Abstract
Context: Tuberculosis (TB) is a worldwide health concern. In 2011, about 8.7 million new cases developed TB and 1.4 million people died from it.
Objective: Enhancement of ethambutol hydrochloride activity and safety in treatment of TB through niosomal encapsulation.
Materials and methods: Niosomes were prepared by the thin-film hydration method. They were characterized, investigated for in vitro release, lung disposition and in vivo biological evaluation.
Results: Entrapment efficiency of ethambutol hydrochloride ranged from 12.20% to 25.81%. Zeta potential values inferred stability of neutral and negatively charged formulations. In vitro release was biphasic. Lung targeting was increased by niosomal encapsulation. Biological evaluation revealed superiority of niosomal ethambutol hydrochloride over the free drug.
Discussion: Neutral and negatively charged niosomal vesicles are dispersed homogenously unlike positively charged vesicles. Niosomal encapsulation results in controlled drug release. Niosomal formulations targeted more drugs to mice lungs for a prolonged period of time resulting in: decreased root-specific lung weight, bacterial counts in lung homogenates and optimizing pathological effect on guinea pigs lungs, livers and spleens.
Conclusion: Encapsulation of ethambutol hydrochloride in niosomal formulations for the treatment of TB provides higher efficacy and safety compared with the free drug.
Introduction
Ethambutol is bacteriostatic against Mycobacterium tuberculosis (M. tuberculosis) with an minimum inhibitory concentration (MIC) of 0.5–8 μg/ml; it is bactericidal at higher concentrations. It possesses little sterilizing activity. Resistant strains of M. tuberculosis are readily produced if ethambutol is used alone (Martindale, Citation2011). Ethambutol is well absorbed from the gastrointestinal tract, with bioavailabilities of 70–80% (Lee et al., Citation1977, Citation1980).
Tuberculosis (TB) is a disease caused by infection with the parasitic bacillus M. tuberculosis. Although the disease may affect any of the body organs, it affects most commonly the lungs (pulmonary TB) (Farrar, Citation1988). TB is a worldwide health concern. In 2011, about 8.7 million new cases developed TB and 1.4 million people died from it (Global Tuberculosis Report, 2012). Treatment of intracellular M. tuberculosis pathogens still remains difficult, despite the emergence of new antibiotics, because infections are localized within phagocytic cells and most antibiotics, although highly active in vitro, do not actively pass through cellular membranes, hence it is difficult to achieve relatively high concentrations of the drugs within infected cells (Xu et al., Citation2006; Liu et al., Citation2007; Briones et al., Citation2008). The main challenge for intracellular chemotherapy is to design and develop a carrier system for antibiotics that could be efficiently endocytosed by phagocytic cells and, once inside the cells should prolong release of the antibiotics so that the number of doses and associated drug toxicity can be reduced. The problems associated with delivering antibiotics to the intracellular space have led to the investigation of improved drug carriers for treating intracellular pathogens, including microspheres, liposomes and niosomes (El-Ridy et al., Citation2007, Citation2008, Citation2011b).
Niosomes are osmotically active, and are stable on their own and increase the stability of the entrapped drugs (Palozza et al., Citation2006; Abdelkader et al., Citation2012), moreover, the encapsulated drug can be released in a controlled fashion (Cortesi et al., Citation2007). Niosomes improve the therapeutic performance of drug molecules by delayed clearance from the circulation, protecting the drug from biological environment and restricting its effects to target cells (Biju et al., Citation2006).
The single drug (ethambutol hydrochloride) was chosen in this investigation because it is the fourth single tuberculocidal drug encapsulated in niosomes, by El Ridy et al. (El-Ridy et al., Citation2008, Citation2009, Citation2011b) for increasing efficacy and decreasing toxicity.
The aim of this work was to prepare and characterize ethambutol hydrochloride niosomes, for the purpose of drug targeting into the lungs and hopefully to macrophages to acquire decreased side effects and to achieve sustainment of ethambutol hydrochloride antimycobacterial systemic effect.
Methods
Materials
Ethambutol hydrochloride was a gift sample from El-Nasr Pharmaceutical Chemicals Company, Abuzaabal, Egypt. Sorbitan monostearate (Span 60), Merck Schuchardt OHG, Hohenbrunn, Germany. Sorbitan trioleate (Span 85), Cholesterol (CHOL) from Lanolin, minimum 99% (GC), Dihexadecyl hydrogen-Phosphate (Dicetyl Phosphate, free acid crystalline) (DCP),Fluka, Sigma-Aldrich Chemie GmbH, Steinheim, Germany. Octadecylamine (Stearylamine) (SA), minimum 97% (GC), Sodium thioglycolate (2-Mercapto acetic acid sodium salt), Sigma-Aldrich Co., St. Louis, MO. Copper (II) chloride dehydrate, Merck, Damstadt, Germany. All other chemicals were of analytical grade.
Biological materials
Mycobacterium tuberculosis, (strain H37Rv), supplied by bacterial Diagnostic Products Research Department, Veterinary Serum and Vaccine Research Institute (VSVRI), Abbasia, Cairo, Egypt.
Preparation of ethambutol hydrochloride niosomes
Ethambutol hydrochloride niosomes were prepared by the thin film hydration method (Baillie et al., Citation1985; El-Ridy et al., Citation2005, Citation2007, Citation2008, Citation2011a,Citationb, Citation2012), using non-ionic surfactants, Span 60 and Span 85 which are safe and non-toxic. The LD50 of Span 60 is reported as 31 000mg/kg and that of Span 85 is 39 800mg/kg (Material Safety Data Sheet, 2003, 2012). Cholesterol was used as an enhancer of niosomal membrane rigidity (Manosroi et al., Citation2003). Two molar ratios between non-ionic surfactant and cholesterol were employed, 1:1 (Hao et al., Citation2002) and 4:2 (Uchegbu & Florence, Citation1995), (El-Ridy et al., Citation2007). DCP was used to prevent niosome aggregation and impart negative surface charge to the vesicles (Cable, Citation1989; Yoshioka et al., Citation1994). SA was used as a positive charge inducer. Composition of ethambutol hydrochloride niosomal formulations were illustrated in .
Table 1. Types of prepared ethambutol hydrochloride niosomal formulations with mean percent entrapment.
Determination of entrapment efficiency
The amount of entrapped drug was estimated by subtracting the amount of free drug, measured at λmax 673 nm, collected from the separation and washing of the niosomal suspension, from the initial amount added at the start of the preparation (Aggarwal & Kaur, Citation2005) using table top refrigerated centrifuge, Unio 32R, Hanil Scientific Industrial Co., Korea. The entrapment efficiency (%) was defined as:
(Ruckmani et al., Citation2000; Aggarwal & Kaur, Citation2005)
Characterization of ethambutol hydrochloride niosomes
Transmission electron microscopy
Transmission electron micrographs of ethambutol hydrochloride niosomal formulations were obtained using transmission electron microscope, Jeol, JEM-1230, Japan. The dye used was 1% phosphotungstic acid (Muzzalupo et al., Citation2005; Manconi et al., Citation2006; Palozza et al., Citation2006).
Particle size and zeta potential determinations
Vesicle properties, particle size diameter and zeta potential, were determined at room temperature by Zeta Potential/Particle Sizer NICOMP™ 380 ZLS, PSS-NICOMP particle sizing systems, Santa Barbara, CA, equipped with a 5-mW laser with a wavelength output of 632.8 nm. Niosomal formulations were diluted with phosphate buffered saline, pH 7.4, 10- and 20-folds for Zeta potential and particle size determination, respectively.
Differential scanning calorimetry
Niosomal pellets were lyophilized. Differential scanning calorimetry (DSC) thermograms for individual components, Span 60, Span 85, Cholesterol, SA and DCP, as well as the drug powder, were investigated. Weights used for the niosomal preparations were equivalent to 2 mg Span 60 or Span 85. A heating rate of 10 °C/min was employed over a temperature range (30–250) °C for Span 60 niosomes and (−100–300) °C for Span 85 niosomes.
In vitro release study
Two ethambutol hydrochloride niosomal formulations and their corresponding blanks, were selected for this study, (F2) and (F4). Pellets were suspended in phosphate buffered saline (pH 7.4), and placed on a rotary shaker (El-Ridy et al., Citation2005, Citation2007, Citation2008, Citation2011a,Citationb, Citation2012; Mokhtar et al., Citation2008) at a rate of 150 strokes/min and temperature 37 °C. Five milliliter samples were withdrawn and analyzed spectrophotometrically at different time intervals; 0, 3, 6, 24 and 48 h. Each result was the mean of four determinations. Percentage drug released was plotted as a function of time. Release efficiency (RE) was calculated from the area under the release curve at time t (AUC), according to the trapezoidal rule (Khan, Citation1975). AUC under in vitro release profiles was computed to compare RE of formulations (F2) and (F4).
Selection of ethambutol hydrochloride niosomal formulations for in vivo studies
Niosomal formulations prepared using Span 60, were chosen for in vivo evaluation. This was rationalized by the experimental observations that, the precipitated niosomal pellets, on centrifugal separation, were very well packed, compared with those of Span 85. The two molar ratios, 1:1 and 4:2 were considered. For the molar ratio (1:1), the negatively charged formulation (F2), possessed higher entrapment efficiency 19.642%. For the molar ratio (4:2), the neutral formulation (F4) was selected based on higher entrapment efficiency. The respective drug free niosomes were considered as control. The two formulations (F2) and (F4) with different surface charge and high encapsulation, were selected for in vivo studies to utilize the least volume of injection into experimental animals. The particle size of the stated formulations are ∼812 and 324 nm, respectively. It was reported that particles from 150 nm to 2 µm exhibit maximal phagocytosis by macrophages (Shi et al., Citation2006). Hence, the two selected formulations fulfill the desired size for the required objective of this work.
Drug disposition study in the mice lungs
Sixty-six Swiss Albino mice of average weight 20 gm were employed. They were divided randomly into six groups. Mice of treatment group 1 were injected subcutaneously (s.c.) with a single dose equivalent to 10 mg free ethambutol hydrochloride in phosphate buffer saline (pH 7.4). Treatment group 2 received the formula (F4) and treatment group 3 received the formula (F2), each equivalent to 10 mg drug. Mice of the first control group were injected s.c. with phosphate buffer saline (pH 7.4) and the second, drug-free neutral niosomes, molar ratio (4:2). The third control group received negatively charged drug-free niosomes, molar ratio (1:1:0.1). At 3, 24, 48 and 72 h after injection, five animals from each treatment group were sacrificed. One animal from each control group was sacrificed after 3 and 24 h. Mice lungs were separated and washed twice with phosphate buffer saline pH 7.4 to get rid of excess blood, then stored frozen till further use.
Mice lungs were, air-dried for 30 min on filter papers, weighed and homogenized completely in mobile phase. The lung homogenates were centrifuged for 30 min (at 3000 rpm and room temperature); the supernatant was filtered through nylon syringe filter (0.45 μm). The clear supernatant was assayed for ethambutol hydrochloride by HPLC assay (Jiang et al., Citation2002). The λmax for quantitation was 260 nm, injection volume, 20 µl and elution flow rate, 1 ml/min.
In vivo biological evaluation of ethambutol hydrochloride niosomes
Thirty guinea pigs, weighing ∼300–400 g, were employed. Infection took place using a suspension of M. tuberculosis; (strain H37Rv), maintained on Lowenstein–Jensen medium in sterile saline. Guinea pigs were infected by i.m. injection of 0.1 ml of the mycobacterial suspension. This is equivalent to a load of bacilli of about 1 × 105 of bacilli (Pandey & Khuller, Citation2005).
Frozen ethambutol hydrochloride niosomal formulations were thawed, diluted so that each treatment dose was equivalent to 50 mg drug/kg/day (Karlson, Citation1961). Free ethambutol hydrochloride solution in saline was prepared and injected s.c., where each 400 gm guinea pig received 50 mg drug/kg.
Three weeks after infection, guinea pigs were randomly divided into six groups, two control and four treatment groups; each consisted of five guinea pigs. Group 1 was s.c. injected with normal saline 6 days/week for 6 weeks (36 doses). Group 2 was s.c. injected with neutral drug-free niosomes, molar ratio (4:2) twice weekly for 6 weeks (12 doses). Both were considered control groups. Group 3 was given free ethambutol hydrochloride dissolved in saline (50 mg/kg) (Karlson, Citation1961) 6 days/week for 6 weeks (36 doses). Group 4 was given free ethambutol hydrochloride in saline twice weekly for 6 weeks (12 treatment doses). Group 5 was given the formula (F2) twice weekly for 6 weeks (12 treatment doses were given s.c.). Group 6 was given the formula (F4) twice weekly for 6 weeks (12 treatment doses were given s.c.). Each group of animals was kept in a separate cage, fed with standard pellet diet supplied from the Bacterial Diagnostic Products Research Department, Veterinary Serum and Vaccine Research Institute (VSVRI).
Assessment parameters
After the last treatment dose, three animals were sacrificed from each of the control and treatment groups. These were dissected and the lungs, livers, spleens of each guinea pig were separated and kept in tightly closed containers. This process took place in laminar flow cabinet using sterile equipment.
Evaluation of root-specific lung weight
The weight of the guinea pigs was determined before the animals were sacrificed. After dissection, the lungs of each guinea pig were removed and weighed. The root-specific lung weight (RSLW) was calculated (Orozco et al., Citation1986):
Bacterial counts
The lungs were weighed then homogenized applying a method modified from a previous report (Marks, Citation1972). Homogenization took place in a sterile mortar containing washed sterile sand. Excess fat was trimmed and the lungs were cut into small pieces, 2 ml sterile distilled water were added to the crushed tissue, homogenized and ground till a suspension was obtained. About 2 ml 4% sulphuric acid was added to the mixture and incubated at 37 °C for 20 min. The mixture was further diluted with 16 ml sterile distilled water, and centrifuged at 3000 rpm for 20 min.
The culture medium for organ homogenates was Lowenstein–Jensen medium (Becton Dickinson Company, NJ). The medium was prepared and placed in screw-capped tubes which were arranged in slanted positions, coagulated and condensed at 85 °C for 45 min. The supernatants of lung homogenates were cultured on a duplicate set of Lowenstein–Jensen slants (Qurrat-ul et al., Citation2003). The slants were incubated at 37 °C for 8 weeks and examined weekly for visible growth. The number of live bacilli was counted as number of Colony Forming Unit (CFU) per gram of animal lung weight 8 weeks after culture.
Histopathological study
Autopsy samples were taken from lungs, livers and spleens of guinea pigs in different groups and fixed in 10% formal saline for 24 h. The obtained tissue sections were processed and stained with hematoxylin and eosin stain for histopathological examination by light microscope (Banchroft et al., Citation1996). The organs were examined for the type and severity of lesions.
Ethical aspects
Animal experiments were conducted with the approval of the Research Ethics Committee of the National Research Centre, Egypt.
Statistical analysis
All data were presented as mean values ± SD (mean ± SD). Analysis of variance (ANOVA, one way) followed by LSD, using SPSS® software. Independent Student’s t-test was also employed. Difference at p ≤ 0.05 was considered to be significant.
Results
Assessment of entrapment efficiency
The results of entrapment efficiency are illustrated in . It is observed that there is no obvious effect of surface charge on entrapment efficiency.
Niosomal molar ratio effects an impact on entrapment efficiency where (F4) shows significant increase (p ≤ 0.001) in % drug entrapped (23.176%) compared with (F1); 12.201 % using unpaired Student’s t-test. The entrapment efficiency of charged and neutral ethambutol hydrochloride niosomes prepared with the molar ratio (4:2:1) is higher than that of niosomes prepared with the other molar ratio (1:1:0.1) even after changing the type of non-ionic surfactant.
Surfactant type affects entrapment efficiency. Unpaired Student’s t-test (p ≤ 0.01 level) reveals a significant increase in mean % entrapment of both neutral and negatively charged ethambutol hydrochloride niosomes (F7, F8), prepared using Span 85 (17.907% and 24.604%, respectively) compared with those prepared using Span 60 (F1, F2) (12.201% and 19.642%, respectively).
Characterization of ethambutol HCl niosomes
Transmission electron microscopy
Transmission electron micrographs confirm the formation of vesicular structures of ethambutol hydrochloride niosomes prepared using Span 60 () and Span 85 (). The vesicles are discrete and separate with uniform size.
Figure 1. (a) Transmission electron micrographs of niosomal formulations prepared using Span 60 [Mag. ×25 000]. F* = Drug-free niosomes, F3 [Mag. ×60 000]. (b) Transmission electron micrographs of niosomal formulations prepared using Span 85 [Mag. ×25 000]. F* = Drug-free niosomes, F8 [Mag. ×60 000].
Zeta potential determination
The values of zeta potential of niosomal vesicles are represented in .
Table 2. ZP, mean particle diameters and PI of investigated niosomes.
The zeta potential values of neutral and negatively charged niosomal formulations, prepared using Span 60 and Span 85, of either molar ratio, falls in the zone in which the colloidal system dispersion is stable (more positive than +30 mV or more negative than −30 mV), above +30 mV. In contrast, the zeta potential values of drug-free and ethambutol HCl positively charged niosomal formulations prepared using Span 60 of either molar ratio, falls in the zone in which the colloidal system dispersion is unstable (−30 ↔ +30 mV).
Particle size determination and polydispersity index
reveals that the niosomal formulations prepared using Span 60, with or without charge inducing agent, molar ratio (1:1:0.1) are in the nano-size range except the positively charged drug-free niosomes which exhibit larger size, mean particle diameter: 1084.45 nm.
reveals that the polydispersity values of the investigated formulations were high, indicating a heterogenous population.
Differential scanning calorimetry
Differential scanning calorimetry was carried out on the freeze-dried niosomal pellets of ethambutol hydrochloride niosomes and drug-free niosomes. DSC for the individual constituents of niosomes, Span 60, cholesterol, DCP and SA and ethambutol hydrochloride shows thermal peaks at 57.79 °C, 151.29 °C, 75.97 °C, 51.45 °C and 199.29 °C, respectively, corresponding to their melting temperatures. shows that both transition temperature and transition energy of Span 60 peak of all niosomal formulations are less than that of pure Span 60.
Figure 2. (a) DSC thermograms of niosomal formulations prepared using Span 60. (b) DSC thermograms of niosomal formulations prepared using Span 85.
The effect of entrapped ethambutol hydrochloride on thermodynamic parameters of the niosomal vesicles () was evident where the niosomal formulations entrapping ethambutol hydrochloride exhibit disappearance of its characteristic exothermic peak.
shows that the DSC thermograms of niosomal formulations prepared using Span 85 with or without charge inducing agent, using either molar ratio, reveal the effect of niosomal formulation on the individual constituents of niosomes as well as the effect of entrapped drug by changing the thermodynamic parameters of the thermal peaks.
In vitro release study
The obtained results () reveal that the release of ethambutol hydrochloride from niosomes is biphasic, with an initial relative fast release phase followed by a slower one. Rapid drug leakage is observed during the initial phase, 0–24 h, where 31.56% and 18.17% of the entrapped drug is released during the first 24 h from (F2) and (F4), respectively.
Figure 3. Mean percent ethambutol hydrochloride released from F2 and F4 in phosphate buffer saline (pH 7.4).
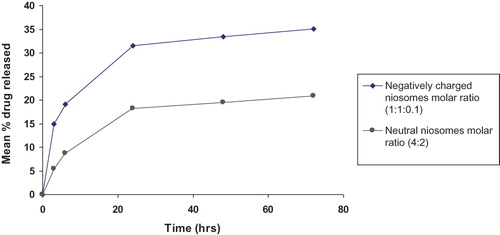
Statistical analysis using Unpaired Student’s t-test reveals that the rate of ethambutol hydrochloride release from neutral niosomes (F4) is significantly slower than that released from negatively charged niosomes (F2) (p ≤ 0.001) throughout the drug release pattern investigated.
The release efficiencies [RE0–72 h (%)] of the two selected niosomal formulations were calculated for further comparisons. Release efficiency of ethambutol hydrochloride neutral niosomes (F4) (16.80% ± 1.22) is significantly lower than that of negatively charged niosomes (F2) (29.61% ± 0.55) (p ≤ 0.001).
Drug disposition study in mice lungs
The HPLC spectrum of ethambutol hydrochloride dissolved in mouse lung homogenate solution in mobile phase shows a retention time of 7.7 min at λmax 260 nm. reveals that 3 h after injection, free ethambutol hydrochloride solution shows highest drug concentration (115.82 ng/mg), compared with both niosomal formulations investigated, 45.77 ng/mg and 87.10 ng/mg for (F4) and (F2), respectively. A significant difference (p ≤ 0.01) is observed between drug levels in the three treatment groups, 3 h after s.c. injection.
Figure 4. Mean amount of ethambutol hydrochloride deposited in mice lungs (ng/mg lung) after single s.c. injection of free drug compared with F2 and F4.
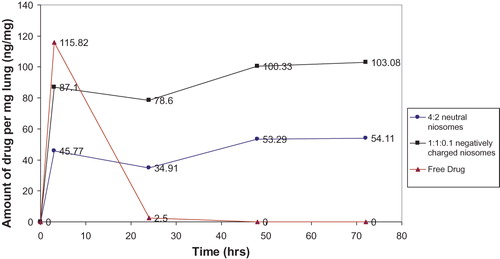
After 24 h, both niosomal formulations, show higher drug levels than the free drug solution, 34.91 and 78.60 ng/mg for (F4) and (F2), respectively, compared with 2.50 ng/mg for drug solution. A significant difference (p ≤ 0.001) is evident between ethambutol hydrochloride levels of the three treatment groups at 24 h after injection. The drug levels in mice lungs receiving niosomal formulations (F4) and (F2), are decreased from 45.77 ng/mg and 87.10 ng/mg, 3 h after s.c. injection, to 34.91 and 78.60 ng/mg, after 24 h, respectively.
The drug levels in mice lungs receiving the drug niosomal formulations (F4) and (F2), are significantly increased (p ≤ 0.05) 48 h, after s.c. injection, (53.29 ng/ml and 100.33 ng/mg) compared with those after 24 h ().
After 72 h, the drug levels in mice lungs for the two investigated niosomal formulations (F4) and (F2), remain approximately unchanged (54.11 and 103.08 ng/mg) compared with the 48 h values (53.29 ng/ml and 100.33 ng/mg).
Areas under the curves (AUC) reflecting the amount of ethambutol HCl deposited into mice lungs (ng/mg lung) after s.c. injection of the three treatment groups were calculated by trapezoidal method. The calculated values were 1446.09, 3263.055 and 6458.742 h ng/mg lung for free ethambutol hydrochloride solution, (F4) and (F2), respectively ().
Figure 5. AUC of ethambutol HCl deposited in mice lungs (ng/mg lung) after single s.c. injection of the three treatment groups.
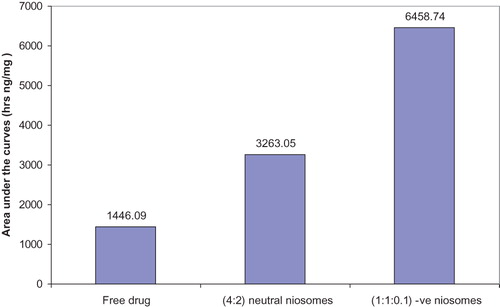
It is noteworthy that, the mean drug level value in mice lungs receiving the niosomal formulation (F4), is lower than that for the niosomal formulation (F2). This observation is emphasized by statistical analysis using independent Student’s t-test (p ≤ 0.01).
Biological evaluation of ethambutol hydrochloride niosomes
Evaluation of root-specific lung weight
As shown in , the control group (group 1) shows the highest RSLW value, 18.55, while group 5 treated with the niosomal formulation (F2), twice weekly, shows the least RSLW value, 9.71.
Table 3. Mean RSLW values for lungs and bacterial counts of guinea pig lung homogenates receiving different treatments.
One-way ANOVA reveals a significant difference between the six groups, difference at p ≤ 0.05 is considered to be significant.
Statistical analysis reveals that both control groups (1 and 2) show a significant higher RSLW value than that of the four treatment groups (3–6) (p ≤ 0.001).
Root-specific lung weight value of group 6 (11.06) that was infected and treated with neutral niosomes, (F4) is significantly lower than that of group 4 treated with free ethambutol hydrochloride twice weekly (13.15) (p ≤ 0.01). Although RSLW value shows no significant difference (p > 0.05) between group 6 treated with neutral niosomes (F4) (11.06) and group 3 treated with free drug (6 days/week) (11.94), yet the dosage regimen of group 6 (twice weekly) is 3 times less than that of group 3 (6 days weekly) which in turn decreases the toxicity of the drug.
The mean RSLW value of group 5 receiving the formulation (F2) is lower than that receiving (F4) as revealed using Independent Student’s t-test (p ≤ 0.05).
Bacterial counts of lung homogenates
The results of bacterial counts of homogenized lungs of guinea pigs (), reveal that the two control groups (1 and 2), exhibit the highest severity of infection as revealed by the highest mean values of log Colony Forming Unit (CFU) per gram lung weight (9.38 and 9.32, respectively).
The two groups of guinea pigs (5 and 6), infected and treated with niosomal ethambutol hydrochloride formulations twice weekly, (F2) and (F4), exhibit lower mean values of log CFU per gram lung weight (5.41 and 6.09, respectively).
One-way ANOVA reveals a significant difference between the six groups, difference at p ≤ 0.05 is considered to be significant.
Histopathological study
Histopathological findings in lungs, livers and spleens of guinea pigs of the control and treated groups () show that for group 3 () lungs exhibit focal lymphoid cells aggregation in the parenchyma as well as diffuse lymphocytes infiltration between the alveoli associated with areas of collapse and emphysema. The livers show severe (+++) granuloma in the epitheloid and lymphoid cells of parenchyma associated with very severe (++++) central necrosis and focal lymphoid cells aggregation in the adjacent area with hyperplasia in the bile ducts. The spleens show mild (+) depletion in the central portion of some white pulps.
Group 4 shows that lungs exhibit severe (+++) cellular granuloma with central moderate (++) necrosis in the parenchyma. Livers show severe focal cellular (+++) granuloma associated with haemorrhages and hyperplasia in the bile ducts. The spleens show lymphoid depletion and severe (+++) necrosis in the central portion of the white pulps.
Group 5, where guinea pigs are infected and treated (F2), lungs exhibit areas of collapse and emphysema in the parenchyma. The livers show severe (+++) hepatic parenchyma focal cellular granuloma associated with hyperplasia in the bile ducts. The spleens show depletion of some white pulps in the central portion with diffuse hemosiderosis.
Group 6, of guinea pigs infected and treated with (F4), show severe granulomatous reaction (+++) with moderate central necrosis (++) in the parenchyma of lungs. The livers show lymphoid and few epitheloid cells granuloma formation (+++) with hyperplasia in the bile duct and degeneration in the hepatic parenchyma. Spleens show lymphoid depletion as well as mild (+) lymphoid cellular necrosis in the white pulps.
The two control groups (1 and 2) show the most severe infection compared with the preceding four treatment groups. Group 1 shows lungs with peribronchiolar and very severe (++++) parenchymal cellular granuloma formation associated with moderate (++) necrosis in lung parenchyma and focal lymphoid cells aggregation replacing the air alveoli. The livers show focal circumscribed round very severe (++++) cellular granuolma replacing the hepatic parenchyma associated with hyperplasia in the bile duct and severe (+++) degeneration in other hepatocytes as well as severe (+++) necrosis in the central zone in some granuolma. The spleens show diffuse proliferation of the epitheloid cells all over the splenic tissue associated with moderate (++) necrobiosis in the lymphoid cells and diffuse hemosiderosis.
Treatment with (F2) protects the investigated organs from necrosis. Treatment with (F4), as well as, free drug twice weekly, leads to the development of moderate (++) necrotic reaction in the lungs of guinea pigs. However, spleens of guinea pigs treated with (F4) exhibit mild (+) necrobiosis of lymphoid cells, compared with severe (+++) necrobiosis when treated with free drug twice weekly. Guinea pigs treated with free ethambutol hydrochloride, either twice weekly or 6 days/week, exhibit necrotic reactions. The severity of infection is high in both groups. The two control groups show most severe infection.
Discussion
Regarding encapsulation efficiency, neutral ethambutol hydrochloride niosomes molar ratio (4:2) (F4) show significant increase in the mean % drug entrapped (23.176%) as compared with that of the other molar ratio (1:1) (F1); 12.201%. These results are in good agreement with that previously reported (Muzzalupo et al., Citation2005), where the best encapsulation efficiency was obtained when niosomes were made up of surfactant and cholesterol at molar ratio (4:2). Similarly (Manosroi et al., Citation2003), niosomes with the molar ratio of Span 60/cholesterol at (7:3) gave the highest entrapment efficiency, for the water-soluble fluorescent marker (calcein), than those with equal molar ratio of Span 60/cholesterol. It was reported that incorporation of cholesterol into niosomes was found to increase the encapsulation efficiency of the drug up to the optimum concentration of cholesterol (Guinedi et al., Citation2005; Nasr et al., Citation2008). Further increase of cholesterol content reaching 1:1 molar ratio, reduces the entrapment efficiency. This is explained by the fact that, higher amounts of cholesterol may compete with the drug for packing space within the bilayer, hence excluding the drug as the amphiphiles assemble into the vesicles (Balakrishnan et al., Citation2009).
Both neutral and negatively charged ethambutol hydrochloride niosomes (F7, F8), prepared using Span 85, molar ratio 1:1:0.1, show an increase in entrapment efficiency compared with those prepared using Span 60. It was reported that the lower the HLB of the surfactant the higher the drug entrapment efficiency will be (Guinedi et al., Citation2005). Span 85 possesses an HLB value (1.8) lower than that of Span 60 (4.7) (Uchegbu & Florence, Citation1995). Accordingly, entrapment efficiency into Span 85 niosomes is higher than in Span 60 niosomes.
reveals that the values of zeta potential of all neutral and negatively charged niosomal vesicles, using Span 60 and Span 85 for either molar ratio, are above +30 mV. This indicates that these niosomal vesicles are not aggregated together and hence dispersed homogenously in the buffer solution (phosphate buffer saline pH 7.4) so, the dispersion system is stable. The zeta potential values for drug-free and ethambutol hydrochloride positively charged niosomal vesicles falls within the unstable region. This indicates that the positively charged niosomal vesicles, aggregate followed by sedimentation and caking in the buffer solution (phosphate buffer saline pH 7.4) hence, the dispersion system is unstable. This result is in a good agreement with that previously obtained (El-Ridy et al., Citation1988) who reported that the positively charged liposomes aggregate in the buccal cavity.
demonstrates the results of mean particle diameters for niosomal formulations prepared using Span 60, with or without charge inducing agent, molar ratio (1:1:0.1). They are in the nano-size range except the positively charged drug-free niosomes exhibiting larger diameter; 1084.45 nm. Negatively charged ethambutol hydrochloride niosomes (F2) have a mean particle diameter larger than neutral niosomes (F1), which could be explained by, the positively charged drug molecules tend to neutralize the negative charge of DCP hence, the repulsive force between DCP molecules decreases, so the curvature of niosomal vesicles decreases and consequently the mean particle diameter increases.
On using Span 85, negatively charged niosomes, drug-free or entrapping drug, have mean particle diameters larger than neutral niosomes. This may be due to the negatively charged lipid DCP which would increase the hydrophilicity of the niosomal vesicles, increasing the water intake of the bilayers of these vesicles, which results in increased particle size (Carafa et al., Citation1998).
The results of polydispersity index (PI) () show that the values of all the investigated formulations were high, indicating a heterogenous population. The prepared vesicular formulations were mostly heterogenous, which is usually obtained with vesicles prepared using the thin film hydration method (Pereira-Lachataignerais et al., Citation2006).
The DSC study reveals that both transition temperature and transition energy of Span 60 peak of all niosomal formulations are less than those for pure Span 60 (). Similar results were obtained (Pardakhty et al., Citation2007). This is due to presence of cholesterol which is able to abolish the gel–liquid phase transition of niosomal systems (Cable, Citation1989) resulting in niosomes that are less leaky (Rogerson et al., Citation1987).
The effect of drug entrapment on thermodynamic parameters of all the niosomal vesicles reveals disappearance of the characteristic exothermic ethambutol hydrochloride peak; this is in agreement with that obtained for vinpocetine (El-Laithy et al., Citation2011), aceclofenac (Nasr et al., Citation2008) and insulin (Pardakhty et al., Citation2007). To recapitulate, the absence of the melting exotherm of ethambutol hydrochloride and shifting of the endotherms of bilayer components of niosomes suggest significant interaction of ethambutol hydrochloride with bilayer components and accounts for the enhanced entrapment of ethambutol hydrochloride into the niosomal formulations (Nagarsenker & Joshi, Citation1997; Hathout et al., Citation2007).
reveals that the release of ethambutol hydrochloride from niosomes is biphasic. Rapid drug leakage is observed during the initial phase, 0–24 h which is explained by desorption of the drug from the surface of niosomes while drug release in the slower phase is regulated by diffusion through the swollen niosomal bilayers (Pardakhty et al., Citation2007). This biphasic release pattern seems to be a general characteristic of bilayer vesicles, as previously reported for some liposomes (Bhaskaran et al., Citation2011; Kumar et al., Citation2011) and niosomes (Pardakhty et al., Citation2007; Mokhtar et al., Citation2008; Aboelwafa et al., Citation2010; El-Laithy et al., Citation2011). The release data indicate that, by encapsulation of drug into niosomes, it is possible to sustain and control the release of the drug (Ruckmani et al., Citation2000); this in turn leads to decreasing the dosage regimen of the drug, thus decreasing drug toxicity; in addition to achieving a rapid onset of action of the drug due to the initial fast release.
The release efficiencies [RE0–72 h (%)] of the two niosomal formulations selected for in vivo investigation, (F4) and (F2) reveal that the value for the neutral niosomes (F4) is significantly lower than that of negatively charged niosomes (F2) (p ≤ 0.001). This is explained by the inverse relation between the entrapment efficiency and the drug release. Entrapment efficiency is a measure of the vesicle ability to retain the drug; thus, the more the drug is retained in the vesicle, the slower the release profile will be (Uchegbu & Florence, Citation1995). The entrapment efficiency of ethambutol hydrochloride into (F4) (23.18%) is higher than that into (F2) (19.64%). Accordingly, the formula (F4) exhibits a drug release profile slower than that exhibited by (F2). The results are in a good agreement with those reported (Guinedi et al., Citation2005) indicating that niosomal formulation with the highest entrapment efficiency showed the lowest drug release profile. Another report (Abd-Elbary et al., Citation2008) mentioned that niosomal formulation which had significant higher entrapment efficiency would provide a better control of drug release rate than that with a lower entrapment efficiency. The same was reported elsewhere (Muzzalupo et al., Citation2007; Kakkar et al., Citation2011; Sathyavathi et al., Citation2012).
Niosomes are stable vesicles. The values of zeta potential of formulations (F2) and (F4) are ∼70 and 52, respectively, thus falling in the stable zone. It is worthy to mention that niosomes intended for parenteral application are stored as lyophilized powders (dry form) not as suspension form and reconstituted directly before use. In a previous report, it is stated that non-ionic surfactant vesicular systems (niosomes) are a novel and efficient approach to drug delivery. As a drug delivery device, niosomes are osmotically active and stable. They also improve the stability of entrapped drug during delivery (Kumar & Rajeshwarrao, Citation2011). In another report, non-ionic surfactants as Span 20, 40 and 60 were used in preparing nimesulide niosomes. The stability study showed that niosomal preparations stored at refrigerated temperature for 60 days showed maximum drug retention for all formulations compared to room and elevated temperature conditions (Singh et al., Citation2011a).
represents the results of the lung disposition experiment. It is clear that 3 h after injection, free ethambutol hydrochloride solution shows the highest drug concentration, compared with the niosomal formulations investigated. After 24 h, both niosomal formulations show higher drug concentration than the drug solution. Most of the free drug is cleared within 24 h from the mice lungs receiving free ethambutol hydrochloride solution due to its low elimination half life value (2.5 h) in serum (Peloquin et al., Citation1999) or (3–4 h) (Martindale, Citation2011). The drug levels in the lungs, of mice groups receiving drug niosomal formulations, are non-significantly decreased 24 h after s.c. injection, compared with 3 h, yet this slight decrease may represent the clearance of ethambutol hydrochloride portion reaching the lung in the free form. The same behaviour was achieved by liposomally encapsulated amikacin inhaled into rat lungs (Meers et al., Citation2008). After 48 h, the drug level in mice lungs receiving the niosomal formulations are significantly increased (p ≤ 0.05) compared with 24 h. After 72 h, the levels of drug in the mice lungs are nearly the same as the 48-h levels for both niosomal formulations. This behaviour reveals a sustained release of niosomally encapsulated ethambutol hydrochloride in mice lungs.
It is to be mentioned that niosomes are preferentially biodistributed to the reticuloendothelial system, comprising phagocytic cells, located in organs such as lung, liver and spleen, so niosomes are good carriers for targeting drugs to treat diseases related to these organs (Baillie et al., Citation1986; Singh et al., Citation2011b). Niosomal encapsulation of ethambutol hydrochloride aimed at targeting more drugs to the lungs thus increasing its tuberculocidal activity.
Area under the curve (AUC) values were computed (). The drug level in mice lungs receiving (F4) and (F2) is larger than that in the group receiving free ethambutol hydrochloride solution by 2.26 and 4.47 times, respectively. These findings reveal the superiority of niosomes to dispose higher amount of the drug, by successful targeting to the lung, in addition to prolonging the duration of drug action. The mean drug level in mice lungs of (F4), is lower than that of (F2), throughout 72 h. This finding can be well correlated with the in vitro release profile of the two investigated niosomal formulations, where the statistical analysis reveals that the rate of ethambutol hydrochloride release from neutral niosomes (F4) is significantly slower than that released from negatively charged niosomes (F2) (p ≤ 0.001).
illustrates the results of the biological evaluation of ethambutol hydrochloride niosomes. It is reported that a lower value of RSLW indicates less severity of infection and better efficacy in treatment of TB (Deol et al., Citation1997). The results of RSLW and bacterial counts of lung homogenates () indicate that among the four treatment groups, the best formula for the treatment of M. tuberculosis, infection is ethambutol hydrochloride negatively charged niosomes (F2), followed by ethambutol hydrochloride neutral niosomes (F4), these two formulae exhibit better performance compared with the free drug either at a lower dosage (twice weekly) or higher dosage (6 days/week) regimen. That is due to targeting in addition to prolonging the action of ethambutol hydrochloride entrapped into niosomes compared with the free drug which has a low elimination half-life value of 40 min in guinea pigs (Thomas & Durr, Citation1983).
These obtained results prove the superiority of ethambutol hydrochloride niosomal formulations, in treatment of TB, compared with the free form of the drug.
These results are in a good agreement with those reported (El-Ridy et al., Citation2011b), regarding the biological evaluation of niosomal pyrazinamide.
Histopathological study () proves the superiority of formula (F2) [Span 60: Cholesterol: DCP, 1:1:0.1], for the treatment of M. tuberculosis infection. This is correlated with the results of RSLW value and bacterial counts of lung homogenates.
Figure 6. Histopathological examination of guinea pigs organs infected and differently treated (H&E, ×40), (a) Lung infected and treated with free ethambutol hydrochloride 6 days weekly for 6 weeks, show focal lymphoid cells aggregation (arrow) and diffuse lymphoid cells infiltration in between the air alveoli, (b) Livers show wide circumscribed area of necrosis replacing the hepatic parenchyma (n), (c) Spleens show mild depletion in central portion of the white pulp (f), (d) Lungs infected and treated with free ethambutol hydrochloride twice weekly for 6 weeks, show granuloma formation mainly cellular (g) with central necrosis, (e) Livers show granuloma formation (g) with haemorrhages (h) and hyperplasia in bile duct (arrow), (f) Spleens show the necrosis in the lymphoid cells in the center of the white pulps (g), (g) Lungs infected and treated with ethambutol hydrochloride niosomes (1:1:0.1 negative) (F2) twice weekly for 6 weeks, show collapse (arrow) and emphysema (e) in air alveoli, (h) Livers show hyperplasia in bile duct (arrow) in the portal area, (i) Spleens show intact white pulp (w) with hemosiderosis in red pulp, (j) Lungs infected and treated with ethambutol hydrochloride niosomes (4:2 neutral) (F4) twice weekly for 6 weeks, show cellular granuloma with central necrosis (g) replacing the lung parenchyma, (k) Livers show granuloma formation mainly from lymphoid cells (g) with hyperplasia in bile duct (arrow) and degeneration in other hepatocytes (d), (l) Spleens show lymphoid cellular necrosis in the white pulp (d), (m) Lungs of the control group infected and receiving saline, show granuloma with central necrosis replacing the lung parenchyma (g, n), (n) Livers show necrosis in the central zone of the granuloma (n) surrounded by other leucocytes (m), (o) Spleens show diffuse proliferation of epitheloid cells (m) with necrobiosis in the lymphoid cells and diffuse hemosiderosis (h), (p) Lungs of the control group infected and receiving neutral drug-free niosomes of the molar ratio Span 60: cholesterol (4:2), show cellular granuloma formation without central necrosis (g), (q) Livers show granuloma formation with central necrosis without fibrous connective tissue capsule formation (g) and (r) Spleens show cellular granuloma formation with central necrosis (N).
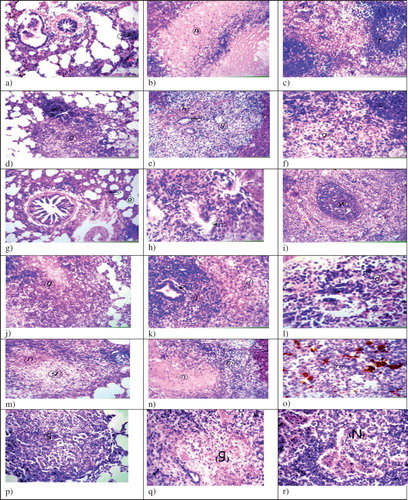
It is to be mentioned that drug-loaded niosomes accumulate in the macrophages as they are preferentially biodistributed to the reticuloendothelial system (Singh et al., Citation2011b). Hence, ethambutol hydrochloride niosomes accumulate in the macrophages through targeting more drugs to the lungs. The implication of this is to obtain a higher tuberculocidal activity with a lower drug dose.
Conclusions
Ethambutol hydrochloride niosomal entrapment efficiency ranges from 12.20% to 25.81%. Zeta potential values of neutral and negatively charged niosomal formulations falls in the stable zone unlike positively charged ones. Drug-loaded niosomal formulations show mean particle diameters in the nano-range except the positively charged niosomes prepared using Span 60. The release of drug from the investigated niosomal formulations is biphasic, with an initial faster release rate. Niosomal formulations have a clear advantage over the free form of the drug, proven by targeting more of the drug to mice lungs for a prolonged period, decreasing the RSLW and bacterial counts in lung homogenates of guinea pigs compared with the free form of the drug. Histopathological examination shows that the drug niosomal formulation (F2) [Span 60: Cholesterol: DCP, 1:1:0.1] to be safer than (F4) [Span 60: Cholesterol, 4:2]. The encapsulation of ethambutol hydrochloride in niosomal formulations for the treatment of TB provides more eminent efficacy and safety compared with the free drug. Also, there is a possibility of dose reduction which will help in adherence to treatment and thus improving patient compliance. Ethambutol hydrochloride was used as a single niosomal tuberculocidal drug following previous successful research on pyrazinamide, rifampicin and isoniazid niosomes. These achievements would propel the authors, in the future, to study niosomal encapsulation of multiple tuberculocidal drugs to avoid resistance.
Declaration of interest
The authors report no declarations of interest. The authors appreciate funding the work by the National Research Centre, Dokki, Giza, Egypt, through Prof. El-Ridy projects 2/8/3 and 80/30/118.
References
- Abd-Elbary A, El-Laithy HM, Tadros MI. (2008). Sucrose stearate-based proniosome-derived niosomes for the nebulisable delivery of cromolyn sodium. Int J Pharm 357:189–98
- Abdelkader H, Wu Z, Al-Kassas R, Alany RG. (2012). Niosomes and discomes for ocular delivery of naltrexone hydrochloride: morphological, rheological, spreading properties and photo-protective effects. Int J Pharm 433:142–8
- Aboelwafa AA, El-Setouhy DA, Elmeshad AN. (2010). Comparative study on the effects of some polyoxyethylene alkyl ether and sorbitan fatty acid ester surfactants on the performance of transdermal carvedilol proniosomal gel using experimental design. AAPS Pharm Sci Tech 11:1591–602
- Aggarwal D, Kaur IP. (2005). Improved pharmacodynamics of timolol maleate from a mucoadhesive niosomal ophthalmic drug delivery system. Int J Pharm 290:155–9
- Baillie AJ, Coombs GH, Dolan TF, Laurie J. (1986). Non-ionic surfactant vesicles, niosomes, as a delivery system for the anti-leishmanial drug, sodium stibogluconate. J Pharm Pharmacol 38:502–5
- Baillie AJ, Florence AT, Hume LR, et al. (1985). The preparation and properties of niosomes non-ionic surfactant vesicles. J Pharm Pharmacol 37:863–8
- Balakrishnan P, Shanmugam S, Lee WS, et al. (2009). Formulation and in vitro assessment of minoxidil niosomes for enhanced skin delivery. Int J Pharm 377:1–8
- Banchroft JD, Stevens A, Turner DR. (1996). Theory and practice of histological techniques. New York, London, San Francisco, Tokyo: Churchil Livingstone
- Bhaskaran S, Harish CG, Lakshmi PK. (2011). Methotrexate liposome as oral drug delivery carriers. J Pharm Res 4:3237–40
- Biju SS, Talegaonkar S, Mishra PR, Khar RK. (2006). Vesicular systems: an overview. Indian J Pharm Sci 68:141–53
- Briones E, Colino CI, Lanao JM. (2008). Delivery systems to increase the selectivity of antibiotics in phagocytic cells. J Control Rel 125:210–27
- Cable C. (1989). An examination of the effects of surface modifications on the physicochemical and biological properties of non-ionic surfactant vesicles. Ph. D. Thesis, Strathclyde, Glasgow, UK
- Carafa M, Santucci E, Alhaique F, et al. (1998). preparation and properties of new unilamellar non-ionic surfactant vesicles. Int J Pharm 160:51–9
- Cortesi R, Esposito E, Corradini F, et al. (2007). Non-phospholipid vesicles as carriers for peptides and proteins: production, characterization and stability studies. Int J Pharm 339:52–60
- Deol P, Khuller GK, Joshi K. (1997). Therapeutic efficacies of isoniazid and rifampin encapsulated in lung-specific stealth liposomes against Mycobacterium tuberculosis infection induced in mice. Antimicrob Agents Chemother 41:1211–4
- El-Laithy HM, Shoukry O, Mahran LG. (2011). Novel sugar esters proniosomes for transdermal delivery of vinpocetine: preclinical and clinical studies. Eur J Pharm Biopharm 77:43–55
- El-Ridy MS, Abd El-Bary AA, El-Gazayrly O, et al. Study of niosomal encapsulation for the antitubercular drug, isoniazid. The Fourth International Conference of Pharmaceutical and Drug Industries Division; 2009 NRC, Cairo, Egypt
- El-Ridy MS, Abdelbary A, Essam T, et al. (2011a). Niosomes as a potential drug delivery system for increasing the efficacy and safety of nystatin. Drug Dev Ind Pharm 37:1491–508
- El-Ridy MS, Abdelbary A, Nasr EA, et al. (2011b). Niosomal encapsulation of the antitubercular drug, pyrazinamide. Drug Dev Ind Pharm 37:1110–8
- El-Ridy MS, Abdelbary A, Khalil RM, et al. (2008). Niosomal encapsulation of the tuberculocidal drug rifampicin. Egypt Pharm J (NRC) 7:69–83
- El-Ridy MS, Badawi AA, Safar MM, Mohsen AM. (2012). Niosomes as a novel pharmaceutical formulation encapsulating the hepatoprotective drug silymarin. Int J Pharm Pharmaceut Sci 4:549–59
- El-Ridy MS, Kassem M, Akbarieh M, Tawashi R. (1988). The effect of surface charge of liposomes on aggregation in the buccal cavity. Proceeding of the 15th International Symposium on Controlled Release of Bioactive Materials, Basel, Switzerland, 344, No. 200
- El-Ridy MS, Mostafa DM, Shehab A, et al. (2007). Biological evaluation of pyrazinamide liposomes for treatment of Mycobacterium tuberculosis. Int J Pharm 330:82–8
- El-Ridy MS, Shehab A, Emmara LM, Moustafa DM. (2005). A three month chemoprophylaxis of Schistosomiasis using liposome-encapsulated oxamniquine. Bull NRC Egypt 30:57–72
- Farrar K. (1988). Tuberculosis and its treatment. Pharm J 240:149–51
- Global Tuberculosis Report (2012). Available from: http://apps.who.int/iris/bitstream/10665/75938/1/9789241564502_eng.pdf [Online]
- Guinedi AS, Mortada ND, Mansour S, Hathout RM. (2005). Preparation and evaluation of reverse-phase evaporation and multilamellar niosomes as ophthalmic carriers of acetazolamide. Int J Pharm 306:71–82
- Hao Y, Zhao F, Li N, et al. (2002). Studies on a high encapsulation of colchicine by a niosome system. Int J Pharm 244:73–80
- Hathout RM, Mansour S, Mortada ND, Guinedi AS. (2007). Liposomes as an ocular delivery system for acetazolamide: in vitro and in vivo studies. AAPS Pharm Sci Tech 8:E1–2
- Jiang Z, Wang H, Locke DC. (2002). Determination of ethambutol by ion-pair reversed phase liquid chromatography with UV detection. Anal Chim Acta 456:189–92
- Kakkar R, Rekha R, Kumar DN, Sanju N. (2011). Formulation and characterisation of valsartan proniosomes. Maejo Int J Sci Technol 5:146–58
- Karlson AG. (1961). Therapeutic effect of ethambutol (dextro-2,2'-[ethylene-diimino]-di-1-butanol) on experimental tuberculosis in guinea pigs. Amer Rev Resp Dis 84:902–4
- Khan KA. (1975). The concept of dissolution efficiency. J Pharm Pharmacol 27:48–9
- Kumar GP, Rajeshwarrao P. (2011). Nonionic surfactant vesicular systems for effective drug delivery-an overview. Acta Pharm Sinica B 1:208–19
- Kumar MR, Aithal BK, Udupa N, et al. (2011). Formulation of plumbagin loaded long circulating pegylated liposomes: in vivo evaluation in C57BL/6J mice bearing B16F1 melanoma. Drug Deliv 18:511–22
- Lee CS, Brater DC, Gambertoglio JG, Benet LZ. (1980). Disposition kinetics of ethambutol in man. J Pharmacokinet Biopharm 8:335–46
- Lee CS, Gambertoglio JG, Brater DC, Benet LZ. (1977). Kinetics of oral ethambutol in the normal subject. Clin Pharmacol Ther 22:615–21
- Liu Y, Miyoshi H, Nakamura M. (2007). Nanomedicine for drug delivery and imaging: a promising avenue for cancer therapy and diagnosis using targeted functional nanoparticles. Int J Cancer 120:2527–37
- Manconi M, Sinico C, Valenti D, et al. (2006). Niosomes as carriers for tretinoin. III. A study into the in vitro cutaneous delivery of vesicle-incorporated tretinoin. Int J Pharm 311:11–9
- Manosroi A, Wongtrakul P, Manosroi J, et al. (2003). Characterization of vesicles prepared with various non-ionic surfactants mixed with cholesterol. Colloid Surf B: Biointerfaces 30:129–38
- Marks J. (1972). Ending the routine guinea-pig test. Tubercle 53:31–4
- Martindale. (2011). The complete drug reference. In: Sweetman SC, ed. The extra pharmacopoeia. 37 edn. London: Pharmaceutical Press
- Material Safety Data Sheets (2003). Sorbitan trioleate, SPAN® 85, Uniqema, International business of Imperical Chemical Industries PLC. [Online]
- Material Safety Data Sheets (2012). Sorbitan monostearate (Span 60) MSDS, science lab.com [Online]. science lab.com. (Last ubdated 6/9/2012)]
- Meers P, Neville M, Malinin V, et al. (2008). Biofilm penetration, triggered release and in vivo activity of inhaled liposomal amikacin in chronic Pseudomonas aeruginosa lung infections. J Antimicrob Chemother 61:859–68
- Mokhtar M, Sammour OA, Hammad MA, Megrab NA. (2008). Effect of some formulation parameters on flurbiprofen encapsulation and release rates of niosomes prepared from proniosomes. Int J Pharm 361:104–11
- Muzzalupo R, Nicoletta FP, Trombino S, et al. (2007). A new crown ether as vesicular carrier for 5-fluoruracil: synthesis, characterization and drug delivery evaluation. Colloids Surf B: Biointerfaces 58:197–202
- Muzzalupo R, Trombino S, Iemma F, et al. (2005). Preparation and characterization of bolaform surfactant vesicles. Colloids Surf B: Biointerfaces 46:78–83
- Nagarsenker MS, Joshi AA. (1997). Preparation, characterization and evaluation of liposomal dispersions of lidocaine. Drug Dev Ind Pharm 23:1159–65
- Nasr M, Mansour S, Mortada ND, Elshamy AA. (2008). Vesicular aceclofenac systems: a comparative study between liposomes and niosomes. J Microencap 25:499–512
- Orozco LC, Quintana FO, Beltran RM, et al. (1986). The use of rifampicin and isoniazid entrapped in liposomes for the treatment of Murine tuberculosis. Tubercle 67:91–7
- Palozza P, Muzzalupo R, Trombino S, et al. (2006). Solubilization and stabilization of β-carotene in niosomes: delivery to cultured cells. Chem Phys Lip 139:32–42
- Pandey R, Khuller GK. (2005). Solid lipid particle-based inhalable sustained drug delivery system against experimental tuberculosis. Tuberculosis 85:227–34
- Pardakhty A, Varshosaz J, Rouholamini A. (2007). In vitro study of polyoxyethylene alkyl ether niosomes for delivery of insulin. Int J Pharm 328:130–41
- Peloquin CA, Bulpitt AE, Jaresko GS, et al. (1999). Pharmacokinetics of ethambutol under fasting conditions, with food, and with antacids. Antimicrob Agents Chemother 43:568–72
- Pereira-Lachataignerais J, Pons R, Panizza P, et al. (2006). Study and formation of vesicle systems with low polydispersity index by ultrasound method. Chem Phys Lip 140:88–97
- Qurrat-ul A, Sharma S, Khuller GK, Garg SK. (2003). Alginate-based oral drug delivery system for tuberculosis: pharmacokinetics and therapeutic effects. J Antimicrob Chemother 51:931–8
- Rogerson A, Cummings J, Florence AT. (1987). Adriamycin-loaded niosomes: drug entrapment, stability and release. J Microencap 4:321–8
- Ruckmani K, Jayakar B, Ghosal SK. (2000). Nonionic surfactant vesicles (niosomes) of cytarabine hydrochloride for effective treatment of leukemias: encapsulation, storage, and in vitro release. Drug Dev Ind Pharm 26:217–22
- Sathyavathi V, Abdul Hasansathali A, Ilavarasan R, Sangeetha T. (2012). Formulation and evaluation of niosomal in situ gel ocular delivery system of brimonidine tartrate. Pharm Sci 2:L-82–95
- Shi B, Fang C, Pei Y. (2006). Stealth PEG-PHDCA niosomes: effects of chain length of PEG and particle size on niosomes surface properties, in vitro drug release, phagocytic uptake, in vivo pharmacokinetics and antitumor activity. J Pharm Sci 95:1873–87
- Singh CH, Jain CP, Kumar BN. (2011a). Formulation, characterization, stability and in vitro evaluation of nimesulide niosomes. Pharmacophore 2:168–85
- Singh G, Dwivedi H, Saraf SK, Saraf SA. (2011b). Niosomal delivery of isoniazid-development and characterization. Trop J Pharm Res 10:203–10
- Thomas JP, Durr FE. (1983). Ethambutol dose-plasma level correlation studies in guinea pigs. Am Rev Respir Dis 127:352–3
- Uchegbu IF, Florence AT. (1995). Non-ionic surfactant vesicles (niosomes): physical and pharmaceutical chemistry. Adv Colloid Interface Sci 58:1–55
- Xu ZP, Zeng QH, Lu GQ, Yu AB. (2006). Inorganic nanoparticles as carriers for efficient cellular delivery. Chem Eng Sci 61:1027–40
- Yoshioka T, Sternberg B, Florence AT. (1994). Preparation and properties of vesicles (niosomes) of sorbitan monoesters (span-20, span-40, span-60 and span-80) and a sorbitan triester (span-85). Int J Pharm 105:1–6