Animals were housed under standard conditions of light and dark cycles with free access to food and water, acclimated for at least 3 days, and fasted 12 h before the experiment. Rats were fasted overnight with free access to water before the experiment. The rat was anesthetized by intraperitoneal injection of 1% sodium pentobarbital (0.4 ml/100 g body weight) and was placed under an infrared lamp to keep normal body temperature. Upon verification of the loss of pain reflex, a 5-cm-long midline longitudinal incision was made, the intestinal segment of interest was located and PVC tubing was cannulated. The outlet tubing was then cannulated ∼10 cm aboral to the first opening. Both of the cannulae were secured with surgical silk sutures. The selected intestinal segment was then gently rinsed with prewarmed saline (37 ± 1 °C) and attached to the perfusion assembly. Then it was rinsed with physiological saline and rinsed by air, followed by being connected to a constant-flow pump with the catheters to the prefusion system. The schematic diagram was described in . Care was taken to handle the small intestine gently and to minimize the surgery in order to maintain an intact blood supply. The entire surgical area was covered with a piece of sterilized absorbent gauze wetted with normal saline. At the beginning of the test, sample solution was perfused through the intestine at a flow rate of 5 ml/min. Ten minutes later, the volume of perfusion solution in the circulation system as the 0 min volume was recorded and the flow rate was adjusted to 2.5 ml/min. During 6-h perfusion period, at predetermined time interval, 1 ml of sample solution was taken out, the volume of solution in the circulation system was recorded, and then 2 ml Krebs-Rings solution was added in. Samples were frozen immediately and stored at −20 °C until analysis. The perfusion solution was Krebs-Rings buffer consisting of NaCl (128.5 mM), KCl (4.7 mM), MgCl2 (2.3 mM), CaCl2 (3.3 mM),NaH2PO4ċ2H2O (1.87 mM), NaHCO3 (16.3 mM) and glucose (7.8 mM), which was adjusted to pH 6.8 with 1.0 M phosphoric acid. The absorption constant (Ka) is calculated using Fick’s equation:
(5)
where X0 is the amount of drug before absorption, X is the residue amount of drug after absorption. Ka can be obtained as the slope from the regression curve of −ln (X/X0) versus time, and t1/2 can be obtained when X is equal to X0/2.
Figure 6. Schematic representation of intestinal recirculating perfusion technique as employed for the self-emulsifying drug delivery system formulation in rat.
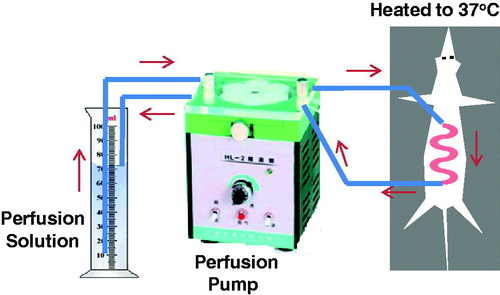
In order to investigate transport mechanism of curcumin in SMEDDS, the method of perfusion in rat intestine was used in the present study because it can give a reliable prediction of oral absorption of drug in humans (Cui et al., Citation2009). The intestinal absorption kinetics of microemulsification of SMEDDS with low (25 mg/kg), middle (50 mg/kg), high dose (100 mg/kg) was investigated. The results showed there was not a significant difference (p > 0.05) in absorption parameters such as Ka, t1/2 and uptake percentage among variable doses of SMEDDS. From absorption parameters among different doses or concentrations in perfusing solutions, curcumin-loaded SMEDDS demonstrated as a concentration-independent absorption in rat’s intestine, which might contribute to intestinal absorption of curcumin in SMEDDS via passive transfer by diffusion across the lipid membranes. In rat intestinal perfusing experiment, a dye (e.g. phenol red) was added into the sample solution as a non-absorbed marker. However, phenol red was reported to be partly absorbed in small intestine and may interfere with the transport of some definite drugs, especially the poorly water-soluble drugs (Kunze & Vogt, Citation1967; Gotoh et al., Citation1996; Yamamoto et al., Citation1996). In order to overcome the disadvantages, many simply methods such as gravimetric determination and direct-volume recording were adopted (Jain et al., Citation2007). In this article, the residue drug was calculated by recording volume of perfusion solution in circulation system directly at predetermined intervals. Our investigation showed the error between volume values by recording directly and real volumes of perfusion solution at predetermined intervals was <3%.
Single-pass intestinal perfusion
Sections of intestine from anesthetized rats are isolated, cannulated, perfused with drug solution and sampled for analysis (Lane et al., Citation2006; Ho et al., Citation2008). To maintain normal body temperature (36 °C ∼ 37 °C), the rats are placed on a heated slide warmer under a heating lamp. Breathing is facilitated by the introduction of aplastic tube into the trachea during surgery. It is important to maintain adequate circulation during surgery and to keep the exposed segment moist with the saline maintained at body temperature (Yao et al., Citation2008; Liu et al., Citation2009).
The intestinal permeability of nobiletin was studied as a function of concentration in each segment of the intestine to find out the best absorption sites (Yao et al., Citation2008). After an overnight fast, male SD rats weighing ∼180–220 g were anesthetized with an i.p. injection of 20% (w/v) urethane solution (urethane: 1 g/kg). To maintain normal body temperature, rats were placed on a warming pad under a surgical lamp. The abdomen was opened with a midline incision, an interest intestinal segment of ∼10 cm was chosen and cannulated with plastic tubing. Initially, the intestinal segment was rinsed with isotonic saline (37 °C) until the outlet solution was clear. Using an infusion pump, the intestinal segments were perfused at a flow rate of 0.2 ml/min with the bland Krebs-Ringer’s buffer solution. The preparation time took ∼30 min. Then the pump was connected to the reservoir containing the nobiletin-loaded SMEDDS dilutions with the marker (Phenol Red). Setting t = 0 when the buffer solution has been completely pushed out. Each perfusion experiment lasted for 180 min and the perfusate was quantitatively collected in the following intervals: 0–60, 60–75, 75–90, 90–105, 105–120, 120–135,135–150, 150–165 and 165–180 min. Samples were stored in a refrigerator at 4 °C until analysis by HPLC. According to effective permeation coefficient (Peff) values in each intestinal segment, the Peff at each concentration in the colon was significantly higher than those in other segments.
Application of solid self-emulsifying drug system in herbal medicine
SEDDS are normally prepared either as liquids or encapsulated in soft gelatin capsules, which have some shortcomings especially in the manufacturing process, leading to high production costs (Jiang & Mo, Citation2010). Furthermore, SEDDS can be absorbed onto porous particles that can be manufactured as granules, pellets and tablets (Beg et al., Citation2012, Citation2013; Hu et al., Citation2012). These solid SMEDDS can be used for delivering poorly water-soluble drug such as herbal drugs (puerarin, lycopene and rographolide) (Yu et al., Citation2006; Faisal et al., Citation2013; Sermkaew et al., Citation2013). A high content of liquid SEDDS can be loaded (up to 70%) onto a carrier, which not only maintains good flow ability but also enables the production of tablets with good cohesive properties and good content uniformity in both capsules and tablets. This clearly expands the options available to the formulator. In terms of functionality and performance, the solubilizing properties of the solid SEDDS should remain unaffected by both the adsorption of the liquid SEDDS onto a carrier and the state of the drug in the lipid SEDDS (solubilized versus suspended). This technique offers formulators an additional option in the quest to achieve product performance, product design and manufacturability (Yi et al., Citation2008; Kohli et al., Citation2010; Oh et al., Citation2011). Until now, solid self-emulsifying systems have been already produced by loading liquid self-emulsifying systems on solid carriers using different technologies: extrusion/spheronization (Newton et al., Citation2001), co-extrusion (Iosi et al., Citation2011), wet granulation in high shear mixer (Franceschinis et al., Citation2005) and spray drying (Christensen et al., Citation2001; Dollo et al., Citation2003; Yi et al., Citation2008).
The extrusion/spheronization process is commonly used in the pharmaceutical industry to make uniformly sized pellets (Setthacheewakul et al., Citation2011). Pellet, as a multiple unit dosage form, possess many advantages such as flexibility of manufacture, reducing intrasubject and intersubject variability of plasma profiles and minimizing GI irritation without lowering drug bioavailability (Gandhi et al., Citation1999; Bolcskei et al., Citation2013). Thus, it is very appealing to combine the advantages of pellets with those of SEDDS. Iosi et al. (Citation2011) develop new solid self-emulsifying pellets to deliver milk thistle extract (silymarin). These pellets were prepared via extrusion/spheronization procedure, using a self-emulsifying system or SES (Akoline MCM® (AarhusKarlshamn, Sweden), Miglyol (Sasol GmbH Oleochemicals, Witten, Germany), Tween 80® (Seppic, Castres, France)), microcrystalline cellulose and lactose monohydrate. These preliminary technological and pharmacokinetic data demonstrated that extrusion/spheronization is a viable technology to produce self-emulsifying pellets of good quality and able to improve in vivo oral bioavailability of main components of a phytotherapeutic extract of >100 times by enhancing the lymphatic route of absorption. In another study, curcumin-SMEDDS pellets were also prepared by the extrusion/spheronization technique (Setthacheewakul et al., Citation2010).
Spray drying is an important technology for the preparation of solid SMEDDS for the oral route (Yan et al., Citation2011). This technique involves the preparation of a formulation by mixing lipids, surfactants, drug, solid carriers and solubilization of the mixture before spray drying. Liquid formulation is then atomized into a spray of droplets. The droplets are introduced into a drying chamber, where the volatile phase (e.g. the water contained in an emulsion) evaporates, forming dry particles under controlled temperature and airflow conditions. S-SNEDDS containing phosphatidylcholine was prepared for the delivery of lutein by spray drying using Aerosil 200 VV Pharma as the inert solid carrier. Briefly, colloidal silica suspension was prepared by suspending Aerosil 200 VV Pharma (500 mg) in 100 ml ethanol by magnetic stirring. One milliliter of SNEDDS containing lutein (4% w/w) was then added into the polymer suspension with constant stirring, and homogenous suspension was obtained by stirring the above mixture at room temperature for 15 min. The resultant suspension was then spray dried in a laboratory mini spray B-190 apparatus (Buchi, Switzerland) utilizing inlet temperature of 60 °C, outlet temperature of 35 °C and aspiration of ∼85%. The feeding rate of the suspension was set to 5 ml/min. The resultant powder was collected from the apparatus and measured for the final drug content using a validated HPLC method. Reconstitution evaluation of S-SNEDDS demonstrated excellent self-emulsification properties similar to SNEDDS. The bioavailability study in rabbits showed significantly enhanced bioavailability of lutein from S-SNEDDS compared with phosphatidylcholine (Shanmugam et al., Citation2011).
Improvement of solubility and oral bioavailability of herbal drugs by SEDDS
Herbal drugs with low aqueous solubility present a major challenge during formulation as their high hydrophobicity prevents them from being dissolved in most of approved solvents. The novel synthetic hydrophilic oils and surfactants usually in SEDDS dissolve herbal drugs to a greater extent than conventional vegetable oils. The release of the drug compound from SEDDS takes place upon its partitioning into the intestinal fluids during droplet transport and disintegration along the GI tract. It was proposed that two main factors, small particle size and polarity of the resulting oil droplets determine the efficient release of the drug compound from SEDDS (Mandawgade et al., Citation2008; Shen et al., Citation2010).
Ginkgo biloba extract (GBE) has become a widely used herbal remedy for increasing cognitive function in elderly people. The research found that GBE-loaded SEDDS could efficiently improve oral absorption of the sparingly soluble drugs by rapid self-emulsification and subsequently dispersion in the absorption sites. The optimized formulation for bioavailability assessment consisted of 45% Tween 80-Cremophor EL35 (1:1, w/w), 10% 1,2-propanediol and 45% ethyl oleate. The in vitro dissolution rates of the active components of GBE-SEDDS form were significantly faster than those of the GBE tablets. After single oral administration of 800 mg GBE as SEDDS or tablets to fasted dogs, the relative bioavailability of SEDDS for bilabolide and ginkgolide A and B was 162.1%, 154.6% and 155.8% compared with the reference tablets, respectively (Tang et al., Citation2008). Another herbal drug Fructus Schisandral Chinensis (Wurenchun) has also been formulated as SEDDS for improved solubility and bioavailability. The optimized formulation for bioavailability assessment consisted of 20% oleic acid, 65% Tween 20 and 15% Transcutol P. The mean droplet size distribution of the optimized SEDDS was ∼240 nm when diluted with 1000-fold volume of the distilled water. The in vitro dissolution rates of the active components of Wurenchun SEDDS were significantly higher than those of the commercial capsules. SEDDS have significantly increased the Cmax and area under the curve (AUC) of Wurenchun compared to reference capsules (p < 0.05). And the relative bioavailability of SEDDS for schisandrin and schisandrin B was 292.2% and 205.8% compared to the commercial capsules, respectively (Shao et al., Citation2010). All of the results suggested the potential promising of SEDDS for the efficient delivery of the sparingly soluble drugs such as herbal drugs.
Conclusion
Most of herbal drugs are poorly soluble and have hydrophobic properties and poor distribution, leading to reduced bioavailability and hence decreased treatment efficacy, requiring repeated administration or increased dose. SEDDS is a promising approach for the formulation of herbal drugs with poor aqueous solubility. An extensive research is going on the area of SEDDS and targeting for herbal drugs. Furthermore, SEDDS can be absorbed onto porous particles that can be manufactured as granules, pellets and tablets. These solid SEDDS can be used for delivering herbal drugs. Moreover, GI irritation is avoidable and controlled/sustained release of drug is achievable. Even though it is an effective technique, here also some disadvantages are there, like lack of good predicative in vitro models for assessment of the formulations, and higher concentrations of surfactant to be used may causes some allergic reactions. Further development should be based on in vitro–in vivo correlations and more attention should be paid to research the excipients and preparation technology to reduce their toxicity, enhance their activity and provide more suitable dosage forms to satisfy the needs of clinical application. At present, the SEDDS of the herbal medicine mainly focus on single natural active ingredient, few research are involved in the effective parts of herbal drugs and Chinese medicinal prescription. Further study should pay attention to this field.
Declaration of interest
The authors report no conflicts of interest in this work.
References
- Ajazuddin, Saraf S. (2010). Applications of novel drug delivery system for herbal formulations. Fitoterapia 81:680–9
- Basalious EB, Shawky N, Badr-Eldin SM. (2010). SNEDDS containing bioenhancers for improvement of dissolution and oral absorption of lacidipine. I: development and optimization. Int J Pharm 391:203–11
- Beg S, Jena SS, Patra Ch N, et al. (2013). Development of solid self-nanoemulsifying granules (SSNEGs) of ondansetron hydrochloride with enhanced bioavailability potential. Colloids Surf B Biointerfaces 101:414–23
- Beg S, Swain S, Singh HP, et al. (2012). Development, optimization, and characterization of solid self-nanoemulsifying drug delivery systems of valsartan using porous carriers. AAPS PharmSciTech 13:1416–27
- Bent S. (2008). Herbal medicine in the United States: review of efficacy, safety, and regulation: grand rounds at University of California, San Francisco Medical Center. J Gen Intern Med 23:854–9
- Bent S, Ko R. (2004). Commonly used herbal medicines in the United States: a review. Am J Med 116:478–85
- Bhattaram VA, Graefe U, Kohlert C, et al. (2002). Pharmacokinetics and bioavailability of herbal medicinal products. Phytomedicine 9:1–33
- Bolcskei E, Regdon G Jr, Sovany T, et al. (2013). Preparing of pellets by extrusion/spheronization using different types of equipment and process conditions. Drug Dev Ind Pharm. Apr 18. [Epub ahead of print]. doi:10.3109/03639045.2013.783588
- Cai Q, Liang L, Huang YP, Hou SX. (2007). In vitro evaluation of self-emulsifying drug delivery system of volatile oil from rhizome of Ligusticum chuanxiong. Zhongguo Zhong Yao Za Zhi 32:2003–7
- Cao F, Ding B, Sun M, et al. (2011). Lung-targeted delivery system of curcumin loaded gelatin microspheres. Drug Deliv 18:545–54
- Charman SA, Charman WN, Rogge MC, et al. (1992). Self-emulsifying drug delivery systems: formulation and biopharmaceutic evaluation of an investigational lipophilic compound. Pharm Res 9:87–93
- Chen H, Chang X, Weng T, et al. (2004). A study of microemulsion systems for transdermal delivery of triptolide. J Control Release 98:427–36
- Chen H, Wu J, Sun M, et al. (2012a). N-trimethyl chitosan chloride-coated liposomes for the oral delivery of curcumin. J Liposome Res 22:100–9
- Chen LJ, Liu Y, Li L, Gao F. (2012b). Enhanced bioavailability of total paeony glycoside by self-microemulsifying drug delivery system. Yao Xue Xue Bao 47:1678–86
- Cho YD, Park YJ. (2013). In vitro and in vivo evaluation of a self-microemulsifying drug delivery system for the poorly soluble drug fenofibrate. Arch Pharm Res. Jun 11. [Epub ahead of print]. doi:10.1007/s12272-013-0169-4
- Christensen KL, Pedersen GP, Kristensen HG. (2001). Preparation of redispersible dry emulsions by spray drying. Int J Pharm 212:187–94
- Cui J, Yu B, Zhao Y, et al. (2009). Enhancement of oral absorption of curcumin by self-microemulsifying drug delivery systems. Int J Pharm 371:148–55
- Cui S, Zhao C, Chen D, He Z. (2005). Self-microemulsifying drug delivery systems (SMEDDS) for improving in vitro dissolution and oral absorption of Pueraria lobata isoflavone. Drug Dev Ind Pharm 31:349–56
- Cui Y, Xu Q, Chow PK, et al. (2013). Transferrin-conjugated magnetic silica PLGA nanoparticles loaded with doxorubicin and paclitaxel for brain glioma treatment. Biomaterials 34:8511–20
- Desai J, Khatri N, Chauhan S, Seth A. (2012). Design, development and optimization of self-microemulsifying drug delivery system of an anti-obesity drug. J Pharm Bioallied Sci 4:S21–2
- Djordjevic L, Primorac M, Stupar M, Krajisnik D. (2004). Characterization of caprylocaproyl macrogolglycerides based microemulsion drug delivery vehicles for an amphiphilic drug. Int J Pharm 271:11–19
- Dollo G, Le Corre P, Guerin A, et al. (2003). Spray-dried redispersible oil-in-water emulsion to improve oral bioavailability of poorly soluble drugs. Eur J Pharm Sci 19:273–80
- Eisenberg DM, Davis RB, Ettner SL, et al. (1998). Trends in alternative medicine use in the United States, 1990–1997: results of a follow-up national survey. JAMA 280:1569–75
- Faisal W, Ruane-O’Hora T, O’Driscoll CM, Griffin BT. (2013). A novel lipid-based solid dispersion for enhancing oral bioavailability of Lycopene – in vivo evaluation using a pig model. Int J Pharm 453:307–14
- Franceschinis E, Voinovich D, Grassi M, et al. (2005). Self-emulsifying pellets prepared by wet granulation in high-shear mixer: influence of formulation variables and preliminary study on the in vitro absorption. Int J Pharm 291:87–97
- Gandhi R, Lal Kaul C, Panchagnula R. (1999). Extrusion and spheronization in the development of oral controlled-release dosage forms. Pharm Sci Technolo Today 4:160–70
- Gao Y, Wang C, Sun M, et al. (2012). In vivo evaluation of curcumin loaded nanosuspensions by oral administration. J Biomed Nanotechnol 8:659–68
- Gershanik T, Benita S. (2000). Self-dispersing lipid formulations for improving oral absorption of lipophilic drugs. Eur J Pharm Biopharm 50:179–88
- Goldman P. (2001). Herbal medicines today and the roots of modern pharmacology. Ann Intern Med 135:594–600
- Gotoh S, Nakamura R, Nishiyama M, et al. (1996). Effects of protease inhibitors on the absorption of phenol red and fluorescein isothiocyanate dextrans from the rat intestine. J Pharm Sci 85:858–62
- Goyal U, Arora R, Aggarwal G. (2012). Formulation design and evaluation of a self-microemulsifying drug delivery system of lovastatin. Acta Pharm 62:357–70
- Grassi M, Cadelli G. (2001). Theoretical considerations on the in vivo intestinal permeability determination by means of the single pass and recirculating techniques. Int J Pharm 229:95–105
- Groves MJ, Mustafa RM, Carless JE. (1974). Phase studies of mixed phosphated surfactants, n-hexane and water. J Pharm Pharmacol 26:616–23
- Gursoy RN, Benita S. (2004). Self-emulsifying drug delivery systems (SEDDS) for improved oral delivery of lipophilic drugs. Biomed Pharmacother 58:173–82
- Gursoy RN, Cevik O. (2013). Design, characterization and in vitro evaluation of SMEDDS containing an anticancer peptide, linear LyP-1. Pharm Dev Technol. May 16. [Epub ahead of print]. doi:10.3109/10837450.2013.795170
- Ho YF, Lai MY, Yu HY, et al. (2008). Application of rat in situ single-pass intestinal perfusion in the evaluation of presystemic extraction of indinavir under different perfusion rates. J Formos Med Assoc 107:37–45
- Hu X, Lin C, Chen D, et al. (2012). Sirolimus solid self-microemulsifying pellets: formulation development, characterization and bioavailability evaluation. Int J Pharm 438:123–33
- Huang Y, Tian R, Hu W, et al. (2013). A novel plug-controlled colon-specific pulsatile capsule with tablet of curcumin-loaded SMEDDS. Carbohydr Polym 92:2218–23
- Iosi T, Voinovich D, Perissutti B, et al. (2011). Oral bioavailability of silymarin phytocomplex formulated as self-emulsifying pellets. Phytomedicine 18:505–12
- Jain R, Duvvuri S, Kansara V, et al. (2007). Intestinal absorption of novel-dipeptide prodrugs of saquinavir in rats. Int J Pharm 336:233–40
- Jakki R, Afzal Syed M, Kandadi P, Veerabrahma K. (2013). Development of a self-microemulsifying drug delivery system of domperidone: In vitro and in vivo characterization. Acta Pharm 63:241–51
- Jiang YN, Mo HY. (2010). Preparation of self-emulsifying soft capsule and its pharmacokinetic in rats for epimedium flavonoids. Zhong Yao Cai 33:767–71
- Kang BK, Lee JS, Chon SK, et al. (2004). Development of self-microemulsifying drug delivery systems (SMEDDS) for oral bioavailability enhancement of simvastatin in beagle dogs. Int J Pharm 274:65–73
- Khan J, Alexander A, Ajazuddin, Saraf S. (2013). Recent advances and future prospects of phyto-phospholipid complexation technique for improving pharmacokinetic profile of plant actives. J Control Release 168:50–60. doi:10.1016/j.fitote.2010.05.001 and doi:10.1016/j.jconrel.2013.02.025
- Kohli K, Chopra S, Dhar D, et al. (2010). Self-emulsifying drug delivery systems: an approach to enhance oral bioavailability. Drug Discov Today 15:958–65
- Kunze H, Vogt W. (1967). Absorption of phenol red from the rat small intestine. Naunyn Schmiedebergs Arch Exp Pathol Pharmakol 256:139–48
- Lane ME, Levis KA, Corrigan OI. (2006). Effect of intestinal fluid flux on ibuprofen absorption in the rat intestine. Int J Pharm 309:60–6
- Lee BS, Kang MJ, Choi WS, et al. (2009). Solubilized formulation of olmesartan medoxomil for enhancing oral bioavailability. Arch Pharm Res 32:1629–35
- Li S, Ji Z, Zou M, et al. (2011a). Preparation, characterization, pharmacokinetics and tissue distribution of solid lipid nanoparticles loaded with tetrandrine. AAPS PharmSciTech 12:1011–18
- Li W, Yi S, Wang Z, et al. (2011b). Self-nanoemulsifying drug delivery system of persimmon leaf extract: optimization and bioavailability studies. Int J Pharm 420:161–71
- Li YH, Zhang M, Wang JC, et al. (2006). Effects of absorption enhancers on intestinal absorption of lumbrokinase. Yao Xue Xue Bao 41:939–44
- Liu W, Tian R, Hu W, et al. (2012). Preparation and evaluation of self-microemulsifying drug delivery system of baicalein. Fitoterapia 83:1532–9
- Liu Y, Zhang P, Feng N, et al. (2009). Optimization and in situ intestinal absorption of self-microemulsifying drug delivery system of oridonin. Int J Pharm 365:136–42
- Luo Z, Liu Y, Zhao B, et al. (2013). Ex vivo and in situ approaches used to study intestinal absorption. J Pharmacol Toxicol Methods 68:208–16
- Ma H, Zhao Q, Wang Y, et al. (2012). Design and evaluation of self-emulsifying drug delivery systems of Rhizoma corydalis decumbentis extracts. Drug Dev Ind Pharm 38:1200–6
- Mandawgade SD, Sharma S, Pathak S, Patravale VB. (2008). Development of SMEDDS using natural lipophile: application to beta-Artemether delivery. Int J Pharm 362:179–83
- Mekjaruskul C, Yang YT, Leed MG, et al. (2013). Novel formulation strategies for enhancing oral delivery of methoxyflavones in Kaempferia parviflora by SMEDDS or complexation with 2-hydroxypropyl-beta-cyclodextrin. Int J Pharm 445:1–11
- Nazzal S, Smalyukh, II, Lavrentovich OD, Khan MA. (2002). Preparation and in vitro characterization of a eutectic based semisolid self-nanoemulsified drug delivery system (SNEDDS) of ubiquinone: mechanism and progress of emulsion formation. Int J Pharm 235:247–65
- Nekkanti V, Karatgi P, Prabhu R, Pillai R. (2010). Solid self-microemulsifying formulation for candesartan cilexetil. AAPS PharmSciTech 11:9–17
- Newton M, Petersson J, Podczeck F, et al. (2001). The influence of formulation variables on the properties of pellets containing a self-emulsifying mixture. J Pharm Sci 90:987–95
- Ofokansi KC, Chukwu KI, Ugwuanyi SI. (2009). The use of liquid self-microemulsifying drug delivery systems based on peanut oil/tween 80 in the delivery of griseofulvin. Drug Dev Ind Pharm 35:185–91
- Oh DH, Kang JH, Kim DW, et al. (2011). Comparison of solid self-microemulsifying drug delivery system (solid SMEDDS) prepared with hydrophilic and hydrophobic solid carrier. Int J Pharm 420:412–18
- Patel DM, Patel NM, Pandya NN, Jogani PD. (2007). Gastroretentive drug delivery system of carbamazepine: formulation optimization using simplex lattice design: a technical note. AAPS PharmSciTech 8:E1--E5
- Rang MJ, Miller CA. (1999). Spontaneous emulsification of oils containing hydrocarbon, nonionic surfactant, and oleyl alcohol. J Colloid Interface Sci 209:179–92
- Sermkaew N, Ketjinda W, Boonme P, et al. (2013). Liquid and solid self-microemulsifying drug delivery systems for improving the oral bioavailability of andrographolide from a crude extract of Andrographis paniculata. Eur J Pharm Sci 50:459--66
- Setthacheewakul S, Kedjinda W, Maneenuan D, Wiwattanapatapee R. (2011). Controlled release of oral tetrahydrocurcumin from a novel self-emulsifying floating drug delivery system (SEFDDS). AAPS PharmSciTech 12:152–64
- Setthacheewakul S, Mahattanadul S, Phadoongsombut N, et al. (2010). Development and evaluation of self-microemulsifying liquid and pellet formulations of curcumin, and absorption studies in rats. Eur J Pharm Biopharm 76:475–85
- Shafiq S, Shakeel F, Talegaonkar S, et al. (2007). Development and bioavailability assessment of ramipril nanoemulsion formulation. Eur J Pharm Biopharm 66:227–43
- Shah SR, Parikh RH, Chavda JR, Sheth NR. (2013). Self-nanoemulsifying drug delivery system of glimepiride: design, development, and optimization. PDA J Pharm Sci Technol 67:201–13
- Shahba AA, Mohsin K, Alanazi FK. (2012). Novel self-nanoemulsifying drug delivery systems (SNEDDS) for oral delivery of cinnarizine: design, optimization, and in-vitro assessment. AAPS PharmSciTech 13:967–77
- Shahnaz G, Hartl M, Barthelmes J, et al. (2011). Uptake of phenothiazines by the harvested chylomicrons ex vivo model: influence of self-nanoemulsifying formulation design. Eur J Pharm Biopharm 79:171–80
- Shanmugam S, Baskaran R, Balakrishnan P, et al. (2011). Solid self-nanoemulsifying drug delivery system (S-SNEDDS) containing phosphatidylcholine for enhanced bioavailability of highly lipophilic bioactive carotenoid lutein. Eur J Pharm Biopharm 79:250–7
- Shao B, Tang J, Ji H, et al. (2010). Enhanced oral bioavailability of Wurenchun (Fructus Schisandrae Chinensis extracts) by self-emulsifying drug delivery systems. Drug Dev Ind Pharm 36:1356–63
- Shen H, Zhong M. (2006). Preparation and evaluation of self-microemulsifying drug delivery systems (SMEDDS) containing atorvastatin. J Pharm Pharmacol 58:1183–91
- Shen Q, Li X, Yuan D, Jia W. (2010). Enhanced oral bioavailability of daidzein by self-microemulsifying drug delivery system. Chem Pharm Bull (Tokyo) 58:639–43
- Sierant M, Paluch P, Florczak M, et al. (2013). Photosensitive nanocapsules for use in imaging from poly(styrene-co-divinylbenzene) cross-linked with coumarin derivatives. Colloids Surf B Biointerfaces 111C:571–8
- Singh B, Bandopadhyay S, Kapil R, et al. (2009). Self-emulsifying drug delivery systems (SEDDS): formulation development, characterization, and applications. Crit Rev Ther Drug Carrier Syst 26:427–1
- Singh B, Singh R, Bandyopadhyay S, et al. (2013). Optimized nanoemulsifying systems with enhanced bioavailability of carvedilol. Colloids Surf B Biointerfaces 101:465–74
- Song Z, Feng R, Sun M, et al. (2011). Curcumin-loaded PLGA-PEG-PLGA triblock copolymeric micelles: preparation, pharmacokinetics and distribution in vivo. J Colloid Interface Sci 354:116–23
- Su D, Li L. (2011). Trends in the use of complementary and alternative medicine in the United States: 2002–2007. J Health Care Poor Underserved 22:296–310
- Subramanian N, Ray S, Ghosal SK, et al. (2004). Formulation design of self-microemulsifying drug delivery systems for improved oral bioavailability of celecoxib. Biol Pharm Bull 27:1993–9
- Tang J, Sun J, Cui F, et al. (2008). Self-emulsifying drug delivery systems for improving oral absorption of ginkgo biloba extracts. Drug Deliv 15:477–84
- Thi TD, Van Speybroeck M, Barillaro V, et al. (2009). Formulate-ability of ten compounds with different physicochemical profiles in SMEDDS. Eur J Pharm Sci 38:479–88
- Venkatesh G, Majid MI, Mansor SM, et al. (2010). In vitro and in vivo evaluation of self-microemulsifying drug delivery system of buparvaquone. Drug Dev Ind Pharm 36:735–45
- Wang S, Chen P, Zhang L, et al. (2012). Formulation and evaluation of microemulsion-based in situ ion-sensitive gelling systems for intranasal administration of curcumin. J Drug Target 20:831–40
- Wang S, Zhao S, Yang R, et al. (2010). Self-microemulsifying drug delivery system increasing solubility and intestinal absorption in situ of tanshinones. Zhongguo Zhong Yao Za Zhi 35:1119–22
- Warisnoicharoen W, Lansley AB, Lawrence MJ. (2000). Nonionic oil-in-water microemulsions: the effect of oil type on phase behaviour. Int J Pharm 198:7–27
- Wei L, Li G, Yan YD, et al. (2012). Lipid emulsion as a drug delivery system for breviscapine: formulation development and optimization. Arch Pharm Res 35:1037–43
- Xi J, Chang Q, Chan CK, et al. (2009). Formulation development and bioavailability evaluation of a self-nanoemulsified drug delivery system of oleanolic acid. AAPS PharmSciTech 10:172–82
- Xiao L, Yi T, Liu Y. (2013). A new self-microemulsifying mouth dissolving film to improve the oral bioavailability of poorly water soluble drugs. Drug Dev Ind Pharm 39:1284–90
- Xie Y, Rong R, Li G, et al. (2009). Studies on self-microemulsifying drug preparations of total flavones of Hippophae rhamnoides. Zhongguo Zhong Yao Za Zhi 34:43–6
- Xuan XY, Wang YJ, Tian H, et al. (2012). Study on prescription of self-microemulsifying drug delivery system of mangiferin phospholipid complex. Zhong Yao Cai 35:1508–11
- Yamamoto A, Uchiyama T, Nishikawa R, et al. (1996). Effectiveness and toxicity screening of various absorption enhancers in the rat small intestine: effects of absorption enhancers on the intestinal absorption of phenol red and the release of protein and phospholipids from the intestinal membrane. J Pharm Pharmacol 48:1285–9
- Yan YD, Kim JA, Kwak MK, et al. (2011). Enhanced oral bioavailability of curcumin via a solid lipid-based self-emulsifying drug delivery system using a spray-drying technique. Biol Pharm Bull 34:1179–86
- Yao J, Lu Y, Zhou JP. (2008). Preparation of nobiletin in self-microemulsifying systems and its intestinal permeability in rats. J Pharm Pharm Sci 11:22–9
- Yen FL, Wu TH, Lin LT, et al. (2009). Naringenin-loaded nanoparticles improve the physicochemical properties and the hepatoprotective effects of naringenin in orally-administered rats with CCl(4)-induced acute liver failure. Pharm Res 26:893–902
- Yi T, Wan J, Xu H, Yang X. (2008). A new solid self-microemulsifying formulation prepared by spray-drying to improve the oral bioavailability of poorly water soluble drugs. Eur J Pharm Biopharm 70:439–44
- Yoo JH, Shanmugam S, Thapa P, et al. (2010). Novel self-nanoemulsifying drug delivery system for enhanced solubility and dissolution of lutein. Arch Pharm Res 33:417–26
- You X, Wang R, Tang W, et al. (2010). Self-microemulsifying drug delivery system of patchoulic alcohol to improve oral bioavailability in rats. Zhongguo Zhong Yao Za Zhi 35:694–8
- Yu AH, Zhai GX, Cui J, Liu H. (2006). Preparation of puerarin solid self-microemulsion. Zhong Yao Cai 29:834–8
- Zhang L, Cao F, Ding B, et al. (2011). Eudragit(R) S100 coated calcium pectinate microspheres of curcumin for colon targeting. J Microencapsul 28:659–67
- Zhang L, Zhu W, Yang C, et al. (2012). A novel folate-modified self-microemulsifying drug delivery system of curcumin for colon targeting. Int J Nanomed 7:151–62
- Zhang P, Liu Y, Feng N, Xu J. (2008). Preparation and evaluation of self-microemulsifying drug delivery system of oridonin. Int J Pharm 355:269–76
- Zhao L, Du J, Duan Y, et al. (2012). Curcumin loaded mixed micelles composed of Pluronic P123 and F68: preparation, optimization and in vitro characterization. Colloids Surf B Biointerfaces 97:101–8
- Zhao L, Zhang L, Meng L, et al. (2013). Design and evaluation of a self-microemulsifying drug delivery system for apigenin. Drug Dev Ind Pharm 39:662–9
- Zhao Y, Wang C, Chow AH, et al. (2010). Self-nanoemulsifying drug delivery system (SNEDDS) for oral delivery of Zedoary essential oil: formulation and bioavailability studies. Int J Pharm 383:170–7
- Zhou P, Li LP, Luo SQ, et al. (2008). Intestinal absorption of luteolin from peanut hull extract is more efficient than that from individual pure luteolin. J Agric Food Chem 56:296–300
- Zhou R, Zhu X, Hung G, et al. (2007). Preparation and quality evaluation of Iohexol liposomes. Sheng Wu Yi Xue Gong Cheng Xue Za Zhi 24:918–22
- Zhu W, Yu A, Wang W, et al. (2008). Formulation design of microemulsion for dermal delivery of penciclovir. Int J Pharm 360:184–90