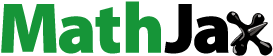
Abstract
The present article reports the preparation, characterization and performance evaluation of solid lipid nanoparticles (SLNs) based on polyoxyethylene-40 stearate (PEG-40 stearate) for the administration of antifungal agents such as ketoconazole and clotrimazole. These nanoparticles could be useful in the treatment of vaginal infections sustained by Candida albicans. In particular, PEG-40 stearate was made to react with acryloyl chloride in order to introduce an easily polymerizable moiety for the creation of a second shell and to ensure a slow drug release. In addition, the differences on the release profiles between PEG-40 stearate-based nanoparticles, PEG-40 stearate acrylate based and polymerized ones, were analyzed under conditions, simulating the typical environment of Candida albicans infection. Then, the antifungal activity of nanoparticles was also evaluated in terms of minimal inhibitory concentration. Moreover, the nanoparticles were submitted to in vitro studies for evaluating the drug permeability at the site of action. Results indicated that the obtained particles are potentially useful for the treatment of vaginal infections sustained by Candida albicans.
Introduction
Mucoadhesive drug delivery systems (MDS) have become hugely interesting in the last years. Their ability to stick to mucous membranes helps us to solve the problem of low bioavailability of traditional delivery systems. In particular, the MDS improve the penetration of medicinal agent in the organs, interested by the movement of tissues or production of various secretions, such as vagina (Ugwoke et al., Citation2005). In fact, they give a rapid absorption and good bioavailability due to the considerable surface area and the high blood flow of the considered site (Richardson & Illum, Citation1992; Vermani & Garg, Citation2000). In addition, drug delivery across the mucosa bypasses the first-pass hepatic metabolism thus avoiding drug degradation by gastrointestinal enzymes and, in the same time, favors the systemic delivery also of molecules characterized by a high-molecular weight (Shaikh et al., Citation2011).
In particular, the vaginal epithelium is permeable to a wide range of drugs, like hormones, antimycotics, peptides and proteins. In addition, a prolonged contact of a delivery system with the vaginal mucosa may be achieved more easily than at other absorption sites like rectum or intestinal mucosa (Valenta et al., Citation2001). The currently available vaginal delivery systems have limitations, such as leakage, messiness and low residence time, which contribute to poor patient compliance. This work, inserting in this context, aims to produce a new controlled delivery system for the vaginal administration of clotrimazole and ketoconazole drugs widely used for the treatment of mycotic infections of the genitourinary tract (Achkar & Fries, Citation2010). The goal is also to obtain a potential mucoadhesive structure, providing long-term therapeutic concentrations at the site of infection. For this reason, the use of polyethylene glycol (PEG) fits perfectly with this perspective. It is characterized by two terminal primary hydroxyl groups, and is the most common commercial polymer, widely used as an inert polymeric support in organic synthesis and as a conjugating agent for biologically active molecules, due to its unique characteristics of solubility and stability (Rhee et al., Citation1994). The formation of a covalent bond with PEG allows the stabilization and solubilization in vivo of pharmacologically active molecules, reducing their toxicity and antigenicity, as well as enhancing cell penetration (Chiappetta & Sosnik, Citation2007). In addition, the solubility of the PEG can solve the problems related to the transport and distribution of drugs in tissues.
In particular, this work is devoted to the preparation of solid lipid nanoparticles (SLNs) based on polyoxyethylene-40 stearate (PEG-40 stearate) for the administration of antifungal agents useful in the treatment of vaginal infections due to Candida albicans. SLNs are spherical particles made from solid lipid, in the nanometer range, which are dispersed in water or in aqueous surfactant solutions (Gasco, Citation1997; Müller, Citation2007). These nanoparticles combine the advantages of different colloidal drug delivery systems, avoiding some of their disadvantages (Kaur et al., Citation2008) and their bioacceptable and biodegradable nature makes them less toxic as compared to polymeric nanoparticles (Trombino et al., Citation2009, Citation2013). In this work, PEG-40 stearate was reacted with acryloyl chloride in order to introduce a polymerizable moiety for the creation of a second shell with the purpose to obtain a slow drug release. The differences on the release profiles between PEG-40 stearate-based systems and PEG-40 stearate derivatized and polymerized-based ones, under conditions, simulating the pathologic environment, were also evaluated. Furthermore, the antifungal activity of SLNs was tested in vitro by drugs minimal inhibitory concentration (MIC) evaluation. Finally, in order to investigate how prepared nanocarriers could enhance drug permeability at the site of action, the mechanism of action of SLNs and the way of drug absorption across a monolayer were examined.
Methods
Reagents
Acetone, butanol, chloroform, ethanol, isopropanol, methanol, n-hexane and tetrahydrofuran (THF) were purchased from Carlo Erba Reagents (Milan, Italy). Polyoxyethylene-40 stearate (PEG-40 stearate), acryloyl chloride (C3H3ClO), triethylamine (Et3N), polyoxyethylene-20 sorbitan monolaurate (Tween-20), taurodeoxycolic acid (C26H45NO6S), ammonium persulfate [(NH4)2S2O8], N,N′-methylene-bisacrylamide (MBAA), sorbitan trioleate (Span-85), polyoxymethylene sorbitan trioleate (Tween-85), N,N,N′,N′-tetramethylethylenediamine (TMEDA), ketoconazole (C26H28Cl2N4O4), clotrimazole (C22H17ClN2) and tryptic soy broth (TSB) were purchased from Sigma-Aldrich (Sigma Chemical Co., St Louis, MO).
Instruments
Infrared spectra were performed on KBr pellets using a Fourier transform infrared spectrometer (1720; Perkin-Elmer, Norwalk, CT), in the range 4000–400 cm−1 (16 scans). 1H-NMR analyses were performed by a spectrometer (Bruker VM-300 ACP, Billerica, MA); chemical shifts are expressed in δ and are related to the solvent. UV-Vis spectra were carried out using UV spectrophotometer V-530 (JASCO, Halifax, Nova Scotia, Canada). Samples were freeze-dried using a freeze-drying Micro Modulyo Edwards apparatus (Edwards, Haverhill, MA). Dimensional analyses of microparticles were realized through a dynamic laser light scattering Brookhaven 90 Plus (Holtsville, NY) particle size analyzer (PSA) and transmission electron microscopy (TEM) (ZEISS EM 900, Oberkochen, Germany). Morphologic and superficial features of nanoparticles were analyzed by using a scanning electron microscope (Leo 420 Stereoscan, Rochester, NY). The toxicity of nanoparticles was performed using a plate reader (EL′808, BioTek Instruments Inc. Bedfordshire, United Kingdom). HPLC analyses were effected using Agilent Technologies HPLC 1200 series equipment and Agilent Chemstation Software (Santa Clara, CA).
Acrylation of PEG-40 stearate
In a three-neck flask fitted with a reflux condenser, funnel dripper, magnetic stirring, thoroughly flamed and maintained under nitrogen bubbling, PEG-40 stearate (2 g, 6.1 mmol) was dissolved in dry THF (60 ml) and added of triethylamine (Et3N 0.85 ml, 6.1 mmol) and acryloyl chloride (0.50 ml, 6.1 mmol). Reaction mixture was maintained under vigorous stirring at 90 °C and monitored through thin-layer chromatography (TLC) on silica gel plates (eluent chloroform). Obtained product, as a waxy white solid, was dried at reduced pressure and purified through a silica chromatographic column (eluent mixture chloroform/methanol 5:5). Finally, it was characterized by FT-IR and 1H-NMR spectroscopies. Yield: 78%.
SLNs preparation
SLNs were prepared by a microemulsion technique at moderate temperature according to Gasco procedure (1997) as shown in . Briefly, PEG-40 Stearate or PEG-40 stearate acrylate in the absence and/or in the presence of the drug (ketoconazole or clotrimazole) were melted at 70–75 °C. A warm water solution of sodium taurocholate, butanol and Tween-20 was then added to obtain an optically transparent system. The warm microemulsion was immediately dispersed in cold water (∼2 °C) under high-speed homogenation (Model SL2, Silverson Machines Ltd, Buckinghamshire, UK) at 8000 rpm for 15 min. The volume ratio of warm microemulsion to cold water was 1:20. Dispersions were washed two times using an Amicon TCF2A ultrafiltration system (Amicon-Grace, Beverley, MA; Amicon Diaflo membrane YM 100).
Table 1. Composition of SLNs based on PEG-40 stearate and PEG-40 stearate acrylate.
Size distribution analyses
Particles' size was determined by dynamic light scattering (DLLS) using a 90 Plus PSA (Brookhaven Instruments Corporation, Holtsville, NY) at 25 °C by measuring the autocorrelation function at 90 ° scattering angle. Cells were filled with 100 μl of sample solution and diluted to 4 ml with filtered (0.22 μm) water. The polydispersity index (PI) indicating the measure of the distribution of nanoparticle population (Koppel, Citation1972), was also determined. Six separate measurements were made to derive the average. Data were fitted by the method of inverse “Laplace transformation” and Contin (Provencher Citation1982a, Citationb).
Percentage of entrapped drug into SLNs
The efficiency encapsulation of SLNs formulations was evaluated through a spectrophotometer UV-Vis Jasco V-530. Briefly, the amount of unencapsulated drug in the SLNs is removed by centrifugation (at 8000 rpm for 30 min) and filtration. Subsequently the obtained samples were diluted in methanol (1:9) and analyzed. The absorbance (Å) of the samples was measured using quartz cells with a thickness of 1 cm and operating at specific wavelengths for each drug [λ(ketoconazole) = 220 nm; λ(clotrimazole) = 238 nm].
Polymerization of SLNs based on PEG-40 stearate acrylate
Microspheres based on PEG-40 stearate acrylate were produced by radical polymerization reaction in reverse-phase emulsion. Briefly, a cylindrical glass reactor of 100–150 ml equipped with mechanical stirrer and dripping funnel, screw cap with puncture-proof rubber septum was flamed in a nitrogen flow and after cooling was immersed in a bath thermostatically controlled at 40 °C. Then, required amount of n-hexane (20 ml) and chloroform (18 ml), constituting the dispersant phase, were introduced into the reactor. After 30 min of N2 bubbling, this mixture was treated with 4 ml of distilled water containing 0.1 g of nanoparticles based on PEG-40 stearate acrylate, the comonomer MBAA (0.009 g, 5.7 × 10−2 mmol) and ammonium persulfate such as radical initiator [(NH4)2S2O8 0.15 g, 0.66 mmol]. Under stirring at 1000 rpm, the mixture was treated with Span-85 (100 µl) and Tween-85 (50 µl), then after 10 min with TMEDA (150 µl) and stirring was continued for another 60 min (Iemma et al., Citation2006; Cassano et al., Citation2011, Citation2012; Trombino et al., Citation2011). Microspheres so obtained were filtered, washed several times with 50 ml portions of 2-propanol, ethanol, acetone and diethyl ether to remove all traces of free acrylic moieties, comonomer and initiator and dried overnight under vacuum at 40 ºC. Their characterization was effected by light scattering and TEM.
Release studies from nanoparticles
Release studies were performed into dialysis membranes (MW cutoff 12–14 KDa) and at different pH values (2.7, 4.6 and 6.5), simulating the vaginal environment during infections sustained by Candida albicans. Aliquots of nanoparticles (0.05 g) dissolved in different PBS solutions, according to considered pH, were placed in a beaker containing 25 ml of the same buffered solution. Finally, this beaker was placed in a shaking and thermostated bath in the dark and at 37 °C. At different time-points (30 min, 1 h, 2 h, 4 h and 6 h) solutions were analyzed spectrophotometrically [λ(ketoconazolo) = 220 nm; λ(clotrimazolo) = 238 nm]. The percentage of drug released was expressed in relation to the absorbance.
In vitro antimycotic activity of nanoparticles
Antimycotic activity of SLNs was tested in vitro. In order to enhance drug release and diffusion, nanoparticles were suspended in NaCl 0.85% physiological solution to obtain a concentration equal to 100 µg/ml and maintained at 37 °C for 48 h. After that, the MIC of each drug was tested through the dilution method in the culture broth as follows. In 12 sterile tubes, 0.5 ml of TSB, used in microbiology laboratories as a culture broth to grow aerobic bacteria, were added; the first tube was used as control; tubes from 2nd to 10th were added of 0.5 ml of solution containing drug-loaded SLNs. Subsequently, doubling scalar dilutions were conducted by adding at the end in each tube 0.5 ml of culture broth containing Candida albicans diluted 1–1000 parts per million (ppm). The first tube represents the growth control tube without drug. All tubes were incubated at 37 °C and examined after 24 and 48 h. MIC was calculated by considering the antibiotic concentration of the first tube without no visible microorganism growth.
In vitro mechanistic studies
In order to investigate how prepared nanocarriers could enhance drug permeability at the site of action, we decided to examine the mechanism of action of SLNs and the exact way of drug absorption across a monolayer (paracellular or transcellular).
To this purpose, we used a human vaginal epithelial cell line known as Vk2/E6E7 purchased from ATCC (American Tissue Culture Collection, USA); these cell line provides the basis of a valid and reproducible in vitro model for studies on cervicovaginal physiology and infections and for testing pharmacologic agents for intravaginal application. The conditions of growth of this cell line were the following ones: keratinocyte serum-free medium (GIBCO®) with 0.1 ng/ml human recombinant epidermal growth factor, 0.05 mg/ml bovine pituitary extract, and additional calcium chloride 44.1 mg/l (final concentration 0.4 mM).
Before to study this mechanism of action, it was necessary to calculate the toxicity of nanoparticles by performing MTT assays: Vk2/E6E7 cells were cultured in 96-well plates at a cell density of 5 × 105 cells/well; every column receiving different dilutions prepared from a stock suspension (10 mg/ml) of different SLNs types. Plates were read by using a plate reader (EL × 808, BioTek Instruments Inc.) to determine the IC50 values of microspheres by plotting the percentage of living cells versus log10 concentration.
After that, we examined the influence of our nanocarriers on the integrity of tight intercellular junctions using the Vk2/E6E7 cell monolayer model and we also evaluated transepithelial electrical resistance (TEER). TEER is a measure of monolayer permeability to ions, with a decrease in TEER indicating permeability to ions and other hydrophilic solutes (Anderberg & Artursson, Citation1993).
In order to examine the effect of nanoparticles on the TEER and drug uptake into cells, Vk2/E6E7 cell monolayers were prepared. Vk2/E6E7 cells (passage 8–15) were seeded on Transwell® 12-well plate having a polycarbonate membrane (area 1 cm2, pore diameter 0.4 µm) at a seeding density of 1.44 × 105 cells/ml (equivalent to 7.2 × 104 cells/insert or to 6.4 × 104 cells/cm2). Cell suspension was added to the apical compartment (0.5 ml) and medium added to the basolateral compartment (1.5 ml) and incubated (37 °C, 5% humidity, 95% CO2). The medium in both the apical and basolateral sides were changed every other day. At least 21 days were given to allow the cells to mature and form a monolayer after which both the TEER and cell-uptake studies were performed.
SLNs suspensions (2.3 mg/ml), in a non-cytotoxic concentration, were prepared in HBSS containing Ca2+ and Mg2+ supplemented with penicillin (100 U/ml) and streptomycin (100 U/ml). The culture medium was first aspirated from the Transwells® and HBSS was added into both the apical (0.5 ml) and basolateral (1.5 ml) compartments and the cells incubated for 1 h (37 °C, 95% of humidity and 5% CO2). TEER of the monolayer in HBSS was then measured prior to the addition of empty SLN suspensions (0.5 ml) in the apical chambers. TEER measurements were carried out at 40 min intervals. After 160 min, polymeric matrices were removed and replaced with fresh HBSS and the TEER was measured again after 1 h to determine if the effect of SLNs on the monolayers was reversible. The values coming from the measurements in HBSS alone (empty inserts not containing monolayers) were taken as negative control and PEI (polyethileneimine), known to open tight junction, was taken as the positive control. The TEER of the monolayers were measured using a Millicell®-ERS meter (Millipore, Bedford, MA) connected to a pair of electrodes which were separately placed in the apical and basolateral compartments.
The TEER across the Vk2/E6E7 monolayers after 21 days was 300–500 Ω cm−2 similarly to values reported in literature. As expected, PEI 25 kDa, the positive control known to significantly reduce TEER (Cheng et al., Citation2006), caused a 25% reduction in the TEER of the cell monolayers. The effect was reversible in the long term.
Due to the formation of tight junctions by the Vk2/E6E7 cell monolayer, Lucifer yellow, a paracellular marker, which is transported in between tight junctions, was loaded into nanoparticles as previously reported to demonstrate very low compartments. Cell suspension in the same medium (0.5 ml) was added to the apical compartments, and the cells were incubated at 37 °C in an atmosphere of 95% relative humidity and 5% CO2. Cells were maintained for 21 days to allow a confluent monolayer to develop. Culture media in both apical and basolateral compartments were changed every other day. TEER measurements were performed and transport studies were conducted 21-days post-seeding, after confirming the integrity of the monolayers. TEER of the Vk2/E6E7 cell monolayers was measured using a Millicell-ERS meter (Millipore). Nanocarriers dispersions (5 mg ml−1) were prepared in Hanks balanced salt solution (HBSS) containing Ca2+ and Mg2+ supplemented with penicillin (100 U ml−1) and streptomycin (100 μg ml−1). The culture medium was first aspirated from Transwells, HBSS was added into both the apical and basolateral compartments, and the cells were incubated for 1 h at 37 °C in an atmosphere of 95% humidity and 5% CO2. TEER in HBSS was then measured prior to the addition of nanocarriers dispersions (0.5 ml) in the apical compartments. TEER measurements were carried out at 40-min intervals. After 160 min, nanocarriers dispersions were removed and replaced with fresh HBSS and TEER was measured again after removal of the polymer dispersions. HBSS and PEI (25 kDa) solutions were used as negative and positive controls, respectively.
Moreover, in order to study the transport kinetics, a paracellular transport marker, Lucifer yellow, was used. SLNs dispersions (5 mg ml−1) containing Lucifer yellow (0.5 mg ml−1) were prepared in HBSS as previously described for the loading of clotrimazole or ketoconazole. SLNs containing Lucifer yellow were added to the apical compartments of Transwells, and this plates were incubated at 37 °C, 95% humidity and 5% CO2 for 160 min. Basolateral samples (1 ml) were taken every 40 min and replaced with equal volumes of fresh HBSS, and fluorescence readings (excitation wavelength = 485 nm, emission wavelength = 530 nm) were recorded. Lucifer yellow concentrations were determined with reference to a calibration curve using standard solutions (0.2−20 μg ml−1) for the fluorescence emission (y = 4.917x + 8.7023, r2 > 0.99), and control samples (generated in the absence of nanoparticles) were also analyzed.
After that, the effect of nanoparticles on transcellular transport was also analyzed by using a transcellular marker known as antipyrine. SLN dispersions (2.3 mg ml−1) containing antipyrine (0.4 mg ml−1) were prepared in HBSS and added to the apical compartments, and Transwells were incubated at 37 °C, 95% humidity and 5% CO2 for 160 min. Basolateral samples (0.5 ml) were taken every 40 min and replaced with equal volumes of fresh HBSS, and the concentration of antipyrine was analyzed by HPLC. Samples (20 μl) were chromatographed over two Onyx Monolithic C18 5-μm columns (2 × 100 × 4.6 mm) using Agilent Technologies HPLC 1200 series equipment. Columns were fitted with a guard column maintained at 30 °C in the thermostated column compartment (Agilent Technologies 1200 series) at a flow rate of 1.5 ml min−1. Samples were eluted with a methanol, water (30:70) mobile phase, and antipyrine was detected at a wavelength of 254 nm and had a retention time of 5.0 min. The data was analyzed using the Agilent Chemstation Software and a calibration curve (y = 27.738x − 1.0777, r2 = 0.9997) prepared with standard solutions (0.5−10 μg ml−1).
Results and discussion
In recent years, great attention has been paid to the development of drug delivery systems to achieve site-specific action of the drug against the mucosa (Ugwoke et al., Citation2005). Hence, the idea of designing mucoadhesive systems are useful for the drugs release. These materials (Shaikh et al., 2011) must adhere to the mucous membranes for the time necessary to release the drug contained therein in a controlled manner. The present work, inserting in this context, was aimed at the design, synthesis and evaluation of controlled drug release from SLNs. These last are based on PEG-40 stearate, a synthetic polymer composed of stearic acid (a fatty acid found in nature) and PEG, a typical biocompatible and non-toxic substrate. The particular attention paid to PEG (Chiappetta & Sosnik, Citation2007), besides on its mucoadhesive properties, also arises from its peculiar chemical characteristics: it is soluble in water as in many other solvents; it is commercially available in various forms and is susceptible to various chemical modifications, in particular, its acryloyl derivative may easily be polymerized, and it could provide a good degree of cellular adhesion. In this work, PEG-40 stearate acrylated and not acrylated nanoparticles have been realized by microemulsion technique (Trombino et al., Citation2009, Citation2013). A part of acrylated ones have been polymerized by the emulsion polymerization in reverse phase. This has allowed realizing a polymeric film around the nanoparticles with the goal of delaying the release of the drug from the system matrix. In fact, the hydrophylic structure of polymerized nanoparticle enables the mucoadhesion and the drugs permeation. In particular, the conventional SLNs were formed with lipophilic blocks of molecules linked by hydrophobic interaction. On the other hand, the outer polymeric shell was formed by the hydrophilic blocks of the copolymers (PEG), playing an important role in the in vivo behavior, particularly for their ability to interact with the hydrophilic moieties of mucine.
In each type of lipid particles, ketoconazole or clotrimazole were encapsulated. Both of them are antifungals drugs with imidazole structure, used in infections caused by various microorganisms including Candida albicans, a fungus that is the leading cause of candidiasis in the mouth, stomach, urinary tract and, in particular, in vagina.
Acrylation of PEG-40 stearate
The mechanism of this reaction consists in the nucleophilic attack of the free hydroxyl group of PEG-40 stearate to the electrophilic carbonyl group of the acryloyl chloride with the formation of HCl, in the reaction medium, neutralized by triethylamine.
The obtained compound was characterized by means of the common spectroscopic techniques: FT-IR (KBr) n (cm−1): 3449, 2890, 1733, 1722, 1114, 812 and 807; 1H-NMR (D2O) d (ppm): 1.487–1.923 (m, 31H), 2.109–2.231 (m, 4H), 3.123–3.677 (m, 88H), 3.719–3.880 (m, 15H), 3.947–3.972 (m, 31H), 4.011–4.370 (m, 26H), 5.75 (dd, 1H), 5.90 (dd, 1H) and 6.20 (dd, 1H).
SLNs preparation and characterization
In the present work, SLNs based on PEG-40 stearate or on PEG-40 stearate acrylate were prepared using the microemulsion technique. These particles were characterized by PSA which provides information related to the diameter of the particles and their index of PI ().
Table 2. Mean particle size, PI index and EE (%) of SLNs. Overall *p50.05 (data are expressed as mean ± standard error of mean of six individual samples per group).
Nanoparticles dimensions are strongly influenced by the nature of the substrate used. Substrates containing hydrophilic portions, during assembly, will tend to assume a spatial configuration to allow the maximum exposure of the polar head (–OH) toward the aqueous environment. In fact, the SLNs are prepared in water and the substrates are oriented in such a way that the exposure to the water of hydrophobic portion is minimal. For this reason, nanoparticles based on PEG-40 stearate acrylate possess diameters smaller than those obtained from PEG-40 stearate.
Characterization through UV-Vis spectrophotometer
The characterization of the particles by spectrophotometer is useful for the determination of the percentage of encapsulation of each drug in the respective solid lipid particles, since (by the law of Lambert–Beer) the absorbance is concentration dependent. The encapsulation efficiency (EE) (%) is the percentage of drug encapsulated in SLNs expressed referring to the initial drug amount used. This is calculated using the following equation:
where gi indicates the grams of drug initially used and gf the final amount of drug effectively entrapped into nanoparticles. Data related to the efficiency of encapsulation () revealed that clotrimazole is encapsulated in great amount inside the nanoparticles based on PEG-40 stearate and on PEG-40 stearate acrylate. This is attributable to the greater lipophilicity of clotrimazole compared to ketoconazole and to its lower steric hindrance.
Characterization by TEM
Through this technique it is possible to observe that nanoparticles have a spherical shape (). Moreover, TEM confirms dimensions previously obtained with PSA ().
The small difference in terms of dimensions between data reported in is due to the fact that by using PSA it is possible to measure just a hydrodynamic radius that is slightly greater.
Polymerization of SLN based on PEG-40 stearate acrylate
Particles based on PEG stearate derivatized with acryloyl chloride were polymerized by radical polymerization reaction in reverse-phase emulsion. With this innovative and versatile technique is very easy to obtain materials with adaptable properties. The radical polymerization has a chain growth mechanism that begins with the formation of primary radicals produced by cleavage of a suitable initiator. In order to propagate the reaction, allowing cross-linking, functional groups capable of interacting with the radical species and to be converted into active centers of propagation, must be present. These functional groups have to be introduced with appropriate derivatization using a chemical containing a feature likely to radical polymerization or double bonds able of undergoing free radical polymerization, such as acrylic and methacrylic compounds. The properties of obtained microspheres depend on the degree of cross-linking, which is in turn influenced by the degree of acrylation and the amount of monomer and reticulant agents used in the polymerization reaction. In particular, this technique consists in the addition of an aqueous solution of a monomer (dispersed phase) in an excess of organic solvents immiscible with water (dispersing phase). Under stirring, small droplets of dispersed phase are formed and, to decrease their free energy interfacial, they assume a spherical shape.
The radical polymerization (Iemma et al., Citation2006; Cassano et al., Citation2011, Citation2012; Trombino et al., Citation2011) provides a mechanism of chain growth which starts with the generation of primary radicals following the cleavage of a suitable initiator. These radicals will then react with the acrylic function present on the side chain of PEG-40 stearate determining the progressive cross-linking. The formation of spherical particles was favored by using surfactants that are substances capable of lowering the surface tension of a liquid, favoring the wettability of the surfaces or the miscibility between different liquids. On the other hand, TMEDA allows accelerating the process of decomposition of the radical initiator.
Characterization of polymerized nanoparticles
Polymerized nanoparticles were characterized, in analogy to the other two types of particles previously described, through PSA and TEM. Obtained results revealed that the diameter of polymerized particles is greater than that of non-polymerized ones since a polymer coating around them is formed and this contributes to increasing their size ( and ).
Polymerized particles were obtained from those based on PEG-40 stearate acrylate containing the drug (ketoconazole or clotrimazole) and have an efficiency of encapsulation equal to the particles used as substrate.
Release studies from nanoparticles
Release studies revealed that the particles effectively release the drug at pH values corresponding to those typical of the vaginal environment in the presence of Candida albicans ( and ).
Figure 3. Profiles of clotrimazole release from SLNs PEG-40 stearate and PEG-40 stearate acrylate nanoparticles at three different pH (2.7, 4.6 and 6.5), simulating the conditions of infection with Candida albicans in the vagina, and at different time intervals (1, 2, 4, 6 and 12 h) in a shaking bath at 37 °C. Results indicate mean of three independent experiments done in triplicate.
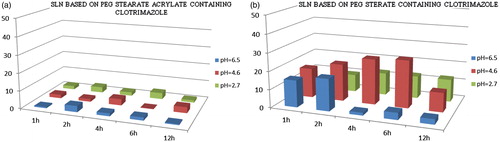
Figure 4. Profiles of ketoconazole release from SLNs PEG-40 stearate and PEG-40 stearate acrylate nanoparticles at three different pH (2.7, 4.6 and 6.5), simulating the conditions of infection with Candida albicans in the vagina, and at different time intervals (1, 2, 4, 6 and 12 h) in a shaking bath at 37 °C. Results indicate mean of three independent experiments done in triplicate.
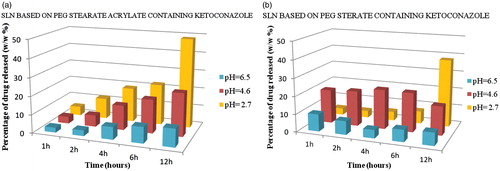
Obtained results showed that SLNs based on PEG-40 stearate acrylate release less drug than those based on PEG-40 stearate, and release more than SLNs polymerized. This is due to the more lipophilic nature of the matrix, that being more compact, release smaller amounts of drug. More hydrophilic matrices, instead, enhance drug diffusion and release it more easily. The release is influenced also by the chemical nature of the loaded drug. In fact, ketoconazole is less lipophilic of clotrimazole and consequently is released in greater amounts. The release of drugs from polymerized SLNs is delayed in time due to the presence of the polymeric coating around the lipid matrix ().
Figure 5. Polymerized SLNs profiles of clotrimazole and ketoconazole release at three different pH (2.7, 4.6 and 6.5), simulating the conditions of infection with Candida albicans in the vagina, and at different time intervals (1, 2, 4, 6 and 12 h) in a shaking bath at 37 °C. Results indicate mean of three independent experiments done in triplicate.
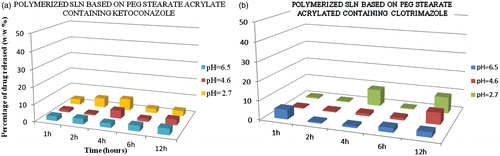
Evaluation of nanoparticles antifungal activity
The antifungal activity of drugs, encapsulated in SLNs, was evaluated in terms of minimum inhibitory concentration (MIC). The results, reported in , show that the nanoparticles containing ketoconazole or clotrimazole, characterized by a very high value of MIC, can only be used for a topical therapy, resulting toxic for systemic administration. On the contrary, nanoparticles characterized by a low MIC value, such as those based on PEG stearate acrylate, containing clotrimazole, showing a MIC of 1.56 µg/ml, are administrable systemically because non-toxic to the patient. Consequently, the results of this work indicate that the SLNs obtained are potentially useful for the treatment of vaginal infections supported by Candida albicans.
Table 3. Drugs MIC values.
In vitro mechanistic studies
Before to study the drug transport mechanism, MTT assay performed to calculate IC50 values for empty SLNs revealed that this value is equal to ∼2.21 mg/ml for every tested nanoparticles type. This was necessary in order to know the amount and concentration of SLNs dispersions we could use during subsequent permeability studies. MTT assays, as it can be seen by observing , revealed that obtained carriers are rather not toxic delivery systems since the obtained value represents a quite high concentration of substrate to work with.
To investigate the mechanism of action of prepared substrates, in the first instance we examined the influence of SLNs on the integrity of tight intercellular junctions using the Vk2/E6E7 cell monolayer model and an evaluation of the TEER. The TEER across Vk2/E6E7 monolayers after 21 days was 300−500 Ω cm−2. As expected, PEI 25 kDa, the positive control known to significantly reduce TEER, caused a 25% reduction in the TEER of the cell monolayers. The effect was reversible in the long term. There was no reduction in the TEER observed with our nanoparticles at the high concentration of 5 mg ml−1.
Due to the formation of tight junctions by Vk2/E6E7 cell monolayer, Lucifer yellow, a paracellular marker, which is transported between tight junctions, demonstrated very low permeability, with negligible amounts crossing the monolayer. The positive control, poly(ethylenimine), which increases the paracellular permeability across Vk2/E6E7 cell monolayer, significantly increased (by over 20-fold) the amount of Lucifer yellow transported across the monolayer from the apical to the basolateral compartments. The prepared SLNs did cause a slight increase in the transport of Lucifer yellow (). However, the amount of Lucifer yellow transported across the monolayer when loaded into our nanoparticles was only 10% of that seen in the presence of poly(ethylenimine) nanoparticles loaded with the same dye. It is clear that they do not appreciably alter the permeability of the monolayers to ions, or increase the paracellular permeability of the monolayer to any great extent, all indications that nanoparticles do not open tight junctions. We conclude that SLNs in solution behave very differently from the cationic polymer poly(ethylenimine) that instead opens tight junctions. It is speculated that the self-assembly of prepared SLNs prevents the interaction of the hydrophilic groups with the appropriate tight junction proteins and in this way prevents their opening.
Antipyrine is a transcellular marker which passively diffuses across the Vk2/E6E7 monolayer with good permeability. When antipyrine alone was applied to the apical compartment of the Vk2/E6E7 cell monolayer, the concentration of antipyrine in the basolateral chamber reached a plateau after 80 min () indicating that this was the maximum amount that can traverse the monolayer unaided. All SLN samples significantly increased the transport of antipyrine resulting in a higher maximum plateau. It is clear that all particles tested have a significant effect on the transcellular transport of antipyrine and that polymer-loaded SLN are more effective at promoting transcellular transport than PEG stearate-loaded SLN and PEG stearate acrylate-loaded SLN ().
Conclusions
This work reports the preparation of SLNs based on PEG-40 stearate and PEG-40 stearate acrylate containing antifungal drugs, useful in vaginal infections caused by Candida albicans, such as ketoconazole and clotrimazole. The obtained particles have a spherical shape and nanometric dimensions depending on the substrate used for the preparation of the SLNs. In particular, nanoparticles based on PEG-40 stearate have a larger diameter respect to that PEG-40 stearate acrylate based that, being more lipophilic, possess a more compact matrix. Acrylated SLNs were also polymerized with the aim to obtain a polymeric film, around the particles, which could retard the release of the entrapped drug. These particles possess a greater diameter, respect to not polymerized ones, due to the polymeric coating around them.
All materials were tested in terms of EE. Results revealed that the percentage of encapsulation depends on the type of the drug and on the matrix properties. Precisely, due to the higher lipophilicity of clotrimazole compared with ketoconazole, the amount of clotrimazole encapsulated is greater compared to ketoconazole.
The prepared nanoparticles were submitted even to the antifungal activity evaluation. Results revealed that all particles appear to be potentially effective for a topical administration. For a systemic administration, only particles based on PEG-40 stearate acrylate, containing clotrimazole, may be used since these show a low MIC value that ensure not toxicity for the patient.
Besides, to verify the SLNs behavior in the vaginal environment, release studies were performed at three different pHs 2.7, 4.6 and 6.5, simulating the conditions of infection with Candida albicans, and at different time intervals. The obtained results showed that SLNs based on PEG-40 stearate acrylate release less than those based on PEG-40 stearate, and release more than polymerized ones. This is due to the more lipophilic nature of acrylated matrices that, consequently, release a smaller amount of the loaded drug. Instead, more hydrophilic matrices, favor drug diffusion and release. This last is also influenced by the drug characteristics. Ketoconazole is less lipophilic of clotrimazole, so it is released massively. With regard to the polymerized SLNs, drug release is consequently delayed and prolonged in time for the presence of the polymeric coating around the lipid matrix. Obtained data showed the possibility of using SLNs based on PEG-40 stearate, PEG-40 stearate acrylate and the polymerized ones, such systems for the controlled release and site-specific active ingredients useful in the treatment of vaginal infections and, in particular, caused by Candida albicans. Finally, all nanoparticles were submitted also to the drug permeability studies revealing that all particles tested have a significant effect on the transcellular transport. In particular, the polymerized loaded SLNs are more effective at promoting transcellular transport than PEG stearate-loaded SLNs and PEG stearate acrylate-loaded SLNs.
Declaration of interest
The authors report no conflicts of interest. The authors alone are responsible for the content and writing of this article.
The present work was financially supported by Italian Ministry of University and Research (MIUR) Project: PRIN 2008.
References
- Achkar JM, Fries BC. (2010). Candida infections of genitourinary tract. Clin Microbiol Rev 23:253–73
- Anderberg EK, Artursson P. (1993). Epithelial transport of drugs in cell-culture. VIII: effects of sodium dodecyl sulfate on cell membrane and tight junction permeability in human intestinal epithelial (Caco-2) cells. J Pharm Sci 82:392–8
- Cassano R, Trombino S, Ferrarelli T, et al. (2011). Respirable rifampicin-based microspheres containing isoniazid for tuberculosis treatment. J Biomed Mat Res Part A 100:536–42
- Cassano R, Trombino S, Ferrarelli T, et al. (2012). Preparation, characterization and in vitro activities evaluation of curcumin based microspheres for azathioprine oral delivery. React Function Polym 72:446–50
- Cheng WP, Gray AI, Tetley L, et al. (2006). Polyelectrolyte nanoparticles with high drug loading enhance the oral uptake of hydrophobic compounds Biomacromolecules 7:1509–20
- Chiappetta DA, Sosnik A. (2007). Poly(ethylene oxide)-poly(propylene oxide) block copolymer micelles as drug delivery agents: improved hydrosolubility, stability and bioavailability of drugs. Eur J Pharm Biopharm 66:303–17
- Gasco MR. (1997). Solid lipid nanospheres from warm microemulsions. Pharm Techn Eur 9:52–8
- Iemma F, Spizzirri G, Puoci F, et al. (2006). pH-sensitive hydrogels based on bovine serum albumin for oral drug delivery. Int J Pharm 312:151–7
- Kaur IP, Bhandari R, Bhandari S, Kakkar V. (2008). Potential of solid lipid nanoparticles in brain targeting. J Contr Release 127:97–109
- Koppel DE. (1972). Analysis of macromolecular polydispersity in intensity correlation spectroscopy: method of cumulants. J Chem Phys 57:4814–20
- Müller RH. (2007). Lipid nanoparticles: recent advances. Adv Drug Deliv Rev 59:375–6
- Provencher SW. (1982a). A constrained regularization method for inverting data represented by linear algebraic or integral equations. Comp Phys Comm 27:213–27
- Provencher SW. (1982b). Contin: a general purpose constrained regularization program for inverting noisy linear algebraic and integral equations. Comp Phys Comm 27:229–42
- Rhee W, Wallace DG, Michaels AS. (1994). Biologically inert, biocompatible-polymer conjugates. Patent Document No. 5324775, 28 June 1994
- Richardson JL, Illum L. (1992). The vaginal route of peptide and protein drug delivery. Adv Drug Deliv Rev 8:341–66
- Shaikh R, Raghu T, Singh R, et al. (2011). Mucoadhesive drug delivery systems. J Pharm Bioallied Sci 3:89–100
- Trombino S, Cassano R, Muzzalupo R, et al. (2009). Stearyl ferulate-based solid lipid nanoparticles for the encapsulation and stabilization of beta-carotene and alpha-tocopherol. Coll Surf B Biointerfaces 72:181–7
- Trombino S, Cassano R, Cilea A, et al. (2011). Synthesis of pro-prodrugs l-lysine based for 5-aminosalicylic acid and 6-mercaptopurine colon specific release. Int J Pharm 420:290–6
- Trombino S, Cassano R, Ferrarelli T, et al. (2013). Trans-ferulic acid-based solid lipid nanoparticles and their antioxidant effect in rat brain microsomes. Coll Surf B Biointerfaces 109:273–9
- Ugwoke MI, Agu RU, Verbeke N, Kinget R. (2005). Nasal mucoadhesive drug delivery: background, applications, trends and future perspectives. Adv Drug Deliv Rev 57:1640–65
- Valenta C, Kast CE, Harich I, Bernkop-Schnurch A. (2001). Development and in vitro evaluation of a mucoadhesive vaginal delivery. J Contr Release 77:323–32
- Vermani K, Garg S. (2000). The scope and potential of vaginal drug delivery. Pharm Sci Technol Today 3:359–64