Abstract
There is no doubt that controlled and pulsatile drug delivery system is an important challenge in medicine over the conventional drug delivery system in case of therapeutic efficacy. However, the conventional drug delivery systems often offer a limited by their inability to drug delivery which consists of systemic toxicity, narrow therapeutic window, complex dosing schedule for long term treatment etc. Therefore, there has been a search for the drug delivery system that exhibit broad enhancing activity for more drugs with less complication. More recently, some elegant study has noted that, a new type of micro-electrochemical system or MEMS-based drug delivery systems called microchip has been improved to overcome the problems related to conventional drug delivery. Moreover, micro-fabrication technology has enabled to develop the implantable controlled released microchip devices with improved drug administration and patient compliance. In this article, we have presented an overview of the investigations on the feasibility and application of microchip as an advanced drug delivery system. Commercial manufacturing materials and methods, related other research works and current advancement of the microchips for controlled drug delivery have also been summarized.
Introduction
In bygone days, synthesis or discovery of new chemical entities, with desirable therapeutic properties but without undesirable side effects, was one of the major goals of pharmaceutical research. Pharmacokinetic and pharmacological studies have revealed that the rate and extent of drug absorption rather than the dose, ultimately regulate therapeutic properties in systemic treatment (Urquhart et al., Citation1984). This led to the development of drug delivery systems that achieve pharmacological selectivity not solely on the basis of chemical structure but also on the basis of controlled rate of drug delivery (Goldman Citation1982; Banerjee & Robinson, Citation1991). At present, controlled drug delivery technology is one of the most rapidly advancing areas of science for human health care (Langer, Citation1990) which are intelligent enough to respond to the behavior of the surrounding physiological environment (Razzacki et al., Citation2004). Many chronic medical conditions require taking specific drugs at specific dose levels on a regular/periodic basis (Polla et al., Citation2000) but patients often overlook, are unwilling, or are unable to take their medication during long time using of medications (Santini et al., Citation1998; Kuo & Violante, Citation2012). Due to the improving the limits of conventional drug administration, a variety of devices for controlled drug delivery have been developed in last few years. Among them micropumps, osmotics pumps and microelectromechanical systems (MEMS) based drug delivery devices are used which are developed by different techniques for device fabrication and used in different rout of administration (Sharma et al., Citation2006). Over the last few years, the advent of microfabrication technology has been successfully applied on the development of a variety of microelectromechanical systems (MEMS) for health care-related products including diagnostic (lab-on-a-chip) systems and techniques and apparatus for high throughput screening of new drug entities (Gourley, Citation1996; Drews, Citation2000; Tao & Desai, Citation2003).
The use of traditional microfabrication techniques are the recent and alternative method of creating drug delivery platforms and these are the same processing techniques for manufacturing the microelectronic chips (Breimer, Citation1999; Tao & Desai, Citation2003). MEMS based devices have the potential to store the drugs in their most stable form, and release multiple medications at the appropriate time at which to dose each drug by opening various reservoirs on command (Maloney, Citation2003; Grayson et al., Citation2004a; Bramstedt, Citation2005). Such as, implantable microchips, a MEMS based drug delivery system, can be used for long-term treatment, complex dosing schedule, and/or an individualized or emergency-based dosing regimen (Staples et al., Citation2006; Staples, Citation2010). At this age of rapid advances in computer technology, it may not be long before microchips find their way from the desktop to the pharmacy (Langer, Citation1990). In this review, we retrieve to highlight the MEMS based implantable microchip as controlled drug delivery device, including its microfabrication techniques and some recent advent of microchips as drug delivery system by scientists and pharmaceutical drug delivery device manufacturers.
Controlled release and pulsatile release of drug
Controlled release refers to materials or devices for controlling the release time of a chemical, the release rate or both (Langer, Citation1990). In general, injected or ingested drugs follow first-order kinetics, with initial high blood levels of the drug after the first administration, followed by an exponential fall in blood concentration which often can show toxicity and diminished efficacy respectively. That's why sustained zero-order release () of a therapeutic agent over a prolonged period of time is required for controlled drug delivery system which will ultimately reduce the toxicity (Santini et al., Citation2000b; Siegel & Rathbone, Citation2012).
Figure 1. Example of plasma drug concentration versus time for conventional and controlled release drug delivery device. Here, the controlled drug delivery profile is characterized as sustained release.
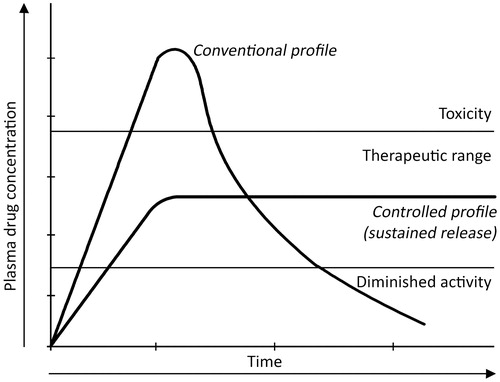
Usually, sustained or pulsatile drug release is achieved by using controlled release of drug delivery (Hilt & Peppas, Citation2005) which means the rapid and transient release of a certain amount of molecules within a short time-period immediately after a predetermined off-release period (; Jain et al., Citation2011). This delivery method works better in certain cases because it closely mimics the way in which the human body naturally produces some compounds (Santini et al., Citation2000a). Insulin (Kikuchi & Okano, Citation2002; Grayson et al., Citation2003; Chung et al., 2008) and the hormones of the anterior pituitary gland, for example gonadotropin (GnRH) and growth hormone (Grayson et al., Citation2003), are well-known to be secreted by the body in a pulsatile manner (Hilt & Peppas, Citation2005).
Figure 2. Showing plasma drug concentration versus time curve for controlled release and pulsatile release profile of zero-order kinetics.
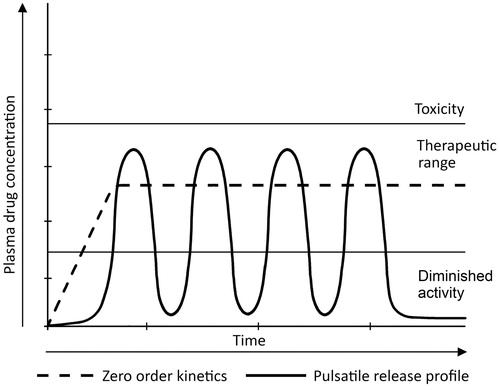
Controlled drug delivery systems over the conventional drug delivery systems, e.g. oral tablets, injections, pulmonary, transdermal (Shawgo et al., Citation2002; Tao & Desai, Citation2003; Hilt & Peppas, Citation2005; Chien & Lin Citation2006), aerosols and ointments (Langer, Citation1990) could provide one or more of the following benefits compared to conventional dosage forms including (Langer, Citation1990; Putney & Burke, Citation1998; Medlicott & Tucker, 1999; Hilt & Peppas, Citation2005; Chien & Lin Citation2006; Jain et al., Citation2011; Lee et al., Citation2012; Siegel & Rathbone, Citation2012) the following factors.
Maximization of efficacy–dose relationship.
Reduced toxicity and intensity of side effects.
Protection of mucosa from irritating drugs.
Enhancement of patient compliance and convenience.
Controlled administration of a therapeutic dose at a desirable rate of delivery.
Maintenance of drug concentration within an optimal therapeutic range for prolonged duration of treatment.
Predictable and prolonged time for action of drug with less frequent administration or eliminating the need for specialized drug administration (e.g. repeated injections).
Reduced variability of performance of drug products.
Preservation of medications that are rapidly destroyed by the body (biologically sensitive molecules such as proteins).
Reduced need for follow-up care.
Overview on microfabrication technologies
In last 30 years, the revolution of integrated circuits and microelectronics has fueled the subsequent development of additional microtechnology devices (Schulz, Citation1999). Drug delivery remains a vital challenge in medicine and microfabrication techniques may be utilized to develop novel drug delivery devices which are not convenient with current systems. Conventionally, microelectromechanical systems (MEMS) are utilized for the production of micron scale functional devices, such as sensors, switches, filters and gears, from silicon, the dominant material used throughout the IC industry (Sant et al., Citation2012). Microfabrication techniques can be considered as surface or bulk micromachining, which are based on IC manufacturing technologies. Surface micromachining or additive process is the fabrication of micromechanical structures from deposited thin films, such as silicon nitride, polycrystalline silicon and other materials (Wolf & Tauber, 1986; Bustillo et al., Citation1998). On the other hand, bulk micromachining or subtractive process uses the selective removal of significant amounts of silicon, or other material, from a substrate to form microstructures (Kovacs & Knapp, Citation1998). Furthermore, these fabrication processes have been utilized to manufacture the sensors, actuators and microdevices, including pressure sensors, accelerometers, flow sensors, ink-jet printer heads and micromirrors for projection (Hilt & Peppas, Citation2005). For microfabrication, the most important techniques are photolithography, soft lithography, film deposition, etching and bonding (Betancourt & Brannon-Peppas, Citation2006). These devices are fabricated by the repetitive application of unit process steps such as thin-film deposition, photolithography and etching. Such microfabrication techniques allow for the accurate control over surface microarchitecture, topography and feature size (Tao & Desai, Citation2003).
Microscale devices inherently have many benefits over their conventional counterparts. Advanced micro- and nano-technologies have enhanced the development of new drug delivery technologies for the transformation of biological prospective into clinical authenticity (LaVan et al., Citation2002; Langer & Peppas, Citation2003). Till date, a miniature device can be the prime element to the manufacture of portable, hand-held, or implantable devices, which is highly desirable for drug delivery devices. These minute size microdevices typically need less than a microliter of drug, which saves money and time. In addition, the utility of microtechnology to tailor the size, shape, reservoir number, reservoir volume, unidirectional openings and surface characteristics of the drug delivery devices in conjunction with appropriate surface chemistry, are potentially influential in the area of controlled release. Moreover, microfabrication causes the simple integration of electronic elements into microfabricated devices which permits the straightforward control and manipulation of the operation of the device (Tao & Desai, Citation2003; Hilt & Peppas, Citation2005).
Implantable controlled release microchips: design and components
It can be hard to accurately tailor the shape of the drug-loaded systems with conventional drug delivery systems to control the drug delivery precisely (Santini et al., Citation2000b; Lee et al., Citation2012). To overcome these precincts, one of the most sophisticated methods, microfabrication technology for MEMS has been implemented for drug delivery systems (Polla et al., Citation2000; Webb, Citation2004; Sharma et al., Citation2006) which is the same processing techniques used to make microprocessors for computer microchips (Santini et al., Citation2000b). MEMS technology has been used to create reservoirs on silicon chips that can be filled with drug, reservoir seals can be added to protect and contain the drug, and device circuitry can be designed and programmed to open seals on individual reservoirs and release drug on command (Staples, Citation2010). A highly programmable drug release at the site of action is one of the most important benefits of these microfabricated drug delivery systems which improve drug bioavailability (Hilt & Peppas, Citation2005). Multiple fabrication steps of photolithography, deposition and etching have been utilized to achieve a programmed drug release device with required three-dimensional structure (Ziaie et al., Citation2004).
Santini, Cima and Langer, at the Massachusetts Institute of Technology, developed the first experimental demonstration of an electrochemically activated solid-state silicon drug delivery microchip with potential application in drug delivery which was reported in the journal Nature in Citation1999 (Santini et al., Citation1999; Dario et al., Citation2000; Lesniak & Brem, Citation2004; Gardner, 2006; Farra et al., Citation2012; Stevenson et al., Citation2012). In this depicted microchip, a thin gold membrane, (Santini et al., Citation2000b; Betancourt & Brannon-Peppas, Citation2006; Sharma et al., Citation2006) capped drug reservoirs in silicon (Stevenson et al., 2006) serves as an anode in an electrochemical reaction (Dario et al., Citation2000; Santini et al., Citation2000b). Furthermore, the purpose for developing a microfabricated device was to avoid the moving parts, but it was capable to store and release multiple chemical entities. The size of that experimental prototype was 17 mm × 17 mm × 310 µm square pyramidal reservoirs which were etched completely through the wafer (Tao & Desai, Citation2003) which can accommodate over 1000 reservoirs (Santini et al., 2000a). The thickness of the silicon substrate varied between 295 and 315 µm and each reservoir had a volume ∼25 nl (Santini et al., Citation1999, Citation2000b). However, the size of these devices are not restricted so that it could be much larger or smaller (<2 mm) depending on use (Santini et al., Citation2000a). These microchip devices are now being commercially developed by the company by a Bedford, MA, called MicroCHIPS Technology (Webb, Citation2004; Gardner, Citation2006; Sharma et al., Citation2006; Staples et al., Citation2006). This company took its first step toward proving such a device is possible in March 2006, when results of the first animal test of an implantable drug-delivery system were published (Betancourt & Brannon-Peppas, Citation2006; Jonietz, Citation2006).
Kim et al. (Citation2007) have done the first investigational application with these microchips in chemotherapy. They used a resorbable, multi-reservoir polymer microchip drug delivery system which has been tested against a tumor model. Furthermore, they loaded the microchip reservoirs with 1,3-bis (2-chloroethyl)-1-nitrosourea (BCNU) which has serious side effects when delivered systemically at the level required for efficacious therapy in the brain. Local drug delivery would therefore be a welcome alternative, to maintain efficacy while decreasing or even elimination serious side effects (Kim et al., Citation2007; Daniel et al., Citation2009).
Theory of operation
Many researchers have been reported that, implantable drug delivery systems can be divided into two classes (; Grayson et al., Citation2003; Staples et al., Citation2006; Nisar et al., Citation2008; Receveur et al., Citation2007; Daniel et al., Citation2009; Sakamoto et al., Citation2010; Stevenson et al., Citation2012)
Active device or solid-state silicon microchip – controlled drug releasing system after implantation using mechanical, electrical, magnetic, laser or other means.
Passive device or resorbable polymeric microchip – pre-determined drug releasing system by the materials, fabrication methods or drug formulation and cannot be controlled after implantation.
Figure 3. Active and Passive time released devices. (A) Active microchips formed by substrate where release systems contain molecules for delivery, reservoirs caps serve as anode and finally the cathodes having the conductive materials. (B) Passive microchips are also made by substrate like active microchips; contain reservoirs etched into substrate and permeable or degradable reservoir caps (Cima et al., 2000).
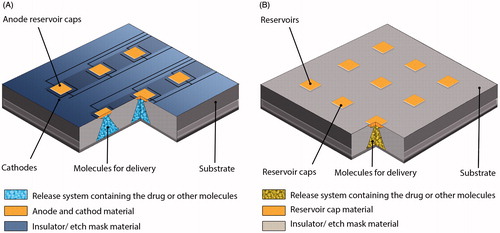
The active silicon-based microchip membranes are thin layers of gold. When a drug needs to be released from a reservoir in the active device, a voltage is applied, which causes electrochemical dissolution of the gold anode membrane. Active systems include mechanical pumping, electrolysis and other actuation methods (Mansoor et al., Citation2009; Choonara et al., Citation2010; Eljarrat-Binstock et al., Citation2010). In contrast, the passive polymeric device contains bio-degradable polymer membranes. Passive systems utilize diffusion, osmotic potential or concentration gradients as their driving forces. The composition, molecular weight and thickness of the membrane determine when the drug depot is released from reservoirs in the passive polymeric device. Both active and passive microchips have demonstrated in vitro and in vivo pulsatile release of multiple compounds (Daniel et al., Citation2009).
Design and components of controlled release microchips
Microtechnology is utilized to tailor the size, shape, reservoir number, reservoir volume, unidirectional openings and surface characteristics of the drug delivery systems in conjunction with appropriate surface chemistry is potentially influential in the area of controlled release. Each microchip device for drug delivery consists of a substrate, reservoir, reservoir cap, and a release system containing drug molecules (Santini et al., Citation1998, Citation2005).
The substrate
The etched or machined reservoirs containing substrates serve as the support for the microchip (Cima et al., 2000). Any substrate material, which can serve as a support such as ceramics, semiconductors and degradable (e.g. polyethylene glycol) and non-degradable polymers (e.g. silicon) (Uhrich et al., 1999; Santini et al., Citation2005, Citation2011), polycrystalline and amorphous silicon, glass or plastic materials (Voldman et al., Citation1999; Armani & Liu, Citation2000), is appropriate for etching or machining, and is also the molecules to be delivered cannot penetrate into the surrounding fluids, e.g. water, blood etc. (Santini et al., Citation1999, Citation2005). The molecules to be delivered may be inserted into the reservoirs or they may be encapsulated within or by a release system where the molecules can be homogenously or heterogeneously distributed. Assortment of the release system depends on the desired release rate system can be used for delivery of molecules (Santini et al., Citation1998).
Therapeutic agents to be released
In 2005, Santini Jr. and his colleagues had reported that microchips can contain multiple drugs in a variety of forms, including solid, liquid or gel (Orive et al., Citation2004; Santini et al., Citation2005). The drugs which to released can be a single drug or multiple drugs (Santini et al., Citation2000b, , Citation2011). There are some drugs listed in which can be contained in microchip. However, a number of chemicals can be deposited and released from the microchip with a different chemical or combination of chemicals. Microfluidic devices for example pumps have the limitations to delivering liquids. But the controlled-release microchip can release materials on demand because it consists of a reservoir covered by a thin membrane of materials that can be dissolved as pulsatile manner. Furthermore, the state of the drugs or chemicals inside the reservoirs, polymer matrices, excipients or any other substances have very minute or no effect on the electrochemical behavior of the membrane. For this reason, controlled release microchips can store and release different types of chemicals (Santini et al., Citation2005).
Table 1. Name of therapeutic agents that can be delivered by microchip drug delivery system.
Reservoir caps
Reservoir caps control the time at which molecules are released from the reservoirs. The reservoir caps serves as anode in active devices and consists of a thin film of conductive material such as copper, gold (Santini et al., Citation1998, Citation1999; Tao & Desai, Citation2003), silver, zinc (Santini et al., Citation2005), platinum or titanium (Grayson et al., Citation2004a), and some polymers (Santini et al., Citation1998). These materials are deposited over the reservoir according to the desired geometric pattern (; Santini et al., Citation1998, Citation2005). Depending on the utilization of the device and electric potential control manner, fabrication of cathodes with their size and placement is also applied (; Cima et al., 2000).
Figure 4. Fabrication process of active microchips. Fabrication starts with the depositing and photolithographically patterning of insulating or dielectric materials on the substrate. This serves as etch mask during reservoir etching. The insulating materials are deposited on both side of silicon wafer by different kinds of deposition techniques as described by Cima et al. (2000). Different types of etching processes are applied to pattern the reservoirs into the insulator film on one side of the wafer. Then a photoresist is patterned as electrodes on the surface of substrate and the on the reservoir opening it acts as anode. The insulating or dielectric materials, over the entire surface of the device act as cathode. After that, the thin membranes of insulating materials are removed before filling the reservoirs and injection/inkjet printing or spin coating fills reservoirs. The reservoirs are then sealed by wafer bonding or by other processes.
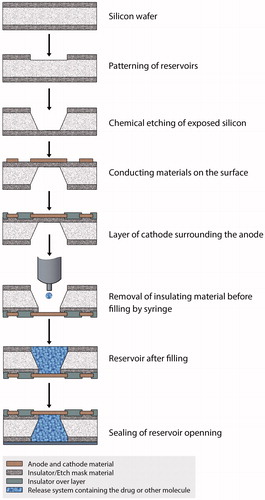
Figure 5. Fabrication process of passive microchips. The reservoir etching process of passive microchips are like active microchips. But the reservoir cap material is injected by micro syringe (I) or by inkjet printer (II). The cap formation is completed if it is done by inkjet printing method (II). But in case of spin coating (I), the thickness of the film is obtained by the etching. Then the molecules to be released are inserted into the reservoirs (III and IV). Unlike active microchips, the thin insulating or dielectric membranes are removed after filling the reservoirs. And, finally, the reservoirs are sealed with appropriate method described by Cima et al. (2000).
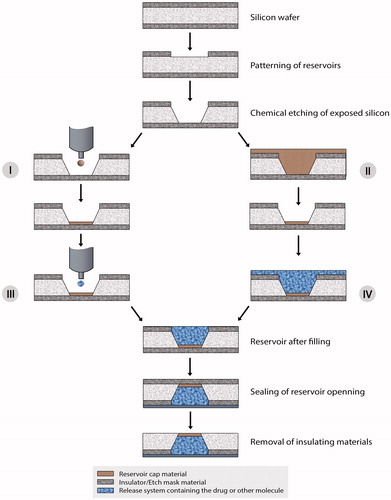
Unlike active devices, in passive devices, the reservoir caps are formed from a material such as polymers that go for degradation or dissolves over time, or the membrane either degrade or dissolve but is permeable to the molecules to be released (; Santini et al., Citation1999, Citation2005).
Fabrication of microchip reservoirs and reservoir caps
To control the both of rate and time release of multiple chemical substances, fabrication methods are applied for microchips and also allow for the release of a number of molecules in a continuous or pulsatile method (Cima et al., 2000). The device is microfabricated by means of a sequential technique using silicon wafer (Lang, Citation1996; Santini et al., Citation1999) and microelectronic processing techniques including ultraviolet photolithography (Santini et al., Citation1998; Hilt & Peppas, Citation2005), chemical vapor deposition (Daniel et al, Citation2009), electron beam evaporation (Santini et al., Citation1998) and reactive ion etching (Santini et al., 2000b; Hilt & Peppas, Citation2005), bonding (Santini et al., Citation2011) using highly biocompatible materials (Ge et al., Citation2009). Each device contained reservoirs that extended completely through the wafer. The reservoirs can be square pyramidal in shape (Santini et al., Citation1999). In case of passive timed release reservoir caps, silicon nitride is usually used as insulating material and the inkjet filled with solution or suspension of cap material is used to print the cap. Etching of the exposed cap material in this reservoir is stopped after the desired cap thickness is obtained. However, it is possible to fabricate a passive timed release device without reservoir caps (Santini et al., Citation1998, Citation2011).
Moreover, the thin membrane of insulating or dielectric material to protect the electrodes from corrosion, degradation, or dissolution in all areas where electrode film removal is not necessary for release on active timed release reservoir caps. This membrane is used as an anode on the surface of the substrate having the reservoirs covered for microfabrication (Santini et al., Citation1998). Usually there are two methods for removing the membrane, an ion beam or reactive ion plasma and chemical etching. The molecules of release systems for delivery are inserted in to the reservoirs by injection, inkjet printing or spin coating (Santini et al., Citation1998; Tao & Desai, Citation2003).
Insulating or dielectric material such as aluminium oxide or silicon nitride are usually used to prepare the packages. They are used to permit the easy inter connection of components to power source and to each other. Polyethylene glycol or polytetrafluoroethylene-like materials are used for packaging the entire including all components (Santini et al., Citation2011). The fabrication process of active and passive devices is shown in and , respectively, as described by Santini et al. (Santini et al., 1998, 2005; Cima et al., 2000).
Control circuitry and Source of power
In the study performed by Santini et al. has been reported that, the control circuitry consists of a timer, a demultiplexer, a microprocessor and an input source, e.g. memory source, single receiver or biosensor () could be integrated in implantable microchips. Small size power source capacity, integrating ability into the control circuitry and to be recharged and the length of time before recharging are essential for selecting a power source. As a source of power, lithium-based or rechargeable microbatteries can be used (Satini et al., 2000c, 2005).
Figure 6. Schematic views of control circuitry of a typical microchip. A timer, a microprocessor, a de-multiplexer and an input source (e.g. memory source, a signal receiver or a biosensor) are used to arrange the control circuitry for microchips (Santini et al., Citation2000a).
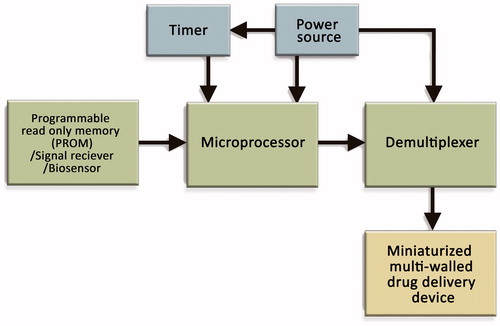
The researchers have claimed that electrodes can be maintained by two primary control methods within a specific range. One is potentiostatic control where the potential is constant during reservoir activation and another one is galvanostatic control where the current is constant during reservoir activation. This electric potential can be utilized to change the local pH level near the anode reservoir cap to solubilize the insoluble ions or oxidation products and the reservoir caps are dissolved to expose the release system to the surrounding fluids (Santini et al., 1998 & 2011; Cima et al., 2000).
Challenges and potential prospective of microchips for drug delivery
The controlled released microchips, which are the microreservior devices, are highly suitable in case of chronotherapy because of their ability to attain repeated pulsatile release of drug depots. Moreover, comparing with micropumps, these microchip devices contain no moving parts and are able to deliver drugs in the solid, liquid and gel state. The microchip design also protects the drug depot from the outside environment before release. For this reason, a small amount of drug might be required to see a therapeutic effect or increase the time period over which delivery of active drug can occur (Daniel et al., Citation2009). Furthermore, this kind of reservoir-based implants can be applied in both systemic and localized drug delivery applications. These micro implants offer a great deal of promise in addressing current unmet medical needs and have resulted in some of the most pioneering and elegant drug delivery concepts (Stevenson et al., Citation2012). Microchip technology has the potential to change the way clinicians practice drug delivery (Staples, Citation2010). These devices offer a range of advantages. Challenges and potential prospective of microchip for drug delivery are discussed in .
Table 2. Challenges and potential prospective of implantable microchip for drug delivery.
Biocompatibility of implantable microchip
MEMS devices have unique biocompatibility issues. The biocompatibility of a fabricate drug delivery microreservoir device depends only on those materials in contact with tissue (Grayson et al., Citation2004a–c; Graham-Rowe, Citation2012) such as, silicon (used as a substrate and structural material), gold (used for electrodes) and silicon dioxide, silicon nitride etc. (used as dielectrics) (Shawgo et al., Citation2002; Chung et al., 2008). The implantable microchip devices directly interact with the body so the biocompatibility of silicon and other materials of fabrication have become much more important (Grayson et al., Citation2004a–c). Silicon is exposed to body fluids inside the wells which causes bio-incompatibility, but can be silanated by a number of simple surface passivation processes. Therefore, the surface modifications can be applied to increase biocompatibility and decrease biofouling (Erickson et al., 2008; Shawgo et al., Citation2002).
Current researches on implantable microchips for drug delivery
Some magnificent effort is being done to modify the implantable microchips for better understanding of the aspects affecting the microfabrication of the device and other factors. In order to improve the implantable controlled released microchips, Petersen (Citation1982) has explained that, the mechanical properties of silicon and the purpose for the development a wide range of low-cost, batch-fabricated, high-performance sensors and transducers that could be easily interfaced with advanced microelectronics. Nevertheless, favorable electrical and mechanical properties have made the silicon as the initial material of choice in these microfabricated devices. Besides this, other materials, such as ceramics and polymers are also applicable for the fabrication of microdevices because of their desirable properties, e.g. biocompatibility and price (Peppas & Byrne, Citation2003; Peppas, Citation2004).
A current improvement was made in this field by using integrating active components including battery clocks, reference electrodes, biosensors etc. (Tao & Desai, Citation2003). Alternative materials for microfabrication processing schemes for microchips will be modified to optimize mass production (decreases cost) and to produce defect-free membranes (prevents drug leakage) (Santini et al., Citation2000b; Staples, Citation2010). In 2003, MicroCHIPS developed reservoir arrays where the metal membranes coating the reservoirs were opened by electrothermal mechanism that behaves similarly to an electrical fuse (Maloney et al., Citation2005) instead of electrochemically (Smith et al., 2007; Stevenson et al., Citation2012). The structure of this device is similar to the first controlled release microchip demonstrated experimentally by Santini and his colleagues in Nature (Maloney et al., Citation2005; Smith et al., 2007).
Before that, Grayson and co-workers from MIT (Brazil, Citation2003) have derived a biodegradable polymeric microreservoir device on the solidstate silicon microchip for controlled-release drug delivery relies on the degradation of thin poly (lactic-co-glycolic acid; Vogelhuber et al., Citation2001; Grayson et al., Citation2004b, Lesniak & Brem, Citation2004; Betancourt & Brannon-Peppas, Citation2006; Staples et al., Citation2006) which is a 50:50 ratio of lactic acid to glycolic acid (Grayson et al., Citation2003). In another study by Santini et al., (2005), a flexible microchip device has been patented by to deliver the drug into the eye that is made up of an array of several small microchip device elements that are attached to a flexible supporting layer (Santini et al., Citation2005). On the other hand, Sheppard Jr. and his colleagues have been developed a microchip reservoir device using wireless transmission of power and data and it has been patented in 2007. By this technology an orally administered drug delivery microchips can be activated at a specific time or at a specific location in the gastrointestinal tract using pre-programmed microprocessors, remote control systems (e.g. wireless systems or biosensors; ). The microchip could release drug after receiving power from the RF transmitter (Sheppard et al., Citation2007).
Figure 7. An orally administered drug delivery microchip using wireless transmission of power and data can be activated at a specific time or at a specific location in the gastrointestinal tract (Sheppard et al., Citation2007).
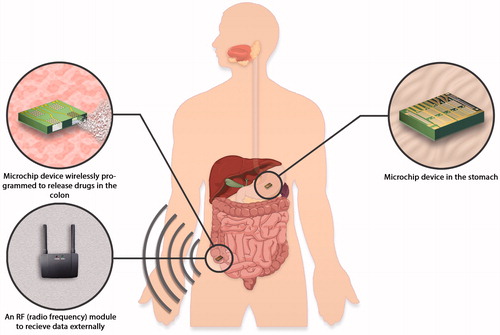
The development of microchips for controlled release drug delivery steps forward when researchers have developed a disk-shaped biodegradable polymeric chip with the combination of self-regulated release reservoir designed by microfabrication technique to attain multipulse drug release (Liu et al., Citation2007). Furthermore, the structure of microchip presented by Santini, has been modified by Chung and his colleagues (Chung et al., 2008). Their depicted device contain the silicon layer containing the drug well(s) and the upper set of electrodes, while the polydimethylsiloxane (PDMS; Staples, Citation2010) layer served to seal the well and hold the lower electrode (Chung et al., 2008). In a recent mechanistic study, it was suggested that polypyrrol (PPy) is suitable and attractive candidates for accommodating drugs for polypyrrole-based controlled release microchip due to unique redox properties (Ge et al., Citation2009; Staples, Citation2010). This microchip has been developed by electrochemical deposition of drug-doped polypyrrole films on the gold microelectrode arrays (Ge et al., Citation2009). Compared to the other reservoir-based microchip, the preparation procedure of this PPy-based microchip is simple and convenient (Ge et al., Citation2009). More recently, Lee and his co-workers have been described a drug delivery microchip for sustained drug release on diffusion through microchannels by which a single microchip can release drug continuously (Lee et al., 2007).
Development of wireless controlled microchip for anti-osteoporosis
First human trial of an implanted microchip was implanted subcutaneously into eight Danish osteoporotic postmenopausal women (Cheung, Citation2012; Farra et al., Citation2012; Graham-Rowe, Citation2012; Watson, Citation2012) that releases a drug for osteoporosis on command from wireless signals has been reported by Robert Farra, the president of MicroCHIPS Inc. (Cheung, Citation2012; Watson, Citation2012), that was spun out of the Massachusetts Institute of Technology in Cambridge (). A paper describing the results was also published online February 16, 2012 in Science Translational Medicine (Cheung, Citation2012; Gershon, Citation2012). Human parathyroid hormone fragment (1–34) [hPTH(1–34)] (Liu et al., Citation2007) is used to treat osteoporosis (Jonietz, Citation2006) which requires burdensome daily injections (Gershon, Citation2012). The goal of such a device is to improve current chronic delivery methods, which often involve needles and repeated injections, for example, insulin for patients with diabetes (Watson, Citation2012). The microchip created by Farra and colleagues (Gershon, Citation2012; Graham-Rowe, Citation2012; Watson, Citation2012) holds 20 pinprick-sized programmed reservoirs, each containing a 600-nl dose of the drug and release 20 doses once daily.
Figure 8. An implantable microchip for releasing parathyroid hormone fragment (1–34) [hPTH(1–34)] (Farra et al., Citation2012).
![Figure 8. An implantable microchip for releasing parathyroid hormone fragment (1–34) [hPTH(1–34)] (Farra et al., Citation2012).](/cms/asset/d9a6de75-faf0-4a86-b0a2-e307e6e1fcdc/idrd_a_903579_f0008_c.jpg)
Researchers are claiming that this device will save patients from the painful ritual of daily injections (Cheung, Citation2012). Within 30 min, the device can be implanted under local anesthetic in a doctor's office were well tolerated, with no toxic or adverse effects from the implant. They have successfully showed that this microchip-based drug delivery device provided pharmacokinetic profiles similar to subcutaneous injections, even with the fibrous tissue capsule those obtained by multiple injections (Gershon, Citation2012; Watson, Citation2012). However, in this study, the device failed in one patient (an eighth patient, not included in their analysis; Cheung, Citation2012; Watson, Citation2012). But still it needs several years to bring this technology to approval by the U.S. Food and Drug Administration (FDA; Watson, Citation2012). In most recently, MicroCHIPS is planning to design such a device which will contain the reservoirs for a year's supply of doses and within 2014, they will have a version for regulatory testing (Graham-Rowe, Citation2012).
Conclusion
A stable upgrading has been gained from the first implantable microchip to the present time. There is no doubt that in near future implantable microchip will replace the conventional drug delivery systems which are using in these days. As a promising approach, a lot of improvement is required for these implantable microchip devices including biocompatibility, accurate size and shape, more patient compliance for administration and improved rate of drug delivery in surrounding fluid. The challenges for the microchips in coming days are the possibility of the scaling-up processes and the improvement of the present implantable microchips as multifunctional microchips which will be able to perform the different types of biological and therapeutic necessities in the intervening time.
Declaration of interest
The authors report no declarations of interest.
References
- Armani DK, Liu C. (2000). Microfabrication technology for polycaprolactone, a biodegradable polymer. Micro Electro Mech Sys 10:80–4
- Banerjee PS, Robinson JR. (1991). Novel drug delivery systems: an overview of their impact on clinical pharmacokinetic studies. Clin Pharma 20:1–14
- Betancourt T, Brannon-Peppas L. (2006). Micro- and nanofabrication methods in nano technological medical and pharmaceutical devices. Int J Nanomed 1:483–95
- Bramstedt AK. (2005). When microchip implants do more than drug delivery: Blending, blurring, and bundling of protected health information and patient monitoring. Tech Health Care 13:193–8
- Brazil M. (2003). Drug delivery: biodegradable multi-drug dispenser. Nat Rev Drug Discov 2:946
- Breimer DD. (1999). Future challenges for drug delivery. J Control Release 62:3–6
- Bustillo JM, Howe RT, Muller RS. (1998). Surface micromachining for microelectromechanical systems. Proc IEEE 86:1552–74
- Cima MJ, Langer RS, Santini JT Jr. Massachusetts Institute of Technology, assignee. (2000). Fabrication of microchip drug delivery device. US Patent US6123861, 26 Sept 2000
- Cheung R. (2012). AAAS Meeting: wireless drug delivery achieved: implanted microchip releases medication on command. Sci News 181:8
- Chien YW, Lin S. (2006). Drug delivery: controlled release (Chapter 73). In: James S, ed. Encyclopedia of pharmaceutical technology. Florida, USA: CRC Press, Taylor & Francis Group,1082–102
- Choonara YE, Pillay V, Danckwerts MP, et al. (2010). A review of implantable intravitreal drug delivery technologies for the treatment of posterior segment eye diseases. J Pharm Sci 99:2219–39
- Chung AJ, Kim D, Erickson D. (2008). Electrokinetic microfluidic devices for rapid, low power drug delivery in autonomous microsystems. Lab on a Chip 8:330–8
- Daniel K, Duc HL, Cima M, Langer R. (2009). Controlled release microchips (Chapter 9). In: Youan BC, ed. Chronopharmaceutics: science and technology for biological rhythm-guided therapy and prevention of diseases. New Jersey, USA: John Wiley & Sons Inc. Publication, 187–212
- Dario P, Carrozza MC, Benvenuto A, et al. (2000). Micro-systems in biomedical Applications. Microeng J Micromech 10:235–44
- Drews J. (2000). Drug discovery: a historical perspective. Science 287:1960–4
- Eljarrat-Binstock E, Pe'er J, Domb AJ. (2010). New techniques for drug delivery to the posterior eye segment. Pharm Res 27:530–43
- Farra R, Sheppard NF, McCabe L, et al. (2012). First-in-human testing of a wirelessly controlled drug delivery microchip. Sci Transl Med 4:1–10
- Gardner P. (2006). Microfabricated nanochannel implantable drug delivery devices: trends, limitations and possibilities. Exp Opin Drug Deliv 3:479–87
- Ge D, Tian X, Qi R, et al. (2009). A polypyrrole-based microchip for controlled drug release. Electrochim Acta 55:271–5
- Gershon D. (2012). Drug delivery goes remote. Nat Med 18:506
- Goldman P. (1982). Rate-controlled drug delivery. N Engl J Med 307:286–90
- Gourley PL. (1996). Semiconductor microlasers: a new approach to cell-structure analysis. Nat Med 2:942–4
- Graham-Rowe D. (2012). Wireless drug implant takes the trouble out of treatment. Nature News & Comment. doi:10.1038/nature.2012.10045. Available at: http://www.nature.com/news/wireless-drug-implant-takes-the-trouble-out-of-treatment-1.10045
- Grayson ACR, Choi IS, Tyler BM, et al. (2003). Multi-pulse drug delivery from a resorbable polymeric microchip device. Nat Mater 2:767–72
- Grayson ACR, Chima JM, Langer R, et al. (2004a). A BioMEMS review: MEMS technology for physiologically integrated devices. IEEE 92:6–21
- Grayson ACR, Cima MJ, Langer R. (2004b). Molecular release from a polymeric microreservoir device: influence of chemistry, polymer swelling, and loading on device performance. J Biomed Mat Res Part A 69A:502–12
- Grayson ACR, Scheidt Shawgo R, Li Y, Cima MJ. (2004c). Electronic MEMS for triggered delivery. Adv Drug Deliv Rev 56:173–84
- Hilt JZ, Peppas NA. (2005). Microfabricated drug delivery devices. Int J Pharma 306:15–23
- Jain D, Raturi R, Jain V, et al. (2011). Recent technologies in pulsatile drug delivery systems. Biomatter 1:57–65
- Jonietz E. (2006). Implantable medication: programmable drug chips pass longevity milestone. MIT Tech Rev May/June:23
- Kikuchi A, Okano T. (2002). Pulsatile drug release control using hydrogels. Adv Drug Deliv Rev 54:53–77
- Kim GY, Chima MJ, Tupper MM, et al. (2007). Resorbable polymer microchips releasing BCNU inhibit tumor growth in the rat 9L flank model. J Control Release 123:172–8
- Kovacs GTA, Knapp TR, LipoMatriz, Inc., assignee. (1998). Implantable biosensing transponder. US Patent US5833603, 10 Nov 1998
- Kuo Y, Violante MR, Pharma Nova Inc., assignee. (2012). Implantable drug delivery device. US Patent US0059349 A1, 8 Mar 2012
- Lang W. (1996). Silicon microstructuring technology. Mat Sci Eng R Rep 17:1–55
- Langer R. (1990). New methods of drug delivery. Science 249:1529–33
- Langer R, Peppas NA. (2003). Advances in biomaterials, drug delivery, and bionanotechnology. AIChE J 49:2990–3006
- LaVan DA, Lynn DM, Langer R. (2002). Moving smaller in drug discovery and delivery. Nat Rev Drug Discov 1:77–84
- Lee SH, Park M, Park CG, et al. (2012). Microchip for sustained drug delivery by diffusion through microchannels. AAPS PharmSciTech 13:211–17
- Lesniak SM, Brem H. (2004). Targeted therapy for brain tumours. Nat Rev Drug Discov 3:499–508
- Li Y, Shawgo RS, Tyler B, et al. (2004). In vivo release from a drug delivery MEMS device. J Control Release 100:211–19
- Liu X, Pettway GJ, McCauley LK, et al. (2007). Pulsatile release of parathyroid hormone from an implantable delivery system. Biomaterials 28:4124–31
- Maloney MJ. (2003). Proceedings of IMECE'03. 15–21 Nov 2003 ASME International Mechanical Engineering Congress, Washington, DC
- Maloney JM, Uhland SA, Polito BF, et al. (2005). Electrothermally activated microchips for implantable drug delivery and biosensing. J Control Release 109:244–55
- Mansoor S, Kuppermann BD, Kenney MC. (2009). Intraocular sustained release delivery systems for triamcinolone acetonide. Pharm Res 26:770–84
- Medlicott NJ, Tucker IG. (1999). Pulsatile release from subcutaneous implants. Adv Drug Deliv Rev 38:139–49
- Nisar A, Afzulpurkar N, Mahaisavariya B, et al. (2008). MEMS-based micropumps in drug delivery and biomedical applications. Sensor Actuat B Chem 130:917–42
- Orive G, Gascón AR, Hernández RM, et al. (2004). Techniques: new approaches to the delivery of biopharmaceuticals. Trends Pharma Sci 25:382–7
- Peppas NA. (2004). Intelligent therapeutics: biomimetic systems and nanotechnology in drug delivery. Adv Drug Deliv Rev 56:1529–31
- Peppas NA, Byrne ME. (2003). New biomaterials for intelligent biosensing, recognitive drug delivery and therapeutics. Bull Gattefosśe 96:23–35
- Petersen KE. (1982). Silicon as a mechanical material. Proc IEEE 70:420–57
- Polla DL, Erdman AG, Robbins WP, et al. (2000). Microdevices in medicine. Ann Rev Biomed Eng 2:551–76
- Putney SD, Burke PA. (1998). Improving protein therapeutics with sustained-release formulations. Nat Biotech 16:153–7
- Razzacki SZ, Thwar PK, Yang M, et al. (2004). Integrated microsystems for controlled drug delivery. Adv Drug Deliv Rev 56:185–98
- Receveur RAM, Linemans FW, de Rooij NF. (2007). Microsystem technologies for implantable applications. J Micromech Microeng 17:R50–80
- Sakamoto JH, Van de Ven AL, Godin B, et al. (2010). Enabling individualized therapy through nanotechnology. Pharmacol Res 62:57–89
- Sant S, Tao SL, Fisher O, et al. (2012). Microfabrication technologies for oral drug delivery. Adv Drug Deliv Rev 64:496–507
- Santini JT Jr, Cima MJ, Langer RS, Massachusetts Institute of Technology, assignee. (1998). Microchip drug delivery devices. US Patent US5797898 25 Aug 1998
- Santini JT Jr, Cima MJ, Langer RS. (1999). A controlled-release microchip. Nature 397:335–8
- Santini JT Jr, Chima MJ, Langer RS. (2000a). Microchip as controlled drug-delivery device. Angew Chem Int Ed 39:2396–407
- Santini JT Jr, Richards AC, Scheidt RA, et al. (2000b). Microchip technology in drug delivery. Ann Med 32:377–9
- Santini JT Jr, Cima MJ, Langer RS, et al., MicroCHIPS Inc., assignee. (2005). Flexible microchip devices for ophthalmic and other applications. US Patent US6976982 B2, 20 Dec 2005
- Santini JT Jr, Cima MJ, Langer RS. Massachusetts Institute of Technology, assignee. (2011). Method for operating microchip reservoir devices. US Patent US7901397B2, 8 Mar 2011
- Schulz M. (1999). The end of the road for silicon? Nature 399:729–30
- Shawgo RS, Richards Grayson AC, Li Y. (2002). BioMEMS for drug delivery. Mater Sci 6:329–34
- Sharma S, Nijdam AJ, Sinha PM, et al. (2006). Controlled-release microchips. Exp Opin Drug Deliv 3:379–94
- Sheppard Jr NF Santini Jr JT, Herman SJ, et al. MicroCHIPS Inc., assignee. (2007). Microchip Reservoir devices using wireless transmission of power and data. US Patent US7226442 B2, 5 June 2007
- Siegel RA, Rathbone MJ. (2012).Overview of controlled release mechanisms (Chapter 2). In: Siepmann J, Siegel RA, Rathbone MJ, eds. Advances in delivery science and technology: fundamentals and applications of controlled release drug delivery. New York, USA: Springer, 19–43
- Smith S, Tang TB, Terry JG, et al. (2007). Development of a miniaturised drug delivery system with wireless power transfer and communication. IET Nanobiotech 1:80–6
- Staples M. (2010). Microchips and controlled-release drug reservoirs. WIREs Nanomed Nanobiotech 2:400–17
- Staples M, Daniel K, Cima MJ, Langer R (2006). Application of micro and nano-electromechanical devices to drug delivery. Pharma Res 23:847–63
- Stevenson CL, Santini JT Jr, Langer R. (2012). Reservoir-based drug delivery systems utilizing microtechnology. Adv Drug Deliv Rev 64:1590–602
- Tao SL, Desai TA. (2003). Microfabricated drug delivery systems: from particles to pores. Adv Drug Deliv Rev 55:315–28
- Uhrich KE, Cannizzaro SM, Langer RS. (1999). Polymeric systems for controlled drug release. Chem Rev 99:3181–98
- Urquhart J, Fara JW, Willis KL. (1984). Rate-controlled delivery systems in drug and hormone research. Ann Rev Pharmacol Toxicol 24:199–236
- Vogelhuber W, Rotunno P, Magni E, et al. (2001). Programmable biodegradable implants. J Control Release 73:75–88
- Voldman J, Gray ML, Schmidt MA. (1999). Microfabrication in biology and medicine. Annu Rev Biomed Eng 1:401–25
- Watson J. (2012). Reengineering device translation timelines. Sci Trans Med 4:1–2
- Webb EC. (2004). Chip shots: implanted semiconductors will allow drugs to be delivered exactly when and where they are needed. IEEE Spectrum 41:49–53
- Wolf S, Tauber RN. (1986). Silicon: single-crystal growth & wafer preparation (Chapter 1). In: Wolf S, Tauber RN, eds. Silicon processing for the VLSI Era, Vol. 1: Process technology. California: Lattice Press, 22–31
- Ziaie B, Baldi A, Lei M, et al. (2004). Hard and soft micromachining for BioMEMS: review of techniques and examples of applications in microfluidics and drug delivery. Adv Drug Deliv Rev 56:145–72