Abstract
Over the years, research has focused on strategies to increase benefit/risk ratio of corticoids. However, vehicles intended for topical glucocorticoids delivery with an improved benefit/risk ratio are still on demand. The aim of this work was the in vitro and in vivo characterization of cold processed oil-in-water (o/w) emulsions intended for mometasone furoate (MF) delivery to induce drug targeting to upper skin strata, decreasing adverse effects. Two o/w emulsions, containing 0.1% of MF, were developed differing in the glycol used (2-methyl-2,4-pentanediol – PT and ethoxydiglycol – TC emulsions). In vitro permeation studies revealed that these emulsions are suitable vehicles for the delivery of MF containing ingredients which are responsible for a drastically increased on the permeability coefficients of MF from a theoretical value of 1.18 × 10−4 cm/h to 5.20 × 10−4 ± 2.05 × 10−4 cm/h and 6.30 × 10−4 ± 2.94 × 10−4 cm/h, for PT and TC, respectively. The tape stripping results showed that the amount of drug that reached the viable skin layers was very low (1.99 %) and the amount that remained in the stratum corneum (SC) was 10.61%. The in vivo studies showed that the developed formulations decreased the edema and erythema in mice skin in more that 90%, assuring, at least, the same anti-inflammatory effect compared with the commercial cream. PT placebo demonstrated to contribute to restore the skin barrier by increasing the amount of lipids within the human skin.
Introduction
The main barrier to the percutaneous absorption of topically applied drugs is the stratum corneum (SC). Due to the barrier nature of the SC, topically applied compounds may accumulate, i.e. the SC may serve as a reservoir from which substances can be subsequently absorbed over long periods of time (Bucks, Citation1984). The reservoir function of SC was first reported by Vickers (Citation1963), who demonstrated that topically applied corticosteroid forced into SC by occlusion for a few hours remained there for as long as 7–14 d.
Topical glucocorticoids (TG) are the most frequently prescribed drugs by dermatologists. Their clinical effectiveness in the treatment of psoriasis and atopic dermatitis is related to their vasoconstrictive, anti-inflammatory, immunosuppressive, and anti-proliferative effects (Kerscher et al., Citation1991; Wiedersberg et al., Citation2008). Mometasone furoate (MF) is a potent corticosteroid which presents an improved risk/benefit ratio. It is, therefore, of great value for inflammatory skin diseases, showing a strong anti-inflammatory action, rapid onset of action, and low systemic bioavailability after topical application (Teng et al., Citation2003; Valotis et al., Citation2004).
The target cells for TG are the keratinocytes and fibroblasts within the viable epidermis and dermis, where the glucocorticoid receptors are located. The transport across the cell membrane of TG is a non-mediated, passive diffusion process related to drug lipophilicity. After they reach the glucocorticoid-receptor, the complex is translocated into the nucleus to either stimulate or inhibit transcription and regulate thereby the inflammatory process. The inhibition of IL-1 α in keratinocytes has anti-inflammatory effects, whereas the same inhibition in fibroblasts has anti-proliferative and atrophogenic effects (Ahluwalia, Citation1998; Wiedersberg et al., Citation2008).
The key factors in the management of atopic dermatitis are not only related to the use of effective topical anti-inflammatory agents but also in providing skin hydration and barrier repair (Jung & Stingl, Citation2008), thus the ingredients selected to such vehicles are of extremely importance. Emollients are often used in the treatment of atopic dermatitis and other inflammatory dermatitis with the aim of improving skin hydration and mitigating xerosis. Given their resemblance to the lipids in the SC, beneficial effect of skin lipid supplementation both in composition and in the structuring of topical formulations for skin repair was already described (Coderch et al., Citation2002; Carneiro et al., Citation2011).
Complex approaches, such as iontophoresis, electroporation, eutectic mixtures (Banga et al., Citation1999), supersaturated systems (Davis & Hadgraft, Citation1991; Raghavan et al., Citation2000), lipid nanocarriers (Maia et al., Citation2002; Zhang & Smith, Citation2011; Doktorovová et al., Citation2010), classical, or deformable liposomes (Cevc & Blume Citation2004; Clares et al., Citation2009), have been studied in order to increase benefit/risk ratio of corticoids. The cost–benefit of these new dosage forms is often difficult to anticipate (Raposo et al., Citation2013a). To overcome this drawback, cold processed emulsions can be used. As they are easier to process due to the elimination of the phase of heating and cooling down, the time of production can be decreased, increasing production capacity (Shah et al., Citation2010) as well as decrease the energy and the water consumption which are translated in a decrease of production costs.
The aim of this work was the in vitro and in vivo characterization of cold processed oil-in-water (o/w) emulsions intended for MF delivery to induce glucocorticoid targeting to upper skin strata, decreasing adverse effects of TG. The role of the vehicles in providing skin hydration and lipid supplementation to the skin will also be discussed.
Materials and methods
Materials
MF was purchased from Crystal Pharma (Valladolid, Spain). Ethoxydiglycol (Transcutol® CG) was a gift from Gattefosse (Genas Saint-Priest cedex, France); 2-methyl-2,4-pentanediol (pentanediol), 99%, hidroxy propyl methyl cellulose (HPMC), and isopropyl myristate were obtained from Sigma Aldrich (Munich, Germany). Bis-PEG/PPG-16/16 PEG/PPG-16/16 dimethicone (and) caprylic/capric triglyceride (Abil® Care 85), PEG-20 glyceryl laurate (Tagat® L2), and C12-15 alkyl benzoate (Tegosoft® TN) were a gift from Evonik (Westfalen, Germany) and cetrimide BP was a gift from D.S Produtos Químicos (Aboboda, Portugal). Methyl vinyl ether/maleic anhydride copolymer cross-linked with decadiene (PVM/MA) (Stabileze® QM) was purchased from ISP (Württemberg, Germany).
Preparation of cold processed emulsions
MF-loaded cold processed emulsions were developed as described elsewhere (Raposo & Ribeiro, Citation2011; Raposo et al., Citation2013b). Briefly the oil in water emulsions was created, initially, by the preparation at room temperature of an oil liquid phase, achieved by dissolving the bis-PEG/PPG-16/16 PEG/PPG-16/16 dimethicone (and) caprylic/capric triglyceride and the co-emulsifier (PEG-20 glyceryl laurate) into the oils (C12-15 alkyl benzoate and isopropyl myristate) and mixing (Helipath® 130 rpm) at room temperature for about 30 min.
Next, an aqueous phase was prepared at room temperature by dispersing the aqueous thickening agents (HPMC and PVM/MA) in water. The cetrimide at 0.075 % w/w and the glycol (10% w/w; ethoxydiglycol or pentanediol) with the drug previously solubilized (0.1% w/w) were added to the aqueous solution and the resulting mixture was homogenized until a clear homogeneous gel was achieved. The emulsification phase was performed at room temperature by slowly adding the oil phase to the aqueous phase with high shear mixing at a rate about 12 800 rpm/min (IKA® T25 Ultra Turrax (IKA, Staufen, Germany)). The pH value was adjusted to 3.8–4.1 with sodium hydroxide 1 M. indicates the composition of the final formulations prepared by this process. Formulations PT and TC differ with regard to the type of glycol used, pentanediol or ethoxydiglycol, respectively, and PT placebo had the same composition of PT emulsion without MF.
Table 1. Qualitative and quantitative composition of the developed emulsions.
HPLC method for the determination of MF
A Hitachi Elite Lachrom System (VWR, Morris Plains, NJ,) equipped with four Pumps L-2130, an autosampler L-2200, a column oven L-2300, an UV Detector L-2400, and a software EZ Chrom Elite Version 3.2.1 were used in all chromatographic analysis. An analytical reversed-phase (RP) Lichrospher 100 RP18 (125 mm × 4 mm, 5 µm, Merck, Darmstadt, Germany) was used.
The method used an isocratic gradient mobile phase with 70% (v/v) methanol and 30% (v/v) water. A flow rate of 1.5 mL/min was used with a 10 µL injection volume. The auto-sampler chamber was maintained at 4 °C, and the eluted peaks were monitored at excitation and emission wavelengths of 248 nm. The run time was 11 min.
The experimental validation of the analytical method was performed in accordance with the CPMP/ICH/381/95 guideline (CPMP/ICH/381/95, Citation2005).
Solubility studies of MF in the glycols
Solubility studies of MF in the pure glycols
MF was added to ethoxydiglycol and pentanediol until saturation. Saturation was achieved when excess solid persisted for more than 12 h with constant shaking (200 rpm) at 22 °C. After ensuring that the solute–solvent equilibrium had been reached, the solution was centrifuged at 4000 rpm during 10 min and the supernatant solution diluted with methanol (1:10) and assayed using HPLC. Measures were performed in triplicate and data are displayed as mean ± SD.
Co-solvent solubility studies of MF in pentanediol–water and ethoxydiglycol–water mixtures
MF was added to a series of pentanediol–water and ethoxydiglycol–water mixtures varying from 100% water to 100% of the glycol and stirred at 200 rpm at 22 °C during 48 h. After ensuring that the solute–solvent equilibrium had been reached, the solution was centrifuged at 4000 rpm during 10 min and the supernatant solution diluted with methanol (1:10) and assayed using HPLC.
Physical and chemical stability of emulsions
Samples were stored for 60 d at room temperature (25 °C) and during 30 d at 40 °C. Before storage and on days 7, 30, and 60 after storage, samples were analyzed for macroscopic appearance, pH, and MF chemical stability. For every evaluation time points, an accelerated stability test was also performed centrifuging samples for 15 min at 5000 rpm. Macroscopic appearance was checked by visual inspection. The pH was measured by immersing the probe directly into the sample using a pH meter Metrohm® pH Meter 744 (Herisau, Switzerland), with a glass electrode. HPLC was used to analyze the chemical stability of MF.
In vitro release and permeation of MF from PT and TC emulsions
Release and permeation of MF from PT and TC emulsions were measured in infinite dose conditions using a hydrophilic polysulfone membranes filters 0.45 µm (Tuffryn®) from Pall Corporation (Washington, NY) with a diffusion area of 1 cm2 and human epidermis obtained from a surgical intervention to reduce abdominal mass in a healthy Caucasian female of 54 years of age, after ethical approval and informed consent. The silicone membranes were washed and equilibrated with ethanol/water 1:1 during 30 min and then mounted between the donor and receiver compartments on static vertical Franz diffusion cells (receptor volume: 3 mL, permeation area: 1 cm2). The skin was removed from the involucre, placed in ≈60 °C isotonic phosphate buffer at pH 7.4 until thawed, the excess of fat was carefully removed, and the epidermis was gently separated from the remaining tissue. The epidermis was visually inspected for any defects, and then cut into sections large enough to fit on Franz Cells.
Ethanol/water (50:50) was used as a receptor phase to assure perfect sink conditions in the whole experiment. It was constantly stirred with a small magnetic bar (200 rpm) and thermostated at 32 ± 0.5 °C throughout the experiments. The PT and TC emulsions were then applied (0.2 g) evenly on the surface of the membrane in the donor compartment and sealed by Parafilm® (Pechiney Plastic Packaging Company, Chicago, IL) immediately to prevent water evaporation.
Samples were collected from the receptor fluid at pre-determined time points – 1, 2, 3, 4, 5, and 6 h for release studies and 1, 2, 4, 6, 8, 24, 32, and 48 h for permeation experiment and replaced with an equivalent amount (200 µL) of receptor medium. The drug content in the withdrawn samples was analyzed by HPLC. Repeated measures design using at least six replicated cells for each formulation was used.
The data obtained from in vitro release studies were fitted to two different kinetic models:
(1) Zero order
(2) Higuchi model
The coefficient of determination (R2) was determined for each model as it is an indicator of the model’s suitability for a given dataset.
Skin permeation parameters
The cumulative amount of MF permeated (Qt) through excised human skin was plotted as a function of time and determined based on the following equation:
(3)
where Ct is the drug concentration of the receiver solution at each sampling time, Ci the drug concentration of the sample applied on the donor compartment, and Vr and Vs the volumes of the receiver solution and the sample, respectively, S represents the skin surface area (1 cm2). The slope and intercept of the linear portion (between 8 and 32 h) of the plot was derived by regression using the Prism1, V. 3.00 software (GraphPad Software Inc., San Diego, CA). MF fluxes (J, µg cm−2 h−1) through the skin, from the assayed formulations were calculated from the slope of linear portion of the cumulative amounts permeated through the human skin per unit surface area versus time plot. The permeability coefficients (Kp, cm h−1) were obtained by dividing the flux (J) by the initial drug concentration (C0) in the donor compartment applying Fick's second law of diffusion (4), and it was assumed that under sink conditions the drug concentration in the receiver compartment is negligible compared to that in the donor compartment.
(4)
In vitro tape stripping of PT emulsion
Three individual tape stripping experiments were performed for PT emulsion on human skin. The human skin was obtained from a surgical intervention to reduce abdominal mass in a Caucasian female of 54 years of age, after ethical approval and informed consent. The tissue was treated as described in In vitro release and permeation of MF from PT and TC emulsions section. After the fat being discarded, the two outermost skin layers (epidermis and dermis) were mounted between the donor and receiver compartments of static vertical Franz diffusion cells. The procedure adopted was the same described before. The formulations were kept in contact with the human skin for 24 h then the skin samples were rinsed with cotton and distilled water to remove the excess of formulation.
The adhesive films employed to remove the superficial SC layers were Scoth® tape (3 M, London, UK). Pressure was applied with the thumb covered in a vinyl glove to ensure a rolling movement and thus minimizing the influence of wrinkles. After applying pressure for 3 s, the tape was removed in a single rapid movement.
Fifteen sequential tape strips were used to separate the SC from epidermis and dermis, the 15 pieces were placed in conical tubes containing 50 mL of tetrahydrofuran/water (75:25, v/v). The remaining skin (viable epidermis and dermis) was cut in small pieces and also placed into conical tubes with 50 mL tetrahydrofuran/water (75:25, v/v). The tissues were left in contact with the extraction solvent for 24 h, then the SC and epidermis and dermis samples were homogenized using a hand-held tissue homogenizer (Polytron PT3000, Kinematica AG, Switzerland; 16.6 × 1000 rpm) for 3 min, sonicated for 20 min, centrifuged for 5 min at 3000 rpm, filtered through a 0.45 μm pore membrane, and assayed for MF content by HPLC. The amount of MF remained in the donor compartment was also quantified by HPLC.
In vivo anti-inflammatory activity studies
The croton oil-induced ear inflammation model for investigating anti-inflammatory effects of nonsteroidal and steroidal compounds (Young & De Young, Citation1989) was tested in female NMRI mice (23–25 g) purchased to Charles River (Cerdanyola del Vallés, Spain). Mice were used after 1-week acclimatization to the laboratory environment. All animal experiments were carried out with the permission of the local animal ethical committee in accordance with the EU Directive (2010/63/UE), Portuguese law (DR 129/92, Portaria 1005/92) and all the following legislations. The experimental protocol was approved by Direcção Geral de Veterinária.
The inside of an animal ear was challenged with 10 μL of 5% croton oil dissolved in acetone and left to dry. After the challenge, each animal was kept individually in a separate cage. One hour after the challenge, tested formulations (PT, TC emulsions 1 month after preparation and commercial cream with the same MF dose) were applied (10 μL) on the challenged area and left to dry. The resulting edema was determined 16 h later. The ear thickness was measured with a Mitutoya® micrometer with three readings per ear. The degree of edema inhibition was calculated as a percentage of inhibition, determined by comparing the drug treated group with untreated controls. Three mice were used per group.
Mouse ear histology
In order to evaluate the surroundings of the site of application, animals were sacrificed 16 h after the treatment with PT emulsion and commercial cream. An ear challenged with 10 μL of 5% croton oil dissolved in acetone was used as positive control and a native ear as negative control. The ears were resected and fixed in 10% buffered formalin solution. Tissue samples were processed for embedding in Parafin wax by routine protocol. About 5 µm thick sections were stained with hematoxylin and eosin (H&E). The slides were examined using a light microscopy (Axioscope camara Leica Software Leica Image Manage IM 50, Wetzlar, Germany) and 400× magnification images were acquired using Microsoft Image Composite Editor (Microsoft Research, Redmond, WA). The histopathological appearance of tissues was compared for structure changes and cell infiltration.
In vitro cytotoxicity
A spontaneously immortalized human keratinocyte cell line, HaCaT (CLS, Eppelheim, Germany) and mouse embryonic fibroblast cell line, NIH 3T3 – (ATCC CRL-1658) were grown in RPMI-1640® (Gibco, Paisley, UK) medium supplemented with 10% (w/V) fetal bovine serum (FCS, Life Technologies, Inc., UK), penicillin (100 IU/mL), and streptomycin (100 μg/mL) in a humidified 95% O2/5% CO2 environment at 37 °C. For the subculture, cells growing as monolayer were detached from the tissue flasks by treatment with 0.05% (w/V) trypsin/EDTA (Invitrogen, Warrington, UK).
To determine in vitro drug effects on cell viability, cells (cultured in 96-well microplates) were incubated with MF in solution (DMSO), PT and TC emulsions, and MF solubilized in ethoxydiglycol and pentanediol for 72 h, and cell viability was determined with the MTT assay as described in detail elsewhere (Cadete et al., Citation2012; Lopes et al., Citation2012). The percentage of viable cells was established relatively to cells treated with vehicle, for MF solubilized in the glycol (ethoxydiglycol or pentandiol), and PT and TC placebos for PT and TC emulsions containing MF, respectively. The inhibitory concentration (IC50) for the drug in DMSO was calculated using Graphpad Prism software v5.0 (GraphPad Software, Inc., San Diego, CA) by the sigmoidal curve fitting method. The IC50 obtained was used to determine the cell viability of the cells incubated with PT and TC emulsions and MF solubilized in ethoxydiglycol and pentanediol.
Biological effects of the PT placebo
The epidermal capacitance and skin surface lipids of the SC after application of PT placebo were evaluated with a Corneometer CM 820 and a Sebumeter SM 810 (C + K Electronics GmbH, Crailsheim, Germany) respectively. A uniform volunteers panel was chosen (n = 10, young healthy females – 18–25 y.a., same professional activity) and subjects were included in the study after written and informed consent. The formulation was applied in the forearm and the results were compared with a defined control area (anatomically equivalent) on the same forearm with any treatment. Measurements were performed under standardized conditions, at room temperature (Berardesca et al., Citation1997).
Data analysis
The data were analyzed using the ANOVA test (Kaleida Graph, version 4.0, Synergy Systems, Reading, PA) and expressed as the mean ± SE (standard error); p < 0.05 was considered to be statistically significant.
Results
Solubility studies of MF in the glycols
MF has a low solubility in water, but it is more soluble in glycols. MF presented five-fold higher solubility in ethoxydiglycol when compared with pentanediol (34.84 ± 0.59 mg/g and 6.96 ± 0.76 mg/g, respectively).
In order to understand the influence of the solubilizers in the percentage used in the emulsions, co-solvent solubility plots were done ().
Figure 1. Co-solvent solubility plot of MF in (a) pentanediol–water mixtures and (b) ethoxydiglycol–water mixtures at 22 °C. Measurements were performed at least in duplicate (mean ± SD).
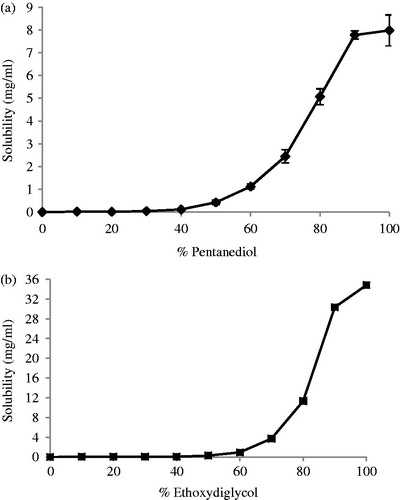
It was observed that the MF solubility in 10% of glycol in water, which corresponds to the percentage of glycol used in the emulsions, is not significant different (p > 0.05) for ethoxydiglycol and pentanediol (2.83 ± 0.76 and 3.04 ± 0.46 mg/100 mL).
Physical and chemical stability of emulsions
The stability of PT and TC emulsions in terms of drug content, pH values, and macroscopic characteristics during 60 d at ambient temperature () was performed.
Table 2. Stability test results for PT and TC emulsions during 60 d at 25 °C (n = 3; mean ± SD).
The emulsions remained white with a creamy and homogeneous aspect after 60 d. The stability of the emulsions was assessed by visual observation of the possible phase separation or other phenomena. After 30 d of storage at room temperature and at 40 °C, macroscopic observations did not reveal any sign of instability; however, after submission to centrifuge force TC emulsion stored at 40 °C presented a slightly phase separation. No significant changes were observed for the drug content which remained within the predefined limits 95–105%.
The MF presents their maximum stability in acidic conditions (Teng et al., Citation2003). In order to access the pH values stability of the emulsions, this parameter was evaluated during 60 d (). The results showed that the pH values are maintained during 60 d avoiding drug degradations than may occur in non-optimal conditions.
The acidity of the skin ranges from pH 4 to 6, thus both findings make these formulations suitable for topical application (Lodén, Citation2003).
In vitro release and permeation of MF from PT and TC emulsions
The results of the amount of MF released and permeated through the Tuffryn® membrane and the skin are shown in and , respectively. The in vitro data were fit to different equations and kinetic models to explain the profiles.
Figure 2. Release profile of MF from PT and TC emulsions through Tuffryn® membrane (mean ± SD, n = 6).
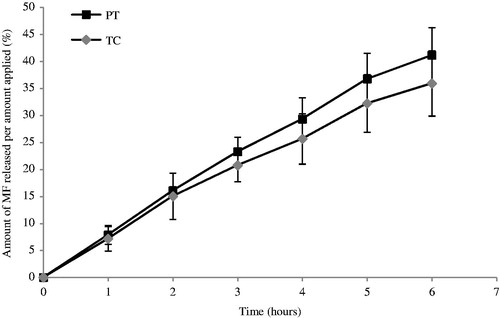
From the ANOVA statistical analysis, there were no significant differences between the release profiles of MF from these formulations (p > 0.05), thus the glycol seems not influence the release of MF from the matrix.
Regression analyses of each line were performed and the respective rates of release were determined from the lines slopes and are presented in . The release profiles were expressed by zero order (1) and Higuchi model (2).
Table 3. Kinetic parameters obtained after fitting the release data from the formulations to different release models.
Model fitting showed that both formulations followed a zero-order model, which had the highest values for R2 () and thus, statistically described best the drug release mechanism.
shows the permeation profiles of MF from PT and TC through human skin, presenting the cumulative amounts of drug permeated as a function of time, during 48 h.
The fluxes, permeability coefficients, and lag time () where obtained fitting the single curves of the permeation profiles in the linear region (between 8 and 32 h). There was no significant differences among PT and TC concerning the amount of MF permeated (p > 0.05).
Table 4. Flux, permeability coefficient (Kp) and lag time of MF through skin membrane (mean ± SD; n = 6) for PT and TC formulations.
In vitro tape stripping of PT emulsion
An extraction of the drug from the skin by tape stripping was performed for PT emulsion; the results are showed in .
The amount for MF extracted in the SC, in viable skin layers (epidermis and dermis), and in the donor compartment was analyzed by HPLC. The values of MF obtained were 30.82 μg (10.61 %) in the SC, and 5.72 μg (1.99 %) in the viable skin layers (epidermis and dermis) and 232.01 μg (80.56 %) in the donor compartment.
In vivo anti-inflammatory activity studies
Application of croton oil (5% in acetone) induced erythema and edema which was remarkably attenuated by the local application of both tested formulations (PT and TC) containing 0.1% MF (). The degree of edema inhibition is proportional to the anti-inflammatory activity of the formulation. Moreover, when cold processed emulsions were compared with the commercial cream containing the same amount of drug (0.1%), the results showed that there were no significant differences between the anti-inflammatory actions (p > 0.05).
Mouse ear histology
Histological analysis of the mice ear skin did not reveal morphological tissue changes neither cell infiltration signs after application of the PT emulsion, since the structure of the SC, epidermis, and dermis were preserved, as observed in . Moreover, comparing the PT emulsion with the commercial cream, the same trend is observed, the edema (observed after the application of croton oil, ) decreased significantly after the application of both formulations.
Figure 6. Effect of MF on croton oil-induced inflammatory cell infiltration of mouse ear. H&E-stained histological sections were prepared from ears resected 16 h after challenge: (a) unchallenged ear, (b) ear from mouse challenged with croton-oil in the absence of any treatment, (c) ear from mouse challenged with croton-oil post-treated with PT emulsion, and (d) ear from mouse challenged with croton-oil post-treated with commercial cream. Magnification: 40×.
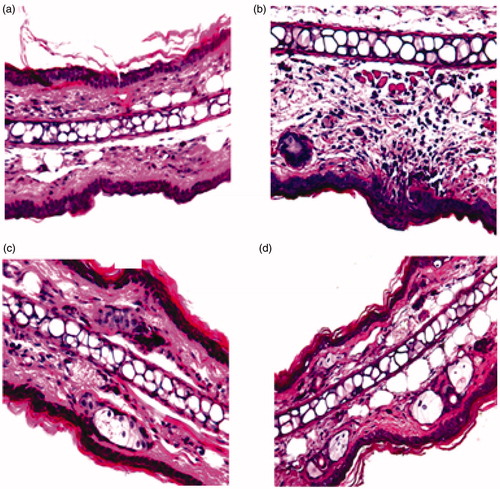
In vitro cytotoxicity
To investigate the potential cytotoxicity of the MF-loaded cold processed o/w emulsions, the cell viability was evaluated using NIH 3T3 and HaCaT cell lines in a MTT assay. First, the IC50 of the drug in solution (DMSO) was determined by non-linear regression analysis, and subsequently, the concentration found was used to determine the cell viability after application of PT and TC emulsions. The cytotoxicity of MF in ethoxydiglycol and pentanediol (0.1% w/w) was also analyzed.
The MF solubilized in the glycol or formulated in the emulsions presented different behaviors in terms of cell toxicity. It was found that the IC50 of the MF in DMSO was 5.1 × 10−6 g/mL, using the same concentration, it was observed that the glycols increased the cytotocixity of MF, which was more evident in NIH 3T3 cell line. However, it was found that the two emulsions were not cytotoxic in both NIH 3T3 and HaCaT cells on the concentration tested ().
Table 5. Cell viability in NIH 3T3 and HaCaT cell lines after 72 h of incubation with PT and TC emulsions and MF solubilized in ethoxydiglycol and pentandiol (mean ± SD; n = 6).
The incorporation of MF into the emulsions did not increase the cytotoxicity, in contrast to what happens when MF was solubilized in the glycol alone. Finally, the incorporation of the drug in emulsion protects cells both mouse fibroblasts and human keratinocytes from the cytotoxicity of the drug.
Biological effects of the PT placebo
The biological effects using human volunteers were tested using one of the developed formulations, PT emulsion. SC water retention and skin surface lipids properties are a crucial factor in keeping the skin supple and flexible and influence skin permeability to molecules. The methodological procedure chosen allowed the identification of positive results regarding skin water dynamics, expressed in terms of corneometry changes () and skin lipids expressed in terms of sebum ().
Figure 7. Comparison of skin hydration values in terms of capacitance during 21 d between PT placebo (black bars) and control (gray bars) (mean ± SD, n = 10).
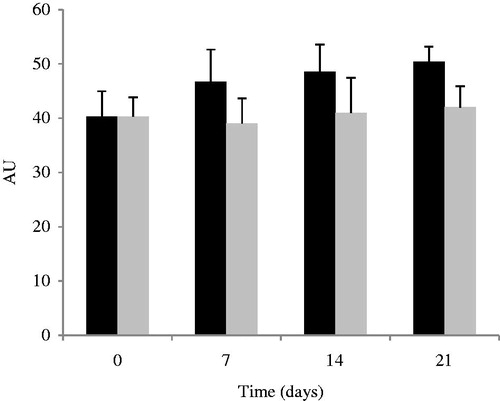
Figure 8. Effect of the application of PT placebo on the skin surface lipids. PT placebo (black bars) and control (gray bars) (mean ± SD, n = 10).
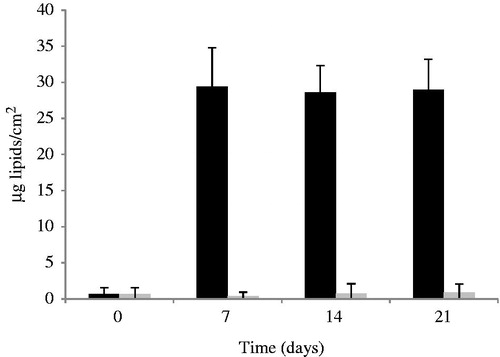
shows that the amount of lipids per cm2 drastically increased after the application of PT placebo.
Discussion
A cold process emulsification method for the preparation of PT and TC emulsions was used. According to Raposo (Citation2013b), this method allowed a total production savings of more than 17% when compared to the traditional hot process. This value is obtained comparing this method with a hot process emulsification method regarding the equipment costs per hour in terms of water and electrical costs, reactor amortization, and human resources costs per hour.
In this study, we demonstrated that the release and permeation profiles of MF from the emulsions were not influenced by the glycol used which was in accordance with the solubility results. It was been demonstrated that, at a given concentration, the release is ordinarily faster from the vehicle in which the drug is completely solubilized (Katz & Poulsen, Citation1972).
As demonstrated in previous studies (Raghavan et al., Citation2001; Fini et al., Citation2008), MF is insoluble in water and exponentially increases as the amount of glycol is increased. It was shown that in co-solvent mixtures, the solubility of MF was similar for the two glycols, around 3 mg/100 mL. As the solubility of MF was the same in pentanediol and ethoxydiglycol at 10% (w/v) and the concentration of MF is the same in the two emulsions, different release profiles were not expected.
The plot obtained of the cumulative drug amount in the acceptor versus time shows an increase with time, which approaches a constant slope as the experiment continues, this profile is typical in infinite dose experiments where the applied dose is so large that the depletion of the permeant in the donor chamber caused by evaporation or diffusion into and through the barrier is negligibly small (Selzer et al., Citation2013).
The fluxes, permeability coefficients, and lag time were obtained fitting the single curves of the permeation profiles in the linear region. The last point was not considered because according the OECD guidance (OECD, Citation2004), at this time, the skin barrier cannot be regarded as intact anymore. Moreover, as the receptor fluid is composed by water:ethanol (1:1), it is possible that evaporation of the receptor fluid occurs explaining the high standard deviations. Although co-solvents added to the receptor solution can alter the structure of the skin, it has been shown that the skin barrier function was not modified using as receptor solution 50% ethanol in water (Magnusson & Koskinen, 2000).
Potts & Guy (Citation1992) elegantly demonstrated that the permeability of a chemical from an aqueous solution through the SC could be estimated from only two parameters, the octanol/water partition coefficient and the molecular weight:
(5)
The Log P obtained by the Virtual Computational Chemistry Laboratory Munich, Germany, for MF was 2.81 (VCCLAB, Citation2005) and the published MW for this drug is 521.44 (Mometasone Furoate Drug Master File, Citation2006). Applying Pots and Guy Equation (Equation5(5) ), the value obtained for Kp is 3.27 × 10−8 cm/s, that is 1.18 × 10−4 cm/h.
The enhancement ratio between 2.7 and 7.8 comparing the theoretical and experimental values of Kp for MF emulsions can be explained regarding the excipients used. In fact, we used co-solvents, (ethoxydiglycol and pentanediol) which in addition to affecting the drug solubility in the vehicle, may alter the structure of the skin and modify the penetration rate (Miller et al., Citation1993). It is believed that glycols integrate into hydrophilic regions of the packed lipids and increase the distance in the lamellar phase by incorporation between the hydrophilic head groups of the bilayers and in the perpendicular direction to the bilayer. Moreover, the presence of isopropyl myristate, a well-known penetration enhancer, contributes to the percutaneous penetration of MF by a synergic mechanism. Isopropyl myristate affects the lipids of the SC and the partition coefficient between the SC and vehicle of both drug and solvent (Lane et al., Citation2012). It was demonstrated that the use of cosolvent in combination with a potential penetration enhancer may offer synergistic enhancement (Arellano et al., Citation1998; Goodman & Barry, Citation1989).
Not only the presence of HPMC, as explained before, contributed to the increase on Kp, but also the presence of surfactants. Surfactants can also act as penetration enhancers due to their potential for solubilizing SC lipids besides their capacity to interact with keratin, resulting in a disruption of order within the corneocytes (Förster et al., Citation2009). Moreover, it was demonstrated that glyceryl laurate enhanced the penetration of drugs through cadaverous skin and hairless rat skin in vitro (final report of the amended safety assessment of Glyceryl Laurate, Citation2004). The assemblement of these ingredients was translated in an enhancement of the Kp for MF through human epidermis.
The low amount of permeation (less than 4.0% after 48 h for PT and TC emulsions) can be explained by the reservoir effect largely described for the SC, which can have advantages for clinical applications of topical steroids.
The results obtained confirm the reservoir effect theory for SC, which can be positive for the treatment of skin diseases avoiding the adverse effects caused by the action of corticoids on fibroblasts receptors on dermis and minimize the systemic effects.
Cytotoxicity results showed that the MF solubilized in the glycols, presents a higher cytotoxicity, comparing to the free drug in both cell lines which could be explained by the enhancement effect of these glycols in the release of the MF. However, when incorporated in the emulsions, the cell viability increased to more than 90%. Moreover, according to the OECD guideline (OECD no. 439, Citation2010), an irritant substance is predicted if the mean relative tissue viability is found below 50% of the mean viability of the negative controls for a 15–60 min exposition time. In the present assay, cells were exposed to test samples for 72 h with the cell viability above 50%. Thus, the formulations can be considered non-irritant.
The in vivo studies for human skin hydration showed a slight increase after application of PT emulsion when compared to the control area. The principal mechanisms of hydration are humectancy, emolliency, and occlusion. The hydration provided by PT emulsion is attributed to humectants (glycols) and emollients (PEG-based surfactants, isopropyl myristate, and C12-15 alkyl benzoate). In fact, humectants promote water retention within the SC, whereas emollients smooth the skin by filling spaces between skin flakes and adding a complementary occlusive activity which contributes to SC hydration (Kraft & Lynde, Citation2005).
In contrast, a drastic increase on the skin lipids occurred after application of PT emulsion, these results can be explained by the mechanism of action of emollients as a role substitution of skin lipids by lipid ingredients from the formulation. Although barrier function requires cholesterol, free fatty acids, and ceramides, applications of exogenous non-physiologic lipids seem to contribute to the barrier function (Mao-Qiang et al., Citation1995). The increase on skin lipids is of great importance in impaired eczematous skin due to the ability to restore the lipid barrier, ability to attract, retain, and redistribute water. These findings suggest that not only the anti-inflammatory agent (MF) itself but also the formulation will play an important role in the treatment of skin disorders like atopic dermatitis.
It was demonstrated that the amount of sebum affects the permeability of skin to molecules and the presence of sebum on the forehead or forearm increased the diffusion of both hydrophilic and lipophylic molecules through the human skin (Tsai et al., Citation2012). The increase of the amount of lipids in the SC can, thus, explain the formulation effect on the enhancement of MF permeation across the human epidermis.
Conclusions
This study reports the in vitro and in vivo studies of MF emulsions obtained by cold process preparation method. In vitro release and permeation studies revealed that the glycols used had no influence on the release and permeation profiles of MF which was in agree with the solubility results for the two glycols. Moreover, it was demonstrated that these emulsions are suitable vehicles for the delivery of MF containing ingredients which are responsible for a drastically increased on the permeability coefficients of MF. It was demonstrated an accumulation of MF in the SC for PT emulsion which can decrease the adverse effects wildly described for topical corticoids. Additionally, PT vehicle demonstrated to contribute to restore the skin barrier by increasing the amount of lipids within the skin.
Acknowledgements
Authors gratefully acknowledge the support from Fundação para a Ciência e Tecnologia 610 (Portugal), FEDER (Doctoral Grant SFRH/BDE/33550/2009, Strategic Project PEstOE/SAU/UI4013/2011) and Laboratório Edol S.A.
The authors acknowledge the generous donation of many chemicals from Evonik (Germany) and Gattefossé (France). We are grateful to Dr. Paulo Salústio for the technical support in the release models as well as to Dr. Adelaide Ferreira to the support on histological tissues observations.
Declaration of interest
The authors declare no conflicts of interest.
References
- Ahluwalia A. (1998). Topical glucocorticoids and the skin–mechanisms of action: an update. Mediat Inflamm 7:183–93
- Arellano A, Santoyo S, Martın C, Ygartua P. (1998). Influence of propylene glycol and isopropyl myristate on the in vitro percutaneous penetration of diclofenac sodium from carbopol gels. Eur J Pharm Sci 7:129–35
- Banga A, Bose S, Ghosh T. (1999). Iontophoresis and electroporation: comparison and contrasts. Int J Pharm 179:1–19
- Berardesca E; The EEMCO Group. (1997). EEMCO guidance for the assessment of stratum corneum hydration: electrical methods. Skin Res Technol 3:126–32
- Bucks D. (1984). Skin structure and metabolism: relevance to the design of cutaneous therapeutics. Pharm Res 1:148–53
- Carneiro R, Salgado A, Raposo S, et al. (2011). Topical emulsions containing ceramides: effects on the skin barrier function and anti-inflammatory properties. Eur J Lipid Sci Technol 133:961–6
- Cadete A, Figueiredo L, Lopes R, et al. (2012). Development and characterization of a new plasmid delivery system based on chitosan–sodium deoxycholate nanoparticles. Eur J Pharm Sci 45:451–8
- Cevc G, Blume G. (2004). Hydrocortisone and dexamethasone in very deformable drug carriers have increased biological potency, prolonged effect, and reduced therapeutic dosage. Biochim Biophys Acta 1663:61–73
- Clares B, Gallardo V, Medina MM, Ruiz MA. (2009). Multilamellar liposomes of triamcinolone acetonide: preparation, stability, and characterization. J Liposome Res 19:197–206
- Coderch L, De Pera M, Fonollosa J, et al. (2002). Efficacy of stratum corneum lipid supplementation on human skin. Contact Dermatitis 47:139–46
- CPMP/ICH/381/95. (2005). GUIDELINE ICH. Q2 (R1), validation of analytical procedures: text and methodology. EU: Adopted by CPMP, November 94, 2–15
- Davis AF, Hadgraft J. (1991). Effect of supersaturation on membrane transport: 1. Hydrocortisone acetate. Int J Pharm 76:1–8
- Doktorovová S, Araújo J, Garcia ML, et al. (2010). Formulating fluticasone propionate in novel PEG-containing nanostructured lipid carriers (PEG-NLC). Colloids Surf B 75:538–42
- Final report of the amended safety assessment of Glyceryl Laurate, Glyceryl Laurate SE, Glyceryl Laurate/Oleate, Glyceryl Adipate, Glyceryl Alginate, Glyceryl Arachidate, Glyceryl Arachidonate, Glyceryl Behenate, Glyceryl Caprate, Glyceryl Caprylate, Glyceryl Caprylate/Caprate, Glyceryl Citrate/Lactate/Linoleate/Oleate, Glyceryl Cocoate, Glyceryl Collagenate, Glyceryl Erucate, Glyceryl Hydrogenated Rosinate, Glyceryl Hydrogenated Soyate, Glyceryl Hydroxystearate, Glyceryl Isopalmitate, Glyceryl Isostearate, Glyceryl Isostearate/Myristate, Glyceryl Isostearates, Glyceryl Lanolate, Glyceryl Linoleate, Glyceryl Linolenate, Glyceryl Montanate, Glyceryl Myristate, Glyceryl Isotridecanoate/Stearate/Adipate, Glyceryl Oleate SE, Glyceryl Oleate/Elaidate, Glyceryl Palmitate, Glyceryl Palmitate/Stearate, Glyceryl Palmitoleate, Glyceryl Pentadecanoate, Glyceryl Polyacrylate, Glyceryl Rosinate, Glyceryl Sesquioleate, Glyceryl/Sorbitol Oleate/Hydroxystearate, Glyceryl Stearate/Acetate, Glyceryl Stearate/Maleate, Glyceryl Tallowate, Glyceryl Thiopropionate, and Glyceryl Undecylenate. 2004. Int J Toxicol 23:55–94
- Fini A, Bergamante V, Ceschel G, et al. (2008). Control of transdermal permeation of hydrocortisone acetate from hydrophilic and lipophilic formulations. Pharm Sci Tech 9:762–8
- Förster M, Bolzinger MA, Fessi H, Briançon S. (2009). Topical delivery of cosmetics and drugs. Molecular aspects of percutaneous absorption and delivery. Eur J Dermatol 19:309–23
- Goodman M, Barry BW. (1989). Lipid–protein-partitioning (LPP) theory of skin enhancer activity: finite dose technique. Int J Pharm 57:29–40
- Jung T, Stingl G. (2008). Atopic dermatitis: therapeutic concepts evolving from new pathophysiologic insights. J Allergy Clin Immunol 122:1074–81
- Katz M, Poulsen B. (1972). Corticoid, Vehicle, and skin interaction in percutaneous absorption. J Soc Cosmet Chem 23:565–90
- Kerscher M, Williams S, Lehmann P. (1991). Topical treatment with glucocorticoids. In: Ruzicka T, Ring J, Przybilla B, eds. Handbook of atopic eczema. Berlin: Springer-Verlag, 483–4
- Kraft N, Lynde C. (2005). Moisturizers: what they are and a practical approach to product selection. Skin Therapy Lett 10:1–8
- Lane M, Santos P, Watkinson AC, Hadgraft J. (2012). Pasive skin permeation enhancement. In: Benson H, Watkinson AC, eds. Transdermal and topical drug delivery. Principles and practice. New Jersey: Wiley, 35–7
- Lodén M. (2003). Role of topical emollients and moisturizers in the treatment of dry skin barrier disorders. Am J Clin Dermatol 4:771–8
- Lopes R, Eleutério CV, Gonçalves LM, et al. (2012). Lipid nanoparticles containing oryzalin for the treatment of leishmaniasis. Eur J Pharm Sci 45:442–50
- Magnusson BM, Koskinen LD. (2000). In vitro percutaneous penetration of topically applied capsaicin in relation to in vivo sensation responses. Int J Pharm 195:55–62
- Maia CS, Mehnert W, Schaller M, et al. (2002). Drug targeting by solid lipid nanoparticles for dermal use. J Drug Target 10:489–95
- Mao-Qiang M, Brown B, Wu-Pong S, et al. (1995). Exogenous nonphysiologic vs physiologic lipids divergent mechanisms for correction of permeability barrier dysfunction. Arch Dermatol 131:809–16
- Miller II, Rao KJ, Goodwin YH, et al. (1993). Solubility and in vitro percutaneous absorption of tetracaine from solvents of propylene glycol and saline. Int J Pharm 98:101–11
- Mometasone Furoate Micronized ASMF, Drug master file, Crystal Pharma, 2006
- OECD. (2004). Guidance document for the conduct of skin absorption studies. Paris: OECD
- OECD Test No. 439. (2010). In vitro skin irritation: reconstructed Human epidermis test method, OECD guidelines for the testing of chemicals 1:1–18
- Potts RO, Guy RH. (1992). Predicting skin permeability. Pharm Res 9:663–9
- Raghavan SL, Trividic A, Davis AF, Hadgraft J. (2000). Effect of cellulose polymers on supersaturation and in vitro membrane transport of hydrocortisone acetate. Int J Pharm 193:231–7
- Raghavan SL, Trividic A, Davis AF, Hadgraft J. (2001). Crystallization of hydrocortisone acetate: influence of polymers. Int J Pharm 212:213–21
- Raposo S, Ribeiro HM. (2011). Composição e método de preparação a frio de uma emulsão utilizada como veículo de fármacos anti-inflamatórios in Portuguese Patents, PT 105982, Portugal
- Raposo S, Simões S, Almeida AJ, Ribeiro HM. (2013a). Advanced systems for glucocorticoids dermal delivery. Expet Opin Drug Deliv 10:857–77
- Raposo S, Salgado A, Eccleston G, et al. (2013b). Cold processed oil-in-water emulsions for dermatological purpose: formulation design and structure analysis. Pharm Dev Technol 19:417--29
- Selzer D, Abdel-Mottaleb MA, Hahn T, et al. (2013). Finite and infinite dosing: difficulties in measurements, evaluations and predictions. Adv Drug Deliv Rev 65:278–94
- Shah V, Besancon L, Stolk P, et al. (2010). The Pharmaceutical Sciences in 2020: report of a conference organized by the Board of Pharmaceutical Sciences of the International Pharmaceutical Federation (FIP). Pharmaceut Res 27:396–9
- Teng X, Cutler D, Davies N. (2003). Degradation kinetics of mometasone furoate in aqueous systems. Int J Pharm 259:129–41
- Tsai JC, Lu C, Lin M, et al. (2012). Effects of sebum on drug transport across the human stratum corneum in vivo. Skin Pharmacol Physiol 25:124–32
- Valotis A, Neukam K, Elert O, Högger P. (2004). Human receptor kinetics, tissue binding affinity, and stability of mometasone furoate. J Pharm Sci 3:1337–50
- VCCLAB. (2005). Virtual Computational Chemistry Laboratory, Available from: http://www.vcclab.org. [last accessed on 10 May 2013]
- Vickers CF. (1963). Existence of reservoir in the SC. Arch Dermatol 88:20–3
- Wiedersberg S, Leopold CS, Guy RH. (2008). Bioavailability and bioequivalence of topical glucocorticoids. Eur J Pharm Biopham 68:453–66
- Young JM, De Young LM. (1989). Cutaneous models of inflammation for the evaluation of topical and systemic pharmacological agents. In: Spector J, Back N, Liss AR, eds. Pharmacological methods in the control of inflammation. New York: Alan R. Liss, Inc., 215–31
- Zhang J, Smith E. (2011). Percutaneous permeation of betamethasone 17-valerate incorporated in lipid nanoparticles. J Pharm Sci 100:896–903