Abstract
In cases of malignant brain tumors, infiltrating tumor cells that exist at the tumor-surrounding brain tissue always escape from cytoreductive surgery and, protected by blood-brain barrier (BBB), survive the adjuvant chemoradiotherapy, eventually leading to tumor recurrence. Local interstitial delivery of chemotherapeutic agents is a promising strategy to target these cells. During our effort to develop effective drug delivery methods by intra-tumoral infusion of chemotherapeutic agents, we found consistent pattern of leakage from the tumor. Here we describe our findings and propose promising strategy to cover the brain tissue surrounding the tumor with therapeutic agents by means of convection-enhanced delivery. First, the intracranial tumor isograft model was used to define patterns of leakage from tumor mass after intra-tumoral infusion of the chemotherapeutic agents. Liposomal doxorubicin, although first distributed inside the tumor, distributed diffusely into the surrounding normal brain once the leakage happen. Trypan blue dye was used to evaluate the distribution pattern of peri-tumoral infusions. When infused intra- or peri-tumorally, infusates distributed robustly into the tumor border. Subsequently, volume of distributions with different infusion scheduling; including intra-tumoral infusion, peri-tumoral infusion after tumor resection, peri-tumoral infusion without tumor removal with or without systemic infusion of steroids, were compared with Evans-blue dye. Peri-tumoral infusion without tumor removal resulted in maximum volume of distribution. Prior use of steroids further increased the volume of distribution. Local interstitial drug delivery targeting tumor surrounding brain tissue before tumor removal should be more effective when targeting the invading cells.
Introduction
Although the incidence of primary brain tumors is increasing, the treatment options and prognosis for patients have seen little improvement during last decade (Jemal A et al., Citation2003). Treatment for high-grade gliomas such as glioblastoma frequently consists of surgical resection followed by radiochemotherapy (Stupp et al., Citation2005). Although, advent of temozolomide improved the efficacy of systemic chemotherapy, it still provides limited improvement in survival (Brandes, Citation2003; DeAngelis, Citation2003). The major problem is the invasion of tumor cells into surrounding normal brain. Even at initial presentation, infiltrating tumor cells extend at least 2 cm away from the radiographic enhancing mass (Watanabe M et al., Citation1992). Systemic chemotherapeutic agents are generally ineffective because of limited drug delivery, especially in the normal brain surrounding the tumor that contains the invading tumor cells where the blood-brain barrier (BBB) is still intact. Therefore, new strategies targeting infiltrating tumor cells are clearly needed.
Local drug delivery, a leading candidate, bypasses the BBB, reduces systemic drug exposure to minimize the side-effects of chemotherapy, and achieves prolonged elevations of intra-cerebral chemotherapeutic agents relative to those obtainable by systemic administration (Walter, et al., Citation1995; Groothuis, Citation2000). An important advance in local drug delivery is the development of convection-enhanced delivery (CED). This technique uses bulk flow to directly deliver small or large molecules to targeted sites in clinically significant volumes of tissue, and provides improved volumes of distribution (Vd) compared with simple diffusion techniques (Bobo et al., Citation1994). Many investigators are now applying this technique to clinical treatment of brain tumors. Among several studies, a study of IL13-PE38QQR, a protein composed of IL-13 combined with a truncated form of Pseudomonas exotoxin (PE38QQR), is the most advanced (Kunwar et al., Citation2007; Vogelbaum et al., Citation2007). The Phase III Randomized Evaluation of Convection-Enhanced Delivery of IL13-PE38QQR with survival Endpoint (PRECISE) trial evaluated efficacy of IL13-PE38QQR infused peri-tumorally after resection. Although the efficacy of interstitial infusion was observed, it failed to achieve statistically significant study endpoints. Poor drug distribution was suggested as a possible explanation for this unfavorable result (Sampson et al., Citation2010).
During our effort to develop effective drug delivery methods by intra-tumoral infusion of chemotherapeutic agents (Saito et al., Citation2004; Sugiyama et al., 2007; Kikuchi et al., Citation2008), we found a consistent pattern of leakage of drug from the tumor. Here we describe our findings and propose a promising strategy to cover the brain tissue surrounding the tumor with therapeutic agents via convection-enhanced delivery.
Materials and methods
PEGylated liposomal doxorubicin, Trypan blue, and Evans blue
PEGylated liposomal doxorubicin (PLD: Doxil®, doxorubicin hydrochloride, ALZA Pharmaceuticals, Mountain View, CA) was obtained commercially. The PLD solution contained 2 mg/ml of doxorubicin. Trypan blue was obtained from Sigma-Aldrich (Tokyo, Japan), and was diluted with PBS to a concentration of 4 mM prior to infusion. Evans blue was obtained from Wako Co. Ltd. (Osaka, Japan), and was diluted with 0.9% saline to a concentration of 0.2% v/v.
Tumor cell line, animals, and intracranial isograft technique
An established rodent glioma cell line, F98, was obtained commercially from the American Type Culture collection (Rockville, MD). The 9L glioma cell line was a generous gift from Dr. Souichiro Shibui, Department of Neurosurgery, National Cancer Center (Tokyo, Japan). Cells were maintained as monolayers in a complete medium consisting of Eagle's minimal essential medium supplemented with 10% fetal calf serum and non-essential amino acids. Cells were cultured at 37 °C in a humidified atmosphere containing 95% air and 5% CO2. Twelve-week-old male Fisher 344 rats, weighing between 190 and 210 g, were purchased from CLEA Japan Inc. (Tokyo, Japan) and were housed under aseptic conditions, which included filtered air and sterilized food, water, bedding, and cages. All protocols used in the animal studies were approved by the Institutional Animal Care and Use Committee by the Institute for Animal Experimentation, Tohoku University Graduate School of Medicine. For the intracranial isograft tumor model, cells were harvested by trypsinization, washed once with PBS and resuspended in PBS for implantation. For the 9L model, we used a cell suspension containing 5 × 105 cells/10 μl PBS, and 1 × 105 cells/10 ml PBS for the F98 model, for implantation into the rat striatal region. Under deep isoflurane anesthesia, rats were placed in a small-animal stereotactic frame (NARISHIGE CO., Tokyo, Japan). A sagittal incision was made through the skin to expose the cranium, and a burr-hole was made in the skull 0.5 mm anterior and 3 mm lateral from the bregma with a small dental drill. At a depth of 4.5 mm from the brain surface, 5 μl of cell suspension were injected; after a wait of 2 min, a further 5 μl of cells was injected at a depth of 4 mm, and after a final 2-minute wait, the needle was removed and the wound was sutured.
Convection-enhanced delivery
PLD or 4 mM Trypan blue solution at a volume of 20 μl was delivered by CED as described previously (Saito R et al., Citation2004; Kikuchi T et al., Citation2008). The needle was inserted through the same cannula tract as used for tumor implantation. At a depth of 4.5 mm from the brain surface, CED was performed with the following ascending infusion rates to achieve the 20 μl infusion: 0.2 μl/min (15 min) + 0.5 μl/min (10 min) + 0.8 μl/min (15 min). Five 9L intracranial isografts received CED infusions of 20 ml PLD (2 mg/ml doxorubicin) 7 days after tumor cell implantation. Similarly, three F98 intracranial isografts received CED infusions of 20 ml 4 mM Trypan blue solution 7 days after tumor cell implantation.
Comparison of different mode of infusion
Twenty-two rats that received 9L tumor cell implants were randomly divided into four groups: (a) the intra-tumoral CED of Evans blue (n = 8); (b) peri-tumoral CED of Evans blue after tumor removal (n = 7); (c) peri-tumoral CED of Evans blue (n = 8); (d) peri-tumoral CED of Evans blue with intraperitoneal injection of dexamethasone (n = 7). For rats in group (a), intra-tumoral infusion of 20 ml Evans blue was performed 7 days after tumor implantation. Rats in group (b) underwent removal of the tumor mass. Briefly, the burr-hole used for tumor cell implantation was enlarged in anesthetized rats with a dental drill and the tumor mass was removed with small forceps. Immediately after removal of the tumor, rats received peri-tumoral CED with four needles. Coordinates for these four needles were 1 mm anterior, posterior, medial, and lateral from the initial tract used for tumor implantation. For this peri-tumoral CED, the infusion volume was 5 μl for each needle resulting in a 20 ml infusion with four needles. The infusion rates for each needle was as follows: 0.2 μl/min (5 min) + 0.5 μl/min (3 min) + 0.8 μl/min (3.1 min). For rats in group (c), 7 days after tumor implantation peri-tumoral CED was performed with four needles, each inserted from 1 mm anterior to posterior and medial to lateral from the initial tract used for tumor implantation. Rats in group (d) were treated the same as those in group (c) but received an intraperitoneal injection of dexamethasone (3 mg/kg) 24 h before drug infusion.
Evaluation of distribution
In each experiment, rats were euthanized 1 h after CED and subjected to histological evaluation. The brains were harvested, freshly frozen in a dry-ice/isopentane bath, and cut into serial coronal sections (25 μm) with a cryostat. Because doxorubicin generates fluorescence under UV illumination, areas of distribution were visualized with fluorescence microscope and a charged-coupled device camera with a fixed aperture. For detection of Evans blue distribution, brain sections were visualized with the light microscope under blight field. Blue areas of brain sections were manually delineated and volumes of distribution were calculated. Open-source OsiriX imaging software (Pixmeo, Geneva, Switzerland) was used for this calculation.
Statistical analyses
Statistical analyses were computed with SPSS for Windows Version 16.0 software package (SPSS Inc., Chicago, IL). An unpaired Student's t-test was used to compare values from each group, and a probability level less than 0.05 was considered significant.
Results
Convection-enhanced delivery produces extensive distribution of liposomal doxorubicin within tumor tissue and tumor margins
H & E staining of a representative rat brain section at 1 mm interval shows the tumor formation (). Fluorescent detection of doxorubicin in same sections revealed the robust distribution of the PLD in the tumor mass and surrounding tumor margin (). Although PLD was widely distributed inside the tumor mass, coverage of entire tumor mass was difficult. Once leakage from tumor occurred, PLD distributed diffusely into the surrounding normal brain. These findings were consistent in all five animals.
Figure 1. Seven days after tumor implantation, convection-enhanced delivery of PEGylated liposomal doxorubicin was performed into tumor of 9L isografted intracranial tumor model. Hematoxylin and Eosin (H&E) staining of representative two rat brain sections show the tumor formation (A, C). Fluorescent detection of the doxorubicin in same sections revealed robust distribution of PLD in the tumor mass and surrounding tumor margin (B, D). Note the intra-tumoral distribution (arrow) and extra-tumoral distribution (arrowhead).
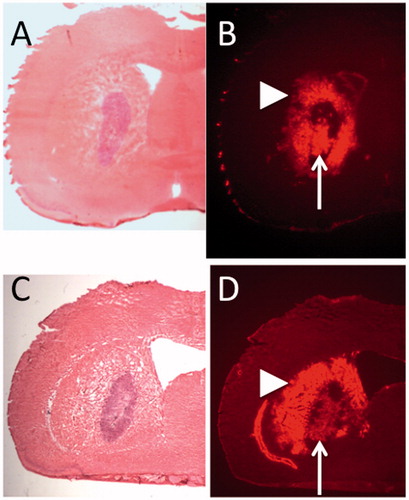
Peri-tumoral convection-enhanced delivery produces extensive distribution of infusate into brain tissue surrounding the tumor
As the PLD distributed widely into the brain surrounding the tumor after leakage from the tumor, we hypothesized that peri-tumoral CED may provide effective coverage of brain surrounding the tumor. We used the F98 glioma intracranial isograft model, and infused trypan blue dye peri-tumorally by CED. The dye effectively distributed into the tumor surrounding brain at the tumor-brain interface ().
Figure 2. In the F98 glioma intracranial isografted model, trypan blue dye was infused peri-tumorally by CED. Results from 2 representative rats are shown (upper and lower). Distribution of Trypan-blue dye (left) and H&E staining of the same section (right). The dye effectively distributed into the tumor surrounding brain at the tumor-brain interface.
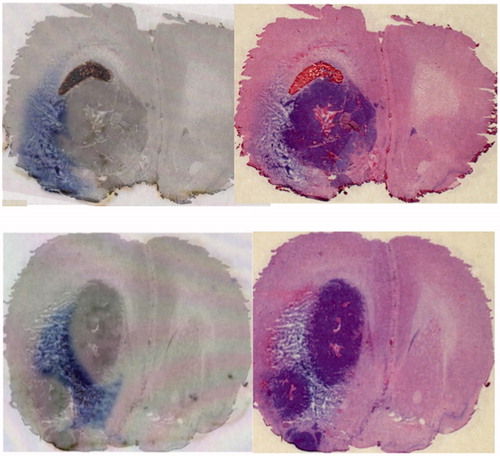
Comparison of different modes of infusion revealed the superiority of peri-tumoral infusion pre-resection
Several modes of infusions were tested for their efficacy. These included intra-tumoral infusion, peri-tumoral infusion after tumor removal, peri-tumoral infusion before tumor removal, and peri-tumoral infusion before tumor removal with prior use of steroids. Volume of distribution after one needle intra-tumoral CED was as large as 97.6 ± 3.9 mm3 (mean ± standard deviation). In comparison, the volume of distribution after peri-tumoral infusion following tumor removal was smaller (p = 0.0008), i.e. 74.5 ± 14.4 mm3. On the other hand, robust distribution of 112.7 ± 4.7 mm3 was observed with pre-resection peri-tumoral CED (p < 0.0001, compared to one needle intratumoral infusion). Moreover, the use of dexamethasone 24 h prior to CED resulted in a further enlargement of distribution of 130 ± 6.6 mm3 (p < 0.0001, compared to pre-resection peri-tumoral CED).
Discussion
One of the major issues in the use of convection-enhanced delivery to treat brain tumors is whether it is better to target the tumor itself or the brain surrounding the tumor (Kunwar S, Citation2003). Although the precise mechanism is still not clear, interstitial fluid pressure (IFP) is elevated in tumors (Boucher et al., Citation1990; Boucher & Jain, Citation1992; Navalitloha et al., Citation2006). In contrast, it is known that the IFP is close to 0 mm Hg in normal brain at steady state (Rosenberg GA et al., Citation1980) This generates a pressure gradient between tumor and surrounding normal brain, which drives interstitial fluid into the surrounding normal brain tissue, thereby causing peri-tumoral edema (Baxter & Jain, Citation1989). Convection-enhanced delivery is a method that utilizes bulk flow of the interstitial space to directly deliver small or large molecules to targeted sites. Therefore, the pressure gradient existing heterogeneously inside the tumor and/or between the tumor and surrounding brain becomes a problem. Our study, in which we infused PLD into 9L tumor model (), illustrates this problem. Although PLD first distributed inside the tumor, there was always a leak from the tumor into surrounding brain tissue at some point. Actually this leakage may become beneficial when treating highly infilitative tumor such as gliomas, however, drug distribution inside the tumor mass get compromised. Once this leakage occurred, coverage of the entire tumor with infusate became impossible. Afterward the infusate distributed into the surrounding normal brain especially through tumor-brain interface.
During early development of a treatment strategy where CED was used to infuse IL13-PE38QQR, intra-tumoral infusion was compared to peri-tumoral infusion after resection (Kunwar S, Citation2003). Drug distribution was problematic for the intra-tumoral infusion, and peri-tumoral infusion after resection was used in the subsequent study, including the PRECISE trial. The PRECISE trial is the only completed trial involving CED for malignant glioma. This study compared the efficacy of IL13-PE38QQR to Gliadel wafers in patients with a first recurrence of a glioblastoma. The IL13-PE38QQR was infused through 2–4 catheters placed 2–7 days after tumor resection. IL13-PE38QQR demonstrated a longer progression-free survival (17.7 weeks versus 11.4 weeks; p = 0.008), not a protocol-specified endpoint. However, there was no significant improvement in median survival (36.4 weeks with IL13-PE38QQR compared to 35.3 with Gliadel) (Bidros DS et al., Citation2010). Therefore, IL13-PE38QQR was determined not to be superior to Gliadel wafers. After critical analysis of this study, poor drug distribution was suggested as a possible explanation for the unfavorable result (Sampson JH et al., Citation2010). Therefore, a method to effectively cover the brain surrounding the tumor after tumor resection is required. However, delivering infusate robustly into brain surrounding the tumor after tumor resection is not an easy task. After tumor resection, the brain surrounding the tumor is affected by another lower pressure space, i.e. resection cavity. Once the infusate leaks into the resection cavity during CED, effective distribution into surrounding brain ceases ( and ). As demonstrated in , peri-tumoral CED before tumor removal can distribute infusate robustly into tumor surrounding brain, effectively covering the tumor-brain interface. Moreover, the peri-tumoral infusion before tumor removal may achieve more robust volume of distribution than intra-tumoral infusion (). Use of dexamethasone prior to infusion, further increased the volume of distribution (). The precise mechanism of this enlarged distribution after steroid use is not clear, however, dexamethasone may decrease the interstitial pressure and may provide better conditions for CED. Boucher et al. (1997) demonstrated that the treatment with dexamethasone, mannitol, and fluosemide decreased the interstitial pressure in rodent intracranial tumor model. In this context, Yang et al. (Citation2014) demonstrated that pre-treatment with dexamethasone alone or in combination with mannitol and fluosemide increased the tumor carboplatin concentrations after intra-tumoral CED of carboplatin.
Figure 3. Volume of distribution (Vd) observed in rats that received Evans blue infusions with different mode of infusions were compared. Vd after intra-tumoral infusion, peri-tumoral infusion after tumor removal, peri-tumoral infusion without tumor removal, and peri-tumoral infusion without tumor removal with prior use of dexamethasone, were 97.6 ± 3.9 mm3 (mean ± standard deviation), 74.5 ± 14.4 mm3, 112.7 ± 4.7 mm3, and 130 ± 6.6 mm3, respectively. Representative bright-field images for each group are shown at the bottom. *1; p = 0.0008, *2; p < 0.0001, *3; p < 0.0001.
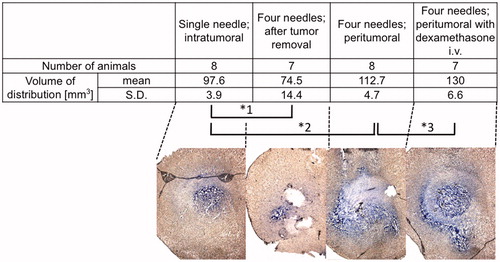
Figure 4. Schema of the current problem for post surgical peri-tumoral CED (A). After tumor resection, the brain surrounding the tumor is adjacent to a lower pressure space, i.e. resection cavity. Once the infusate leaks into the resection cavity during CED, the distribution into surrounding brain ceases. Schema of proposed pre-surgical CED (B). As demonstrated in , peri-tumoral CED prior to tumor removal can distribute infusate robustly into brain surrounding the tumor at the tumor-brain interface. Infusion from multiple cannulae inserted into the peri-tumoral area enables robust drug distribution at the tumor-brain interface. Removal of the tumor afterward may be ideal (C). Brain tissue surrounding resection cavity contains distributed drug after surgical resection.
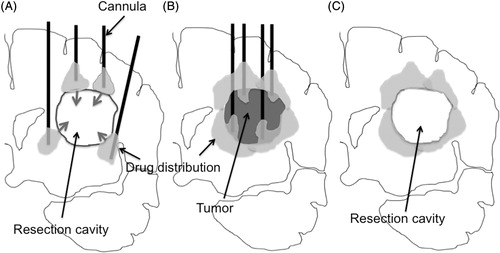
Consequently, these findings implicate the possibility of peri-tumoral infusion before removal of the tumor. It may be able to effectively cover the brain surrounding the tumor if CED of the infusate is performed with multiple cannula targeting the peri-tumoral area ( and ). Removal of the tumor afterward may be a promising strategy in the future development of CED-based anti-glioma chemotherapy (). In addtion, for intratumoral infusion against unresectable tumors such as brainstem glioma, we may need to take the possible leakage into consideration and plan the best position of catheter placement.
Conclusions
Convection-enhanced delivery enables robust distribution of the therapeutic agents at targeted site, therefore has a promise to treat tumor surrounding brain parenchyma that usually contain the invading tumor cells in cases of malignant gliomas. When targeting peri-tumoral brain parenchyma by this method, peri-tumoral infusion without tumor removal resulted in maximum volume of distribution. Prior use of steroids further increased the volume of distribution. Local interstitial convection-enhanced drug delivery targeting tumor surrounding brain tissue before tumor removal should be more effective when targeting the invading cells.
Notice of Correction:
In the version of this article published on 28 May 2014, the authors' affiliations were written incorrectly. The affiliations have been corrected in this version. The authors and editors apologize for any inconvenience caused.
Declaration of interest
The authors report no conflicts of interest. This work was supported in part by Grants-in-Aid for Young Scientists (B-23791583 to R.S.) and by Grants-in-Aid for Basic Research Program (A-21249077 to T.T.) from the Ministry of Education, Culture, Sports, Science and Technology in Japan and from Japan Society for the Promotion of Science.
References
- Baxter LT, Jain RK. (1989). Transport of fluid and macromolecules in tumors. I. Role of interstitial pressure and convection. Microvasc Res 37:77–104
- Bidros DS, Liu JK, Vogelbaum MA (2010). Future of convection-enhanced delivery in the treatment of brain tumors. Future Oncol 6:117–25
- Bobo RH, Laske DW, Akbasak A, et al. (1994). Convection-enhanced delivery of macromolecules in the brain. Proc Natl Acad Sci USA 91:2076–80
- Boucher Y, Baxter LT, Jain RK. (1990). Interstitial pressure gradients in tissue-isolated and subcutaneous tumors: implications for therapy. Cancer Res 50:4478–84
- Boucher Y, Jain RK. (1992). Microvascular pressure is the principal driving force for interstitial hypertension in solid tumors: implications for vascular collapse. Cancer Res 52:5110–14
- Boucher Y, Salehi H, Witwer B, et al. (1997) Interstitial fluid pressure in intracranial tumours in patients and in rodents. Br J Cancer 75:829–36
- Brandes AA. (2003). State-of-the-art treatment of high-grade brain tumors. Semin Oncol 30:4–9
- DeAngelis LM. (2003). Benefits of adjuvant chemotherapy in high-grade gliomas. Semin Oncol 30:15–18
- Groothuis DR. (2000). The blood-brain and blood-tumor barriers: a review of strategies for increasing drug delivery. Neuro-oncol 2:45–59
- Jemal A, Murray T, Samuels A, et al. (2003). Cancer statistics, 2003. CA Cancer J Clin 53:5–26
- Kikuchi T, Saito R, Sugiyama S, et al. (2008). Convection-enhanced delivery of polyethylene glycol-coated liposomal doxorubicin: characterization and efficacy in rat intracranial glioma models. J Neurosurg 109:867–73
- Kunwar S. (2003). Convection enhanced delivery of IL13-PE38QQR for treatment of recurrent malignant glioma: presentation of interim findings from ongoing phase 1 studies. Acta Neurochir Suppl 88:105–11
- Kunwar S, Prados MD, Chang SM, et al. (2007) Cintredekin Besudotox Intraparenchymal Study Group. Direct intracerebral delivery of cintredekin besudotox (IL13-PE38QQR) in recurrent malignant glioma: a report by the Cintredekin Besudotox Intraparenchymal Study Group. J Clin Oncol 25:837–44
- Navalitloha Y, Schwartz ES, Groothuis EN, et al. (2006). Therapeutic implications of tumor interstitial fluid pressure in subcutaneous RG-2 tumors. Neuro-oncol 8:227–33
- Rosenberg GA, Kyner WT, Estrada E (1980). Bulk flow of brain interstitial fluid under normal and hyperosmolar conditions. Am J Physiol 238:F42–9
- Saito R, Bringas JR, McKnight TR, et al. (2004) Distribution of liposomes into brain and rat brain tumor models by convection-enhanced delivery monitored with magnetic resonance imaging. Cancer Res 64:2572–9
- Sampson JH, Archer G, Pedain C, et al. (2010). Poor drug distribution as a possible explanation for the results of the PRECISE trial. J Neurosurg 113:301–9
- Stupp R, Mason WP, van den Bent MJ, et al. (2005). Radiotherapy plus concomitant and adjuvant temozolomide for glioblastoma. N Engl J Med 352:987–96
- Sugiyama S, Yamashita Y, Kikuchi T, et al. (2007). Safety and efficacy of convection-enhanced delivery of ACNU, a hydrophilic nitrosourea, in intracranial brain tumor models. J Neurooncol 82:41–7
- Vogelbaum MA, Sampson JH, Kunwar S, et al. (2007). Convection-enhanced delivery of cintredekin besudotox (interleukin-13-PE38QQR) followed by radiation therapy with and without temozolomide in newly diagnosed malignant gliomas: phase 1 study of final safety results. Neurosurgery 61:1031–7
- Walter KA, Tamargo RJ, Olivi A, et al. (1995). Intra-tumoral chemotherapy. Neurosurgery 37:1128–45
- Watanabe M, Tanaka R, Takeda N. (1992). Magnetic resonance imaging and histopathology of cerebral gliomas. Neuroradiology 34:463–9
- Yang W, Barth RF, Huo T, et al. (2014). Radiation therapy combined with intracerebral administration of carboplatin for the treatment of brain tumors. Radiat Oncol 9:25