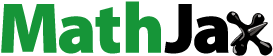
Abstract
To investigate the physicochemical properties, immunosafety and chronic toxicity of monomethoxypoly(ethylene glycol)-b-poly(lactic acid) (mPEG-PLA), a copolymer used as a carrier for paclitaxel (PTX) delivery. The H-Nuclear Magnetic Resonance (H-NMR), dynamic light scattering and fluorescence probe technique were conducted to determine the physicochemical properties of mPEG-PLA copolymer. PTX-loaded polymeric micelles were characterized regarding their particle size, entrapment efficiency (EE), drug loading (DL), in vitro drug release and hemolysis rate. The complement activation in human serum and mast cells degranulation were performed by ELISA and RBL-2H3 cell line in vitro, respectively. The chronic toxicity study was carried out on beagle dogs. The optimized PTX-loaded mPEG-PLA (40/60) micelles showed a particle size of 37 nm and EE of 98.0% with a DL of 17.0% w/w. Transmission electron microscopy (TEM) analyses showed that mPEG-PLA (40/60) micelles have spherical shape with dense core. In vitro release study showed a sustained release for 24 h, and the hemolysis study revealed that mPEG-PLA (40/60) was a safe nanocarrier for intravenous administration. mPEG-PLA (40/60) showed a lower complement activation ability compared to mPEG-PLA (50/50) and Cremophor® EL (Cr EL). Furthermore, the chronic toxicity of PTX-loaded mPEG-PLA (40/60) micelles was significantly lower than those of mPEG-PLA (50/50) and Cr EL.
Introduction
Paclitaxel (PTX), a natural anticancer agent, originally extracted from the bark of the Pacific yew, Taxus brevifolin, has shown an effective activity against a broad spectrum of solid tumors, such as refractory ovarian cancer, metastatic breast cancer, non-small cell lung cancer and AIDS-related Kaposi's sarcoma (Zhang et al., Citation2005; Li et al., Citation2012). Due to its hydrophobicity, PTX is solubilized in the conventional formulation (Taxol™) by a 50:50 mixture of ethanol and Cremophor® EL (Cr EL, polyethoxylated castor oil), which are used as co-solvents in this intravenous formulation. However, a number of studies have reported that Cr EL induces a number of serious side effects including dyspnea, flushing, rash and urticaria (Szebeni et al., 1998). Being different from IgE-mediated type I allergy, the Cr EL-caused hypersensitivity reactions (HSRs) are recognized as pseudoallergy, which are not initiated by pre-existing IgE antibodies. According to the World Allergy Organization, pseudoallergy, also known as non-immune anaphylaxis, is a reaction similar to immune-allergic reactions and can be observed after the first administration of offending drugs. Unlike common allergies, pseudoallergic reactions could be induced by direct release of histamine from mast cells and activation of the complement system, followed by unusual synthesis of eicosanoids and inhibition of bradykinin degradation.
Furthermore, other side effects such as nephrotoxicity and neurotoxicity have been attributed to the use of Cr EL in the conventional PTX formulation (Gelderblom et al., Citation2002). Therefore, great efforts have been devoted to develop new drug delivery systems for PTX free from the above-mentioned solubilizing agent. These systems include parenteral emulsions, liposomes, micelles, polymeric micro/nanoparticles (NPs) and water-soluble prodrugs (Liggins & Burt, Citation2002).
In this study, PTX-loaded polymeric micelles were prepared using a newly synthesized monomethoxypoly(ethylene glycol)-b-poly(lactic acid) (mPEG-PLA) polymer, which had a quantitative ratio of 40/60. The aim of this study was to investigate the immunosafety of mPEG-PLA (40/60) as a promising carrier. Therefore, the role of mPEG-PLA (40/60) in the activation of the complement system and in the degranulation of mast cells was evaluated in vitro. Furthermore, a chronic toxicity study of PTX-loaded polymeric micelles was also conducted in beagle dogs. In addition, by analyzing drug release profile, hemolysis in vitro, entrapment efficiency (EE) and drug-loading (DL), we here provided a new drug delivery system with hypo-allergenic, improved safety and enhanced antitumor activity.
Materials and methods
Materials and animals
d,l-Lactide was purchased from Daigang Biotechnology company (Ji Nan, China); mPEG2000 was obtained from Fluka (Newport News, VA, USA); pyrene was purchased from Aladdin (Shang Hai, China); stannous octoate was purchased from Sigma-Aldrich (St. Louis, MO, USA); methylene dichloride was obtained from Nanjing Chemical company (Nan Jing, China); anhydrous ether was purchased from Nanjing Chemical company; zymosan A was obtained from Sigma; SC5b-9 ELISA kit was purchased from QUIDEL (San Diego, CA, USA); minimum essential medium (MEM) was purchased from Invitrogen (Carlsbad, CA, USA); HyClone fetal bovine serum was obtained from Thermo (Waltham, MA, USA); Hydroxyethylpiperazine Ethane Sulfonic Acid (HEPES) was obtained from Mbchem (Shang Hai, China); TritonX-100 was purchased from Amrecso (Solon, OH, USA); p-nitrophenyl-2-acetamido-2-deoxy-beta-d-glucopy-ranoside was purchased from Sigma; compound 48/80 was purchased from Sigma; Cr EL was supplied by BASF (Mannheim, Germany); dialysis bag was purchased from Visiking (MWCO: 3500, Turkey); soldium salicylate was obtained from Lingfeng Chemical company (Shang Hai, China); paclitaxel injecta was purchased from Jiangsu Aosaikang Pharmaceutical Co., Ltd. (Nan Jing, China); 0.9% NaCl injecta was obtained from Zhang Jiagang Pharmaceutical factory (Zhang Jiagang, China).
Beagles were purchased from An Li Mo Technology Ltd. (Nan Jing, China). New Zealand rabbits were purchased from Qinglong Mountain farms (Nan Jing, China). All animal experiments were conducted according to the National Institute of Health Guide for the Care and Use of Laboratory Animals and approved by the Animal Ethics Committee of China Pharmaceutical University.
Methods
Synthesis of mPEG-PLA (40/60) block copolymer
mPEG-PLA copolymer was synthesized at quantitative ratio of 40/60 by ring opening polymerization (Zheng et al., Citation2010). In brief, appropriate amount of mPEG 2000 and d,l-lactide were added to a flask. The flask was evacuated under vacuum then purged with N2 stream. The mixture was heated under stirring and was completely melted at 130 °C. Appropriate amount of stannous octoate was added under N2 stream; the flask was sealed and maintained in an oil bath at 140 °C under stirring for 6 h. The synthesized polymer was dissolved in methylene chloride and then precipitated in ice-cooled diethyl ether. The resultant precipitated was filtered and dried under vacuum at room temperature for 24 h. The composition and the number average molecular weight of the synthesized mPEG-PLA were determined in CDCl3 solution by H-NMR at 300 MHz (AVANED AV-300, Bruker, Switzerland).
Critical micelle concentration (CMC)
The critical micelle concentration (CMC) was determined by a fluorescence probe technique using pyrene as a fluorescence probe (Aguiar et al., Citation2003). In brief, an aliquot of 0.2 mL pyrene–acetone solution (4.8 μg/mL) was transferred into a series of volumetric flasks, and the solvent was evaporated to dryness under N2 stream. Then mPEG-PLA block copolymer aqueous solution at different concentrations (0.1 μg/mL to 2000.0 μg/mL) was added to each flask. The samples were equilibrated at 25 °C in a lightproof water bath for 24 h before measurement. The excitation spectrum of pyrene was determined using fluorescence spectrophotometer (RF-5301PC, Shimadzu, Kyoto, Japan). The intensity ratio I338/I333 was calculated and plotted against the logarithm of copolymer mass concentration to estimate CMC.
Preparation and stability study of mPEG-PLA blank micelles
The stability of mPEG-PLA blank micelles in bovine serum albumin (BSA) solution was evaluated in term of particle size change (Lo et al., Citation2007). In brief, mPEG-PLA at 40/60 and 50/50 quantitative ratios were accurately weighed and added into flasks, respectively. Ethylene dichloride was added to dissolve the copolymer. Then the solvent was removed using rotary evaporator under vacuum at 60 °C. Double distilled water was added, and the flask was kept under vacuum for 3 min, and 0.6 g of BSA was accurately weighed was transferred. Tris-HCl buffer solution was added to dissolve the BSA, and the volume was completed to 10 mL. The different micellar solutions prepared were mixed with BSA solution at the ratio of 1:1 (v/v). The particle size was determined by dynamic light scattering using a ZetaPlus laser particle analyzer (Brookhaven Instruments Corporation, Holtsville, NY, USA) immediately after 30 min incubation at 37 °C.
Preparation and characterization of PTX-loaded mPEG-PLA (40/60) polymeric micelles
Preparation of PTX-PM
PTX-loaded mPEG-PLA (40/60) polymeric micelles (PTX-PM) were prepared by a simple film hydration method (Zhang et al., Citation1996; Zhang et al., Citation2005). Briefly, PTX and mPEG-PLA polymer were dissolved in acetonitrile. The solvent was removed at 40 °C, and the obtained polymer/drug film was then dispersed by adding double distilled water under gentle shaking for 5 min. The PTX-PM solution was centrifuged at 3000 rpm for 10 min, and then filtered through 0.22 μm filter to remove the unincorporated drug. Afterwards, the resultant filtrate was lyophilized using a LGJ-12 freeze dryer (Songyuanhuaxing Technology Develop Co., Ltd., Beijing, China).
Encapsulation efficiency and DL of PTX-PM
The lyophilized micelles were dissolved in 10 mL of methanol, and PTX content in micelles was determined by HPLC system (Shimadzu). The mobile phase was a mixture of acetonitrile and water (45:55, v/v) at 0.8 mL/min flow rate. All separations were carried out on a C18 column (150 × 4.6 mm, 3.5 μm, Waters, Milford, MA, USA) maintained at 35 °C with a detection wavelength of 227 nm (Qu et al., Citation2013). The injection volume of the samples was 20 μL. The EE and DL were calculated according to the following equations:
The lyophilized micelles were dissolved in double distilled water. The particle size and the zeta potential of PTX-PM were determined with a ZetaPlus laser particle analyzer (Brookhaven Instruments Corporation). The temperature was kept at 25 °C during measurements.
Transmission electron microscopy
TEM was used to determine the structure and shape of the prepared blank and PTX-loaded polymeric micelles. For this purpose, samples were appropriately diluted with double distilled water, loaded onto the copper grid and allowed to dry in air followed by staining with 2% phosphotungstic acid (Chitkara & Kumar, Citation2013). Then, they were examined using JEM-100 CX II (JEOL®, Tokyo, Japan) at an accelerating voltage of 80 kV.
In vitro release of PTX-PM
The release profiles of PTX from micelles were conducted by a dialysis method (Zhang et al., 2010a). One milliliter of PTX-loaded polymeric micelles containing 0.9 mg of PTX was placed in a dialysis bag (MWCO: 3500), and the end-sealed dialysis bag was immersed in sodium salicylate solution at 37 °C and gently shaken in a water bath at 85 rpm (SHZ-28A temperature-controlled oscillation instrument, Taicang Experimental Company, Suzhou, China). One milliliter sample solution was withdrawn at predetermined time intervals and an equal volume of fresh medium was supplemented. The sample was filtered through 0.22 μm filter, and the amount of PTX released was determined by HPLC as described above. PTX release form the conventional formula (Taxol®) was also conducted as a control.
Hemolysis study
The hemolytic method was performed according to the method described previously (Natsume et al., 1999). Blood was collected from anesthetized New Zealand rabbit by cardiac puncture. Whole blood was centrifuged at 3000 rpm for 10 min, and the isolated red blood cells (RBCs) were washed with 0.9% sodium chloride solution. The RBCs were then suspended in 0.9% sodium chloride solution to obtain a concentration of 2% suspension. Aliquots of 2% RBC suspension with different concentrations of the tested carriers (mPEG-PLA 50/50, mPEG-PLA 40/60 and Cr EL) were incubated at 37 °C for 1 h. The positive and negative controls were water and 0.9% saline, respectively. The samples were then centrifuged at 3000 rpm for 10 min to remove non-lysed RBC. The supernatant was collected and analyzed by microplate reader at 540 nm (BioTek, Seattle, WA, USA). The hemolysis rate was determined by the following formula:
Non-clinical study of immunosafety triggered by mPEG-PLA
In vitro complement activation assay
The complement activation effect of mPEG-PLA copolymer and Cr EL was investigated in vitro by measuring the excipients-induced rise of serum complement activation product SC5b-9 using Quidel's ELISA kits. SC5b-9 is the soluble, S-protein-bound form of the terminal complex, a sensitive measure of C5a formation through both the classical and the alternative pathways. The reaction started after adding the required quantity of test agents (in sterile physiological saline) to undiluted serum (activator to serum volume ratio was 1:4) in Eppendorf tubes. The reaction was performed in triplicates. The tubes were placed in a shaking water bath at 37 °C for 30 min. Zymosan (12.5 mg/mL) was used as a positive control for complement activation. The level of the complement activation products was then measured by the ELISA kits and compared with saline control incubations (Szebeni et al., 2005).
Cell culture
RBL-2H3 cells were maintained in monolayer culture in Eagle's MEM with 15% fetal bovine serum and 1% penicillin/streptomycin under an atmosphere of 5% CO2 at 37 °C.
β-Hexosaminidase release assay
The degranulation of mast cells was determined by measuring β-hexosaminidase release (Naal et al., Citation2004). Confluent RBL-2H3 cells were seeded on 96-well plate. The cells attached to microtiter wells were then washed twice with Tyrode's buffer, and the stimulation was initiated by adding 200 μL of respective test agents in Tyrode's buffer. After 1-h incubation at 37 °C, the degranulation was stopped by placing the plates on ice. To determine the amount of β-hexosaminidase released from the cells, 60 μL of supernatant and 60 μL of β-hexosaminidase substrate solution (5 mmol/mL p-nitrophenyl-2-acetamido-2-deoxy-beta-d-glucopy-ranoside in 0.1 mol/mL sodium acetate buffer, pH 4.5) were mixed in a new 96-well plate and incubated for 1 h at 37 °C. The reaction was quenched by the addition of 150 μL of 100 mmol/L Na2CO3 and 100 mmol/L NaHCO3 buffer (pH 10.7). The negative control group with only Tyrode's buffer was used to assess the spontaneous release. Total β-hexosaminidase amount was obtained by lysing the cells with 0.1% Triton-X 100 prior to the supernatant removal. β-Hexosaminidase in the supernatant was quantified by measuring absorbance at 450 nm using Multiskan Ascent (Thermo). The background absorbance with substrate in buffer alone (no cell supernatant) was subtracted from all readings.
Toxicity test of PTX-PM in Beagles
Preparation of testing samples
The PTX-PM were suspended in physiological saline to make a concentration of 0.5 mg/mL. A solution of commercial formulation having the same concentration was also prepared as a positive control. Physiological saline was considered as the negative control.
Route of administration and organ index
The Beagles were injected in the foreleg at a dose volume of 2 mL/kg once per week for four weeks. During the study, the animal's body weights were recorded. After a recovery phase of two weeks, the animals were sacrificed, and the organs were examined for any pathological changes. In addition, the organ indexes (organ weight/body weight) were also calculated and recorded.
Statistical analysis
Data were expressed as means ± SD from triplicate experiments performed in a parallel manner unless otherwise indicated. The results were analyzed using an unpaired, two-tailed Student's t-test. All comparisons were made relative to corresponding controls, and significance of difference was indicated as p < 0.001, p < 0.01 and p < 0.05.
Results and discussion
Synthesis of mPEG-PLA(40/ 60) block copolymer
mPEG-PLA copolymer was synthesized by ring-opening polymerization and a white solid powder was obtained. The structure of the synthesized polymer was determined by H-NMR, and the spectrum is shown in . The peaks at 3.4 ppm and 3.6 ppm were the proton peaks of the methoxyl and the methylene in mPEG segment, respectively, while the proton peaks of methyl and methine in PLA segment appear at 1.5 ppm and 5.2 ppm, respectively. The H-NMR spectrum suggested that the mPEG-PLA block copolymer was successfully synthesized. Comparing the ratio of peak area at 5.2 and 3.6 ppm, the number average molecular weight of the synthesized copolymer was calculated to be 4488.4.
CMC
The CMC was obtained by fluorescent determination. The results are shown in and . It was found that the CMC value (1.30 μg/mL) of mPEG-PLA (40/60) was significantly lower than that of mPEG-PLA (50/50) (2.4 μg/mL). This lower CMC value could be attributed to higher hydrophobicity induced by a longer PLA segment. Therefore, mPEG-PLA with 40/60 weight ratio might form more stable micelles in aqueous solution and that by reducing their core fluidity. Moreover, the amount of copolymer needed in the preparation could be reduced and thus the adverse reactions trigged by this carrier might be avoided especially pseudo allergic ones.
Table 1. Characteristics of a series of mPEG-PLA blank micelles and PTX-PM.
Particle size and BSA stability of mPEG-PLA blank micelles
The particle size of blank polymer micelles, both in aqueous solution and in BSA, was determined and is listed in . The size of blank mPEG-PLA (50/50) and mPEG-PLA (40/60) micelles exhibited a unimodal distribution with a mean diameter of 44.0 nm and 36.7 nm, respectively. The size of mPEG-PLA (50/50) micelles did not vary significantly from mPEG-PLA (40/60) micelles when the blank micelles were suspended in aqueous solution. However, after incubation, the size of mPEG-PLA (50/50) micelles increased from 44.0 nm to 71.4 nm, while the particle size of mPEG-PLA (40/60) micelles did not significantly change (). This implies that mPEG-PLA (40/60) micelles are relatively more stable in BSA, and thus having a low risk of forming antigen with serum proteins in vivo, which reduces the risk of anaphylaxis.
Characteristics of PTX-PM
The PTX-PM were stored in the freeze-dried form. The particle size, zeta potential, EE and DL of PTX-PM are summarized in and , respectively. The PTX-PM showed a relatively high DL capacity of 17 wt% and an EE of almost 98%. The average diameter of the PTX-PM was around 35 nm, which is slightly smaller than that of the corresponding blank micelles. This might be explained by the hydrophobic interaction between the PLA and the PTX molecules trapped in the micelles core. A bimodal distribution of PTX-PM was observed, as shown in . The size of most of the micelles was distributed near 22 nm; while the size of the rest (about 1%) was distributed at 40–60 nm. This might be caused by the aggregates of micelles. The result of zeta potential, illustrated in , shows that blank micelles and PTX-PM belong to the electric neutrality range. After 12 h, the zeta potential had a slight change, but was still in the range of neutrality.
TEM studies
TEM studies can be used for visualization of particles in nano-size range. The difference in the material density also provides the inputs on its localization in the sample. Thus, TEM images () showed that blank mPEG-PLA (40/60) micelles were spherical and nano-meter sized (about 37 nm); on the other hand, the PTX-PM micelles were spherical or oval and the diameter was about 35 nm. The structure was clearly visible that copolymer packed the PTX in the core of micelle ().
In vitro release of PTX-PM
As shown in , 50% PTX released within the first 4 h. After 24 h, the amount of accumulated drug released was 80%, which is significantly higher than that of commercial preparation. Apparently, PTX release from mPEG-PLA micelles exhibited a biphasic mode with an initial burst release in the first hours followed by a sustained release phase. DDSolver software (Nanjing, China) was used to analyze the release mechanism (Zhang et al., 2010a) and it was found that Probit Model best fit the release data. This was confirmed by the coefficient of correlation R2, which was equal to 0.9915 for the polymer micelles group and equal to 0.9993 for the commercial preparation group.
Hemolysis study
The hemolysis rate of mPEG-PLA was compared with the excipient of commercial formulation Cr EL. As shown in , the groups of Cr EL in 50% ethanol caused a concentration-dependent erythrocytes lysis, while mPEG-PLA caused a minimum lysis even at concentration up to 10%. 20% hemolytic rate was widely accepted as the standard for excipient safety. When the concentration was above 6%, Cr EL in 50% ethanol triggered severe hemolysis (above 20%). As reported by Gill et al., the hemolytic effect could be used to evaluate the membrane damage by carriers (Gill et al., Citation1994). This implies that mPEG-PLA has a lower capability of membrane damage than the Cr EL. The results suggested that the mPEG-PLA 40/60, a new carrier for hydrophobic drugs, is not only hypo-allergic but also hypo-hemolytic.
Non-clinical study of pseudoallergy triggered by mPEG-PLA
Effects of different mPEG-PLA on complement activation in vitro
To evaluate the effect of different mPEG-PLA on complement activation, mPEG-PLA (40/60), mPEG-PLA (50/50) and Cr EL in 50% ethanol (with three gradual concentrations of PTX) were incubated with normal human sera, respectively. shows that all the three carriers tested could induce a significant formation of SC5b-9 in a concentration-dependent manner. Furthermore, the extents of SC5b-9 formation vary in the following order: Cr EL in 50% ethanol > mPEG-PLA (50/50) > mPEG-PLA (40/60). Therefore, it seems that mPEG-PLA (40/60), unlike the conventional vehicle (Cr EL), is a poor activator of the complement system.
Figure 4. (A) Effect of mPEG-PLA (40/60), mPEG-PLA (50/50), PTX-PM and Cr EL on complement activation in vitro. n = 3, ± SD. *p < 0.05, **p < 0.01 versus negative control. #p < 0.05, ##p < 0.01 versus Cr EL in 50% ethanol. (B) Effect of mPEG-PLA (40/60), PTX-PM and Cr EL on β-hexosaminidase releasing of RBL-2H3 cells. n = 3,
± SD. *p < 0.05 versus negative control.
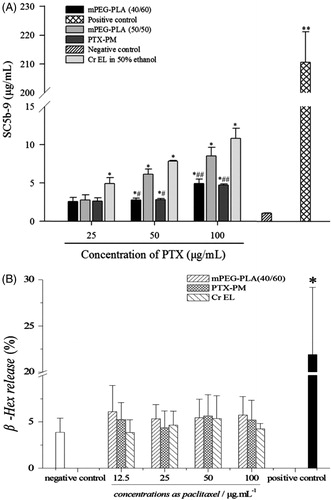
To confirm the above results, the effect of PTX-PM on complement activation was also investigated. As shown in , PTX-PM could cause the complement activation in a concentration-dependent manner. The extent of activation was similar to that of mPEG-PLA (40/60), which was much lower than the conventional vehicle. Combined, these results suggest that the mPEG-PLA (40/60) might have a small effect on the induction of complement activation-related pseudoallergy (CARPA).
Effects of mPEG-PLA (40/60), PTX-PM and Cr EL on RBL-2H3 cells in releasing β-Hexosaminidase
β-Hexosaminidase release assay was conducted to investigate whether mPEG-PLA (40/60) and PTX-PM could induce pseudoallergic reactions through a direct release of histamine from mast cells. As shown in . The raise of mPEG-PLA (40/60), PTX-PM and Cr EL concentrations did not significantly increase β-hexosaminidase release rates compared with negative control.
The β-hexosaminidase release rates, induced by mPEG-PLA and Cr EL, were comparable to positive control, thus, mPEG-PLA and Cr EL were not able to directly stimulate mast cells degranulation. According to our previous results, PTX-PM induced lower complement activation as compared with Cr EL. These findings imply that the new nanocarrier mPEG-PLA (40/60) might have a lower allergenicity, and thus, it is likely safer than mPEG-PLA (50/50) and Cr EL.
Chronic toxicity test of PTX-PM in Beagles
The weights and organ indexes
The data of weights from different groups are listed in . The decrease of the body weight, compared with that of negative control group, was recorded for all the groups. Furthermore, the change in the body weight was not significantly different between the PTX-PM treated groups during the period of study.
Table 2. The body weight (
± SD, n = 6 kg).
The organs, including liver, lung, kidney, thymus, brain, ovary and testis were carefully examined. The organ-body weight indices are listed in . After four weeks, no significant change was observed in the organs of the negative control group. However, the liver and kidney indexes were higher in the positive control group than those in the negative one, while the thymus organ-body weight index was lower. In the group treated with mPEG-PLA (40/60) micelles, there was no sign of pathological changes except the lung congestion. The liver index was higher and the thymus index was lower in mPEG-PLA (50/50) group compared with the corresponding organs in the control group. In addition, pylorus and small intestine congestion were also observed. During the recovery phase, no abnormal changes of vital organs and no delayed type toxicities were observed.
Table 3. Important organ index (
± SD, g/kg).
Discussion
Polymeric micelles are widely employed as carriers for hydrophobic drugs, since they can increase drug solubility and stability, improve the therapeutic index, prevent drug from biological degradation and inactivation and reduce the toxicity (Szebeni, Citation2005). Herein, mPEG-PLA copolymer with the quantitative ratio of 40/60 was successfully synthesized by ring opening polymerization. The CMC values of polymers were lower compared with typical surfactants (Christiansen et al., 2010). The higher the hydrophobic interaction, the lower the CMC will be, indeed, the CMC value of mPEG-PLA (40/60) was lower than that of mPEG-PLA (50/50), and this is due to the PLA segment, which is much longer in mPEG-PLA (40/60). Furthermore, the low CMC value indicates better micelles stability upon dilution in plasma. In fact, mPEG-PLA (40/60) micelles exhibited a higher stability in bovine serum (BSA) compared with mPEG-PLA (50/50) micelles (Wei et al., Citation2012), which had a larger particle size after incubation. Moreover, PTX-loaded mPEG-PLA micelles are very small with a mean diameter around 35 nm. However, their size was slightly smaller than that of mPEG-PLA blank micelles, and that could be explained by stable core fluidity induced by the hydrophobic interaction between PLA and PTX molecules (Gupta et al., 2012). Furthermore, because of their nano-size, mPEG-PLA micelles are able to escape the mononuclear phagocyte system, thus extending their circulation time in the blood, which improve the tumor target ability in vivo. The release of PTX from mPEG-PLA micelles exhibited a biphasic profile, with a burst release followed by a sustained release phase. This progressional release could be explained by hydrolysis of either ester bonds formed between PLA and PEG segments or those of the PLA segment generating lactic acid monomers and/or oligomers. Therefore, the release kinetic of PTX from mPEG-PLA micelles could be adjusted by varying the PEG /PLA blend ratio.
Recently, numerous drug-induced HSRs have been reported. Different from IgE-mediated allergy, these HSR, also called pseudoallergies, are caused by the activation of the complement system. Hence, they have been discerned within the type I category of allergy as “CARPA” (Szebeni et al., 2012). Micellar solvents containing amphiphilic lipids (such as Cr EL) are among the agents that might cause CARPA. Therefore, this study was carried out to investigate the ability of mPEG-PLA, as alternative to Cr EL for PTX delivery, to activate the complement system inducing pseudoallergy. As a method proposed by Janos et al. in Citation1998, in vitro complement activation assay is widely adopted as predictor of clinical reactions. Typically, Serum SC5b-9 is analyzed as a terminal product of complement activation. However, other markers such as C3a-desArg, Bb and C4d can be measured for further investigation of the activation mechanisms. In this study, we proved that Cr EL is responsible for the induction of pseudoallergy through complement activation. Furthermore, the results also showed that mPEG-PLA might activate the complement system in vitro, and thus, induce pseudoallergic reactions. However, mPEG-PLA (40/60) is a poor activator compared with Cr EL. Therefore, further studies on PTX-PM prepared by mPEG-PLA (40/60) have been conducted. The results showed that the ability of these micelles to activate the complement is similar to that of mPEG-PLA (40/60), which proves that these pseudoallergies might be induced by mPEG-PLA (40/60) rather than PTX.
Moreover, we carried out β-hexosaminidase assay to investigate whether mPEG-PLA (40/60) and PTX-TM could stimulate mast cells degranulation. As the results were negative, we postulated that mPEG-PLA (40/60) and PTX-PM were unable to induce pseudoallergy through direct histamine release. These results were consistent with those of toxicity studies carried out on Beagles. Therefore, considering the above findings, we assume that mPEG-PLA induce slight pseudoallergic reactions, which are much lower compared with those of Cr EL, by the activation of the complement system rather than mast cell degranulation. Our results were in accordance with previous published studies. Shan et al. have pointed out that the polymeric NPs modified by PEG own a lower ability of activating complement system (Shan et al., Citation2009). In fact, PEG chains binding to hydrophobic poly (ε-caprolactone) backbone might prevent the NPs from activating the complement system. However, because of differences in the extent of activation between mPEG-PLA (40/60) and (50/50), we postulated that the ratio of the PEG is also an important factor and should be adjusted to have the appropriate extent.
Conclusion
In this study, we successfully testified the hypo-toxicity of mPEG-PLA and low risk to trigger pseudoallergic reactions. mPEG-PLA with 40/60 quantitative ratio was synthesized by ring opening polymerization. The nanomicelles exhibited a low CMC value, a small particle size and an enhanced release profile. Both EE and DL of PTX-PM were relatively higher. Compared to Cr EL, mPEG-PLA (40/60) showed a lower ability to activate the complement system, mast cells degranulation and acceptable hemolysis rate, and this was also confirmed by the conducted Beagles toxicity study. Meanwhile, it was demonstrated that the block copolymer with different blend ratios (50/50 and 40/60) will lead to individual fate of immunosafety. It was postulated that mPEG-PLA induces pseudoallergy by activating the complement system rather than stimulating mast cells degranulation. Therefore, mPEG-PLA could be useful as a biodegradable and safe nanocarrier for intravenous delivery of antitumor drugs.
Correction Statement
This article has been republished with minor changes. These changes do not impact the academic content of the article.
Declaration of interest
This study was supported by National Natural Science Foundation of China (no. 81201182), the Fundamental Research Funds for the Central Universities (Program no. ZJ13056) and the Doctoral Fund of Youth Scholars of Ministry of Education of China (20130096120003).
References
- Aguiar J, Carpena P, Molina-Bolivar JA, et al. (2003). On the determination of the critical micelle concentration by the pyrene 1: 3 ratio method. J Colloid Interface Sci 258:116–22
- Chitkara D, Kumar N. (2013). BSA-PLGA-based core-shell nanoparticles as carrier system for water-soluble drugs. Pharm Res 30:2396–409
- Gelderblom H, Loos WJ, Verweij J, et al. (2002). Modulation of cisplatin pharmacodynamics by Cremophor EL: experimental and clinical studies. Eur J Cancer 38:205–13
- Gill IJ, Illum L, Farraj N, et al. (1994). Cyclodextrins as protection agents against enhancer damage in nasal delivery systems II. Effect on in vivo absorption of insulin and histopathology of nasal membrane. Eur J Pharm Sci 1:237–48
- Janos S, Franco MM, Carl RA. (1998). Complement activation by Cremophor EL as a possible contributor to hypersensitivity to paclitaxel: an in vitro study. J Natl Cancer Inst 90:300–6
- Li L, Kim JK, Huh KM, et al. (2012). Targeted delivery of paclitaxel using folate-conjugated heparin-poly (β-benzyl-l-aspartate) self-assembled nanoparticles. CarbohydrPolym 87:2120–8
- Liggins RT, Burt HM. (2002). Polyether–polyester diblock copolymers for the preparation of paclitaxel loaded polymeric micelle formulations. Adv Drug Deliv Rev 54:191–202
- Lo CL, Huang CK, Lin KM, et al. (2007). Mixed micelles formed from graft and diblock copolymers for application in intracellular drug delivery. Biomaterials 28:1225–35
- Naal RM, Tabb J, Holowka D, Baird B. (2004). In situ measurement of degranulation as a biosensor based on RBL-2H3 mast cells. Biosens Bioelectron 20:791–6
- Qu D, Lin HJ, Zhang N, et al. (2013). In vitro evaluation on novel modified chitosan for targeted antitumor drug delivery. Carbohydr Polym 92:545–54
- Shan XQ, Yuan Y, Liu CS, et al. (2009). Influence of PEG chain on the complement activation suppression and longevity in vivo prolongation of the PCL biomedical nanoparticles. Biomedmicrodevices 11:1187–94
- Szebeni J. (2005). Complement activation-related pseudoallergycaused by amphiphilicdrug carriers: the role of lipoproteins. Curr Drug Deliv 2:443–9
- Wei Y, Wang YX, Wang W, et al. (2012). Microcosmic mechanisms for protein incomplete release and stability of various amphiphilic mPEG-PLA microspheres. Lxsangmuir 28:13984–92
- Zhang XC, Jackson JK, Burt HM. (1996). Development of amphiphilic diblock copolymers as micellar carriers of taxol. Int J Pharm 132:195–206
- Zhang XF, Li YX, Chen XS, et al. (2005). Synthesis and characterization of the paclitaxel/MPEG-PLA block copolymer conjugate. Biomaterials 26:2121–8
- Zheng XL, Kan B, Gou ML, et al. (2010). Preparation of MPEG–PLA nanoparticle for honokiol deliveryin vitro. Int J Pharm 386:262–7
- Zhang Y, Huo MR, Zhou JP, et al. (2010). DDSolver: an add-in program for modeling and comparison of drug dissolution profiles. AAPS J 12:263–71
- Zhang ZS, Zhang QB, Wang J, et al. (2010). Synthesis and drug releasein vitroof porphyran carrying 5-Fluorouracil. Carbohydr Polym 79:628–32