Abstract
Context: Ever since the discovery of cyclodextrins, a family of cyclic oligosaccharides based on α (1 → 4) linkage among glucopyranose subunits, these versatile supramolecular hosts have received tremendous attention for scientific explorations. Due to their property of forming host–guest type inclusion complex, cyclodextrins and their synthetic derivatives exhibit wide range of utilities in different areas viz. pharmaceuticals, drug delivery systems, cosmetics, food and nutrition, textile and chemical industry etc.
Objective: The purpose of this review is to highlight properties, advantages, recent studies and versatile benefits of cyclodextrins and to re-strengthen their prospective applications in novel directions for future research.
Methods: This article summarizes a variety of applications of cyclodextrins in various industrial products, technologies, analytical and chemical processes and recent industrial advancements by extensively literature search on various scientific databases, Google and websites of various associated pharmaceutical industries and patenting authorities across the world.
Results and conclusion: Due to possibility of multidimensional changes in physical and chemical properties of molecules upon inclusion complexation in cyclodextrins, these compounds are of great commercial interest and may offer solution to many of the scientific problems of the current world.
Introduction
Cyclodextrins (CDs) are defined as the class of cyclic oligosaccharides which contains glucopyranose units and which are linked to each other by α-(1,4) bonds (Del Valle, Citation2004). Three naturally occurring cyclodextrins, also known as first generation CDs or parent CDs (Singh et al., Citation2002), are: α-cyclodextrin (α-CD), β-cyclodextrin (β-CD) and γ-cyclodextrin (γ-CD), containing 6, 7 and 8 glucopyranose units, respectively.
When starch is reacted with amylase, which is obtained from Bacillus macerans, a crude mixture of α-CD (∼60%), β-CD (∼20%) and γ-CD (∼20%) together is formed with some traces of CDs containing more than eight glucose units. The purification of this mixture is very difficult because it contains very less amounts of CDs and it also have other linear and branched dextrins along with proteins and other impurities (Chordiya & Senthilkumaran, Citation2012). Various chronological in development of CDs are presented in . Finally, in the 1970s, several biotechnological advances led to major improvements in the production of pure α-CD, β-CD and γ-CD. Cyclodextrin glycosyl transferase (CGTase) enzyme was used for the production of highly pure α-CD, β-CD and γ-CDs (Loftsson & Duchene, Citation2007). Production of parent CDs and some of their commercially important derivatives are represented in .
Figure 1. Parent and representative modified cyclodextrins of pharmaceutical interest [RAMEB: Randomly methylated β-cyclodextrin; HP-β-CD: Hydroxy propyl β-cyclodextrin; HE-β-CD: Hydroxy ethyl β-cyclodextrin; DIME-β-CD: Heptakis (2, 6-dimethyl)-β-cyclodextrin; TRIME-β-CD: Heptakis (2, 3, 6-trimethyl)-β-cyclodextrin].
![Figure 1. Parent and representative modified cyclodextrins of pharmaceutical interest [RAMEB: Randomly methylated β-cyclodextrin; HP-β-CD: Hydroxy propyl β-cyclodextrin; HE-β-CD: Hydroxy ethyl β-cyclodextrin; DIME-β-CD: Heptakis (2, 6-dimethyl)-β-cyclodextrin; TRIME-β-CD: Heptakis (2, 3, 6-trimethyl)-β-cyclodextrin].](/cms/asset/f946aa28-a426-42a9-924d-718f001dc59e/idrd_a_938839_f0001_b.jpg)
Table 1. Chronological in development of cyclodextrins.
Structure and properties of CDs
CDs, white crystalline powder, chemically are α-(1,4)-linked cyclic oligosaccharides containing glucopyranose units. The glucopyranose units possess chair conformation, due to which CDs are not cylindrical, and exhibit torus-like shape (truncated cone), in which the primary hydroxyl groups are present on narrow edge and secondary hydroxyl groups are on the wider edge of the cone in the outer surface. In the interior of the CD molecule, skeletal carbons with hydrogen atoms and oxygen bridges are present leading to hydrophilic outer surface and lipophilic central cavity of CD (Cal & Centkowska, Citation2008; ). Some of the structural and chemical properties of parent CDs are given in .
Table 2. Structural and chemical properties of parent cyclodextrins.
Cyclodextrin derivatives
Although, the natural CDs and their complexes are hydrophilic, but their aqueous solubility is reported to be limited, mainly in the case of β-CD. This is because CD molecules binds relatively strongly in their crystal state (Loftsson & Brewster, Citation2010). CDs contain glucopyranose units which further consists of three free hydroxyl groups differing on the basis of both functions as well as reactivity. The different reaction conditions like pH, temperature and reagents affect the relative reactivity of C-2 and C-3 secondary and the C-6 primary hydroxyls of the CD molecule. With the substitution of hydrogen and hydroxyl group with large number of substituting groups, such as alkyl, hydroxyl alkyl, carboxy alkyl, amino, thio, tosyl, glucosyl, maltosyl etc., and thousands of ethers, esters, anhydro, deoxy, acidic and basic etc., 21 hydroxyl groups of β-CD can be modified to obtain different CD derivatives by chemical and enzymatic reactions.
Such derivatizations are done to accomplish the following objectives:
to enhance the solubility of different CD derivatives and their complexes;
to improve the fitting and/or the association between the CD and its guest, with concomitant stabilization of the guest, reducing its reactivity and mobility;
to achieve insoluble, immobilized CD-containing structures and polymers, e.g. for chromatographic purposes (Szejtli, Citation2004).
CD derivatives are mainly categorized as: (a) chemically modified CD derivatives and (b) natural enzymatically modified CD derivatives.
Enzymatically modified CD derivatives are formed with the help of pullulanase enzyme and are known as branched CDs. When the hydroxyls of β-CD are made to react with a chemical reagent, the substitution occurs and a heterogeneous product is generated (Szente & Szejtli, Citation1999). The extent to which substitution occurs can be defined and expressed in different ways as follows:
Degree of substitution: It is defined as the number of the hydroxyl groups in a glucose moiety of CD molecule that has been substituted. Therefore, it can be ranged from 1 to 3. The average degree of substitution (DS) is the average number of hydroxyl groups being substituted in a glucose unit of CD. Its value can vary from 0 to 3 (Challa et al., Citation2005).
Molar degree of substitution: It is the critical parameter, which is mainly defined as the average number of substituent’s that completely reacts with one glucopyranose repeat unit. If the molar degree of substitution (MS) value is equal to 0, then no substitution or up to 3, when two or more substituents react and form oligomeric or polymeric side chains (Loftsson & Brewster, Citation1996).
CD derivatives like hydroxypropyl derivatives of β and γ-CD (HP-β-CD and HP-γ-CD), randomly methylated β-CD (RM-β-CD), sulfobutylether β-CD sodium salt (SBE-β-CD) and the branched CDs, such as maltosyl-β-CD (M-β-CD) are of great pharmaceutical interest (Ogawa et al., Citation2012).
Formation of inclusion complexes
One of the most remarkable features of CDs is their tendency to form solid inclusion complexes with a wide variety of solid, liquid and gaseous compounds by the process of molecular complexation. The complexes formed are called host–guest type of complexes. Such type of complexes involves a guest molecule (drug) which is properly fitted into the lipophilic cavity of host (CD molecule; Del Valle, Citation2004). There is no breakage or formation of covalent bond during the formation of inclusion complex (Loftsson et al., Citation2005a,b). When the CD is added to an aqueous solution, the polar water molecules get fit into the lipophilic cavity of CD, but these are immediately replaced by the more favored guest molecule, which is less polar than replaced water molecules (Szejtli, Citation2004). The main driving force behind this complex formation is the replacement of high enthalpy water molecules with the guest moiety, Vander Waals interaction, hydrogen bonding and charge transfer interaction (Loftsson et al., Citation2005b). The ratio in which host and guest bind together is generally 1:1 (Loftsson et al., Citation2005a). The binding strength of the formed complex depends on the ability of the guest molecule to bind well with the host to form a stable complex. The formation of an inclusion complex depends upon following factors:
Size of the CD to the size of the guest molecule or some main functional groups within the guest moiety. If the guest is of inappropriate size, it will not fit properly into the host cavity.
Thermodynamic interactions between the various components of the system (CD, guest, solvent; Del Valle, Citation2004).
Structure of added substituent to the CD derivative.
Location of substituent within the CD molecule.
Number of substituent per CD molecule.
Different techniques used for complex formation include physical mixing, kneading method, spray drying, lyophilization, co-precipitation, solvent evaporation method, neutralization precipitation method, milling method and supercritical anti-solvent technique (Patil et al., Citation2010).
Applications of cyclodextrins
Due to unique property of forming inclusion complexes with diverse molecules, CDs offers versatile benefits in many industries. Applications of CDs are summarized in .
Modifications of existing drug molecules for improved efficacy
A new formulating agent serving dual purpose, i.e. improving the physical properties of new active pharmaceutical ingredient and reformulation of existing drugs is the need of hour in the pharmaceutical industries. CDs have been proven to be better than the existing standard formulating agents. The complexes formed between the active ingredients and CDs show improved stability, solubility and bioavailability with very few adverse effects. CDs are the agents which act as a drug delivery system and also have potential drug delivery profiles in many applications. These properties of CDs are due to the fact that CDs have tendency to modify the physical, chemical and biological properties of guest molecules by formation of inclusion complexes. Due to bio-adaptability and multi-functional property of CDs, there is improvement in the unwanted properties of drug molecules in various routes of administration, including oral, rectal, nasal, ocular, transdermal and dermal (Singh et al., Citation2002). Hence, CDs play a very significant role in the field of pharmaceuticals. The process of inclusion complex formation between the drug and CD and subsequent absorption of drug are depicted in .
Figure 4. Schematic representation of cyclodextrins–drug complexation, subsequent release and absorption of drug.
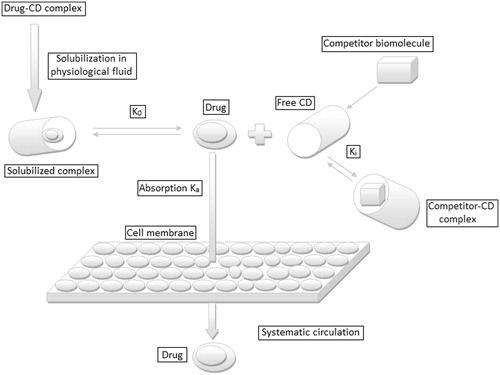
Stability enhancement
CDs play an important role in the chemical stability of drugs. The following properties are concluded after studying the interaction between CDs and labile compounds: (a) CDs retard degradation, (b) CDs have no effect on reactivity or (c) CDs can accelerate drug degradation (Loftsson & Brewster, Citation1996).
CDs are widely known to speed up or slow down the different types of reactions such as hydrolysis, oxidation, photolysis, dehydration and isomerization, based on the characterization of the inclusion complex in aqueous and buffer solutions. This can be well understood by the given examples – in Vaseline and o/w emulsion, the stability of tixoxortol 17-butyrate 21-propionate – a dermocorticoid, was preserved by forming its inclusion complex with β-CD after storing it for 30 days at 40 °C (Matsuda & Arima, Citation1999). In addition, CD derivatives have been proven to better than the parent CDs in showing the stabilizing effects. Derivative of β-CD, O-carboxymethyl-O-ethyl-beta-CD (CME-β-CD) considerably maintained the stability of prostaglandin E (PGE) in an ointment containing fatty alcohol/propylene glycol (FAPG) and in aqueous solution, while the parent β-CD promoted its degradation in neutral and alkaline solutions (Matsuda & Arima, Citation1999).
Solubility and bioavailability improvement
CDs can enhance apparent water solubility by forming dynamic, non-covalent, water-soluble inclusion complexes (Davis & Brewster, Citation2004) as shown in . Enhancement of solubility of the drug further helps in the better bioavailability and hence more therapeutic efficacy of the dosage form. shows various reports on solubility enhancement of therapeutically important pharmaceutics by CD complexation.
Table 3. Various reports on solubility enhancement of therapeutically important pharmaceuticals by CD complexation.
Safety and efficacy betterment
CDs are widely used to alleviate the irritation caused by drugs. The enhanced solubility of drug–CD inclusion complex results in better drug efficacy and potency, which further reduces the drug toxicity since the drug becomes effective at minimum doses. The soluble CD–drug inclusion complex also helps in lowering down the toxicities caused due to the crystallization of poorly water-soluble drugs in case of parenteral formulations. Moreover, drug entrapment in CD cavity at the molecular level protects the biological membranes from direct contact of the drug, which reduces the side effects associated with the drug as well as the local irritation without affecting the therapeutic efficacy of the drug (Rasheed et al., Citation2008).
Drug delivery through biological membranes
The CD permeability through the biological membranes is affected by various factors like its molecular weight, chemical structure and very low octanol/water partition coefficient (log P values) resulting in its inability to cross the biological membranes quickly. The free form of drug, which is not bound to the complex and is in equilibrium with the drug–CD complexes, has the tendency of penetrating the lipophilic membranes. CDs have no role in enhancing the permeability of hydrophilic water-soluble drugs through lipophilic biological membranes. Whether CD increases or decreases the drug delivery across the biological membrane will depend upon the factors like the physicochemical properties of the drug such as its aqueous solubility, the composition of the drug formulation, i.e. aqueous or non-aqueous and physiological composition of the membrane barrier like the presence of an aqueous diffusion layer (Rasheed et al., Citation2008). The drug delivery through aqueous diffusion-controlled barriers can be increased by the use of CDs, but it restricts the drug delivery through lipophilic membrane-controlled barriers. Still, there is an exception to it, i.e. hydrophobic CDs (e.g. methylated β-CDs) can easily pass through mucosa and helps to improve the drug delivery through biological membranes, like the nasal mucosa, by decreasing the barrier property of the membranes (Loftsson et al., Citation2005b).
CD-based drug delivery approaches
Drug delivery through oral route
Among a number of routes available for the drug delivery, oral route has always been the most preferred route for developing a drug delivery system. The release of the drug in oral drug delivery system can be either dissolution controlled, diffusion controlled, osmotically controlled, density controlled or pH controlled. The role of CDs in oral delivery system is as an excipient to carry the drugs across an aqueous phase to the lipophilic absorption surface in the gastrointestinal tract, i.e. forming the inclusion complexes with CDs helps in enhancing the dissolution rate of drugs with low aqueous solubility. Hydrophilic CDs are mostly used for this purpose. In the case of sublingual and buccal routes, the drug–CD complexes with fast dissolution profiles are prepared. A quick rise of drug concentration in the systemic circulation is achieved in sublingual and buccal administration since the issue of hepatic first pass metabolism is resolved via this route (Tiwari et al., Citation2010). The bioavailability of the drug is increased by CDs. This happens because of improved water solubility and drug dissolution rate due to drug–CD complexation. Moreover, they also play an important role as conventional penetration enhancers. However, there is a difference between administration of CD containing formulation via sublingual and oral route. For the drug to show its therapeutic action, it must be released from the inclusion complex before it gets absorbed. This is a bit difficult for sublingual application because a very small amount of aqueous saliva is available for the drug to get dissolve completely and also relatively short residence time. The time for the drug to release from the complex after administration is less since the dissolved drug is removed from the buccal region within few minutes (Rasheed et al., Citation2008).
CDs also help in achieving sustained or site-specific drug release. This can be explained by taking an example of hydrophobic (i.e. lipophilic) CDs like ethylated β-CD and triacetyl-β-CD which are used to get sustained drug release. In addition to this, CDs have also been used in osmotic pumps for controlled drug delivery and in matrix tablets to control solubility-dependent drug release (Okimoto et al., Citation1998). Drug–CD conjugates have also been used to obtain colon-specific drug delivery or sustained plasma levels (Loftsson et al., Citation2004). Many researchers have carried out studies recently to improve oral drug delivery of various drugs, which has been summarized in .
Table 4. Use of cyclodextrin in promoting oral drug delivery.
Drug delivery through rectal route
One of the important applications of CDs is optimizing rectal delivery of drugs meant for systemic use. The action of CD on rectal drug delivery clearly depends upon following factors:
Nature of vehicle used, i.e. hydrophilic or oleaginous.
Physicochemical properties of inclusion complexes formed.
Existence of tertiary excipients like viscous polymer, etc.
In the case of rectal absorption of lipophilic drugs, the improving effects of CDs are commonly dependent on the improved release of vehicles used and the dissolution rates of the drug in rectal fluids, while in the case of rectal delivery of poorly absorbed drugs such as antibiotics, peptides and proteins, the action of CD depends on its direct action on the rectal epithelial cells. Furthermore, the continuous effects of CDs on the levels of drug in blood is due to the sustained release from the vehicles, slower rates of dissolution of drug in the rectal fluid or the obstruction in the rectal absorption of drugs due complex formation having very poor absorption (Matsuda & Arima, Citation1999).
Normally, hydrophilic CDs help in improving the release of drugs with very less water solubility from oleaginous suppository bases because of the minimum interaction of the resulting complexes with the vehicles. The lipophilic drugs become insoluble in hydrophobic vehicles on complexation with hydrophilic CDs, although the complex exists in the form of well-dispersed fine particles in the vehicles. This step serves the dual purpose as it improves the drug dissolution at an interface between the molten bases and the surrounding fluid as well as hinders the reverse diffusion of the drugs back into the vehicles (Rasheed et al., Citation2008). illustrates representative work done in enhancing the rectal drug delivery by using CDs.
Table 5. Representative work done in enhancing the rectal drug delivery using cyclodextrins.
Drug delivery through nasal route
Nasal drug delivery has become an appealing approach for the purpose of systemic delivery of highly potent drugs, which have the issue of low oral bioavailability because of the huge amount of gastrointestinal breakdown and excessive hepatic first-pass metabolism. CDs help in enhancing the nasal absorption of the drugs like peptide and proteins, which have less nasal bioavailability due to their large size and hydrophilic nature, making their transport across the nasal mucosa tough. CD enhances their water solubility by increasing their nasal absorption (Merkus et al., Citation1999). The hydrophobic CDs also have a role as penetration enhancers, particularly in the nasal delivery of peptides. Very less local toxicity of CDs after nasal administration has been reported (Rasheed et al., Citation2008).
CD also serves as a pharmaceutical excipient and acts as a solubilizer and absorption promoter in nasal drug delivery. It is very effective even in a very low concentration and also is inert from a pharmacological–toxicological point of view (Chordiya & Senthilkumaran, Citation2012). Some representative studies, in which CD has played a major role in the drug delivery via nasal route, are given in .
Table 6. Role of cyclodextrins in nasal drug delivery.
Drug delivery through ophthalmic route
The basic remedy to treat the ocular diseases is mainly the drug application topically in the form of aqueous eye drop solutions. Recent reports have proved CDs as useful additives in ophthalmic formulations as they increase the water solubility, aqueous stability and bioavailability of ophthalmic drugs, and helps in decreasing the drug irritation (Loftsson & Jarvinen, Citation1999). The hydrophilic CDs, primarily 2-HP-β-CD and SB-β-CD, have been reported to be nontoxic to the eye and also are well tolerated in aqueous eye drop formulations (Loftsson & Stefansson, Citation2002). Hydrophilic CDs do not have access to the tight biological barriers such as the eye cornea, but increase the ocular bioavailability of lipophilic drugs by keeping the drugs in solution and increasing their availability at the surface of the corneal barrier (Rasheed et al., Citation2008). Very less amount (i.e. <5%) of the drug, which is delivered topically is absorbed into the eye. By the means of CD, solubilization increase in the dose-to-solubility ratio is feasible thereby, facilitating the application of the drugs topically, which were earlier delivered only by systemic routes. CD solubilization of the drug results in the increase in the amount of dissolved drug at the lipophilic membrane surface, but at the same time, if excess of the CD is used, then it will lead to the lowering of the ability of the drug molecules to penetrate into the lipophilic barrier. As a result of this, drug delivery through the cornea is decreased (Loftsson et al., Citation2005b). Some of the recent studies conducted for improvement in drug delivery through ophthalmic route by applying CD–drug complexes are listed in .
Table 7. Application of cyclodextrins in ophthalmic drug delivery.
Drug delivery through dermal route
There is a remarkable safety margin of CD in dermal and transdermal application of drugs meant either for local or systemic use. They have a significant role in improving the solubility as well as stability of drugs in the topical formulations, augmenting the transdermal absorption of drugs, helps in the sustain release of the drug from the vehicle and thus, circumventing the unwanted after effects related with dermally applied drugs. Stratum corneum is the outermost layer of the skin and is the principal hurdle for the absorption of drugs given dermally (Rasheed et al., Citation2008). To reduce its barrier properties, penetration enhancers such as alcohols, fatty acids etc. are used. Due to hydrophobic characteristics, CD-complexes are able to deliver the drug through aqueous diffusion layer only. These complexes cannot penetrate the drug through lipophilic barriers like stratum corneum. Hence, an appropriate aqueous vehicle must be selected for aqueous vehicle must be selected for maximizing the utility of CDs as penetration enhancers. The rate of drug permeability through skin by CD-complexes depend upon two factors; release of drug from an aqueous vehicle; and transport across aqueous diffusion layer on the outer surface of skin. However, if drug permeation and subsequent absorption depend on transport across lipophilic stratum corneum as rate determining factor, then cyclodextrins are not capable to improve the drug delivery (Rasheed et al., Citation2008). Applications of CDs for improving dermal and transdermal drug delivery are shown in .
Table 8. Applications of CDs for improving dermal and transdermal drug delivery.
CDs are also known to enhance the release of drugs from the vehicles by the complexation with the drug. CDs help in easing the skin irritation instigated by drugs by decreasing the extent of free drug occurring due to the inclusion equilibrium (Chordiya & Senthilkumaran, Citation2012). CD discharges certain constituents like proteins, cholesterol and phospholipids with regard to the cutaneous irritation of CDs themselves to skin, from the stratum corneum of skin. As the result of this, a change may occur in the barrier function of skin and permeability of drugs and other xenobiotics. Therefore, special attention should be paid to the possible irritation effects of skin (Matsuda & Arima, Citation1999).
CDs in cosmetics, toiletries and personal care
Recent use of CDs has been discovered in the field of the cosmetic preparations, mainly in vaporization suppression of perfumes, room fresheners and detergents by obtaining the controlled release of fragrances from inclusion complex compounds. The crucial advantages of CDs in this regard are maintenance of stability, odor control and process improvement by converting a liquid ingredient to a solid form (Del Valle, Citation2004).
Following are the major roles of CD in the field of cosmetic preparations.
Shielding from light and oxidation
Presence of CD safeguards cosmetic products from the degredative effects of light and oxidation. In the absence of light and oxygen, pure tea tree oil remains unchanged for a month. Else the skin irritant, p-cymene, is formed because of the reaction between the terpenes present in the oil with light and oxygen. Due to the CD complexation, oil remains stable against light and oxygen. Hence, the possibility of the production of unwanted compounds is circumvented. In addition, there is no change in the antimicrobial and anti-inflammatory properties of tea tree oil after the complex formation (Buschmann & Schollmeyer, Citation2002).
Preventing the loss by evaporation
The phenomenon of complexation by CD aids in the stability of volatile compounds by decreasing their evaporation. The complexes can be added in either powders or liquid formulations. Now a days, even the solid form perfume complexes are used in perfumes. The application of these suspensions on the skin satisfies the demand of a long-lasting effect by slow release of the perfumes (Buschmann & Schollmeyer, Citation2002).
Removal of unpleasant odors
The dry form of CD powders even with the size less than 12 mm are used for odor control in products like diapers, menstrual products and paper towels etc. and are also widely used in hair care preparations for limiting the volatility of odorous mercaptans. Dishwashing and laundry detergents also contain CDs as important constituent, which helps in masking the odors in washed items (Del Valle, Citation2004).
CD complexation eliminates the undesirable odor of dihydroxyacetone which otherwise is very tough to be masked by perfumes. It is used as a tanning agent. Moreover, this CD complexation also causes the slow release of di-hydroxyacetone from the complex due to which there is more uniform tanning of the skin. The product containing this CD complex is marketed as Ultrasun Selftan© (Ultrasun) and in Self-Action Super Tan For Face© (Estee Lauder). CD is also used in deodorants to control the unpleasant smell caused by microbial degradation of sweat (Buschmann & Schollmeyer, Citation2002). Recent reports of cyclodextrins in cosmetics have been given in .
Table 9. Recent reports of cyclodextrins in cosmetics.
CDs in nutrition industry
The food/nutrition industry also demands use of CDs as food additives, for stabilization of flavors, for removal of offensive tastes and other undesirable compounds for instance cholesterol and to get rid of microbiological contaminations and browning reactions (Astray et al., Citation2009). The stability of colorants, flavors, unsaturated fats and vitamins can be elevated by the process of molecular encapsulation of lipophilic food ingredients with CD both in physical and chemical aspect causing prolonged product shelf life (Singh et al., Citation2002). Various experiments were conducted to monitor the effect of CD on the shelf life of the product and it was observed that almost all the natural and synthetic flavors and flavor substances show exceptionally increased shelf life throughout the long-term storage. Along with this, 12 various complexes of natural and synthetic flavor/β-CD have been kept in storage under normal conditions for the time span of 14 years and their actual flavor loads were examined from time to time by GC. Finally, the scientists concluded that the preserving power of the β-CD complexation relies on the structure, polarity and geometry of the different flavor substances. The strongest protection was seen with terpenoid, phenyl-propane and alkylsulfide-type flavors, whereas the flavors of phenolic structure were preserved comparatively to a much lesser amount (Szente & Szejtli, Citation2004).
The use of CDs in food processing and as food additives mainly focuses on the following goals:
Preventing the lipophilic food components that may undergo oxygen and light or heat induced degradation.
To terminate the unpleasant odors or tastes.
Stabilization of fragrances, flavors, vitamins, and essential oils against the undesirable changes.
To obtain a controlled release of certain food constituents.
To promote the solubility of food colorants and vitamins (Astray et al., Citation2009).
β-CD improves the quality and stability of food products by acting as a cholesterol sesquestrant, i.e. it efficaciously removes the cholesterol from animal products thereby, enhancing their nutritional characteristics. In the case of milk, the immobilized β-CD glass beads were prepared by the process of silanization and β-CD immobilization reaction led to cholesterol removal in milk up to 41% and a recycling efficiency of almost 100% (Astray et al., Citation2009). Reduction in cholesterol and decreased oxidation were observed in phytosterol-supplemented mayonnaise with the help of crosslinked β-CD without any change in its physicochemical and sensory properties during storage (Jung et al., Citation2008). When lard was treated under various conditions with 5% crosslinked β-CD cholesterol removal was observed in the range of 91.2–93.0% (Kwak et al., Citation2004).
One of the most important applications of interest of CD is the CD-containing food-packaging materials. CD serves a dual purpose in food-packaging materials. First, all the residual organic volatile contaminants in packaging materials are minimized by CD. Second, the barrier properties of the packaging materials such as diffusion rate and transmission rate are improved by the use of CD further boosting the sensory properties by retaining the food quality and safety. Now a days many CD complexes are employed in foods as antiseptic or conserving agents. For instance, 0.1% iodine-β-CD obstructs the decomposition for 2 months at 20°C in fish paste or in frozen seafood products (Szente & Szejtli, Citation2004).
CD also helps in altering the taste and removal of undesirable bitter tastes and odors of food products. Certain food products have a very bad smell but, on addition of CDs during their manufacturing, inclusion complexes are formed between CD and food components thereby improving the smell of the resultant product. Using the same phenomenon the peculiar odors of food products like fish, soybean milk and soy protein was improved (Astray et al., Citation2009).
The unique property of CD of forming the inclusion complexes helps in the color modification of foods, since they too form complexes with the polyphenol-oxidase enzyme which is the accountable of catalyzing the browning reactions (Astray et al., Citation2009). shows use of cyclodextrin in different food and flavors.
Table 10. Studies on use of cyclodextrins in food and flavors.
Environmental uses of CDs
CDs also have a crucial role to play in the field of environmental science with respect to the enhancement and elimination of organic pollutants and heavy metals from soil, water and atmosphere and solubility of organic contaminants (Gao & Wang, Citation1998). CDs are also put into application in water treatment for enhancing the stabilization aspect, encapsulation and adsorption of contaminants. One can get rid of extremely poisonous and lethal substances from industrial effluent by forming the inclusion complexes utilizing CD (Szejtli, Citation1989). All the aromatic toxic hydrocarbons like phenol, p-chlorophenol and benzene, which are present in wastewaters, are reduced to much lesser extent than its initial amounts after the treatment of wastewaters with β-CD (Singh et al., Citation2002). CDs are also employed in washing away the gaseous effluent from organic chemical industries (Szejtli, Citation1989). Different solubility enhancement techniques of CDs are used in the testing of soil improvement. The deterioration of various classes of hydrocarbons affecting the growth kinetics is promoted by β-CD, resulting in higher biomass yield and superior use of hydrocarbons as a carbon and energy source. β-CD has become the powerful tool for bioremediation process due to its properties such as the low cost, biocompatible and effective degradation (Singh et al., Citation2002).
CDs play a major role in environmental protection, which is its usage in insecticide formulation. CDs are incorporated in the preparation of an insecticide from neem seed extract by forming water-soluble inclusion complex of neem seed kernel extract enclosing azadirachtin-A in a CD carrier molecule. In addition to all its above uses, CDs also helps in the photodegradation process of organophosphorous pesticides in humid water by catalyzing the reaction of pesticides with reactive radicals, which are produced by the humid photosensitizer and inclusion complexed CD (Singh et al., Citation2002).
CDs in textile and packing industry
Since CD has potential to form inclusion complexes with a variety of organic molecules. This property is responsible for its use in different textile applications. CDs can be taken into account as a new category of auxiliary substances for use in the textile industry. Since CD has natural origin and is environmentally safe, so it is considered for textile industry (Voncina, Citation2011).
CD performs the function as retarding reagent in much textile fiber dyeing such as cotton, polyester, polyamide, polypropylene, polyacrylonitrile by complexing with dye molecule, which are released slowly to the fiber thereby, slowing down the dye migration because of the competition for sites on the fiber between CD and dyes irrespective of its mechanism of action. Normally, when CD is used as a leveling agent or a retardant in the dyeing process, superior quality of dyeing together with the improved bath exhaustion is achieved and bath exhaustion in comparison to other already existing marketed auxiliaries; stimulating the better color levelness and some development in color depth when textile fibers are dyed in the presence of CDs. Besides its use in enhancing the quality of dyeing in textile industry, CD also aids in lowering down the environmental damage of exhausted baths. This mainly happens when covalently bonded CDs on textile support form inclusion complexes with organic pollutants. With this, all the adsorbed pollutants will change into water and carbon dioxide by the process known as incineration (Voncina, Citation2011). Superior quality fabrics with novel properties are generated with the help of CDs. The bad smell gained by the fabrics due to cigarette smoke and sweat is concealed by including CD to fabrics.
One of the critical roles of CDs is in the area of packing industry. The presence of CD inclusion complex in the oily antimicrobial and volatile agents helps in its coating on a water-absorbing sheet containing a natural resin binder, which is further used for wrapping fresh products. The usefulness of CD as food-packing material has been confirmed with the manufacturing of food-packaging bag using CD with ethylene-tetracyclo-3-dodecane copolymer and hinokitol as it was odorless and showed good antifungal properties when stored for 1 week at room temperature (Singh et al., Citation2002). Some of the uses of CD in textile industry have been listed in .
Table 11. Cyclodextrins applicability in textile industry.
CDs in separation process
As the CDs efficiently differentiate between various positional isomers, functional groups, homologs and enantiomers, so it is broadly used in separation science. This capability makes them one of the most effective candidates worldwide for a variety of separations (Schneiderman & Stalcup, Citation2000). Their tendency to form inclusion complexes with smaller hydrophobic molecules also contributes to their usage in separation. The factor like shape, size and selectivity of CDs exert the major impact on the separation process (Singh et al., Citation2002). CDs are considered to be the ideal candidate by molecular recognition and further promote the complex forming ability and selectivity in various separations. One of the main uses of CDs is as chemically bonded or sorbed ligands in stationary phase or immobile phase during the separation process. Presently, CDs along with its derivatives are widely used in case of chiral separations. In addition, the hydrophilic CDs have been more commonly used as buffer modifiers in capillary electrophoresis to accomplish the chiral separation of drugs and specialty chemicals (Zhang et al., Citation2011).
Besides, CDs are also greatly used in high-performance liquid chromatography (HPLC) as stationary phases connected to solid support or even as mobile phase additives in HPLC and in capillary electrophoresis to achieve the separation of chiral compounds. CDs also have a role in various chromatographic techniques such as affinity chromatography, capillary zone electrophoresis, ion-exchange, thin layer chromatography, electrokinetic chromatography, microdialysis, isotachophoresis, gel electrophoresis, capillary gas chromatography and separation through membrane. Furthermore, CDs help in the stabilization of an analyte, prevention of non-specific absorption and promoting analyte detection. Besides all the above advantages, CD also aids in microscaling of already used separation technologies; for instance, different capillary techniques including capillary electrophoresis, microbore liquid chromatography and microdialysis. CDs are also employed in bulk scale preparations mainly extractions, dialysis, foam floatation, membrane separation and electrophoresis (De Boer et al., Citation2000). lists few examples of the role of CDs in separation process of various drugs.
Table 12. Role of cyclodextrins in separation process.
CDs as anti-infective agents
Apart from utility of CDs for efficacy enhancement of antibiotics through encapsulation, recently these compounds are reported for direct use as anti-microbial. Anti-infective properties of CDs are mainly attributed to their capability to efficiently block pore forming bacterial toxins because of same symmetry of these molecules as the target pores. Applicability of CDs as anti-infectives and mechanistic insights into inhibition of toxins has been reviewed and reported CD based inhibitors of various bacterial toxins are enlisted recently (Karginov, Citation2013). At least one CD derivative can specifically inhibit various pore forming toxins and thus allows possibilities to develop a novel range of CD derivatives as broad-spectrum antibiotics against various pathogens in near future. shows some uses of CDs for complexation and efficacy improvement of existing anti-infective agents.
Table 13. Cyclodextrin as anti-infectives.
CDs in non-viral gene delivery
Gene delivery is the most promising area of biotechnological research, and has already proved its capability in biomedical applications. The parent CDs are inefficient in complexing with plasmid DNA (pDNA), this drawback of CD limits their transfection efficiency. To overcome this problem, CDs are normally derivatized before using it in gene transfer. This can be well exemplified by CD derivatives polycationic amphiphilic CDs (paCDs; built by the change in the facial anisotropy of the truncated-cone CD by the introducing cationic and hydrophobic elements). By controlling the various molecular parameters such as charge density, hydrophilic–hydrophobic balance, nature of the functional groups and spacer length, the complexing of DNA with CDs can be improved finally, regulating the transfection efficiency of paCDs (Lai, Citation2014).
CDs also have an important role in linking various polymers through covalent linkage forming large molecular structures similar to gene carriers, thereby behaving as a linking agent. This can be well explained by the example of polymers which were synthesized by linkage of low molecular weight poly(ethylenimine) (PEI), a cationic aziridine polymer displaying a high proton buffering capacity over a wide range of pH with β-CD using tosyl chloride, initially generating an amine reactive tosyl deoxy-β-CD, which was later reacted with PEI gives rise to the CD-PEI conjugate (PEI-β-CD; Neu et al., Citation2005). Besides the parent CDs, the derivatives of CDs have also been found to act as linking agents. Their activity as linking agent was confirmed when cross-linking of PEI was done by Huang et al. using 2-HP-β-CD and 2-HP-γ-CD. The two resultant polymers were better in properties as they showed lower cytotoxicity than PEI 25 kDa, with transfection efficiency in SKOV-3 cells approximately 20 and two times greater than that obtained by PEI 600Da and PEI 25 kDa, respectively (Lai, Citation2014).
CDs are also known for its functioning as a structural modulator since they were used to structurally modify already prevailing gene carriers. In this, CD can fulfill the function of both as a threading device as well as that of pendants. The role of CD as threading agent can be better explained by the experiments conducted by Li’s group, who have produced large number of supramolecular polyrotaxanes, which comprise of cationic α-CD rings threaded and blocked on a poly [(ethylene oxide)-ran-(propylene oxide)] [P (EO-r-PO)] random copolymer. The function of CDs as pendants can be explained by taking the example of vectors produced by this method is the polyamidoamine (PAMAM) dendrimer conjugates with all the three parent CDs (Lai, Citation2014). Some of the applications of CD in non-viral gene delivery are given in .
Table 14. Use of cyclodextrins in non-viral gene delivery.
CDs in bioconversion process
The proficiency of bioconversion process suffers from certain constraints due to the inhibitory or toxic influences from either the substrate or product on the biocatalyst. Likewise, another hindrance to this process is that the biocatalyst is most active in its natural environment, mainly an aqueous medium, despite the fact that the majority of organic substrates are lipophilic in nature and sparingly soluble in water. As a result, very less amount of substrate is available to the biocatalyst. Therefore, one of the most suitable approach to overcome these inadequacies involves the addition of CD in bioconversion. CDs have tendency of enhancing the solubilization of organic compounds, reducing the toxicity by complexation with toxins and the biocompatibility. Representative studies on efficiency enhancement of bioconversion processes using CDs are given in .
Table 15. Use of cyclodextrins in bioconversion process.
CDs in plant cell technology
Precursor feeding has been an evident and widespread approach to increase secondary metabolite production in plant cell cultures. The concept is based upon the idea that any compound, which is an intermediate in or at the beginning of a secondary metabolite biosynthetic route, stands a good chance of increasing the yield of the final product (Rao & Ravishankar, Citation2002). The increase in product content is probably due to enhanced availability of precursor to the cells in a more dissolved state due to CD complexation which resulted in more conversion to product. However, most of these intermediates of biosynthetic pathway are poorly soluble in aqueous media and therefore are less accessible for bioconversion to the desired product due to their lipophilic nature in plant cell cultures. This property confines their use as precursors to enhance productivity. Many plant cell cultures barely convert precursors in the presence of organic phases, often as a result of dramatic decrease of cell viability and reduced enzymatic activities in these systems. In order to overcome these problems and enhance availability of water insoluble precursors, complexation with cyclodextrin has been efficaciously used as a novel approach. This method has advantages of both apolar systems (higher solubility of substrate) and aqueous systems (maintenance of cell viability). The culture characteristics, i.e. cell growth and pH does not differ when only CDs are added in cultures (Baldi, Citation2008). The CDs are also reported not to be metabolized by plant cell enzymes or to serve as a carbon source (Woerdenbag et al., Citation1990). Few of the studies involving CDs for precursor availability enhancement in plant cell cultures are given in .
Table 16. Cyclodextrins in plant cell technology.
CDs in catalysis
One of the most promising role of CDs is in the catalytic reactions. They have the capability to function as enzyme mimics. These are fabricated by changing the naturally occurring CDs with the substitution on its primary or secondary face with different functional groups or attaching various reactive groups. The substituted groups attached to the CD molecule are responsible for the molecular recognition which makes the modified CDs beneficial as enzyme mimics (Szejtli, Citation1998). Morozumi et al. (1991) made use of modified β-CD in the catalysis and further employed it for the selective hydroxyl ethylation and hydroxyl methylation of phenol. This chemical modification significantly enhanced the catalytic activity with the resultant CD derivative acting as a transamine mimic and acting as a catalyst for the reaction in which the phenylpyruvic acid is converted to phenylalanine (Singh et al., Citation2002).
The steric effects shown by CDs are held responsible for its role in biocatalytic reactions. It acts by improving the enantio-selectivity. The catalytic reaction can be completely stopped or can be improved in a better way by the participation of CD as it gets involved with the catalyst by forming inclusion complexes (Singh et al., Citation2002).
Toxicity considerations
It has been revealed from the various studies carried out on CD and its derivatives that very limited number of hydrophilic CDs and drug/CD complexes formed can pass through the hydrophobic membranes like gastrointestinal mucosa and skin. Due to this, they exhibit very less absorption hence having no toxic effects inside the body. Therefore, these are safe to administer orally. Unlike hydrophilic CDs, the lipophilic methylated β-CDs can permeate easily through the biological membranes thereby, show better absorption approximately equivalent to 10% from the GIT. As a result of this, oral formulations should contain less amount of hydrophobic CD derivatives to completely get rid of the possibility of adverse effects, which may be caused because of these lipophilic CDs. These CDs are incompatible for parenteral formulations (Loftsson & Duchene, Citation2007). The toxicity studies conducted on different CDs have disclosed that α-CD and β-CD are unfit for incorporation in the parenteral preparations so their utilization via this route should be strictly restricted (Irie & Uekama, Citation1997).
Furthermore, during the animal studies, it was observed that administration of γ-CD intravenously did not show any significant toxic effects. Large-scale toxicology studies were compiled on two main extensively used CD derivatives, i.e. 2-HP-β-CD and SBE β-CD. There are many marketed formulations available containing significant amount of these two CD derivatives (Loftsson & Duchene, Citation2007). 2-HP-β-CD is another better option other than α-, β- and γ-CD, which can be considered for the study as it possess improved aqueous solubility combined with very less toxic effects. Various animal studies done on HP-β-CD using animals such as rats, dogs and mice reached at a conclusion that the oral administration of HP-β-CD is well tolerated in these animals tested with negligible toxic effects even at daily oral dosing of <16 g for at least 14 days (Gould & Scott, Citation2005).
Market potential and future prospects of CDs
Besides the vast applications of CDs at the present time, CD has ample scope in future also involving the evolution of new CD derivatives as well as the demand of already existing derivatives. Till date, there are more than 50 marketed formulations, which contain CD complexes with drugs and a range of excipients are available commercially (Szejtli, Citation2004; Loftsson & Brewster, Citation2010; Kumar Das et al., Citation2013). Some representative examples are:
The oral formulation of the drug limaprost complexed with α-CD is marketed under the trade names of Opalmon and Prorenal (Davis & Brewster, Citation2004).
The sublingual preparation of nitroglycerin containing β-CD is in the market under the trade name of Nitropen in Japan (Zhang & Rees, Citation1999).
The complex of piroxicam β-CD given via oral route is sold in Europe under the trade name of Brexin (Brewster & Loftsson, Citation2007).
The intravenous formulation containing inclusion complex of voriconazole and sulfobutylether β-CD is sold in countries such as Europe, United States under trade name of Vfend (Loftsson & Duchene, Citation2007).
Sporanox is the marketed formulation of Europe and United States taken orally as well as intravenously containing complex of drug, itraconazole and 2-HP-β-CD (Kumar Das et al., Citation2013).
For future prospective, CDs might prove to be beneficial to introduce some better changes with respect to formulation or it may help in drug delivery of protein, peptide and oligonucleotide dosage forms. The problem of agitation-associated aggregation in a mixture of a number of proteins, including human growth hormone, as well as lyophilization related aggregation of interleukin-2 (IL-2) can be overcome by the use of HP-β-CD together with other hydrophilic and lipophilic CD derivatives (Davis & Brewster, Citation2004). The physical and chemical deterioration of insulin formulation can be prevented by the use of CDs (Brewster et al., Citation1989). Therefore, it is responsible for the stabilization of insulin formulations. This is mainly related to the fact that by the interactions with readily available hydrophobic amino acid residues CDs stabilizes the protein conformation. CDs are also known to interact with aggregated/denaturated proteins resulting in natural refolding, thus acting like an artificial chaperones. One more future application of CD lies in its role in enhancing the cellular delivery of oligonucleotides. The uptake of phosphorothiolate oligodeoxynucleotides by human T-cell leukemia cells (H9) is upgraded several folds with the use of HP-β-CD. Moreover, ability of CD to complex is helpful in decreasing the immunogenicity of oligonucleotides as well other undesirable side effects of oligonucleotide administration comprising of the effects of macromolecules on platelet count. Besides its increasing demand as drug carriers, CDs are helpful in extraction of various compounds. Efforts have been made to merge CDs together with polymers and small molecule drugs to limit the amount of CD used along with the release rates of the drug. Biodegradable polymers including complexes of CDs and antibiotics have been formulated and these have showed promising results in the production of bacteria-resistant films and fibers. More advancement is occurring, most important being the discovery of many new CD derivatives. Recently, CDs consisting of quaternary amine groups have been found to act as multifunctional agents in ophthalmic formulations. Earlier also, CDs were included in eye drops as an important ingredient, e.g. eye drops of 2-HP-β-CD complexed indomethacin and RAME-β-CD complexed chloramphenicol, which contain as a complexing agent (Loftsson & Stefansson, Citation2002; Halim Mohamed & Mahmoud, Citation2011). Despite of advancements for more than 100 years and great commercial success, CDs still holds many unexplored potential in diverse facets of science and technology, which should be tapped in future by researcher across the globe.
Conclusion
CDs have been known worldwide and are very beneficial additive used in the pharmaceutical and other industries. All the advantages of the CD have been attributed to its tendency of complexing with varied drug molecules resulting in the formation of inclusion complexes. This interaction between CD and drug is host–guest type, i.e. it acts like a host and drug molecule (guest) gets partially or completely fit into its lipophilic cavity to form a complex. This single interaction is sufficient to change the physiochemical and pharmaceutical properties of the drug molecule in the form of enhanced water solubility of drugs further improving the bioavailability of the drug, better stabilization of drugs under the conditions such as light, heat and oxidizing conditions, i.e. avoids the deterioration of drugs in these sources and also reduction in the volatility of drugs. Therefore, all these improvements brought about in the drug characteristics depict the great potential of the CD as most advanced drug delivery system. Despite the fact that CDs are known and are used pharmaceutically since decades, in the recent times many more applications of CD have emerged apart from its usage in enhancing solubility and maintaining the stability of various drugs. CDs have a function as penetration enhancer. The hydrophobic CDs help in the permeation of the drugs through the biological membranes. One of the most important applications of CD known from the different experiments carried out in humans and animals as well is enhancing the drug delivery of majority of the formulations. CDs help in the delivery of sustained release preparations to be delivered via different possible routes. Most of the research conducted has revealed that the hydrophobic and ionizable CDs derivatives are mainly used as drug carriers in the delivery of sustained-release or delayed release formulations. The property of CDs to complex with various drugs is the ultimate solution to the various issues related to drug delivery. In addition to all these aspects, gene therapy continues to develop the interest of researchers. For the non-viral delivery of nucleic acids, CD containing polycations are used. Even though these agents have given promising results for gene delivery in animals, but no conclusions have been made in case of humans. CDs are also known for acting as molecular chelating agents with huge demand in the area of food, pharmaceuticals and chromatographic techniques established. CDs are also known worldwide for its role as toxicity modifier. CDs have improved biocatalysis reactions by increasing enantio-selectivity. Seeing such vast applications of CDs in number of bioprocesses, further survey and research on CDs should be done at small as well as on large scale to investigate its role in various other fields. Hence, it can be concluded that because of its distinctive structural features, CDs are the most demandable and important requirement of the researchers in the drug development, in various separation procedures, as enzyme mimics and most importantly as a complexing agent in pharmaceutical, food, cosmetic and many other industries.
Acknowledgements
We are thankful to Praveen Garg, Chairman, ISFCP, Moga, Punjab for providing necessary facilities.
Declaration of interest
The authors report no declarations/conflicts of interest. Financial assistance provided by Punjab State Council for Science and Technology, Chandigarh is gratefully acknowledged.
References
- Al Omari MM, Badwan AA, Zughul MB, et al. (2007). Fexofenadine/cyclodextrin inclusion complexation: phase solubility, thermodynamic, physicochemical, and computational analysis. Drug Dev Ind Pharm 33:1205–15
- Aleem O, Kuchekar B, Pore Y, Late S. (2008). Effect of β-cyclodextrin and hydroxypropyl β-cyclodextrin complexation on physicochemical properties and antimicrobial activity of cefdinir. J Pharm Biomed Anal 47:535–40
- Ali N, Harikumar SL, Kaur A. (2012). Cyclodextrin: an excipient tool in drug delivery. Int J Pharm 3:44–50
- Almagro L, Perez AL, Pedreno M. (2011). New method to enhance ajmalicine production in Catharanthus roseus cell cultures based on the use of cyclodextrins. Biotechnol Lett 33:381–5
- Ammar H, Salama H, Ghorab M, et al. (2006). A transdermal delivery system for glipizide. Curr Drug Deliv 3:333–41
- Ansari MT, Batty KT, Iqbal I, Sunderland VB. (2011). Improving the solubility and bioavailability of dihydroartemisinin by solid dispersions and inclusion complexes. Arch Pharmacol Res 34:757–65
- Aranda C, Urbiola K, Mendez Ardoy A, et al. (2013). Targeted gene delivery by new folate-polycationic amphiphilic cyclodextrins-DNA nanocomplexes in vitro and in vivo. Eur J Pharm Biopharm 85:390–7
- Arima H, Kondo T, Irie T, Uekama K. (1992). Enhanced rectal absorption and reduced local irritation of the anti-inflammatory drug ethyl 4-biphenylylacetate in rats by complexation with water-soluble beta-cyclodextrin derivatives and formulation as oleaginous suppository. J Pharm Sci 11:1119–25
- Arima H, Yamashita S, Mori Y, et al. (2010). In vitro and in vivo gene delivery mediated by lactosylated dendrimer/alpha-cyclodextrin conjugates (G2) into hepatocytes. J Control Release 146:106–17
- Astray G, Gonzalez-Barreiro C, Mejuto J, et al. (2009). A review on the use of cyclodextrins in foods. Food Hydrocolloids 23:1631–40
- Awasthi SS, Kumar TG, Manisha P, et al. (2011). Development of meloxicam formulations utilizing ternary complexation for solubility enhancement. Pakistan J Pharm Sci 24:533–8
- Babu R, Pandit J. (2004). Effect of cyclodextrins on the complexation and transdermal delivery of bupranolol through rat skin. Int J Pharm 271:155–65
- Babu RJ, Dayal P, Singh M. (2008). Effect of cyclodextrins on the complexation and nasal permeation of melatonin. Drug Deliv 15:381–8
- Baek JS, Lim JH, Kang JS, et al. (2013). Enhanced transdermal drug delivery of zaltoprofen using a novel formulation. Int J Pharm 453:358–62
- Baldi A. (2008). Production of anticancer drug podophyllotoxin by plant cell cultivation of Linum album [Ph.D]. Thesis, Department of Biochemical Engineering & Biotechnology. Indian Institute of Technology, Delhi
- Balducci AG, Magosso E, Colombo G, et al. (2013). Agglomerated oral dosage forms of artemisinin/β-cyclodextrin spray-dried primary microparticles showing increased dissolution rate and bioavailability. Pharm Sci Tech 14:911–18
- Becket G, Schep LJ, Tan MY. (1999). Improvement of the in vitro dissolution of praziquantel by complexation with alpha-, beta- and gamma-cyclodextrins. Int J Pharm 179:65–71
- Belchi Navarro S, Almagro L, Lijavetzky D, et al. (2012). Enhanced extracellular production of trans-resveratrol in Vitis vinifera suspension cultured cells by using cyclodextrins and methyljasmonate. Plant Cell Rep 31:81–9
- Borghetti GS, Pinto AP, Lula IS, et al. (2011). Daidzein/cyclodextrin/hydrophilic polymer ternary systems. Drug Dev Ind Pharm 37:886–93
- Brewster ME, Loftsson T. (2007). Cyclodextrins as pharmaceutical solubilizers. Adv Drug Del Rev 59:645–66
- Brewster ME, Simpkins JW, Hora MS, et al. (1989). The potential use of cyclodextrins in parenteral formulations. J Pharm Sci Technol 43:231–40
- Buschmann HJ, Schollmeyer E. (2002). Applications of cyclodextrins in cosmetic products: a review. J Cosmet Sci 53:185–92
- Cal K, Centkowska K. (2008). Use of cyclodextrins in topical formulations: practical aspects. Eur J Pharm Biopharm 68:467–78
- Cappello B, Di Maio C, Iervolino M, et al. (2009). Etodolac/cyclodextrin formulations: Physicochemical characterization and in vivo pharmacological studies. Drug Dev Ind Pharm 35:877–86
- Challa R, Ahuja A, Ali J, Khar RK. (2005). Cyclodextrins in drug delivery: an updated review. Pharm Sci Tech 6:329–57
- Chattah AK, Mroue KH, Pfund LY, et al. (2013). Insights into novel supramolecular complexes of two solid forms of norfloxacin and β-cyclodextrin. J Pharm Sci 102:3717–24
- Chordiya MA, Senthilkumaran K. (2012). Cyclodextrin in drug delivery: a review. Res Rev J Pharm Pharm Sci 1:19–29
- Davis ME, Brewster ME. (2004). Cyclodextrin-based pharmaceutics: past, present and future. Nat Rev Drug Discov 3:1023–35
- De Boer T, De Zeeuw RA, De Jong GJ, Ensing K. (2000). Recent innovations in the use of charged cyclodextrins in capillary electrophoresis for chiral separations in pharmaceutical analysis. Electrophoresis 21:3220–39
- Del Valle EM. (2004). Cyclodextrins and their uses: a review. Process Biochem 39:1033–46
- Dos Santos C, Buera MP, Mazzobre MF. (2011). Phase solubility studies and stability of cholesterol/β-cyclodextrin inclusion complexes. J Sci Food Agric 91:2551–7
- Durante M, Caretto S, Quarta A, et al. (2011). β-Cyclodextrins enhance artemisinin production in Artemisia annua suspension cell cultures. Appl Microbiol Biotechnol 90:1905–13
- El Ghoul Y, Blanchemain N, Laurent T, et al. (2008). Chemical, biological and microbiological evaluation of cyclodextrin finished polyamide inguinal meshes. Acta Biomater 4:1392–400
- El Harti J, Cherrah Y, Bouklouze A. (2012). Improvement of water solubility of josamycin by inclusion complex γ-cyclodextrin. Anal Chem 2012:1–6
- Figueiras A, Carvalho RA, Ribeiro L, et al. (2007). Solid-state characterization and dissolution profiles of the inclusion complexes of omeprazole with native and chemically modified β-cyclodextrin. Eur J Pharm Biopharm 67:531–9
- Gao S, Wang L. (1998). Application of cyclodextrin in environmental science. Huanjing KexueJinzhan 6:80–6
- Garnero C, Chattah AK, Longhi M. (2014). Improving furosemide polymorphs properties through supramolecular complexes of β-cyclodextrin. J Pharm Biomed Anal 95:139–45
- Gould S, Scott RC. (2005). 2-Hydroxypropyl-b-cyclodextrin (HP-b-CD): a toxicology review. Food Chem Toxicol 43:1451–9
- Gudmundsdottir E, Stefansson E, Bjarnadottir G, et al. (2000). Methazolamide 1% in cyclodextrin solution lowers IOP in human ocular hypertension. Invest Ophthalmol Vis Sci 41:3552–4
- Halim Mohamed MA, Mahmoud AA. (2011). Formulation of indomethacin eye drops via complexation with cyclodextrins. Curr Eye Res 36:208–16
- Han C, Xu J, Wang X, et al. (2014). Enantioseparation of racemic trans-δ-viniferin using high speed counter-current chromatography based on induced circular dichroism technology. J Chromatogr A 1324:164–70
- Ibrahim N, Abdalla W, El-Zairy E, Khalil H. (2013a). Utilization of monochloro-triazine β-cyclodextrin for enhancing printability and functionality of wool. Carbohydr Polym 92:1520–9
- Ibrahim N, El-Zairy E, Abdalla W, Khalil H. (2013b). Combined UV-protecting and reactive printing of Cellulosic/wool blends. Carbohydr Polym 92:1386–94
- Irie T, Uekama K. (1997). Pharmaceutical applications of cyclodextrins. III. Toxicological issues and safety evaluation. J Pharm Sci 86:147–62
- Jagdale SC, Mohanty P, Chabukswar AR, Kuchekar BS. (2012). Dissolution rate enhancement, design and development of buccal drug delivery of darifenacin hydroxypropyl β-cyclodextrin inclusion complexes. J Pharm 2013:1–11
- Jarosz PA, Fletcher E, Elserafy E, et al. (2013). The effect of α-cyclodextrin on postprandial lipid and glycemic responses to a fat-containing meal. Metabolism 62:1443–7
- Jug M, Becirevic-Lacan M, Kwokal A, Cetina-Cizmek B. (2005). Influence of cyclodextrin complexation on piroxicam gel formulations. Acta Pharma 55:223–36
- Jug M, Mennini N, Kover KE, Mura P. (2014). Comparative analysis of binary and ternary cyclodextrin complexes with econazole nitrate in solution and in solid state. J Pharm Biomed Anal 91:81–91
- Jung TH, Ha HJ, Ahn J, Kwak HS. (2008). Development of cholesterol-reduced mayonnaise with crosslinked beta-cyclodextrin and added phytosterol. Korean J Food Sci Anim Resources 28:211–17
- Kane RN, Kuchekar BS. (2010). Preparation, physicochemical characterization, dissolution and formulation studies of telmisartan cyclodextrin inclusion complexes. Asian J Pharm 4:52–9
- Karathanos VT, Mourtzinos I, Yannakopoulou K, Andrikopoulos NK. (2007). Study of the solubility, antioxidant activity and structure of inclusion complex of vanillin with β-cyclodextrin. Food Chem 101:652–8
- Karginov VA. (2013). Cyclodextrin derivatives as anti-infectives. Curr Opin Pharmacol 13:717–25
- Kasliwal N, Negi JS. (2011). Development, characterization and performance evaluation of oro-dispersible tablet containing aceclofenac hydroxypropyl-β-cyclodextrin binary system. J Incl Phenom Macro Chem 71:215–24
- Kaur N, Puri R, Jain SK. (2010). Drug-cyclodextrin-vesicles dual carrier approach for skin targeting of anti-acne agent. Pharm Sci Tech 11:528–37
- Kondo T, Irie T, Uekama K. (1996). Combination effects of alpha-cyclodextrin and xanthan gum on rectal absorption and metabolism of morphine from hollow-type suppositories in rabbits. Biol Pharm Bull 2:280–6
- Kumar Das S, Rajabalaya R, David S, et al. (2013). Cyclodextrins – the molecular container. Res J Pharm Biol Chem Sci 4:1694–720
- Kwak H, Kim S, Kim J, et al. (2004). Immobilized β-cyclodextrin as a simple and recyclable method for cholesterol removal in milk. Arch Pharmacol Res 27:873–7
- Lai WF. (2014). Cyclodextrins in non-viral gene delivery. Biomaterials 35:401–11
- Lakshmi PJ, Deepthi B, Rama Rao N. (2012). Rectal drug delivery: a promising route for enhancing drug absorption. Asian J Res Pharm Sci 2:143–9
- Li W, Tan G, Zhao L, et al. (2012). Computer-aided molecular modeling study of enantioseparation of iodiconazole and structurally related triadimenol analogues by capillary electrophoresis: chiral recognition mechanism and mathematical model for predicting chiral separation. Anal Chim Acta 718:138–47
- Loftsson T, Brewster ME, Masson M. (2004). Role of cyclodextrins in improving oral drug delivery. Am J Drug Deliv 2:261–75
- Loftsson T, Brewster ME. (1996). Pharmaceutical applications of cyclodextrins. 1. Drug solubilization and stabilization. J Pharm Sci 85:1017–25
- Loftsson T, Brewster ME. (2010). Pharmaceutical applications of cyclodextrins: basic science and product development. J Pharm Pharmacol 62:1607–21
- Loftsson T, Duchene D. (2007). Cyclodextrins and their pharmaceutical applications. Int J Pharm 329:1–11
- Loftsson T, Hreinsdottir D, Masson M. (2005a). Evaluation of cyclodextrin solubilization of drugs. Int J Pharm 302:18–28
- Loftsson T, Jarho P, Másson M, Järvinen T. (2005b). Cyclodextrins in drug delivery. Expert Opin Drug Deliv 2:335–51
- Loftsson T, Stefansson E. (2002). Cyclodextrins in eye drop formulations: enhanced topical delivery of corticosteroids to the eye. Acta Ophthalmol Scand 80:144–50
- Loftsson T, Jarvinen T. (1999). Cyclodextrins in ophthalmic drug delivery. Adv Drug Deliv Rev 36:59–79
- Mahmoud AA, El-Feky GS, Kamel R, Awad GE. (2011). Chitosan/sulfobutylether-β-cyclodextrin nanoparticles as a potential approach for ocular drug delivery. Int J Pharm 413:229–36
- Matsuda H, Arima H. (1999). Cyclodextrins in transdermal and rectal delivery. Adv Drug Deliv Rev 36:81–99
- Merkus F, Verhoef J, Marttin E, et al. (1999). Cyclodextrins in nasal drug delivery. Adv Drug Deliv Rev 36:41–57
- Miletic T, Kyriakos K, Graovac A, Ibric S. (2013). Spray-dried voriconazole–cyclodextrin complexes: solubility, dissolution rate and chemical stability. Carbohydr Polym 98:122–31
- Miyake K, Arima H, Hirayama F, et al. (2000). Improvement of solubility and oral bioavailability of rutin by complexation with 2-hydroxypropyl-β-cyclodextrin. Pharm Dev Technol 5:399–407
- Miyake K, Irie T, Arima H, et al. (1999). Characterization of itraconazole/2-hydroxypropyl-β-cyclodextrin inclusion complex in aqueous propylene glycol solution. Int J Pharm 179:237–45
- Monteiro M, Ozzetti RA, Vergnanini AL, et al. (2011). Evaluation of octyl p-methoxycinnamate included in liposomes and cyclodextrins in anti-solar preparations: preparations, characterizations and in vitro penetration studies. Int J Nanomed 7:3045–58
- Moriwaki C, Costa G, Ferracini C, et al. (2008). Enhancement of solubility of albendazole by complexation with β-cyclodextrin. Braz J Chem Eng 25:255–67
- Morozumi T, Uetsuki H, Komiyama M, et al. (1991) Selective synthesis using cyclodextrins as catalyst: Part 6. Cyclodextrin modification for para selctive hydroxymethylation and hydroxyethylation of phenol. J Mol Catal 70:399–406
- Navarro P, Nicolas TS, Gabaldon JA, et al. (2011). Effects of cyclodextrin type on vitamin C, antioxidant activity, and sensory attributes of a mandarin juice enriched with pomegranate and goji berries. J Food Sci 76:S319–24
- Negi JS, Singh S. (2013). Spectroscopic investigation on the inclusion complex formation between amisulpride and γ-cyclodextrin. Carbohydr Polym 92:1835–43
- Neu M, Fischer D, Kissel T. (2005). Recent advances in rational gene transfer vector design based on poly (ethylene imine) and its derivatives. J Gene Med 7:992–1009
- Nicolescu C, Arama C, Nedelcu A, Monciu CM. (2010). Phase solubility studies of the inclusion complexes of repaglinide with β-cyclodextrin and β-cyclodextrin derivatives. Farmacia 58:620–8
- Nitalikar MM, Sakarkar DM, Jain PV. (2012). The cyclodextrins: a review. J Curr Pharm Res 10:1–6
- Ogawa N, Kaga M, Endo T, et al. (2012). Quetiapine free base complexed with cyclodextrins to improve solubility for parenteral use. Chem Pharm Bull 61: 809–15
- Okimoto K, Miyake M, Ohnishi N, et al. (1998). Design and evaluation of an osmotic pump tablet (OPT) for prednisolone, a poorly water soluble drug, using (SBE) 7m-β-CD. Pharm Res 15:1562–8
- Ozdemir N, Erkin J. (2012). Enhancement of dissolution rate and bioavailability of sulfamethoxazole by complexation with β-cyclodextrin. Drug Dev Ind Pharm 38:331–40
- Ozkan Y, Atay T, DiKmen N, et al. (2000). Improvement of water solubility and in vitro dissolution rate of gliclazide by complexation with β-cyclodextrin. Pharm Acta Helv 74:365–70
- Palem CR, Patel S, Pokharkar VB. (2009). Solubility and stability enhancement of atorvastatin by cyclodextrin complexation. J Pharm Sci Technol 63:217–25
- Patil JS, Kadam D, Marapur S, Kamalapur M. (2010). Inclusion complex system: a novel technique to improve the solubility and bioavailability of poorly soluble drugs: a review. Int J Pharm Sci Rev Res 2:29–34
- Radu CD, Salariu M, Avadanei M, et al. (2013). Cotton-made cellulose support for anti-allergic pajamas. Carbohydr Polym 95:479–86
- Rajendrakumar K, Madhusudan S, Pralhad T. (2005). Cyclodextrin complexes of valdecoxib: properties and anti-inflammatory activity in rat. Eur J Pharm Biopharm 60:39–46
- Rao SR, Ravishankar GA. (2002). Plant cell cultures: chemical factories of secondary metabolites. Biotechnol Adv 20:101–53
- Rasheed A, Kumar A, Sravanthi V. (2008). Cyclodextrins as drug carrier molecule: a review. Sci Pharm 76:567–98
- Rawat S, Jain S. (2003). Rofecoxib–cyclodextrin inclusion complex for solubility enhancement. Die Pharmazie Int J Pharm Sci 58:639–41
- Riekes MK, Tagliari MP, Granada A, et al. (2010). Enhanced solubility and dissolution rate of amiodarone by complexation with β-cyclodextrin through different methods. Mater Sci Eng C 30:1008–13
- Rouf MA, Vural I, Bilensoy E, et al. (2011). Rapamycin-cyclodextrin complexation: improved solubility and dissolution rate. J Incl Phenom Macro Chem 70:167–75
- Sachan NK, Pushkar S, Solanki S, Bhatere D. (2010). Enhancement of solubility of acyclovir by solid dispersion and inclusion complexation methods. World Appl Sci J 11:857–64
- Schneiderman E, Stalcup AM. (2000). Cyclodextrins: a versatile tool in separation science. J Chromatogr B Biomed Sci Appl 745:83–102
- Shokri J, Hasanzadeh D, Ghanbarzadeh S, et al. (2013). The effect of beta-cyclodextrin on percutaneous absorption of commonly used Eusolex® sunscreens. Drug Res 63:591–6
- Singer Y, Shity H, Bar R. (1991). Microbial transformations in a cyclodextrin medium. Part 2. Reduction of androstenedione to testosterone by Saccharomyces cerevisiae. Appl Microbial Biotechnol 35:731–7
- Singh M, Sharma R, Banerjee U. (2002). Biotechnological applications of cyclodextrins. Biotechnol Adv 20:341–59
- Singh R, Bharti N, Madan J, Hiremath S. (2010). Characterization of cyclodextrin inclusion complexes – a review. J Pharm Sci Technol 2:171–83
- Sridevi S, Diwan P. (2002). Effect of pH and complexation on transdermal permeation of gliquidone. Die Pharmazie 57:632–4
- Szejtli J. (1989). Downstream processing using cyclodextrins. Trends Biotechnol 7:170–4
- Szejtli J. (1998). Introduction and general overview of cyclodextrin chemistry. Chem Rev 98:1743–54
- Szejtli J. (2004). Past, present and future of cyclodextrin research. Pure Appl Chem 76:1825–45
- Szente L, Szejtli J. (1999). Highly soluble cyclodextrin derivatives: chemistry, properties and trends in development. Adv Drug Deliv Rev 36:17–28
- Szente L, Szejtli J. (2004). Cyclodextrins as food ingredients. Trends Food Sci Technol 15:137–42
- Tamamoto LC, Schmidt SJ, Lee SY. (2010). Sensory properties of ginseng solutions modified by masking agents. J Food Sci 75:S341–7
- Tewes F, Brillault J, Couet W, Olivier JC. (2008). Formulation of rifampicin-cyclodextrin complexes for lung nebulization. J Control Release 129:93–9
- Tiwari G, Tiwari R, Rai AK. (2010). Cyclodextrins in delivery systems: applications. J Pharm Bio Allied Sci 2:72–9
- Voncina B. (2011). Application of cyclodextrins in textile dyeing. Textile dyeing. Rijeka: In Tech, 373–92
- Wang S, Li D, Ito Y, et al. (2004). An ocular drug delivery system containing zinc diethyl dithiocarbamate and HPβCD inclusion complex-corneal permeability, anti-cataract effects and mechanism studies. J Pharm Pharmacol 56:1251–7
- Wang Y, Qiao X, Li W, et al. (2009). Study on the complexation of isoquercitrin with β-cyclodextrin and its derivatives by spectroscopy. Anal Chimi Acta 650:124–30
- Weisheimer V, Miron D, Silva C, et al. (2010). Microparticles containing lemongrass volatile oil: Preparation, characterization and thermal stability. Die Pharmazie Int J Pharm Sci 65:885–90
- Wempe MF, Wacher VJ, Ruble KM, et al. (2008). Pharmacokinetics of raloxifene in male Wistar–Hannover rats: influence of complexation with hydroxybutenyl-beta-cyclodextrin. Int J Pharm 346:25–37
- Wen J, Liu B, Yuan E, et al. (2010). Preparation and physicochemical properties of the complex of naringenin with hydroxypropyl-β-cyclodextrin. Molecules 15:4401–7
- Woerdenbag HJ, Pras N, Frijlink HW, et al. (1990). Cyclodextrin-facilitated bioconversion of 17 beta-estradiol by a phenoloxidase from Mucuna pruriens cell cultures. Phytochemistry 29:1551–4
- Wu J, Shen Q, Fang L. (2013). Sulfobutylether-β-cyclodextrin/chitosan nanoparticles enhance the oral permeability and bioavailability of docetaxel. Drug Dev Ind Pharm 39:1010–19
- Wu Y, Jiang F, Chen L, et al. (2011). Determination of phenylenediamine isomers in hair dyes by coal cinders micro-column extraction and MEKC. Anal Bioanal Chem 400:2141–7
- Xing J, Chen L, Song J, et al. (2012). Separation and determination of resibufogenin and cinobufagin in Chansu using reversed-phase liquid chromatography with γ-cyclodextrin as mobile-phase modifier. J Sep Sci 35:1884–92
- Yang B, Lin J, Chen Y, Liu Y. (2009). Artemether/hydroxypropyl-β-cyclodextrin host–guest system: characterization, phase-solubility and inclusion mode. Bioorg Med Chem 17:6311–17
- Young O, Gupta R, Sadooghy-Saraby S. (2012). Effects of cyclodextrins on the flavor of goat milk and its yogurt. J Food Sci 77:S122–7
- Zhang J, Shen X, Chen Q. (2011). Separation processes in the presence of cyclodextrins using molecular imprinting technology and ionic liquid cooperating approach. Curr Org Chem 15:74–85
- Zhang MQ, Rees DC. (1999). A review of recent applications of cyclodextrins for drug discovery. Expert Opin Ther Pat 9:1697–717
- Zhao MX, Li JM, Du L, et al. (2011). Targeted cellular uptake and siRNA silencing by quantum-dot nanoparticles coated with β-cyclodextrin coupled to amino acids. Eur J Chem 17:5171–9
- Zhu Q, Guo T, Xia D, et al. (2013). Pluronic F127-modified liposome-containing tacrolimus-cyclodextrin inclusion complexes: improved solubility, cellular uptake and intestinal penetration. J Pharm Pharmacol 65:1107–17