Abstract
The purpose of this study was to evaluate both in vitro and in vivo anticancer activities against colorectal cancer (CRC) of electrospun polylactide (PLA) nanofibers loaded with 5-fluorouracil (5-Flu) and oxaliplatin. For in vitro evaluation, human CRC HCT8 cells were directly exposed to the drug-loaded fiber mats, followed with MTT and flow cytometry (FCM) assay. For in vivo evaluation, the drug-loaded fiber mats were locally implanted into mouse colorectal CT26 tumor-bearing mice, followed with histological analysis and detection of survival rate. The results showed that the drug-loaded fiber mats was similar to that of the combination of free 5-Flu and oxaliplatin in vitro cytotoxicity but was much superior to intravenous injection of free drug in vivo anticancer activities, presenting with suppressed tumor growth rate and prolonged survival time of mice. In conclusion, anticancer activities of 5-Flu and oxaliplatin against CRC can be significantly improved by using PLA electrospun nanofibers as local drug delivery system.
Introduction
Colorectal cancer (CRC) is one of the most common digestive system malignances with high morbidity and mortality. Radical surgery is the main method to cure CRC; however, about 20–30% stage II and 50–60% stage III patients will relapse within 5 years after radical surgery with poor prognosis (Li et al., Citation2013; Yeo & Paty, Citation2014).
Chemotherapy is the most curative treatment option for CRC along with de-bulking surgery to minimize the risk of recurrence. However, anticancer drugs are usually systemically administered and its circulation within the blood exposes not just tumor cells but all other body organs to the toxicity of the drugs. For example, oxaliplatin, together with 5-fluorouracil and leucovorin (Folfox regimen), is currently a mainstay of adjuvant chemotherapy in CRC patients. However, oxaliplatin-induced peripheral neuropathy is the main concern about the use of this drug. In fact, oxaliplatin can cause both acute neuropathy (transient, distal paresthesia during or shortly after the first minutes of infusion) and chronic sensory neuropathy (distal paresthesia and numbness which can lead to functional disability, in the case of grade 3 toxicity). These neurosensory symptoms increase in intensity with cumulative doses, persist between cycles and affect the quality of life in the majority of patients (Coriat et al., Citation2014; Piccolo & Kolesar, Citation2014).
Polymer-based chemotherapeutic drug delivery systems, such as drug-eluting films, gels, wafers, rods, and particles, have long been proposed as a method by which local drug concentrations can be maximized in the immediate tumor environment while systemic exposure and non-target organ toxicity is minimized. The majority of these devices are biodegradable so as to circumvent a second surgery for device removal and to avoid a chronic foreign body immune response (Langer, Citation1980; Kim et al., 2009b; De Souza et al., Citation2010; Wolinsky et al., Citation2012). Among them electrospun fibers are known as an excellent drug carrier by providing large surface area to volume for high drug loading and encapsulation efficiency, and a three-dimensional open porous structure, which can reduce the constraint to drug diffusion leading to an increase in the total fraction of drug that can be released (Qi et al., Citation2010; Wang et al., Citation2012; Zheng et al., 2013b). In addition, electrospinning affords great flexibility in producing polymer fibers with customizable fiber size, porosity, drug loading rate and release mechanisms, leading to a possibility of tailoring the drug release rate for each application. Another advantage of drug-loaded electrospun fibers is their potential as implantable device intratumorally, adjacent to tumor, or at the surgical resection margins for cancer chemotherapy of solid tumors (Xu et al., Citation2009; Toshkova et al., Citation2010; Luo et al., Citation2012; Ignatova et al., Citation2013; Liu et al., Citation2013; Zamani et al., Citation2013, Zheng et al., 2013a).
In the present study, polylactide (PLA) was used to prepare electrospun fibers loaded with oxaliplatin and 5-Flu(coded as O + F/fiber) because of its great biocompatibility and biodegradability. Then the cytotoxicity of the drug delivery system against CRC in vitro and in vivo was studied, respectively.
Materials and methods
Materials
Poly(L-lactide) (PLLA) was a gift from Changchun Institute of Applied Chemistry. Its molecular weight (Mn) and polydispersity (PD) determined by GPC were 13 800 and 3.41, respectively. Oxaliplatin and 5-Flu was purchased from Hisun Pharmaceutical Co. Ltd. (Zhejiang, China) and Aladdin Chemistry Co. Ltd. (Shanghai, China), respectively. Rabbit anti-mouse antibody of bax and bcl-2 were purchased from ZSGB-BIO Co.Ltd. (Beijing, China).
Preparation and characterization of O + F/fiber
O + F/fiber was obtained by electrospinning of PLLA solutions containing oxaliplatin and 5-Flu (4% and 20% in weight percent of PLLA used) in CHCl3/CH3OH/DMSO (80/14/6, v/v/v) at a polymer concentration of 6 wt%. Electrospinning parameters were as follows: electric field strength: 1.8–2.0 kV cm−1; air gap distance: 24 cm; inner diameter of spinneret: 0.4 mm; flow rate of solution: 1–2 ml h−1 and all the experiments were conducted at room temperature in air. In order to remove the residual solvent, the fibers collected were placed under vacuum for about 24 h at 25 °C. As a control, the unloaded PLLA fibers were prepared and coded as PLLA fiber. An environmental scanning electron microscope (ESEM, Model XL 30 ESEM FEG from Micro FEI Philips) was used to observe the morphology of O + F/fiber.
In vitro release
The in vitro drug release profile of O + F/fiber was studied. The O + F/fiber samples (20–30 mg, 2 cm × 2 cm × 0.20 mm) were incubated at 37 °C in 25 ml of acetate buffer (pH 5.0) in a thermostated shaker. At predetermined time intervals, 1.0 mL of the released solution was withdrawn for inductively coupled plasma mass spectrometry (ICP-MS) and ultraviolet (UV) analysis to detect oxaliplatin and 5-Flu concentration, respectively, and equal amount of fresh buffer solution was added back.
Cells and animals
HCT-8 cells (human colorectal cell lines) and CT-26 cells (mouse colorectal cell lines) were a gift from Jilin University. Cells were grown in DMEM media containing 10% fetal bovine serum (FBS, Gibco BRL, Rockville, MD) and 100 U mL−1 penicillin and 100 μg mL−1 streptomycin (Sigma, St. Louis, MO). The cells were cultured at 37 °C in a humidified atmosphere containing 5% CO2, dissociated with 0.25 wt% trypsin in 0.2 M PBS (pH 7.4), and centrifuged at 1000 rpm for 5 min at room temperature.
Balb/c mice were kept by School of Public Health, Jilin University, China. The animals were acclimatized at a temperature of 25 °C and a relative humidity of 70 ± 5% under natural light/dark conditions. All animal procedures were approved and controlled by the local ethics committee and carried out according to the guidelines of Chinese law concerning protection of animal life.
In vitro cytotoxicity of O + F/fiber
The cytotoxicity of O + F/fiber against HCT-8 tumor cells was assessed in vitro by the MTT staining method. HCT-8 cells were inoculated to each well of 24-well plates at a cellular density of 1 × 104 cells in 500 μL of complete DMEM media, and incubated for 24 h to allow the cells to attach to the bottom of wells. Meanwhile, wells in each plate were divided into four groups, three replicates for each group: (1) control group: only test cells were added; (2) free drug group: culture media containing free oxaliplatin and 5-Flu at the ratio of 1:5 was added into each well and the final mixed drug concentration was 0.1, 0.05 and 0.025 mg ml−1, respectively; (3) O + F/fiber group: carefully weighed O + F/fiber mats were immersed into culture media with total drug contents equivalent to their counterparts in (2); (4) PLLA fiber group. At a predetermined time (12, 24, 36, 72, 96 and 120 h), the plates were withdrawn for MTT assay.
Flow cytometry (FCM) assay
Cell cycle analyses were performed on EPICS-XL flow cytometer (Beckman Coulter, USA) and data were analyzed by EXPO32 software. HCT-8 cells were seeded on a six-well plate and pre-incubated for 24 h, followed by drug administration, similar to the protocol shown in “In vitro cytotoxicity of O + F/fiber” but only the final mixed drug concentration of 0.025 mg ml−1 was adopted in this assay. At different time points (24, 48, 72 and 96 h), the cells were washed three times with PBS, detached by trypsinization, spun down by centrifugation, and dispersed again in PBS for FCM analysis.
In vivo antitumor efficacy of O + F/fiber
The tumors were established by subcutaneous injection of CT26 cells in mice as described previously (Riediger et al., Citation2013). Briefly, cells were suspended in PBS at the cell density of 2 × 106 cells mL−1 and injected subcutaneously to each mouse at the back. The tumors were allowed to grow for around 10 d to reach the size of around 100–200 mm3. The tumor-bearing mice were randomly divided into four groups with eight animals in each group, which were treated by implantation of O + F/fiber mats(0.168 mg oxaliplatin and 0.84 mg 5-Flu per mice, O + F/fiber group), empty PLLA mats (PLLA fiber group), tail vein injection of free oxaliplatin and 5-Flu with equivalent total amount to that of O + F/fiber(coded as O + F/i.v group), and nothing as the control group. In O + F/fiber group and PLLA fiber group, animals were anesthetized by intraperitoneal injection of pentobarbital at 25 mgkg−1. A small incision was made on the skin to expose the tumor and fibrous mats were directly placed on the tumor surface. Then the wound was closed by surgical suture.
The tumor volumes of animals were monitored every other day after treatment. The length of the major axis (longest diameter) and minor axis (perpendicular to the major axis) of the tumor were measured with a vernier caliper, and the tumor volume was calculated as described previously (Yue et al., Citation2013). After 10 d treatment, animals of each group were randomly chosen and euthanized to retrieve tumors. The excised tumors were processed routinely, and sections were stained with hematoxylin and eosin (HE), and immunohistochemical (IHC) examinations of bax and bcl-2 expression. The rest mice were allowed to live until they succumbed to the tumor and survival days were recorded for each mouse for survival observation.
Results and discussion
Characterization of O + F/fiber
ESEM micrographs of O + F/fiber revealed conglutinated nanofibers with the size of about 300 nm in average diameter (). The fiber surface was smooth and no drug crystals were detected, indicating that oxaliplatin and 5-Flu had been successfully encapsulated in fibers.
In vitro drug release of O + F/fiber
Given its particular drug delivery route by directly implanting to the tumor region and bypassing the systemic circulation and normal tissues, the drug release behaviors from O + F/fiber were investigated under a simulated tumor environment of pH 5.0. As shown in , the oxaliplatin and 5-Flu release kinetics from O + F/fiber in pH 5.0 could be illustrated in two stages: around 33.5% and 35.2% of initial burst release of oxaliplatin and 5-Flu, respectively, from PLLA fibers at 10 h mainly due to the diffusion of drugs near fiber surfaces, followed by a constant slow release. The initial burst release was actually necessary to prompt delivery of antineoplastic drugs to achieve enough initial dosage to kill tumors and for those that may survive from the initial burst, a continued release was also essential to prevent their further proliferation and migration.
In vitro cytotoxicity assay of O + F/fiber
The in vitro cytotoxicity of O + F/fiber was assessed by MTT assay. As seen in , on the 12, 24 and 36 h of incubation, the free drug at all concentrations (0.1, 0.05 and 0.025 mg ml−1) showed a slightly higher cytotoxicity against HCT-8 cells than O + F/fiber. With increasing the duration of the incubation period, the cytotoxic effect of the tested implants is gradually increased due to the sustained release of drug from the fibers. The inhibition rate of HCT-8 cells in the presence of free drug and O + F/fiber at 120 h was 78.7% and 83.4% (0.025 mg ml−1), 85% and 86.1% (0.05 mg ml−1), 86.8% and 87.3% (0.1 mg ml−1), respectively. The in vitro cytotoxicity of O + F/fiber was lower at the initial period of experiment but approached or slightly surpassed that of the free drug in the later stage, indicating that O + F/fiber exhibits more sustained cytotoxicity than the combination of pure oxaliplatin and 5-Flu in vitro. There was no noticeable cytotoxicity of empty PLLA fibers.
Figure 3. Cytotoxicity of the O + F/fiber mats to human colorectal HCT-8 cells. The final mixed drug concentration of oxaliplatin and 5-Flu (at the mass ratio of 1:5) in free drug group was 0.1 mg ml−1 (A), 0.05 mg ml−1 (B) and 0.025 mg ml−1 (C), respectively. The drug content of oxaliplatin and 5-Flu in O + F/fiber is equivalent to the total drug content used in the free drug group. Each data point represents the average of triplicate samples and error bars represent standard deviation (n = 3).
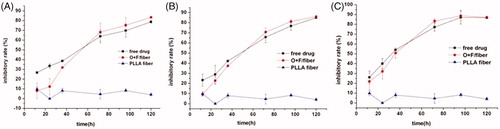
Cell cycle analysis
To further investigate the mechanism by which O + F/fiber influences HCT-8 cell growth, we studied the effects of O + F/fiber on cell cycle progression by FCM analysis. The cells treated with O + F/fiber and free drug induced a prominent change in cell cycle distribution. Rather than shortening cell cycle, this interference reduced cell amounts in cell cycle and accumulated block cells in a certain phase to cease cell cycle. As shown in the , O + F/fiber inhibited the cell growth by causing a typical G0/G1 block of the cell cycle in a time-dependent manner. A total of 50.6% of HCT-8 cells incubated with O + F/fiber were arrested at the G0/G1 phase relative to 54.4% of free drugs at 24 h post-incubation, whereas only 44.97% and 44.29% of cells in empty PLLA fiber group and control group were arrested at the G0/G1 phase (p < 0.05). The above results were in agreement with the previous studies (Hata et al., Citation2005), which showed that in a p53-dependent pathway, oxaliplatin enhanced the expression of p21waf1/cip1 protein, which may be responsible for the growth-inhibitory activity by delaying the transition from G0/G1 to S phase in HCT-116 human colorectal cells.
Figure 4. Cell cycle analysis of HCT-8 cells at 24, 48, 72 and 96 h after treatment with PLLA fiber, free drug, O + F/fiber and nothing as control.
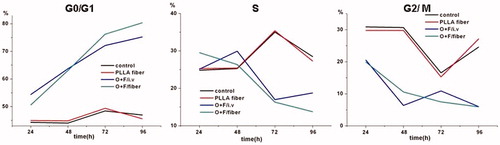
With the accumulative release of drug from O + F/fiber, G0/G1 phase fraction in O + F/fiber group gradually increased to 80.34% at 96 h compared to 75.21% of free drug group. The results indicated that O + F/fiber can more effectively inhibit cell growth against HCT-8 cells than the combination of pure oxaliplatin and 5-Flu in vitro, which is consistent with the result of in vitro cytotoxicity assay.
In vivo efficacy
The in vivo antitumor efficacy of O + F/fiber was studied in balb/c mice bearing experimental CT26 solid tumors. After the tumor size reached 150–200 mm3, the implants were directly placed on the tumor surface after surgical exposure of the tumor. The growth curves of CT26 tumors in mice followed-up for 10 d after implantation are shown in . The tumor volume in the control group and blank PLLA fiber group increased rapidly with an over 3-fold increase in the tumor volume on the 10th day post-surgery, indicating no notable effect of empty fibers and their degradable products on the tumor growth inhibition. The relative tumor volumes on the 5th day post-surgery were not distinguishable between O + F/fiber and O + F/i.v group, but the increase in the tumor volume of mice treated with free drugs became accelerated in the following time period whereas the growth rate of tumor was significantly inhibited in O + F/fiber group all along (p < 0.05, compared with O + F/i.v, PLLA fiber and control group). Obviously, the continuous inhibition of tumor growth was due to a local high dose of oxaliplatin and 5-Flu to kill tumors resulted from the sustained release of drug from fibers.
Figure 5. The curve of tumor volume plotted to growth time in CT26 tumor-bearing mice after treatment with O + F/fiber, O + F/i.v, PLLA fiber, and nothing as control. The relative volumes were obtained by dividing actual cancer volumes by that on Day 0. The results mentioned above are given as mean value ± SD, over eight mice in a group.
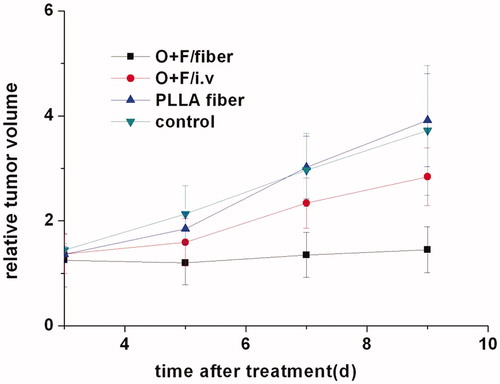
Necrosis within tumors represents a significant prognostic factor of tumor volume after chemotherapy and the survival of patients (Ogston et al., Citation2003). The histopathological studies of the excised tumor tissues, the most accurate method of assessing the response of malignant tumors to chemotherapy, were carried out to assess the necrosis in tumor tissues on the 10th day after the fiber implantation. The studies revealed that large areas of necrotic region (pink area without nuclei) can be obviously seen in tumors after treatment with O + F/fiber and free drug whereas there were still a large amount of living cells in tumors(blue area) in PLLA fiber and control group (). The necrotic region in the O + F/fiber group was larger than that of O + F/i.v.
Figure 6. Histopathological observation of local tissue samples retrieved on day 10 after treatment with O + F/i.v (A), O + F/fiber (B), PLLA fiber (C), and nothing as control (D). Bars represent 500 μm. N = necrosis; T = viable tumor tissue.
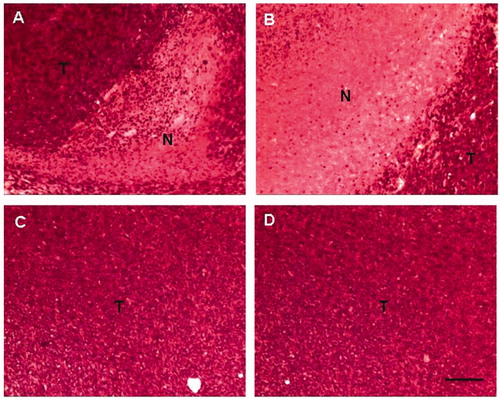
To obtain further information regarding apoptotic modulation, we examined the immunoreactivity of apoptosis-related proteins, including Bax and Bcl-2, in CT26 transplanted tumors by immunohistochemistry. Bax and Bcl-2 function as tumor pro-apoptotic and anti-apoptotic factors and are the two main members of the Bcl-2 protein family, which might interact with each other to form homodimers and heterodimers in regulating apoptosis. Therefore, alteration in the levels of Bax and Bcl-2 protein influences apoptosis (Kim et al., 2009a; Snyder et al., Citation2009). showed the immunohistochemical staining of Bax and Bcl-2 (brown cells) in tumor tissues after 10 d treatment, indicating the most significant up-regulation of Bax and down-regulation of Bcl-2 in O + F/fiber group than the groups of O + F/i.v, PLLA fiber and control (p < 0.05 compared with O + F/i.v, PLLA fiber and control group). Combined with the result of HE staining, it was concluded that the constant and local delivery of drugs from nanofibers into tumors could maintain effective concentration during the tumor development stage and induced necrosis and apoptosis, showing a superior efficacy to free drug injection.
Figure 7. Immunohistochemical staining of Bax and Bcl-2 in tumors retrieved on day 10 after treatment with O + F/i.v (A), O + F/fiber (B), PLLA fiber (C), and nothing as control (D). Bars represent 50 μm.
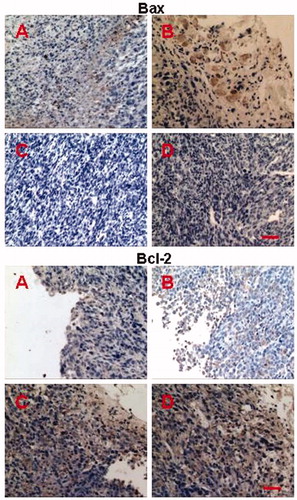
showed that the survival time in O + F/fiber group was greatly prolonged, with the median time to survival of 55 d compared with 40, 35 and 31 d in O + F/i.v, PLLA fiber and control group, respectively (p < 0.05, compared with O + F/i.v, PLLA fiber and control group; log-rank test). The locally sustained release of oxaliplatin and 5-Flu from implanted fibers could retain the local drug concentration within tumors while reducing the side effects. The improved efficacy and systemic safety would undoubtedly be favorable for the quality of life and survival time.
Figure 8. Survival curve of CT26 tumor-bearing mice after treatment with O + F/i.v (A), O + F/fiber (B), PLLA fiber (C), and nothing as control (D).
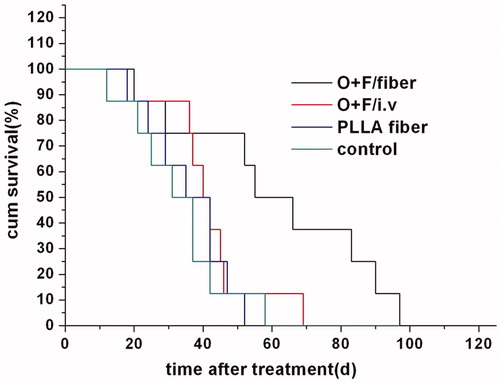
In conclusion, electrospun PLLA fibers were used to local deliver oxaliplatin and 5-Flu against CRC in vitro and in vivo. The drug-loaded PLLA fibers showed in vitro antitumor efficacy against HCT-8 cells in a time-dependent manner similar to that of the combination of free oxaliplatin and 5-Flu. The suppressed tumor growth and prolonged animal survival time after the implantation of drug-loaded PLLA fibers indicated a superior in vivo antitumor activity to other treatment possibly due to its sustained drug release profile, and significant necrosis were also detected in the tumor tissues retrieved. These results indicated the great potential of drug-loaded electrospun fibers as an implantable, easy-to-use local drug delivery system against CRC, especially suitable for the prevention of local tumor recurrence after surgery.
Declaration of interest
This study was financially supported by the National Natural Science Foundation of China (Project No.21004062, 51103148, and 51373167) and by the Ministry of Science and Technology of China (“973 Project”, No. 2009CB930102).
References
- Coriat R, Alexandre J, Nicco C, et al. (2014). Treatment of oxaliplatin-induced peripheral neuropathy by intravenous mangafodipir. J Clin Investig 124:262–72
- De Souza R, Zahedi P, Allen CJ, Piquette-Miller M. (2010). Polymeric drug delivery systems for localized cancer chemotherapy. Drug Deliv 17:365–75
- Hata T, Yamamoto H, Ngan CY, et al. (2005). Role of p21waf1/cip1 in effects of oxaliplatin in colorectal cancer cells. Mol Cancer Ther 4:1585–94
- Ignatova M, Rashkov I, Manolova N. (2013). Drug-loaded electrospun materials in wound-dressing applications and in local cancer treatment. Expert Opin Drug Deliv 10:469–83
- Kim KW, Moretti L, Mitchell LR, et al. (2009a). Combined Bcl-2/mammalian target of rapamycin inhibition leads to enhanced radiosensitization via induction of apoptosis and autophagy in non-small cell lung tumor xenograft model. Clin Cancer Res 15:6096–105
- Kim S, Kim JH, Jeon O, et al. (2009a). Engineered polymers for advanced drug delivery. Eur J Pharmaceut Biopharmaceut 71:420–30
- Langer R. (1980). Polymeric delivery systems for controlled drug release. Chem Eng Commun 6:1–48
- Li S, Gao J, Gu J, et al. (2013). MicroRNA-215 inhibits relapse of colorectal cancer patients following radical surgery. Med Oncol 30:549
- Liu S, Zhou GY, Liu DX, et al. (2013). Inhibition of orthotopic secondary hepatic carcinoma in mice by doxorubicin-loaded electrospun polylactide nanofibers. J Mater Chem B 1:101–9
- Luo XM, Xu GS, Song HX, et al. (2012). Promoted antitumor activities of acid-labile electrospun fibers loaded with hydroxycamptothecin via intratumoral implantation. Eur J Pharmaceut Biopharmaceut 82:545–53
- Ogston KN, Miller ID, Payne S, et al. (2003). A new histological grading system to assess response of breast cancers to primary chemotherapy: prognostic significance and survival. Breast 12:320–7
- Piccolo J, Kolesar JM. (2014). Prevention and treatment of chemotherapy-induced peripheral neuropathy. Am J Health-System Pharm 71:19–25
- Qi RL, Guo R, Shen MW, et al. (2010). Electrospun poly(lactic-co-glycolicacid)/halloysite nanotube composite nanofibers for drug encapsulation and sustained release. J Mater Chem 20:10622–9
- Riediger C, Wingender G, Knolle P, et al. (2013). Fms-like tyrosine kinase 3 receptor ligand (Flt3L)-based vaccination administered with an adenoviral vector prevents tumor growth of colorectal cancer in a BALB/c mouse model. J Cancer Res Clin Oncol 139:2097–110
- Snyder CM, Shroff EH, Liu J, Chandel NS. (2009). Nitric oxide induces cell death by regulating anti-apoptotic BCL-2 family members. Plos One 4:e7059
- Toshkova R, Manolova N, Gardeva E, et al. (2010). Antitumor activity of quaternized chitosan-based electrospun implants against Graffi myeloid tumor. Int J Pharmaceut 400:221–33
- Wang SG, Zheng FY, Huang YP, et al. (2012). Encapsulation of amoxicillin within laponite-doped poly(lactic-co-glycolic acid) nanofibers: preparation, characterization, and antibacterial activity. Acs Appl Mater Interfac 4:6393–401
- Wolinsky JB, Colson YL, Grinstaff MW. (2012). Local drug delivery strategies for cancer treatment: gels, nanoparticles, polymeric films, rods, and wafers. J Control Rel 159:14–26
- Xu XL, Chen XS, Wang ZF, Jing XB. (2009). Ultrafine PEG-PLA fibers loaded with both paclitaxel and doxorubicin hydrochloride and their in vitro cytotoxicity. Eur J Pharmaceut Biopharmaceut 72:18–25
- Yeo HL, Paty PB. (2014). Management of recurrent rectal cancer: practical insights in planning and surgical intervention. J Surg Oncol 109:47–52
- Yue J, Liu S, Xie ZG, et al. (2013). Size-dependent biodistribution and antitumor efficacy of polymer micelle drug delivery systems. J Mater Chem B 1:4273–80
- Zamani M, Prabhakaran MP, Ramakrishna S. (2013). Advances in drug delivery via electrospun and electrosprayed nanomaterials. Int J Nanomed 8:2997–3017
- Zheng FY, Wang SG, Shen MW, et al. (2013a). Antitumor efficacy of doxorubicin-loaded electrospun nano-hydroxyapatite-poly(lactic-co-glycolic acid) composite nanofibers. Polym Chem 4:933–41
- Zheng FY, Wang SG, Wen SH, et al. (2013b). Characterization and antibacterial activity of amoxicillin-loaded electrospun nano-hydroxyapatite/poly(lactic-co-glycolic acid) composite nanofibers. Biomaterials 34:1402–12