Abstract
Development of efficient ocular drug delivery systems was still a challenging task. The objective of this article was to develop a thermosensitive PEG–PCL–PEG (PECE) hydrogel and investigate its potential application for ocular drug delivery of diclofenac sodium (DIC). PECE block polymers were synthesized by coupling MPEG-PCL co-polymer using IPDI reagent, and then its sol–gel transition as a function with temperature was investigated by a rheometer. The results showed that 30% (w/v) PECE aqueous solution exhibited sol–gel transition at approximately 35 °C. In vitro release profiles showed the entrapped DIC was sustained release from PECE hydrogels up to 7 days and the initial drug loading greatly effect on release behavior of DIC from PECE hydrogels. MTT assay results indicated that no matter PECE or 0.1% (w/v) DIC-loaded PECE hydrogels were nontoxic to HCEC and L929 cells after 24 h culturing. In vivo eye irritation test showed that the instillation of either 30% (w/v) PECE hydrogels or 0.1% (w/v) DIC-loaded PECE hydrogels to rabbit eye did not result in eye irritation within 72 h. In vivo results showed that the AUC0–48 h of 0.1% (w/v) DIC-loaded PECE hydrogels exhibited 1.6-fold increment as compared with that of commercial 0.1% (w/v) DIC eye drops, suggesting the better ophthalmic bioavailability could be obtained by the instillation of 0.1% (w/v) DIC-loaded PECE hydrogels.
Introduction
Development of ocular controllable drug delivery system is still a challenging task for pharmaceutical scientists because of the normal ocular protective barriers such as blinking and tear drainage leading to the rapid drug clearance and low drug bioavailability after instillation (Casolaro et al., Citation2012; Li et al., Citation2012; Stella et al., Citation2012). Until to now, more than 90% of marketed ocular drug formulation in clinic therapy was in the form of eye drop due to its simplicity, safety and acceptance by patients (Diebold et al., Citation2007; Gan et al., Citation2012; Holden et al., Citation2012). However, the bioavailability of traditional eye drop was very poor; this might be attributed to the fact that most of the instilled drugs were lost within the first 15–30 s, and less than 5% of total drugs penetrated the corneal barrier to reach intraocular tissues (Seyfoddin et al., Citation2010). Furthermore, prominent efflux transporters that existed on ocular tissues such as p-glycoprotein (p-gp) and multidrug resistance protein (MRP) were also assumed to the poor bioavailability (Urtti, Citation2006; Shirasaki, Citation2008; Gupta et al., Citation2011). As a result, a frequent administration of drug was typically necessary to achieve therapeutic efficiency.
In past several decades, several ophthalmic drug delivery strategies including hydrogels, polymeric macro/nanoparticles, lipid-based nanocarriers, etc., have been developed to overcome these shortcomings and improve its bioavailability after instillation (Anumolu et al.,Citation2009; Asasutjarit et al., Citation2011; Baba et al., Citation2011; Chen et al., Citation2011; Casolaro et al., Citation2012; Li et al., Citation2013a). Among these strategies, the so-called smart or intelligent hydrogels as a platform for novel ocular drug delivery system have gained considerable attention, because it was expected to provide prolonged corneal retention time, reduced precorneal drug loss and convenience in administration as contrasted to conventional formulations (eye drops, suspension and ointment; Sultana et al., Citation2006; Chen et al., Citation2011; Casolaro et al., Citation2012). Furthermore, several hydrogel formulations have been clinically approved or are under clinical trials. More recently, Anumolu et al. (Citation2009) developed an in situ pilocarpine-loaded PEG hydrogels for sustained pharmacological response and the in vivo results showed that the in situ hydrogels formulations could significantly extend pharmacological response without any visible irritation. Our previous studies also illustrated that application of thermosensitive hydrogel (F-127 hydrogel) could significantly resist the blinking and flushing of tear, yet resulting in improvement of drug bioavailability after instillation (Li et al., Citation2013b).
In this study, we attempted to demonstrate the feasibility of a novel thermosensitive PECE hydrogels based on PEG and PCL that have been approved by FDA for various biomedical applications for improvement of drug bioavailability after instillation. The non-steroidal anti-inflammatory drug, diclofenac sodium (DIC) was used as model drug. The sol–gel transition of DIC-loaded PECE hydrogel as a function with temperature was investigated. In vitro cytotoxicity of the developed formulations and its in vitro release behavior was also studied. Furthermore, the in vivo pharmacokinetics test of our formulations reference to marketed DIC formulation was also tested in rabbit eye.
Materials and methods
Materials
MPEG550 and ɛ-CL were bought from Sigma-Aldrich (St. Louis, MO). 3-Isocyanatomethyl-3,5,5-trimethylcyclohexyl isocyanate (IPDI) and stannous octoate (Sn(Oct)2) were provided by Aladdin® (Shanghai, China). Diclofenac sodium (DIC) was obtained from Jinshan Chemical Co. Ltd (Wenzhou, China). Diclofenac sodium (DIC) eye drop was provided by Zhejiang eye hospital (Wenzhou, China). Milli-Q grade water (Millipore, Bedford, MA) was used for the preparation of solution and mobile phase.
Synthesis of PEG–PCL–PEG co-polymer
According to the previous report (Gong et al., Citation2009a), PEG550–PCL2200–PEG550 block polymer was synthesized by coupling MPEG550-PCL1100block polymer using IPDI reagent (Scheme 1). Briefly, 22 g ɛ-CL, 11 g MPEG and 0.4 ml Sn(Oct)2 were added into the three-neck bottle under the nitrogen stream and the reaction was kept at 130 °C for 6 h. Thereafter, 2.5 g IPDI was added into system at 80 °C for reaction for another 6 h, followed by cooling down to room temperature. The resultant product was dissolved in dichloromethane and precipitated using excess cold petroleum ether. Finally, the mixture was filtered and the crude product was dried at 40 °C overnight for further applications.
Preparation of PEG–PCL–PEG thermosensitive hydrogel
Briefly, a certain amounts of PEG–PCL–PEG block polymer were suspended into 2 ml distilled water solution, followed by heating to 55 °C for completely dissolution. Thereafter, the system was cooled down quickly to 4 °C to obtain the homogeneous solution. To determine the sol–gel transition of PEG–PCL–PEG solution as a function with temperature, the sol–gel transition was determined by the vial titling method. When the sample was non-flowable within 20 s, it was regards as the gel state.
Rheological studies
Rheology experiments were carried out by a rheometer (DHR-3, TA instrument) using a parallel-plate of 40 mm diameter. The samples were equilibrated at 4 °C for 2 min before the measurement. Rheological test parameters, storage moduli (G′) and loss moduli (G″) were monitored as a function of temperature at a 1 Hz frequency.
In vitro release study
In vitro release behavior of diclofenac sodium from the 30% (w/v) PEG–PCL–PEG hydrogels were performed at 37 °C in PBS solution (pH = 7.4). Briefly, 1 ml DIC/PECE aqueous solution (0.1, 0.2 and 0.4% DIC; w/v) was placed into a 10 ml test tube, followed by gelled at 37 °C for 10 min. Subsequently, 3 ml pre-warmed PBS solution (pH = 7.4) as release medium was added into test tube for periodical study. At predetermined time points, 1 ml of aliquot release medium was withdrawn for quantitative analysis by RP-HPLC method and the residual release medium was completely replaced with 3 ml freshly pre-warmed PBS solution for continuous study. RP-HPLC analysis was performed on a reversed phase C18 column (4.6 × 150 mm, 5 mm, ZORBAX Eclipse XDB-C18 (Agilent Technologies, Santa Clara, CA, USA)). The mobile phase was a mixture of acetonitrile and 0.1% triethylamine/phosphate buffer solution (65/35; v/v) at flow rate of 1.0 ml/min and the eluent was detected by a DAD detector at 276 nm.
In vitro cytotoxicity test
In vitro cytotoxicity of PECE and DIC/PECE composite solution against HCEC and L929 cells were measured by MTT assay. Cell lines were seeded on 96-well plates with a density of 1 × 104 cells in 0.1 ml growth medium per well. After 24 h of culturing, 0.1 ml PECE aqueous solution with various concentrations in rang of 0–800 μg/ml was added to wells for co-incubation. Untreated cells in growth medium were used as a control. After 24 h of incubation, 20 μl MTT solution (5 mg/ml) was added for each well and incubated for another 2 h. After that, the MTT solution was carefully removed from each well, and 150 μl DMSO was added to dissolve the MTT formazan crystals. The absorbance was recorded at 570 nm using an ELISA microplate reader (Bio-Rad, CA, USA). Cell viability (%) was calculated according to the following equation: cell viability (%) = absorption test/absorption control × 100%, where absorption control is the absorbance from control wells. All data are presented as the mean of six measurements (±SD).
In vivo eye irritation test
The in vivo eye irritation test of either 30% (w/v) PECE hydrogels or 0.1% (w/v) DIC-loaded PECE hydrogels was performed in a group of six New Zealand albino rabbits (∼2.5 kg). About 50 μl of either 30% (w/v) PECE hydrogels or 0.1% (w/v) DIC-loaded PECE hydrogels were instilled into the lower conjunctival sac of the rabbit’s right eye, while the left eye was instilled with Hank’s solution as a reference. After 15 min, 30 min, 120 min, 24 h, 48 h and 72 h of instillation, the changes of corneal, iris, conjunctiva and chemosis were monitored and the degree of eye irritation was calculated following the Draize test (Li et al., Citation2012).
Pharmacokinetics study
Six male New Zealand albino rabbits (∼2.5 kg) were purchased from Wenzhou Medical University Animal Center and fed under pathogen-free conditions. All experimental protocols and animal care were compiled with the Guide for the Care and Use of Laboratory Animals, Institute of Laboratory Animal Resources, and were approved by the Institutional Animal Care and Use Committee of Wenzhou Medical University. After anesthetize with urethane, six mature male New Zealand albino rabbits (∼2.5 kg) were divided into two groups (n = 3). Each group was instilled with 50 μl either 0.1% (w/v) DIC-loaded PECE hydrogels or the commercial 0.1% (w/v) DIC eye drops into the lower conjunctival sac of rabbit’s right eye. At predetermined time intervals (15 min, 30 min, 1 h, 2 h, 3 h, 4 h, 6 h, 8 h, 24 h and 48 h), 20 μl aqueous humor samples were collected using an insulin syringe (29 G) and then mixed with 80 μl mobile phase to remove the protein by centrifugation at 13 000 rpm/min for 10 min. Finally, the drug concentration in humor aqueous solution was detected by HPLC method as described in the “In vitro release study” section.
Statistics analysis
Data was analyzed using the software program Origin Pro 7.5 (Northampton, MA, USA). Statistical comparison between various groups was determined by one-way ANOVA analysis using SPSS software (Chicago, IL, USA) (p ≤ 0.05).
Results and discussion
Preparation of PEG–PCL–PEG thermosensitive hydrogel
Since the Perret & Skoulios (Citation1972) synthesized a series of block polymers for the first time based on PEG and PCL segments, these di/triblock polymers have been extensively studied for various biomedical applications. In this article, triblock polymers of PEG–PCL–PEG (PECE) were successfully synthesized by coupling MPEG-PCL diblock polymer using IPDI reagent (Scheme 1 and Supplementary Material). Due to the amphiphilic property of triblock polymers, PECE aqueous solutions showed thermoreversible properties with the evolution of temperature. As presented in , it was clearly observed that the 30% (w/v) PECE solution was flowable liquid at lower temperature (4 °C), while turned into the unflowable gel at physiological temperature (37 °C). The sol–gel transition behavior of PECE aqueous solution as a function with temperature might be driven by the micelle packing and aggregation mechanism, which was in accordance with previous studies (Gong et al., Citation2009a,b). Furthermore, entrapment of various amount of DIC (0.1, 0.2 and 0.4% w/v) into PECE aqueous solution did not influence its sol–gel transition behavior (data not shown), indicating that the developed PECE hydrogels might be a versatile vehicle for ocular drug delivery of various hydrophilic drugs.
Rheological studies
To understand the sol–gel transition process of 30% (w/v) PECE hydrogel, temperature sweep measurements were performed. More specifically, the storage modulus (G′) represented the elastic behavior and the loss modulus (G″) denoted the viscous behavior were monitored as a function with temperature. As illustrated in , it was clearly observed that the G″ was greater than G′ as temperature was lower than 20 °C, corresponding to the sol state of system. As gelation proceeds, the G′ was equal to G″, indicating the sol–gel transition temperature of system. Subsequently, G′ and G″ increased further with increasing temperature, reaching its highest values of 24 507 Pa and 6600 Pa at about 35 °C, respectively. Thereafter, with temperature greater than 45 °C, the PECE polymer was precipitated out of solutions and hence gel strength was lost. The similar phenomenon was also observed by Gong et al. (Citation2009a,Citationb), who illustrated that the molecular motion of PCL segment was strongly accelerated above 45 °C, yet resulting in the transition from gel phase to sol phase.
In vitro release study
The in vitro release behavior of diclofenac sodium from 30% (w/v) PECE hydrogels was investigated in PBS (pH = 7.4) at 37 °C. As shown in , it is clearly observed that the diclofenac sodium entrapped in the PECE hydrogels shows a sustained drug release up to 7 days with 60–100% of total drug being released from different formulations. From , we also find that the initial drug loading had great effect on the release behavior of diclofenac sodium from hydrogels. A statistically significant (p < 0.05) decrease in drug release was observed as the diclofenac sodium concentration increased in hydrogels. More specifically, 0.1% (w/v) DIC/PECE composite hydrogels had the fastest release rate with nearly 100% of total drug released within 96 h, while 0.2 and 0.4% (w/v) DIC/PECE composite hydrogels showed the slower drug release rate with approximately 60% of total drug released within 168 h. As well known to us, drug release from hydrogels was mainly governed by several factors, such as the nature and molecular weight of the drug, the porosity of hydrogels and its release medium type (Anumolu et al., Citation2009; Casolaro et al., Citation2012; Holden et al., Citation2012). Due to the great water solubility of diclofenac sodium, it could be easily diffused through the pore of hydrogels, yet resulting in the controllable drug release manner.
In vitro cytotoxicity test
The development of an effective and non-toxic vehicle is still a challenge task for ocular drug delivery. In this article, the MTT assay was employed to examine the influence of formulation on the metabolic activity of L-929 mouse fibroblasts (L929) and Human corneal epithelium cells (HCEC). This method was a standard assay of cell viability, which was based on the colorimetric analyses of living cells (Bauer et al., Citation2012). In preliminary experiments, we investigated the cytotoxicity of pure PECE solution (0–800 μg/ml) against HCEC and L929 after 24 h culturing. Untreated cells were used as a control. As depicted in , it was clearly observed that the presence of PECE polymers up to 800 μg/ml had no apparent influence on cell viability (cell viability was nearly 100%) against HCEC and L929 cells after 24 h culturing. According to the ISO 10993-5, the cell viability greater than 70% was generally regarded as non-toxic (Kronek et al., Citation2011). From these results, we could conclude that the synthesized PECE polymers were non-toxic to cells, which might be suitable for various drug delivery applications. Furthermore, we investigated the cytotoxicity of 0.1% (w/v) DIC-loaded PECE hydrogels against HCEC and L929 cells. From , we could find that the 0.1% (w/v) DIC-loaded PECE hydrogels also showed non-toxic to HCEC and L929 cells after 24 h culturing.
In vivo eye irritation test
As a novel vehicle for ocular drug delivery, it is very important to evaluate not only the biopharmaceutical properties but also the ocular tolerability. To examine the ocular tolerability of the developed formulations, an eye irritation experiments was evaluated by using Draize test protocol in rabbit eye (Li et al., Citation2012, Citation2013b). The in vivo results showed that no clinical signs such as corneal opacity, conjunctival redness and abnormality of the iris or changes in body weight were observed in any groups after the instillation of either 30% (w/v) PECE hydrogels or 0.1% (w/v) DIC-loaded PECE hydrogels within 72 h. Taking into account that rabbit eye was more susceptible to extraneous substance than the human eye (Baba et al., Citation2011), it could conclude that the DIC-loaded PECE hydrogels with favorable ocular tolerability are suitable for ocular drug delivery.
In vivo pharmacokinetics study
The concentration–time curves of DIC in aqueous humor after the instillation of 50 μl 0.1% (w/v) commercial DIC eye drops and 0.1% (w/v) DIC-loaded PECE hydrogels are illustrated in . The peak aqueous humor concentration (Cmax) of 0.23 ± 0.12 μg/ml was achieved at 1 h after instillation of 0.1% (w/v) commercial DIC eye drops, while the peak aqueous humor concentration (Cmax) of 0.44 ± 0.19 μg/ml was also achieved at 1 h after instillation of 0.1% (w/v) DIC-loaded PECE hydrogels. The areas under the concentration–time curve (AUC0–48 h) calculated from the whole period of experiment were 2.21 ± 0.96 and 3.67 ± 0.65 μg h/ml, respectively, for DIC eye drops and DIC-loaded PECE hydrogels (). The drug Cmax was achieved practically at the same time for both formulations, despite the developed thermosensitive hydrogel showed a sustained release behavior of DIC from hydrogel compared with commercial DIC eye drops. This might be explained by that the commercial formulations released DIC faster than that the thermosensitive hydrogels after contact with corneal surface, allowing a greater drainage of the drug, and decreasing its ability to permeate the cornea (Chen et al., Citation2011; Gratieri et al., Citation2011; Gan et al., Citation2012). As shown in , it was clearly observed that the AUC0–48 h of 0.1% (w/v) DIC-loaded PECE hydrogels exhibited 1.6-fold increment as compared to the 0.1% (w/v) commercial DIC eye drops. This result suggested that the better ophthalmic bioavailability could be obtained by the instillation of 0.1% (w/v) DIC-loaded PECE hydrogels.
Figure 5. DIC aqueous humor concentrations in rabbits after the instillation of the following: (1) 0.1% (w/v) DIC eye drops; (2) 0.1% (w/v) DIC-loaded PECE hydrogels/Data represent mean ± SD (n = 3).
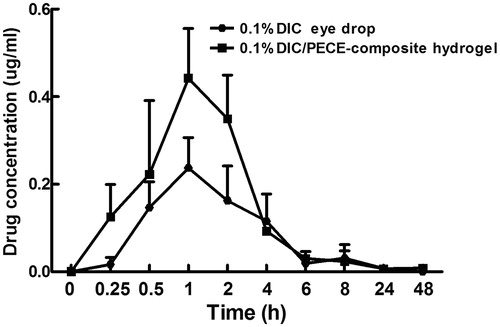
Table 1. Pharmacokinetic parameters of DIC in aqueous humor of rabbits (mean ± SD; n = 3).
Conclusion
In this study, a thermosensitive PECE tri-block polymer was successfully synthesized by coupling MPEG-PCL block polymer and its sol–gel transition as a function with temperature was monitored by a rheometer. 30% (w/v) PECE aqueous solution had sol–gel transition at about 35 °C and entrapment of various concentrations of DIC did not influence its sol–gel transition temperature. In vitro release profiles showed that entrapped DIC was released from PECE hydrogels up to 7 days and the initial drug loading greatly effected the release behavior of DIC from PECE hydrogels. In vivo pharmacokinetics test showed that the developed thermosensitive PECE hydrogels could increase the diclofenac sodium content absorbed into the eyes, yet resulting in the better ophthalmic bioavailability of DIC than that of the commercial DIC eye drop. As a result, we conclude that the developed thermosensitive hydrogel formulation can be used as an alternative to conventional DIC eye drops to increase its ophthalmic bioavailability and patient compliance.
Supplemental Material.pdf
Download PDF (84.3 KB)Declaration of interest
The authors report no conflicts of interest. The authors alone are responsible for the content and writing of this article. This research was supported by the Natural Science Foundation of China (NSFC51303136) and the Specialized Research Fund for the Doctoral Program of Higher Education (20133321120001).
References
- Anumolu SS, Singh Y, Gao D, et al. (2009). Design and evaluation of novel fast forming pilocarpine-loaded ocular hydrogels for sustained pharmacological response. J Control Release 137:152–9
- Asasutjarit R, Thanasanchokpibull S, Fuongfuchat A, Veeranondha S. (2011). Optimization and evaluation of thermoresponsive diclofenac sodium ophthalmic in situ gels. Int J Pharm 411:128–35
- Baba K, Tanaka Y, Kubota A, et al. (2011). A method for enhancing the ocular penetration of eye drops using nanoparticles of hydrolysable dye. J Control Release 153:278–87
- Bauer M, Lautenschlaeger C, Kempe K, et al. (2012). Poly(2-ethyl-2-oxazoline) as alternative for the stealth polymer poly(ethylene glycol): comparison of in vitro cytotoxicity and hemocompatibility. Macromol Biosci 12:986–98
- Casolaro M, Casolaro I, Lamponi S. (2012). Stimuli-responsive hydrogels for controlled pilocarpine ocular delivery. Eur J Pharm Biopharm 80:553–61
- Chen X, Li X, Zhou Y, et al. (2011). Chitosan-based thermosensitive hydrogel as a promising ocular drug delivery system: preparation, characterization, and in vivo evaluation. J Biomat Sci Polym E 27:391–402
- Diebold Y, Jarrín M, Sáez V, et al. (2007). Ocular drug delivery by liposome-chitosan nanoparticle complexes (LCS-NP). Biomaterials 28:1553–64
- Gan L, Wang J, Jiang M, et al. (2012). Recent advances in topical ophthalmic drug delivery with lipid-based nanocarriers. Drug Discov Today 18:290–7
- Gong C, Shi S, Dong P, et al. (2009a). Synthesis and characterization of PEG-PCL-PEG thermosensitive hydrogel. Int J Pharm 365:89–99
- Gong C, Shi S, Wu L, et al. (2009b). Biodegradable in situ gel-forming controlled drug delivery system based on thermosensitive PCL-PEG-PCL hydrogel. Part 2: sol–gel–sol transition and drug delivery behavior. Acta Biomater 5:3358–70
- Gratieri T, Gelfuso GM, de Freitas O, et al. (2011). Enhancing and sustaining the topical ocular delivery of fluconazole using chitosan solution and poloxamer/chitosan in situ forming gel. Eur J Pharm Biopharm 79:320–7
- Gupta H, Aqil M, Khar RK, et al. (2011). Biodegradable levofloxacin nanoparticles for sustained ocular drug delivery. J Drug Target 19:409–17
- Holden CA, Tyagi P, Thakur A, et al. (2012). Polyamidoamine dendrimer hydrogel for enhanced delivery of antiglaucoma drugs. Nanomed Nanotechnol 8:776–83
- Kronek J, Kroneková Z, Lustoň J, et al. (2011). In vitro bio-immunological and cytotoxicity studies of poly (2-oxazolines). J Mate Sci Mater Med 22:1725–34
- Li X, Zhang Z, Li J, et al. (2012). Diclofenac/biodegradable polymer micelles for ocular applications. Nanoscale 4:4667–73
- Li X, Li L, Zhang Z, Chen H. (2013a). An overview on pharmacokinetics, disposition, and safety of nanoparticles in ocular applications. Curr Drug Metab 14:857–62
- Li X, Zhang Z, Chen H. (2013b). Development and evaluation of fast forming nano-composite hydrogel for ocular delivery of diclofenac. Int J Pharm 448:96–100
- Perret R, Skoulios A. (1972). Crystallization of trisequenced poly (ɛ-caprolactone)/poly (oxyethylene)/poly (ɛ-caprolactone) copolymers. I. Copolymers with sequences of very different lengths. Makromol Chem 162:147–62
- Seyfoddin A, Shaw J, Al-Kassas R. (2010). Solid lipid nanoparticles for ocular drug delivery. Drug Deliv 17:467–89
- Shirasaki Y. (2008). Molecular design for enhancement of ocular penetration. J Pharm Sci 97:2462–96
- Stella B, Arpicco S, Rocco F, et al. (2012). Nonpolymeric nanoassemblies for ocular administration of acyclovir: pharmacokinetic evaluation in rabbits. Eur J Pharm Biopharm 80:39–45
- Sultana Y, Jain R, Aqil M, Ali A. (2006). Review of ocular drug delivery. Curr Drug Deliv 3:207–17
- Urtti A. (2006). Challenges and obstacles of ocular pharmacokinetics and drug delivery. Adv Drug Deliver Rev 58:1131–5