Abstract
The aim of this study was to evaluate the effects of therapeutic pulsed ultrasound with gold nanoparticles on oxidative stress parameters after traumatic muscle injury in Wistar rats. The animals were randomly divided into nine groups (n = 6 each): sham (uninjured muscle); muscle injury without treatment; muscle injury and treatment with dimethyl sulfoxide (15 mg/kg); muscle injury and treatment with gold nanoparticles (27 µg); muscle injury and treatment with dimethyl sulfoxide + gold nanoparticles (Plus); muscle injury and therapeutic pulsed ultrasound; muscle injury and therapeutic pulsed ultrasound + dimethyl sulfoxide; muscle injury and therapeutic pulsed ultrasound + gold nanoparticles; and muscle injury and therapeutic pulsed ultrasound + Plus. Gastrocnemius injury was induced by a single-impact blunt trauma. Therapeutic pulsed ultrasound (6-min application, frequency 1.0 MHz, intensity 0.8 W/cm2) was used 2, 12, 24, and 48 h after trauma. Mitochondrial superoxide generation, lipid peroxidation, and protein carbonylation, and the activities of superoxide dismutase, glutathione peroxidase, and catalase were evaluated. The increase in the superoxide production and TBARS and carbonyl levels observed in the control group after muscle damage were reduced in animals exposed to therapeutic pulsed ultrasound plus nanoparticles. Similarly, antioxidants enzymes showed a decreased activity with the same treatment. Our work suggest that therapeutic pulsed ultrasound + dimethyl sulfoxide + gold nanoparticles has beneficial effects on the muscle healing process by inducing a decrease in oxidative stress parameters and most likely decreasing the deleterious effects of the inflammatory response.
Introduction
The healing of the skeletal muscle in response to trauma depends on the type of injury, such as contusion, strain, or laceration, and on its severity. However, in general, the healing process consists of three phases: the destruction phase, the repair phase, and the remodeling phase. The destruction phase is characterized by necrosis, hematoma formation, and an influx of inflammatory cells (Ten Broek et al., Citation2010).
Injury induces the increased generation of reactive oxygen species (ROS) in the skeletal muscle, which alters the intracellular oxidant–antioxidant balance in favor of injury and may result in oxidative damage to the traumatized muscle when the production of ROS overwhelms the antioxidant defense systems (Saborido et al., Citation2011). Multiple potential sites for ROS generation in skeletal muscle have been identified, including mitochondria, NADPH oxidase enzymes, phospholipase A2-dependent processes, and xanthine oxidase (Jackson, Citation2009).
In this context, therapeutic pulsed ultrasound (TPU) is commonly used in the rehabilitation setting to elicit thermal or nonthermal physiologic effects (Johns, Citation2002; Markert et al., Citation2005). TPU is a noninvasive form of mechanical energy transmitted transcutaneously as high-frequency acoustic pressure waves into biological tissues (Lu et al., Citation2009). The beneficial effects of TPU on a variety of connective tissues and related cellular and molecular mechanisms have been assessed biomechanically (Piedade et al., Citation2008; Alfredo et al., Citation2009; Freitas et al., Citation2010).
At the cell level, it has been hypothesized that changes in diffusion rates and membrane permeability to ions due to acoustic streaming and stable cavitation can stimulate cells through the upregulation of signaling molecules (Yang et al., Citation2008; Maruani et al., Citation2010).
According to the hypothesis that TPU affects membrane permeability, it could be used together with anti-inflammatory or antioxidant drugs to promote greater absorption and enhance their effects. Using phonophoresis, it is possible to introduce convenient therapeutic concentrations of the drug transdermally into tissues (Goraj-Szczypiorowska et al., Citation2006).
Gold nanoparticles (GNPs), also called nanogold, have been actively investigated in a wide variety of biomedical applications due to their biocompatibility and facile conjugation with biomolecules (Lévy et al., Citation2004; Sokolov et al., Citation2004). Gold compounds have received great attention as anti-inflammatory agents due to their ability to inhibit the expression of NF-κB and the subsequent inflammatory reactions (Jeon et al., Citation2000; Norton, Citation2008). According to Tsai et al. (Citation2007), nanogold decreases pro-inflammatory cytokines and macrophage infiltration in a model of arthritis. Barathmanikanth et al. (Citation2010) have also shown that GNPs are anti-oxidative agents that inhibit the formation of ROS and scavenge free radicals, thus assisting anti-oxidant defense enzymes.
In previous studies, our group has used extensively the phonophoresis to facilitate the transport of nanoparticles into the injured tissue. Ours results have shown that 5 and 7 days after the muscle injury the anti-inflammatory and anti-oxidants effects are potentiated from application of nanoparticles plus ultrasound. (Freitas et al., Citation2007, Citation2010; Engelmann et al., Citation2012; Silveira et al., Citation2010, Citation2012; Victor et al., Citation2012). Therefore, considering such results, we decided to evaluate the effects of this therapy on oxidative stress parameters at an earlier stage of the inflammatory response (48 h), when the production of ROS is greater.
Materials and methods
Synthesis and characterization of GNs
GNs were prepared as described elsewhere (Turkevich et al., Citation1951; Victor et al., Citation2012). Sodium citrate (Sigma-Aldrich Chemical Co., St. Louis, MO) and hydrogen tetrachloroaurate (HAuCl4) solution (Sigma-Aldrich Chemical Co., St. Louis, MO) were used without further purification. The electronic spectrum was observed with a Shimadzu model UV-1800 spectrophotometer (Shimadzu Corp., Kyoto, Japan). The X-ray diffraction analysis was performed with a Shimadzu LAB-X model XDR-6000 diffractometer with Cu Kα radiation (λ = 1.54056 Å, 30 kV, 30 mA) (Shimadzu Corp., Kyoto, Japan). The scan rate was 2°/min from 20 to 80°. Transmission electron microscopy (TEM) and field-emission scanning electron microscopy (SEM-FEG) measurements were performed with a Hitachi H-9000-NA (Chiyoda-ku, Japan) and a JEOL JSM-7401F (Akishima, Japan) device, respectively. The Au concentration in the GNP solution was determined by atomic absorption analysis (Sonavane et al., Citation2008; Singh et al., Citation2009; Rocha et al., Citation2011) with a VARIAN model AA 240Z atomic absorption spectrometer (Varian Medical Systems, Inc., Palo Alto, CA). The Zeta potential was measured at 25 °C in a Zeta Potential Analyzer (ZetaPALS; Brookhaven Instruments, Holtsville, NY).
Animals
Male Wistar rats (250–300 g) were obtained from the Central Animal House of the Universidade do Extremo Sul Catarinense, Santa Catarina, Brazil, caged in groups of six, offered commercial rat chow and water ad libitum, and maintained under 12-h light/dark cycles. The animals were randomly divided into nine groups (n = 6): sham (uninjured muscle); muscle injury without treatment; muscle injury and treatment with dimethyl sulfoxide (DMSO) [15 mg/kg; adapted from Koksal et al. (Citation2003)] mixed in ultrasound gel; muscle injury and treatment with GNPs [27 µg; adapted from Tsai et al. (Citation2007)] mixed in ultrasound gel; muscle injury and treatment with DMSO + GNPs (Plus) mixed in ultrasound gel; muscle injury and TPU (0.8 W/cm2) + ultrasound gel; muscle injury and TPU (0.8 W/cm2) + DMSO gel (15 mg/kg); muscle injury and TPU (0.8 W/cm2) + GNP gel (27 µg); and muscle injury and TPU (0.8 W/cm2) + Plus gel. All studies were performed in accordance with the guidelines of the National Institutes of Health and with the approval of the Ethics Committee on Animal Use of the Universidade do Extremo Sul Catarinense, Santa Catarina, Brazil.
Muscle injury model
Animals were anesthetized by the intraperitoneal injection of ketamine (70 mg/kg) and xylazine (15 mg/kg). Gastrocnemius injury was induced by a single-impact blunt trauma in a press developed by the Centro Industrial de Equipamentos de Ensino e Pesquisa (CIDEP, Porto Alegre, Rio Grande do Sul, Brazil). Briefly, injury was produced by a metal mass (0.459 kg) falling through a metal guide from a height of 18 cm. The impact kinetic energy delivered was 0.811 J. Sham rats were also anesthetized to ensure standardization but did not undergo muscle trauma (Rizzi et al., Citation2006).
Treatment
Treatment with therapeutic pulsed ultrasound [IBRAMED, Amparo, São Paulo, Brazil; 6-min duration, frequency of 1.0 MHz, intensity of 0.8 W/cm2, effective radiating area (ERA) 1 cm2, 50% duty cycle of 1:2 (5 ms on, 5 ms off) and focused geometry of the ultrasound beam] was used 2, 12, 24 and 48 h after muscle trauma [adapted from Silveira et al. (Citation2010)]. The ultrasound-treated area was ∼2 cm2 (Freitas et al., Citation2007). The movement of the beam was circular. The parameters used for the TPU were established in previous studies by our group (Freitas et al., Citation2007, Citation2010; Silveira et al., Citation2010). The groups with muscle injury and treatment with DMSO, GNPs and Plus were also exposed to circular beam movement for 6 min with the ultrasound in off mode (Saliba et al., Citation2007).
Sacrifice protocol
Two hours after the last application, the animals were euthanized by decapitation and the injured region of the gastrocnemius muscle was surgically removed and immediately processed, aliquoted and stored at −70 °C for subsequent analysis.
Sample preparation
Samples were prepared as previously reported (Silveira et al., Citation2010). Briefly, the injured region of the gastrocnemius was homogenized in the buffer solution, the homogenates were centrifuged at 1000 g for 10 min at 4 °C, and the supernatants were kept at −70 °C until use in the experiments. All biochemical analyses were performed within 5 days.
Biochemical assays
Measurement of mitochondrial superoxide generation
Submitochondrial particles were isolated by differential centrifugation as previously described (Poderoso et al., Citation1996). Superoxide anion production was estimated by measuring adrenaline oxidation in a buffer containing submitochondrial particles, succinate (electron transfer chain initiator), and catalase (CAT). The results were expressed in nanomoles per minute per milligram of protein.
Lipid peroxidation
The formation of thiobarbituric acid reactive substances (TBARS) during a thiobarbituric acid heating reaction was used as an index of lipid peroxidation (Draper & Hadley, Citation1989). Briefly, the samples were mixed with 1 mL of 10% trichloroacetic acid and 1 mL of 0.67% thiobarbituric acid. Subsequently, they were heated in a boiling water bath for 30 min. The level of TBARS was determined by absorbance at 532 nm using 1,1,3,3-tetramethoxypropane as an external standard. The results are expressed as nanomoles per milligram of protein.
Protein carbonylation
Oxidative damage to proteins was assessed by measuring carbonyl groups based on the reaction with dinitrophenylhydrazine (DNPH) (Levine et al., Citation1990). Proteins were precipitated by adding 20% trichloroacetic acid and incubating with DNPH. The samples were then redissolved in 6 M guanidine hydrochloride and carbonylation was determined by the absorbance at 370 nm using a molar absorption coefficient of 22.000 M−1. Total protein was determined from the absorbance at 270 nm in the same sample. The results are expressed as nanomoles per milligram of protein.
Superoxide dismutase activity
Superoxide dismutase (SOD) activity was assayed by measuring the inhibition of adrenaline auto-oxidation using the absorbance at 480 nm (Bannister & Calabrese, Citation1987). The results are expressed in units of SOD activity per milligram of protein.
Glutathione peroxidase assay
Glutathione peroxidase (GPx) activity was measured by using tert-butyl hydroperoxide as the substrate (Wendel, Citation1981). Enzyme activity was measured by monitoring NADPH disappearance at λmax = 340 nm in 50 mM potassium phosphate buffer, pH 7.0, containing 1.0 mM EDTA, 2.0 mM GSH, 0.2 U/mL GSH reductase, 1.0 mM azide, 0.2 mM tert-butyl hydroperoxide, 0.2 mM NADPH, and supernatant containing 0.2–0.3 mg protein mL−1. GPx activity is expressed as nanomoles of NADPH oxidized per minute per milligram of protein, using an extinction coefficient of 6.22 × 106 for NADPH.
CAT activity
CAT activity was measured by the rate of decrease in hydrogen peroxide absorbance at λmax = 240 nm. The results are expressed in units of CAT activity per milligram of protein (Aebi, 1984).
Protein determination
The amounts of protein in the samples that were tested for TBARS, protein carbonylation and enzyme activities were determined using the Lowry technique (Lowry et al., Citation1951).
Statistical analysis
Data were analyzed by one-way analysis of variance followed by Tukey’s test when the p values were significant (p < 0.050). All analyses were performed using the Statistical Package for the Social Sciences (SPSS, version 17.0; IBM Corp., Armonk, NY) software.
Results
Characterization of GNs
In aqueous solution, the electronic spectrum showed a surface plasmon resonance (SPR) band with λmax at 520 nm, typical of spherical GNs. The mean particle diameter of 25 nm was calculated with Scherer’s equation (Suryanarayana & Norton, Citation1998) from the X-ray spectrum of the GNs. The micrography images from TEM corroborated with the value calculated from X-ray diffraction. A concentration of 36 mg L−1 solution was obtained by atomic absorption with theoretical value of 40 mg L−1 (Sonavane et al., Citation2008). The zeta potential revealed a value of −30 mV, indicating the stability of the GNs.
Superoxide anion production
In , the group of rats with muscle injury and no treatment had a significant increase in superoxide compared to the sham group; only the TPU + Plus gel significantly inhibited superoxide production compared to the untreated group [F(8,34) = 2.32, p < 0.05].
Figure 1. Effect of TPU + Plus gel on superoxide anion production in skeletal muscle after injury (48 h). Data are expressed as the means ± standard error of mean for six animals. *p < 0.05 compared to sham, #p < 0.05 compared to muscle injury without treatment, &p < 0.05 compared to TPU + Plus (Tukey’s test).
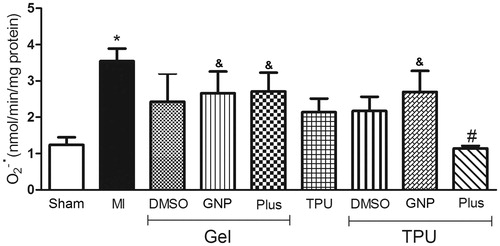
Lipid peroxidation and protein carbonylation
The levels of lipid peroxidation () in the rats with muscle injury and no treatment were significantly increased compared to the sham group. The TPU + DMSO gel and TPU + Plus gel groups similarly and significantly inhibited peroxidation compared to the muscle injury without treatment group [F(8,33) = 3.82, p < 0.05].
Figure 2. Effect of TPU + Plus gel on TBARS (A) and protein carbonylation (B) levels in skeletal muscle after injury (48 h). Data are expressed as the means ± standard error of mean for six animals. *p < 0.05 compared to sham, #p < 0.05 compared to muscle injury without treatment, &p < 0.05 compared to TPU + Plus (Tukey’s test).
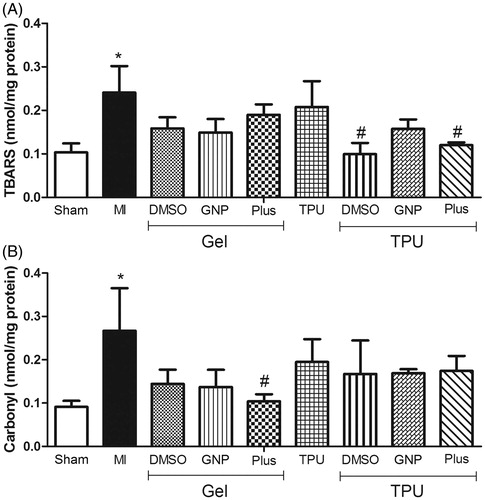
Protein carbonylation () was also significantly increased in the muscle injury without treatment group when compared to the sham group. Only the Plus gel group showed significantly decreased carbonylation compared to the untreated group [F(8,32) = 2.11, p < 0.05].
SOD, GPx, and CAT activities
In , a significant increase in the activity of the three antioxidant enzymes was observed in the muscle injury without treatment group. Only the TPU + Plus group significantly inhibited the increased activities of all three enzymes SOD [F(8,30) = 2.30, p < 0.05], GPx [F(8,34) = 2.05, p < 0.05], and CAT [F(8,34) = 2.00, p < 0.05].
Figure 3. Effect of TPU + Plus gel on SOD (A), GPx (B), and CAT (C) levels in skeletal muscle after injury (48 h). Data are expressed as the means ± standard error of mean for six animals. *p < 0.05 compared to sham, #p < 0.05 compared to muscle injury without treatment, &p < 0.05 compared to TPU + Plus (Tukey’s test).
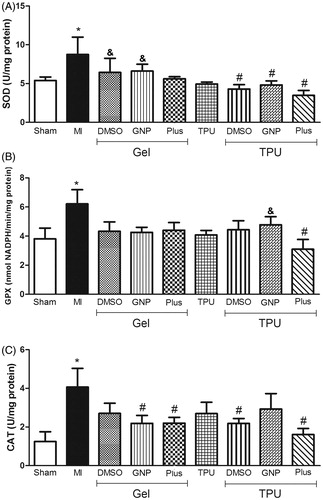
Discussion
The aim of this study was to evaluate the effects of TPU with DMSO and GNPs on oxidative stress parameters 48 h after traumatic muscle injury.
Studies conducted over the past 15 years show that ROS (superoxide, hydroxyl radicals, nitric oxide, peroxynitrite, and the free radical-derived product hydrogen peroxide) play an important role in inflammation and or infection-induced alterations in muscle function (Supinski & Callahan, Citation1985; Tidball, Citation2005; Brunelli & Rovere-Querini, Citation2008; Ciciliot & Schiaffino, Citation2010). ROS are crucial to protect cells against the invasion of infectious agents; however, when ROS production is excessive, biomolecules may be damaged, and the recovery process of the injured tissue could be hindered (Tang et al., Citation2002; Kerkweg et al., Citation2007).
The production of superoxide anion at the site of the lesion and its inhibition by the TPU + Plus gel group were evaluated and compared to the untreated injured group. The results show that only the combination of TPU + DMSO and GNP was effective in decreasing this parameter (). Low-frequency ultrasound is efficient in enhancing the cutaneous penetration of topical drugs into human skin. The explanation for increased transdermal permeability due to low-frequency ultrasound is linked to the thermal effects and is mainly attributed to the cavitation generated by the high vibrational frequency. Because of this cavitation, there is a micromassage effect that increases membrane permeability and protein synthesis (Tezel et al., Citation2002; Maruani et al., Citation2010).
DMSO is commonly used in studies of skeletal muscle as a selective antioxidant or as a solvent for numerous drugs. DMSO has a remarkable and very specific effect on myosin by inhibiting ATPase activity and positively influencing actin, Ca2+ and regulatory proteins (Reid & Moody, Citation1985; Mariano et al., Citation2001). According to Camici et al. (Citation2006), DMSO is also used as a solvent for chemotherapeutic drugs, and due to its anti-inflammatory properties, it has been successfully used in humans for treating rheumatic, pulmonary, gastrointestinal, muscular, neurologic, urinary and dermatologic disorders. Thus, the effects of DMSO can be enhanced when used with TPU.
Recent advances in gold technology have led to probes with improved properties and performance for cell biologists, including increased labeling density, better sensitivity, and greater penetration into tissues. Factors such as shape, size, surface coating and charge affect the probes’ physicochemical properties and are therefore expected to influence interactions with biologic systems. GNPs have an anti-inflammatory effect by decreasing pro-inflammatory cytokine levels and macrophage infiltration in a model of arthritis (Tsai et al., Citation2007). It is likely that the increase in membrane permeability induced by TPU increased the absorption of GNPs and led to the reduction of superoxide anion levels as noted in our results.
To demonstrate that phonophoresis with GNP + DMSO was effective in reducing oxidative stress after muscle injury, oxidative damage and antioxidant enzyme activities were evaluated. The levels of lipid peroxidation were significantly decreased in the TPU + DMSO and TPU + Plus groups; however, decreased protein carbonylation was only observed in the group that received DMSO + GNP (). The TPU + Plus group showed a significant decrease in the activity of SOD, GPx, and CAT (). However, we can also see in that all groups that received treatment with phonophoresis had a decrease in the activity of SOD, showing that this type of therapy has a greater effect in decreasing ROS production and the consequent effects.
Although TPU alone accelerates the recovery of muscle injuries, these effects can be improved with the combined use of pharmacologic agents, as reported previously by our group (Freitas et al., Citation2007). Thus, the combined use of ultrasound and anti-inflammatory agents such as DMSO and GNPs represents an important alternative in the treatment of muscle injuries (Rocha et al., Citation2011). DMSO stabilizes cell membranes from excess free radical formation and abnormal Ca2+ entry into cells, while improving muscle healing. DMSO administration during reoxygenation resulted in a significant increase in ATP associated with markedly lower lactate levels and good muscle recovery (Gilboe et al., Citation1991). Thus, an increase in ATP combined with reduced energy consumption may reflect favorable changes in the balance of energy supply and demand during ischemic/hypoxic events such as in traumatic injury (Jacob & de la Torre, Citation2009).
GNs have received great attention as anti-inflammatory agents due to their ability to inhibit the expression of NF-κB and subsequent inflammatory reactions (Jeon et al., Citation2003; Norton, Citation2008) GNPs block NF-κB activation by interacting with cys-179 of IKK-β and inhibiting the production of proinflammatory cytokines, such as TNF-α and IL-1β (Jeon et al., Citation2000, Citation2003). Another study showed that GNPs inhibited lipopolysaccharide (LPS)-induced NO production and inducible nitric oxide synthase (iNOS) expression in RAW264.7 cells. Furthermore, GNPs suppressed the LPS-induced activation of NF-κB through the inhibition of Akt activity. GNPs also inhibited the LPS-induced phosphorylation of signal transducer and activator of transcription-1 via the down-regulation of interferon-β expression (Ma et al., Citation2010).
Moreover, GNPs are effective in quenching ROS in a dose-dependent manner (Kajita et al., Citation2007). GNPs catalyzed the oxidation of NADH to NAD, enhanced the antioxidant activity of vitamin E and decreased ROS induced in a hepatoma cell line (Nie et al., Citation2007; Martín et al., Citation2010) and inhibited osteoclast formation induced by the receptor activator of NF-κB ligand in bone marrow-derived macrophages (Sul et al., Citation2010). In another wound healing model, GNP decreased CD68 expression and increased SOD1 expression around the wound area, suggesting anti-inflammatory and antioxidative effects.
The TPU has proved effective and safe in treatment of muscle and tendon injuries in humans. Colloidal gold and some of the compounds have recognized therapeutic properties, especially for the treatment of inflammatory and arthritic processes (Kim et al., Citation2007). Several studies performed in our group using phonophoresis and/or iontophoresis with topic application of GNP for treating muscle and tendon injuries in rats show promise and indicate the possibility of use this new therapy in the treatment of these diseases in humans (Freitas et al., Citation2007, Citation2010; Silveira et al., Citation2010, Citation2012; Dohnert et al., Citation2012; Engelmann et al., Citation2012; Victor et al., Citation2012).
Conclusion
Considering results of this study and the previous results from our group, we suggest that TPU + DMSO + GNP has beneficial effects on the muscle healing process by inducing a decrease in oxidative stress parameters and most likely decreasing the deleterious effects of the inflammatory response. Further studies in vivo and in vitro are being conducted in our laboratory to better understand the phonophoresis properties of GNP transdermal permeation in muscle tissue and the mechanisms involved in the antioxidant and anti-inflammatory effects.
Acknowledgements
The authors would also like to thank CNPq and UNESC for fellowship support.
Declaration of interest
The authors report no conflicts of interest. The authors alone are responsible for the content and writing of this article. This work was supported by grants from the Conselho Nacional de Pesquisa e Desenvolvimento (CNPq) and CAPES.
References
- Aebi H. (1984). Catalase in vitro. Methods Enzymol 105:121–6
- Alfredo PP Anaruma CA, Pião AC, et al. (2009). Effects of phonophoresis with Arnica montana onto acute inflammatory process in rat skeletal muscles: an experimental study. Ultrasonics 49:466–71
- Bannister JV, Calabrese L. (1987). Assays for SOD. Methods Biochem Anal 32:279–312
- Barathmanikanth S, Kalishwaralal K, Sriram M, et al. (2010). Anti-oxidant effect of gold nanoparticles restrains hyperglycemic conditions in diabetic mice. J Nanobiotechnol 8:1–16
- Brunelli S, Rovere-Querini P. (2008). The immune system and the repair of skeletal muscle. Pharmacol Res 58:117–21
- Camici GG, Steffel J, Akhmedov A, et al. (2006). Dimethyl sulfoxide inhibits tissue factor expression, thrombus formation, and vascular smooth muscle cell activation: a potential treatment strategy for drug-eluting stents. J Am Heart Assoc 114:1512–21
- Ciciliot S, Schiaffino S. (2010). Regeneration of mammalian skeletal muscle. Basic mechanisms and clinical implications. Curr Pharm Des 16:906–14
- Dohnert MB, Venancio M, Possato JC, et al. (2012). Gold nanoparticles and diclofenac diethylammonium administered by iontophoresis reduce inflammatory cytokines expression in Achilles tendinitis. Int J Nanomedicine 7:1651–7
- Draper H, Hadley M. (1989). Malondialdehyde determination as index of lipid peroxidation. Methods Enzymol 186:421–31
- Engelmann J, Vitto MF, Cesconetto PA, et al. (2012). Pulsed ultrasound and dimethylsulfoxide gel treatment reduces the expression of pro-inflammatory molecules in an animal model of muscle injury. Ultrasound Med Biol 38:1470–5
- Freitas LS, Freitas TP, Silveira PC, et al. (2007). Effect of therapeutic pulsed ultrasound on parameters of oxidative stress in skeletal muscle after injury. Cell Biol Int 31:482–8
- Freitas TP, Gomes M, Fraga DB, et al. (2010). Effect of therapeutic pulsed ultrasound on lipoperoxidation and fibrogenesis in an animal model of wound healing. J Surg Res 161:168–71
- Gilboe D, Kintner D, Fitzpatrick JH, et al. (1991). Recovery of postischemic brain metabolism and function following treatment with a free radical scavenger and platelet-activating factor antagonists. J Neurochem 56:311–9
- Goraj-Szczypiorowska B, Zajac L, Skalska-Izdebska R. (2006). Evaluation of factors influencing the quality and efficacy of ultrasound and phonophoresis treatment. Ortop Traumatol Rehabil 9:449–58
- Jackson MJ. (2009). Redox regulation of adaptive responses in skeletal muscle to contractile activity. Free Radic Biol Med 47:1267–75
- Jacob SW, de la Torre JC. (2009). Pharmacology of dimethyl sulfoxide in cardiac and CNS damage. Pharmacol Rep 61:225–35
- Jeon K, Byun M, Jue D. (2003). Gold compound auranofin inhibits IkappaB kinase (IKK) by modifying Cys-179 of IKKbeta subunit. Exp Mol Med 35:61–6
- Jeon KI, Jeong JY, Jue DM. (2000). Thiol-reactive metal compounds inhibit NF-kappa B activation by blocking I kappa B kinase. J Immunol 164:5981–9
- Johns LD. (2002). Nonthermal effects of therapeutic ultrasound: the frequency resonance hypothesis. J Athl Train 37:293–9
- Kajita M, Hikosaka K, Iitsuka M, et al. (2007). Platinum nanoparticle is a useful scavenger of superoxide anion and hydrogen peroxide. Free Rad Res 41:615–26
- Kerkweg U, Petrat F, Korth HG, et al. (2007). Disruption of skeletal myocytes initiates superoxide release: contribution of NADPH oxidase. Shock 27:552–8
- Kim NH, Lee MY, Park SJ, et al. (2007). Auranofin blocks interleukin-6 signalling by inhibiting phosphorylation of JAK1 and STAT3. Immunology 122:607–14
- Koksal C, Bozkurt AK, Cangel U, et al. (2003). Attenuation of ischemia/reperfusion injury by N-acetylcysteine in a rat hind limb model. J Surg Res 111:236–9
- Levine R, Garland D, Oliver C. (1990). Determination of carbonyl content in oxidatively modified proteins. Methods Enzymol 186:464–78
- Lévy R, Thanh NT, Doty RC, et al. (2004). Rational and combinatorial design of peptide capping ligands for gold nanoparticles. J Am Chem Soc 126:10076–84
- Lowry O, Rosebrough N, Farr AL, Randall RJ. (1951). Protein measurement with the Folin phenol reagent. J Biol Chem 193:265–75
- Lu M-H, Zheng YP, Huang QH, et al. (2009). Low intensity pulsed ultrasound increases the mechanical properties of the healing tissues at bone-tendon junction. Proceedings of the 25th Annual International Conference of the IEEE Engineering in Medicine and Biology Society; 2009 September 3–6, Cancun. 2141–4
- Ma JS, Kim WJ, Kim JJ, et al. (2010). Gold nanoparticles attenuate LPS-induced NO production through the inhibition of NF-κB and IFN-β/STAT1 pathways in RAW264.7 cells. Nitric Oxide 23:214–9
- Mariano AC, Alexandre GM, Silva LC, et al. (2001). Dimethyl sulphoxide enhances the effects of P(i) in myofibrils and inhibits the activity of rabbit skeletal muscle contractile proteins. Biochem J 358:627–36
- Markert CD, Merrick MA, Kirby TE, Devor ST. (2005). Nonthermal ultrasound and exercise in skeletal muscle regeneration. Arch Phys Med Rehabil 86:1304–10
- Martín R, Menchón C, Apostolova N, et al. (2010). Nano-jewels in biology. Gold and platinum on diamond nanoparticles as antioxidant systems against cellular oxidative stress. ACS Nano 4:6957–65
- Maruani A, Boucaud A, Perrodeau E, et al. (2010). Low-frequency ultrasound sonophoresis to increase the efficiency of topical steroids: a pilot randomized study of humans. Int J Pharm 395:84–90
- Nie Z, Liu KJ, Zhong CJ, et al. (2007). Enhanced radical scavenging activity by antioxidant-functionalized gold nanoparticles: a novel inspiration for development of new artificial antioxidants. Free Radic Biol Med 43:1243–54
- Norton S. (2008). A brief history of potable gold. Mol Interv 8:120–3
- Piedade MCB, Galhardo MS, Battlehner CN, et al. (2008). Effect of ultrasound therapy on the repair of gastrocnemius muscle injury in rats. Ultrasonics 48:403–11
- Poderoso JJ, Carreras MC, Lisdero C, et al. (1996). Nitric oxide inhibits electron transfer and increases superoxide radical production in rat heart mitochondria and submitochondrial particles. Arch Biochem Biophys 328:85–92
- Reid MB, Moody MR. (1985). Dimethyl sulfoxide depresses skeletal muscle contractility. J Appl Physiol 76:2186–90
- Rizzi CF, Mauriz JL, Freitas Corrêa DS, et al. (2006). Effects of low-level laser therapy (LLLT) on the nuclear factor (NF)-kappaB signaling pathway in traumatized muscle. Lasers in surgery and medicine 38:704–13
- Rocha A, Zhou Y, Kundu S, et al. (2011). In vivo observation of gold nanoparticles in the central nervous system of Blaberus discoidalis. J Nanobiotechnol 9:1–9
- Saborido, A. Naudí A, Portero-Otín M, et al. (2011). Stanozolol treatment decreases the mitochondrial ROS generation and oxidative stress induced by acute exercise in rat skeletal muscle. J Appl Physiol 110:661–9
- Saliba S, Mistry D, Perrin D, et al. (2007). Phonophoresis and the absorption of dexamethasone in the presence of an occlusive dressing. J Athl Train 42:349–54
- Silveira PC, Victor EG, Schefer D, et al. (2010). Effects of therapeutic pulsed ultrasound and dimethylsulfoxide (DMSO) phonophoresis on parameters of oxidative stress in traumatized muscle. Ultrasound Med Biol 36:44–50
- Silveira PC, Victor EG, Schefer D, et al. (2012). Effects of therapeutic pulsed ultrasound and dimethylsulfoxide phonophoresis on oxidative stress parameters after injury induced by eccentric exercise. Ultrasonics 52:650–4
- Singh P, Kumari K, Katyal A, et al. (2009). Synthesis and characterization of silver and gold nanoparticles in ionic liquid. Spectrochim Acta A Mol Biomol Spectrosc 73:218–20
- Sokolov K, Follen M, Aaron J, et al. (2004). Real-time vital optical imaging of precancer using anti-epidermal growth factor receptor antibodies conjugated to gold nanoparticles. Cancer Res 63:1999–2004
- Sonavane G, Tomoda K, Makino K. (2008). Biodistribution of colloidal gold nanoparticles after intravenous administration: effect of particle size. Colloids Surf B Biointerfaces 66:274–80
- Sul O-J, Kim JC, Kyung TW, et al. (2010). Gold nanoparticles inhibited the receptor activator of nuclear factor-κB ligand (RANKL)-induced osteoclast formation by acting as an antioxidant. Biosci Biotechnol Biochem 74:2209–13
- Supinski GS, Callahan LA. (1985). Free radical-mediated skeletal muscle dysfunction in inflammatory conditions. J Appl Physiol 102:2056–63
- Suryanarayana C, Norton M. (1998). X-ray diffraction: a practical approach. Microsc Microanal 4:513–5
- Tang H, Wang CC, Blankschtein D, Langer R. (2002). An investigation of the role of cavitation in low-frequency ultrasound-mediated transdermal drug transport. Pharm Res 19:1160–9
- Ten Broek RW, Grefte S, Von den Hoff JW. (2010). Regulatory factors and cell populations involved in skeletal muscle regeneration. J Cell Physiol 224:7–16
- Tezel A, Sens A, Mitragotri S. (2002). Investigations of the role of cavitation in low-frequency sonophoresis using acoustic spectroscopy. J Pharm Sci 91:444–53
- Tidball JG. (2005). Inflammatory processes in muscle injury and repair. Am J Physiol Regul Integr Comp Physiol 288:R345–53
- Tsai C-Y, Shiau AL, Chen SY, et al. (2007). Amelioration of collagen-induced arthritis in rats by nanogold. Arthritis Rheum 56:544–54
- Turkevich J, Stevenson PC, Hillier J. (1951). A study of the nucleation and growth processes in the synthesis of colloidal gold. Discuss Faraday Soc 11:55–75
- Victor EG, Silveira PC, Possato JC, et al. (2012). Pulsed ultrasound associated with gold nanoparticle gel reduces oxidative stress parameters and expression of pro-inflammatory molecules in an animal model of muscle injury. J Nanobiotechnol 10:1–9
- Wendel A. (1981). Glutathione peroxidase. Methods Enzymol 77:325–33
- Yang J-H, Kim TY, Lee JH, et al. (2008). Anti-hyperalgesic and anti-inflammatory effects of ketorolac tromethamine gel using pulsed ultrasound in inflamed rats. Arch Pharm Res 31:511–7