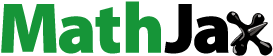
Abstract
Streptokinase is one of the most commonly used thrombolytic agents for the treatment of thromboembolism. Short half-life of the streptokinase requires administration of higher dose which results in various side effects including systemic haemorrhage due to activation of systemic plasmin. To increase the selectivity of the streptokinase and hence to reduce side effects, various novel carriers have been developed. Among these carriers, liposomes have been emerged as versatile carrier. In the present study, highly selective target-sensitive liposomes were developed and evaluated by in vitro and in vivo studies. Prepared liposomes were found to release streptokinase in vitro following binding with activated platelets. Intravital microscopy studies in thrombosed murine model revealed higher accumulation of liposomes in the thrombus area. In vivo thrombolysis study was performed in the human clot inoculated rat model. Results of the study showed that target-sensitive liposomes dissolved 28.27 ± 1.56% thrombus as compared to 17.18 ± 1.23% of non-liposomal streptokinase. Further, it was also observed that target-sensitive liposomes reduced the clot dissolution time as compared to streptokinase solution. Studies concluded that developed liposomes might be pragmatic carriers for the treatment of thromboembolism.
Introduction
The short half-lives of thrombolytic drugs, usually between 3 and 20 min, necessitate administration of high dosages of these agents in systemically active forms over an extended period of time. The short half-life is due to rapid inactivation of plasminogen activators by the components of the fibrinolytic system such as Plasminogen Activator Inhibitor-1 (PAI-1) and circulating antibodies. Various techniques have been employed to enhance stability of plasminogen activators against these components and hence to increase the half-life, which ultimately increase the accumulation at the site of thrombus. PEGylation and novel carrier constructs including liposomes and polymeric particles are most prevalently used. Liposomal encapsulation of plasminogen activators might increase efficacy, improve selectivity, possibly reduce or eliminate antigenic complications, retard systemic deactivation of the agents and effectively lengthen the brief half-lives of the agents. Nguyen et al. (Citation1989) demonstrated in vitro the ability of liposomes to protect streptokinase in plasma without significant loss of therapeutic activity. Plasminogen activators entrapped in liposomes have shown substantial reductions in the time required to obtain reperfusion and increased the digestion of thrombus as compared to freely infused plasminogen activators.
Moreover, for better therapeutic efficacy, drug should be localized at the target site, i.e. thrombus and should avoid its non-specific uptake by unwanted organs. Therefore, a well-designed drug delivery system; surface modified with targeting moiety for the delivery of thrombolytic agents to the site of thrombus may provide an effective alternative (Vyas & Vaidya, Citation2009).
Further, the design of a drug carrier should be such that it unloads the payload when it arrives at the target site. Very few examples of such ideal carrier exist. Liposomes have been used as a drug carrier for almost 3 decades, but few studies have focused on the design of a target-triggered release mechanism until the target-sensitive liposomes were first studied by Huang and associates in the mid-80s.
Under physiological conditions, DOPE aggregates into inverted micellar or inverted hexagonal (HII) structures (Cullis & Kruijff, Citation1979). This is due to the presence of a poorly hydratable phosphorylethanolamine head group (Cevc & Marsh, Citation1986) and the two bulky cis unsaturated fatty acyl chains in the DOPE molecule. The dynamic shape of the molecule is that of an inverted cone which readily aggregates to form inverted micelles or cylinders (Cullis & Kruijff, Citation1979). However, if a charged or highly hydratable amphiphile is mixed with DOPE, the mixture has a much higher tendency to be arranged into bilayer structures. It has already been reported that diacylated peptide imparted more stability to the liposomal bilayer as compared to monoacylated peptide (Brynestad et al., Citation1990). Therefore, to improve the incorporation of acylated peptide to the liposomal bilayer, peptide was palmitized by coupling two palmitic acid residues to the terminal lysine of the cyclic peptide.
In a recent study, we have developed target-sensitive liposomes which were prepared by using moderate affinity peptide c(RGDfK) acylated with palmitic acid (Vaidya et al., Citation2011). In vitro results revealed that liposomes targeted activated platelets and destabilized thereafter interaction with activated platelets. Thus, it was suggested that target-sensitive liposomes might be beneficial in the targeted delivery of thrombolytic agents to the site of blood clot where activated platelets are embedded. However, for in vivo application, targeting ligand with higher affinity to the target site would be more effective than moderate affinity ligand. Thus, in consequence of the previous study, target-sensitive liposomes were developed using peptide having higher affinity towards activated platelets. c(RGD) peptide [CNPRGDY(OEt)RC] has been investigated and found to have higher affinity to GP IIb/IIIa receptors on platelet surface as compared to other receptors. Thus, [CNPRGDY(OEt)RC] peptide was selected in the present study for the preparation of target-sensitive liposomes. Developed liposomes were characterized for in vivo targeting by intravital microscopy. Further, in vivo therapeutic efficacy was evaluated using human clot inoculated rat model.
Material and methods
Peptides were procured from USV Ltd (Mumbai, India). 1-(3-dimethylaminopropyl)-3-ethyl carbodiimide hydrochloride (EDC), N-hydroxy succinimide (NHS), palmitic acid, lysine and dicyclohexyl carbodiimide (DCC) were purchased from Himedia Ltd. (Mumbai, India). Di-tertiary butyl pyrocarbonate was purchased from Spectrochem (Mumbai, India). Sephadex G-75, dioleylphosphatidyl ethanolamine (DOPE) and D-Val-Leu-Lys-p-nitroanilide dihydrochloride (S-2251) were purchased from Sigma Aldrich (St. Louis, MO). Fluorescent labelled streptokinase (FITC-SK) was synthesized by using FITC labelling kit (KT-31, Bangalore Genei, Bengaluru, India) according to the manufacturer instruction. Synthesis of acylated peptide was described in our recent publication (Vaidya et al., Citation2011). Synthesized compound was characterized by FTIR and mass spectroscopy. Activity of acylated peptide was determined by aggregometry study and it was found that acylated peptide shows activity near about to unmodified peptide. Thus, it was suggested that acylated peptide may be used as a targeting motif to target GP IIb/IIIa receptors present on the activated platelets.
Development of liposomes
Liposomes were prepared by the procedure reported earlier by Ho et al. (Citation1986) and recently published by us (Vaidya et al., Citation2011). Briefly, DOPE and acylated peptide were dissolved in chloroform and solvent was evaporated using rotary vacuum evaporator (Strike 102, Steroglass, Perugia, Italy). A thin lipid film was formed which was hydrated using streptokinase (SK) solution (1, 35 000 IU) in PBS (pH 7.4). Liposomal dispersion was vortexed followed by sonication for 3 min to transform them into small unilammelar liposomes. For the formation of fluorescent labelled liposomes, FITC-SK was used in the dispersion media. The unentrapped SK/FITC-SK from the prepared vesicular system was separated by gel filtration (Fry et al., Citation1978) using Sephadex G-100. Control liposomes (C-Lip) were prepared using DOPC as a constituent lipid in place of DOPE by the procedure used for the preparation of target sensitive liposomes.
Physicochemical properties of liposomes
Transmission electron microscopy (TEM) was performed for morphological study (JEOL, Tokyo, Japan). Vesicles size and size distribution was determined using photon correlation spectroscopy (Zeta Sizer Nano ZS 90, Malvern Instruments Ltd, Worcestershire, UK).
Encapsulation efficiency of SK in liposomes was determined by measuring the SK activity after solubilizing the liposomal membrane with 0.1% Triton X-100. The SK activity was determined according to the previously reported method (Bode et al., Citation1991; Couto et al., Citation2004) with a slight modification. Entrapment efficiency was calculated by the following equation: C/T × 100 (C: amount of SK incorporated into liposome, T: total amount of SK).
In vitro release study
In vitro release study was performed according to procedure reported earlier by Baek et al. (Citation2009). Briefly, SK entrapped liposomes were placed in a closed vial and immersed in shaking water bath at 37 °C. At predetermined time intervals, in vitro release rate of SK from liposomes was determined by separating liposomes from the release medium by ultracentrifugation at 150 000 g for 1 h. SK content in the supernatant was determined by following the procedure as described earlier.
Release study was also performed in the presence of activated platelets to evaluate the effect of binding on the release behavior/target sensitivity of the carrier. Briefly, platelets (2 × 108/ml) were incubated in the wells of a collagen-coated microplate. Adhered platelets were activated using thrombin (1 U/ml), to which 10 µl liposomes formulations were added and incubated up to 90 min. At different time intervals, plasmin substrate solution [(1:3 vol/vol) 4 mM S-2251 in H2O and plasminogen (0.75 CU/ml) in S-2251 assay buffer (50 mM Tris and 110 mM sodium chloride)] was added to each well and absorbance was recorded at 405 nm by using a microplate reader (Molecular Devices, Sunnyvale, CA). Absorbance after disruption of liposomes with 0.1% Triton X-100 was considered as 100. The percent content release was calculated according to the formula
where A and At are the absorbance after interaction of formulations with platelets and absorbance after lysis of liposomes with Triton X-100, respectively. A0 is the absorbance of control. To assess the release behavior in the presence of resting platelets that simulate the release in systemic circulation, liposomes were incubated with resting platelets suspension and drug release was determined by the above-described procedure.
Platelet binding studies with RGD-modified liposomes
Targeting potential, i.e. platelets binding ability of the liposomes was evaluated by performing confocal laser scanning microscopy. Further, quantitative binding to the platelets was evaluated using fluorescence-activated cell sorting (FACS) analysis. For the purpose, liposomal formulations were prepared encapsulating FITC-SK as fluorescent marker. For the experiment, platelets were obtained by the reported procedure with minor modification.
Confocal laser scanning microscopy was performed to evaluate the association of FITC-labelled liposomes with rhodamine 6G-stained activated platelets. Initially, platelets were adhered and activated to the collagen-coated coverslips as described earlier (Vaidya et al., Citation2011). Rhodamine 6G was used to stain the platelets adhered onto collagen-coated coverslips (Massberg et al., Citation1998). After staining and washing with PBS (pH 7.4), platelets were incubated with FITC-labelled liposomes. This experiment enabled simultaneous observation of adhered platelets (red fluorescence from rhodamine 6G) and fluorescence associated with platelet (green fluorescence from FITC) after interaction of liposomes with platelets. After 30 min of incubation, coverslips were treated with 1% paraformaldehyde to fix the cells. Fixed platelets were washed 10 times with PBS (pH 7.4), applied with mounting media and observed under confocal microscope (Zeiss LSM 510, Jena, Germany).
For flow cytometry study, platelets (2 × 108/ml) in suspension were activated by incubating with thrombin (1 U/ml) for 30 min. Resting platelet or thrombin-activated platelets were incubated with FITC-labelled liposomes. FITC fluorescence, for gated platelet populations, was analyzed in a Becton Dickinson FACSCalibur flow cytometer for 10 000 counts per sample aliquot and fluorescence data were recorded accordingly.
In vivo studies
In vivo studies were carried out as per the guidelines compiled by the Committee for the Purpose of Control and Supervision of Experiments on Animals (CPCSEA, Ministry of Culture, Government of India). All the study protocols were approved by the Animal Ethical Committee of the Dr. H.S. Gour University (Sagar, India).
In vivo targeting: intravital microscopy
To assess the targeting potential of developed formulations to the thrombus in vivo, intravital microscopy was performed. For the study, Swiss strain mice (male, 6–8 weeks old) of 13 g were used. To confirm the formation of thrombus at the area where FeCl3 treatment has been given, control intravital microscopy was performed (Nayak et al., Citation2011). Briefly, platelets were isolated from mice blood and fluorescently labelled with Calcein-AM (2.5 μg/ml). Mice were anesthetized with Avertin (300 mg/kg). Labelled platelets were infused through retro-orbital plexus and mesentery was exteriorized through a midline abdominal incision. Mesenteric arteriole was visualized at 40× with inverted epifluorescence videomicroscope (Nikon Inc., Melville, NY, model Eclipse Ti-E) equipped with monochrome CCD cooled camera (DS-Qi1MC). Injury was induced to arteriole by topical application of 20% FeCl3-saturated filter paper for 5 min. Digital images of adhesion and aggregation of fluorescently labelled platelets were recorded for 20 min and analyzed using NIS-Elements AR imaging software (Nikon Inc., Melville, NY).
In separate sets of experiments, FITC-labelled liposomal formulations were administered to the mice via femoral vein after 30 min of thrombus formation, i.e. 30 min post application of FeCl3 on the surface of mesenteric artery. Mesenteric arteriole was visualized at 40× with inverted epifluorescence videomicroscope as described above. Images of the liposomes targeting/accumulation to thrombus area were recorded at appropriate time intervals up to 1 h.
Quantitative thrombus localization study
Thrombus localization quantitatively was performed according to the procedure reported earlier (Kim et al., Citation1998; Yim et al., Citation2009), with minor modifications. Wistar rats weighing between 450 and 550 g were anaesthetized with pentobarbitol (100 mg/kg). Incision was made in the neck and the right carotid artery was isolated. A small piece of Parafilm “M” is placed under the vessel to isolate it from surrounding tissues throughout the experiment. Thrombus formation was initiated by application of filter paper saturated with 20% FeCl3 solution, to the carotid artery (Kurz et al., Citation1990). The paper was allowed to remain on the vessel till thrombus formation. After 1 h of blood reperfusion, FITC-labelled liposomal formulations were administered via the femoral vein. To measure the fluorescence intensity, thrombus was excised and homogenized in a glass homogenizer with homogenizing buffer (0.32 M sucrose, 100 mM HEPES, pH 7.4). Homogenate was centrifuged and supernatant was suspended in a lysis buffer (1% Triton X-100, 100 mM NaCl, 0.1% SDS, 0.5% Na-deoxycholate) and incubated for 30 min at 4 °C and then centrifuged. The supernatant was used for fluorescence measurements.
In vivo thrombolysis study
In vivo thrombolysis study was performed according to the procedure reported earlier by Alonso et al. (Citation2009), with modifications. Wistar rats weighing between 450 and 550 g were anaesthetized with pentobarbitol (100 mg/kg). Incision was made in the neck and the right carotid artery was isolated and a small piece of Parafilm “M” is placed under the vessel to isolate it from surrounding tissues throughout the experiment. The internal carotid artery was ligated and a 1 mm2 human whole blood clot was introduced into the common carotid artery by the use of an external carotid artery catheter. The external carotid artery was also ligated afterwards. In the animal containing thrombus, various formulations were administered by femoral veins at a dose equivalent to 7500 IU. Simultaneously, human plasminogen was administered to maintain the physiologic level of plasminogen (Sazonova et al., Citation2009). After 10 min and 60 min of treatment, vessels were dissected and thrombus was blotted using filter paper. Weight of dry thrombus was determined to calculate the percentage thrombolysis.
Results and discussion
Development and characterization of TS liposomes
It has already been reported that fatty acid helps in the stabilization of bilayer formed by DOPE (Duzgunes et al., Citation1985). Therefore, DOPE and acylated-RGD peptide were used to prepare TS liposomes. Liposomes were found to be in the range of 100–120 nm and encapsulation efficiency was found to be ∼18%. TEM study suggested that developed liposomes are spherical in shape and unilamellar in nature (data not shown).
In vitro drug release study showed that drug released from the liposomes in the PBS (pH 7.4) was nearly 40% in 12 h () which depicted that liposomes could trap the drug effectively with minimum release in the systemic circulation while enroute to the target. TS liposomes have the property of not only binding with the target cells but also subsequent self-destabilization property. The entrapped drug released at the target site, i.e. after binding with the activated platelets, is shown in the . It was found that about 90% of entrapped drug released within 40 min of incubation. Drug release after interaction with the platelets adhered on collagen-coated microplate suggested that it may be due to contact of RGD-conjugated TS liposomes to the integrin receptors on the activated platelets followed by destabilization of liposomes. However, the mechanism of destabilization is yet to be explored. It may be either due to migration of acylated peptide from the bilayer and its subsequent accumulation at the surface of platelets or by the contact between the liposomes binds to the same surface of the target cell. However, drug release was inhibited by pre-incubation of platelet surface with an excess free RGD peptide indicating drug release is induced by interaction of the acylated RGD with integrin GP IIb/IIIa on the activated platelets leaving liposomes destabilized. Further, it was recorded that liposomes incubated with resting platelets did not release the content as efficiently as with activated platelets, as the former do not express integrin receptor which corresponds to RGD, hence it remains associated with liposomal bilayer imparting needed stability. Drug release after interaction with resting platelets might be ascribed to some degree of activation of these cells during isolation procedure. Release of content from control liposomes (DOPC-based liposomes) in the presence of resting as well as activated platelets was found to be less than 10% which further confirmed the target sensitivity of the DOPE-based liposomes.
In vitro targeting potential of the developed system
Microscopic observation
In order to confirm the binding of TS liposomes on to the activated platelets, confocal microscopy study was conducted using FITC-labelled RGD-liposomes. Rhodamine 6G was used to label the platelets (Massberg et al., Citation1998). shows platelets stained with rhodamine, whereas represents same platelets treated with FITC-labelled TS liposomes and control liposomes, respectively. show the merged/superimposed representation of these two images (A and B). The results clearly demonstrated that FITC-labeled liposomes co-localize with rhodamine-stained platelets. Further, it was found that control liposomes showed similar fluorescence as with TS liposomes. This may be ascribed to the presence of RGD peptide at the surface of control as well as TS liposomes.
Figure 2. Confocal microscopic images of platelets incubated with TS liposomes (A1,B1,C1) and control liposomes (A2,B2,C2). A1 and A2 represent platelets incubated with rhodamine; B1 and B2 represent platelets incubated with FITC-labeled formulations and C1 and C2 are superimposed images of A and B.
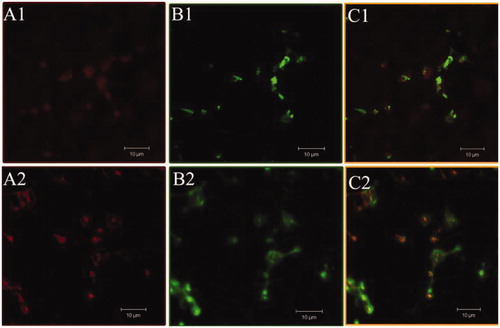
Flow cytometry
Platelets in suspension were used to assess the quantitative binding of the developed liposomes. It may be observed from that both types of liposomes (control as well as TS liposomes) bound equally to resting platelets. However, liposomes binding to the activated platelets were higher as compared to resting platelets and high fluorescent shifting was observed as shown in the fluorescence histogram. Results confirmed that, in resting state, the integrins αIIbβ3 were not appropriately conformed to bind RGD peptide. However, following exposure to thrombin integrin receptors underwent conformational changes and become competent to bind with RGD-containing liposomes with higher affinity as compared to resting platelets.
In vivo targeting by intravital microscopy
To investigate the targeting ability of the developed liposomal formulations, we monitored liposomes accumulation at the sites of FeCl3-induced thrombus in mesenteric arteriole of mice using intravital fluorescence microscopy. In this study, real-time in vivo imaging of the thrombus formation and interaction of administered liposomes with platelets enmeshed in formed thrombus were evaluated.
In control mice, platelets rapidly adhered to the site of endothelial desquamation and formed small thrombi within 10 min, which increased progressively leading to vessel occlusion up to 60% within 20 min (). In a different set of experiment, liposomal formulations were administered to the mice in which thrombus was already created by FeCl3 application. Results showed that liposomes (DOPC- and DOPE-based) selectively accumulated in the area of thrombus (). However, target sensitive liposomes destabilized after interaction with activated platelets embedded in the thrombus as diffuse fluorescence was observed in the area of thrombus (). Further, liposomes were observed to penetrate deeply in the thrombus (marked with arrows in the photographs). Deep penetration of the liposomal formulations may be ascribed to nanosize of the prepared carriers. As it has already been reported that fibrin network forms pores of size less than 1 µm in the thrombus, carriers having size in the nanorange might be penetrated deeply inside the clot. These carriers might release drug inside the clot and induce intraclot lysis, consistent with higher thrombolytic activity observed below.
Figure 4. Intravital microscopic images of thrombus area of the mesenteric arteriole of mice following administration of FITC-labeled control liposomes (B) and FITC-labeled TS liposomes (C). (A) Represents control thrombus area in which platelets were stained with calcein-AM.
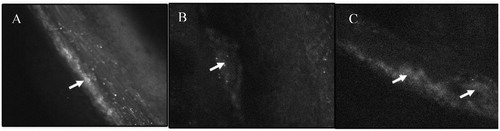
Further, accumulation of the developed liposomes in the thrombus was confirmed by determining the quantity of FITC-labelled liposomes in the thrombus using spectrofluorophotometric analysis (). Initially, accumulation of liposomes was found to be comparatively low as compared to non-liposomal SK. However, it was found that TS liposomes accumulated 3-times higher than free SK after 4 h of administration. Increased localization of RGD peptide-anchored liposomes in the thrombus in comparison to free SK is principally based on the concept of site-specific delivery of carrier contents by using RGD-based navigation. Thus, better uptake of the drug was attributable to receptor-specific accumulation of RGD peptide-anchored liposomes in the thrombus area.
In vivo thrombolysis study
Developed formulations were also evaluated for their therapeutic activity (thrombolysis). In vivo thrombolysis studies were performed in the Wistar rats using human clots placed in the vessels. The main reason for using a human clot is the poor activity of SK against rat clots. It has also been reported that, SK is minimally reactive with rat plasminogen whereas other components of rat fibrinolytic system (e.g. α2AP) appear to react normally with human plasminogen (Lijnen et al., Citation1994; Fay et al., Citation2007). Therefore, in order to simulate human clot model, thrombolysis study was performed in the rats supplemented with physiologic levels of human plasminogen (Sazonova et al., Citation2009).
Results of the study () showed that, thrombolytic activity of liposomal formulations was found higher than that of non-liposomal SK at all time point. After 30 min of treatment, the percent thrombolytic activity of TS liposomes was found 28.27 ± 1.56% as compared to 17.18 ± 1.23% of non-liposomal SK. Results demonstrated that RGD peptide-conjugated TS liposomes expressed significantly higher (p < 0.001) thrombolytic activity as compared to their non-liposomal counterparts. Higher thrombolytic activity of TS liposomes might be due to higher and instant release of SK from target-sensitive liposomes at the site of clot following interaction with activated platelets. Moreover, percent thrombolysis of SK solution was found less as compared to liposomal formulations (24.27 ± 1.56% of SK versus 40.24 ± 1.74% of TS liposomes) following 2 h of treatment, which underscored prolonged actions of liposomal formulations. Further, study with control liposomes revealed that after 30 min treatment non-liposomal SK evoked higher thrombolytic activity as compared to liposomal formulations, which might be ascribed to slow release of SK from liposomes and lesser availability of SK at the site to bind with plasminogen. However, after 1 h of treatment liposomal SK demonstrated higher activity, which confirms the stability of entrapped SK. Entrapped SK released for longer period of time and exhibited higher thrombolytic activity as compared to non-liposomal SK. Furthermore, the activity of TS liposomes-based formulations was higher as compared to control liposomes because of higher and instant release of SK from TS liposomes at the site of thrombus.
Figure 6. Thrombolytic activity of various SK formulations at different time points (data presented are mean ± SD, n = 6).
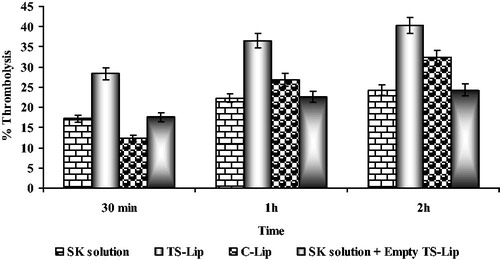
In order to evaluate the effect of liposomes on the thrombolytic activity of SK, extent of thrombolysis was determined following administration of SK solution with empty liposomes. It was found that percent thrombolysis was equal to the activity of SK solution. Thus, on the basis of these studies it can be concluded that higher thrombolytic activity was not due to pressure created by the blocking of the channels in the thrombus. Moreover, it was due to the fact that liposomal encapsulation reduces the premature inactivation and prevents degradation of enzymes in the plasma vis a vis releases drug at the site of thrombus may be by self-destruction mechanism.
Conclusion
Present study was aimed at developing novel carrier systems for delivery of thrombolytic agents to the site of thrombus and release of liposomal contents by self-destabilization mechanism. From in vitro studies prepared liposomes were found to bind more effectively to activated platelets and release the payload. Real-time in vivo imaging demonstrated plasma stability of the system and further confirmed the targeting ability of the prepared system. Nanosize liposomes accumulated in the interior of the thrombus and released the drug thereafter. In the thrombosed animal model, prepared liposomes revealed higher thrombolytic activity as compared to control liposomes and plain streptokinase solution. The present study concluded that prepared systems will be highly effective carriers for the delivery of thrombolytic agents for treatment of thromboembolism.
Acknowledgements
Author B. Vaidya wants to acknowledge All India Council of Technical Education (AICTE), New Delhi, for providing funding in the form of National Doctoral Fellowship (NDF). Authors would like to acknowledge the help of Prof. S. C. Lakhotia, Department of Zoology, BHU, Varanasi, India, to conduct confocal laser scanning microscopy. Authors also want to acknowledge Sophisticated Analytical Instrumentation Facility (SAIF), Punjab University, Chandigarh, India, to perform TEM, FTIR, NMR and mass spectroscopy.
Declaration of interest
The research was supported in part by grants received by D. Dash from the Council of Scientific & Industrial Research (CSIR) and the Department of Science & Technology (DST), Government of India. Funding was also provided from the All India Council of Technical Education (AICTE), New Delhi, in the form of National Doctoral Fellowship (NDF). The authors report no declarations of interest.
References
- Alonso A, Dempfle CE, Martina AD, et al. (2009). In vivo clot lysis of human thrombus with intravenous abciximab immunobubbles and ultrasound. Thromb Res 124:70–4
- Baek SH, Park SJ, Jin SE, et al. (2009). Subconjunctivally injected, liposome-encapsulated streptokinase enhances the absorption rate of subconjunctival hemorrhages in rabbits. Eur J Pharm Biopharm 72:546–51
- Bode C, Meinhardt G, Runge MS, et al. (1991). Platelet-targeted fibrinolysis enhances clot lysis and inhibits platelet aggregation. Circulation 84:805–13
- Brynestad K, Babbitt B, Huang L, Rouse BT. (1990). Influence of peptide acylation, liposome incorporation and synthetic immunomodulators on the immunogenicity of 1-23 peptide of glycoprotein D of Herpes simplex virus: implication for subunit vaccines. J Virol 64:680–5
- Cevc G, Marsh D. (1986). Hydration of noncharged lipid bilayer membranes: theory and experiments with phosphatidylethanolamine. Biophys J 47:21–31
- Couto LT, Donato JL, de Nucci G. (2004). Analysis of five streptokinase formulations using the euglobulin lysis test and the plasminogen activation assay. Braz J Med Biol Res 37:1889–94
- Cullis PR, De Kruijff B. (1979). Lipid polymorphism and the functional roles of lipids in biological membranes. Biochim Biophys Acta 669:399–420
- Duzgunes N, Straubinger RM, Baldwin PA, et al. (1985). Proton-induced fusion of oleic acid-phosphatidylethanolamine liposomes. Biochemistry 24:3091–8
- Fay WP, Garg N, Sunkar M. (2007). Vascular functions of the plasminogen activation system. Arterioscler Thromb Vasc Biol 27:1231–7
- Fry DW, White JC, Goldman ID. (1978). Rapid separation of low molecular weight solute from liposomes without dilution. Anal Biochem 90:809–15
- Ho RJY, Rouse BT, Huang L. (1986). Target-sensitive immunoliposomes: preparation and characterization. Biochemistry 25:5500–6
- Kim IS, Choi HG, Choi HS, et al. (1998). Prolonged systemic delivery of streptokinase using liposome. Arch Pharm Res 21:248–52
- Kurz KD, Main BW, Sandusky GE. (1990). Rat model of arterial thrombosis induced by ferric chloride. Thromb Res 60:269–80
- Lijnen HR, van Hoef B, Beelen V, Collen D. (1994). Characterization of the murine plasma fibrinolytic system. Eur J Biochem 224:863–71
- Massberg S, Enders G, Leiderer R, et al. (1998). Platelet-endothelial cell interactions during ischemia/reperfusion: the role of P-selectin. Blood 92:507–15
- Nayak MK, Singh SK, Roy A, et al. (2011). Anti-thrombotic effects of selective estrogen receptor modulator tamoxifen. Thromb Haemost 106:624–35
- Nguyen PD, O’rear EA, Johnson AE, et al. (1989). Thrombolysis using liposomal-encapsulated streptokinase: an in vitro study. Proc Soc Exp Biol Med 192:261–9
- Sazonova IY, Mcnamee RA, Houng AK, et al. (2009). Reprogrammed streptokinases develop fibrin-targeting and dissolve blood clots with more potency than tissue plasminogen activator. J Thromb Haemost 7:1321–8
- Vaidya B, Nayak MK, Agrawal GP, et al. (2011). Development and characterization of site specific target sensitive liposomes for the delivery of thrombolytic agents. Int J Pharm 403:254–61
- Vyas SP, Vaidya B. (2009). Targeted delivery of thrombolytic agents: role of integrin receptors. Expert Opin Drug Deliv 6:499–508
- Yim YS, Choi JS, Jang SB, et al. (2009). Pharmacokinetic properties and tissue storage of FITC conjugated SA-MnMEIO nanoparticles in mice. Curr Appl Phys 9:e304–7