Abstract
Context & Objective: The aim of present investigation was to formulate and develop lipid-based nanostructured carriers (NLCs) containing Idebenone (IDE) for delivery to brain. Attempts have been made to evaluate IDE NLCs for its pharmacokinetic and pharmacodynamic profile through the objective of enhancement in bioavailability and effectivity of drug.
Methods: Nanoprecipitation technique was used for development of drug loaded NLCs. The components solid lipid Precirol ATO 5, oil Miglyol 840, surfactants Tween 80 and Labrasol have been screened out for formulation development by consideration of preformulation parameters including solubility, Required Hydrophilic lipophilic balance (HLB) of lipids and stability study. Developed IDE NLCs were subjected for particle size, zeta potential, entrapment efficiency (%EE), crystallographic investigation, transmission electron microscopy, in vitro drug release, pharmacokinetics, in vivo and stability study.
Results: Formulation under investigation has particle size 174.1 ± 2.6 nm, zeta potential −18.65 ± 1.13 mV and% EE 90.68 ± 2.90. Crystallographic studies exemplified for partial amorphization of IDE by molecularly dispersion within lipid crust. IDE NLCs showed drug release 93.56 ± 0.39% at end of 24 h by following Higuchi model which necessitates for appropriate drug delivery with enhancement in bioavailability of drug by 4.6-fold in plasma and 2.8-fold in brain over plain drug loaded aqueous dispersions. In vivo studies revealed that effect of drug was enhanced by prepared lipid nanocarriers.
Conclusions: IDE lipid-based nanostructured carriers could have potential for efficient drug delivery to brain with enhancement in bioavailability of drug over the conventional formulations.
Introduction
Nowadays, nanoscience and nanotechnology has proved significant growth of research and its applications in the area of medicine. The definition in core nanotechnology field which confines the “nano” to at least 1–100 nm in one dimension while nanocarriers in the biomedical field are often referred to as particles with a dimension a few nanometers to 1000 nm. The delivery of therapeutics imposes main challenge to the central nervous system (CNS) due to the stringent necessities for therapeutic purpose. For achieving maximal therapeutic effects, the carriers must be designed in such a way that the drugs can be delivered to the target sites with an appropriate available dose and release kinetics (Wong et al., Citation2012).
From many years it was known that prolonged release of drugs occurs from solid–lipid matrices, i.e. drug release from lipid pellets which were produced by spray-congealing technique (Eldem et al., Citation1991). In the subsequent step the first generation of lipid nanoparticles was produced, purported to be as lipid nanopellets for oral administration (Speiser, Citation1990). At the beginning of nineties, the second generation was developed known as solid–lipid nanoparticles (SLN). The SLNs are made from solid lipids, i.e. lipids matrix used is solid at room temperature and also at body temperature which are stabilized by surfactants (Gasco, Citation1993). For preparation of SLNs high-pressure homogenization, micro-emulsions by the phase-inversion temperature (PIT) and more efficient solvent injection techniques are used through the point of attainment in nanoscale particle size (Stancampiano et al., Citation2006; Montenegro et al., Citation2011). Factorial design approach was generally implemented for the determination of optimum levels of formulation and process variables in design and development of SLNs (Carbone et al., 2012b). Such novel drug delivery of SLNs overcame many problems of traditional nanoparticulate carrier technologies which limited the use, e.g. liposomes, polymeric nanoparticles. There are some potential confines like as limitation in drug-loading capacity, drug expulsion during storage, high water content of aqueous SLN dispersions (70–95%). This consequently led to a new, improved novel generation of lipid nanoparticles, nanostructured lipid carriers (NLC). SLNs are produced from solid lipids and NLCs are produced by controlled mixing of solid lipids with spatially incompatible liquid lipids (oils). NLCs consisting of a lipid matrix with special nanostructures has been developed which improves drug loading, firmly incorporates the drug during storage and release properties (Montenegro et al., Citation2006). In addition, modifications in NLCs by incorporating cationic lipids have been exemplified a critical role in increasing the lipids structural order with proficient enhancement in physical stability and exhibition of required therapeutic activity (Carbone et al., Citation2014). NLCs can be produced by high-pressure homogenization and the process can be modified to yield lipid particle dispersions with solid contents from 30–80% (Muller et al., Citation1998). NLCs promote the absorption of a range of drugs and enhancement in bioavailability which supports the use of lipid particles for oral delivery. NLCs avoids the expulsion of drugs from lipid matrix during storage this is due to controlled nanostructures formed by solid lipids and liquid lipids which offers enough space to accommodate the drug (Souto & Muller, Citation2006).
From the last decade NLCs have attracted considerable interest in targeting the drug molecules to brain. Lipid nanocarrier delivery systems have great potential to facilitate the movement of drugs across blood brain barriers (BBB; Alam et al., Citation2010). Diseases of the central nervous system such as schizophrenia, meningitis, migraine, Parkinson’s disease, Dementia and Alzheimer’s disease require delivery of the drug to the brain for treatment. Small particles of NLCs having particle size less than 200 nm revealed for bypassing the activation of reticular endothelial system (RES), reduced hepatic uptake and prolonged blood circulation times (Tosi et al., Citation2008). Based on aforementioned studies, it is also thought that a nanoparticulate size <200 nm is appropriate for brain-cell targeting and controlled release (Lockman et al., Citation2003). So drug delivery by using lipid-based nanostructured carriers could overcome the pharmaceutical hindrances related to bioavailability and therapeutic drug concentration in the brain by endocytosis mechanism (Muller et al., Citation2000; Lockman et al., Citation2004). Idebenone (IDE) molecule is a synthetic short-chain analogue of ubiquinone to the potent antioxidant coenzyme Q10. It’s an integral cell membrane nutrient and contributor to the adenosine triphosphate-producing mitochondrial electron transport chain (ETC). IDE appears to act as an anti-oxidant that also enhances the flux of electrons along the ETC, thereby facilitating the generation of ATP (Suno & Nagaoka, Citation1984; Gillis et al., Citation1994). It could protect cell membranes and mitochondria from oxidative damage and its antioxidant properties could protect against cerebral ischemia and nerve damage in the CNS (European Medicines Agency, Citation2009). Due to high first pass metabolism, less than 1% of parent IDE reaches the systemic circulation where the concentration of metabolite is considerably higher than parent IDE which complements for giving pharmacological action.
SLNs and NLCs are considered as a noteworthy candidates amongst the various colloidal drug delivery systems developed as carriers for IDE, due to their many advantages such as increasing loading capacity of the active compound, minimizing the expulsion of the actives during storage, its protection from chemical degradation, good tolerability, drug targeting, and increasing drug bioavailability (Muller et al., Citation2000; Teeranachaideekul et al., 2007a). In one of the biological study, it has been reported that IDE-loaded SLNs were more effective in inhibiting 2,2′-azobis-2-amidinopropane dihydrochloride (APPH) induced ROS production in primary cultures of newborn rat astrocytes compared to the free drug (Carbone et al., 2012a; Stancampiano et al., Citation2006). Though there are reports about lipid nanocarriers and the various formulations of IDE for delivery of the drug for the topical, cosmetic, parenteral and oral applications, existing in the literature but there is none of the reports which discloses the IDE loaded lipid-based nanostructured carriers in the form of NLCs considered as most advanced generation of lipid nanoparticles as oral drug delivery system for brain to treat the neurodegenerative disorders.
Hence by consideration of above mentioned hypotheses regarding formulation and therapeutic advantages of lipid nanocarriers and pharmacokinetic properties of IDE, there is a continuing need to develop IDE-loaded lipid-based nanostructured carrier formulation of particle size <200 nm for enhancing its bioavailability with by passing RES system and prolonged blood circulation times with efficient brain transport in the treatment of neurodegenerative disorders. Hence, aims and objectives of the present invention are directed to the development of IDE NLCs by solvent injection along with nanoprecipitation technique. So novelty of present research is that, such formulation approach of IDE lipid-based nanostructured carrier system could be beneficial as benchmark in targeting the drug delivery to brain in the era of nanomedicine. Also it could potentially improve the bioavailability and therapeutic effect in terms of cognitive function for the treatment of neurodegenerative disorders such as Dementia and Alzheimer’s disease.
Materials and methods
Materials
The pure active pharmaceutical ingredient IDE [2-(10-Hydroxydecyl)-5,6-dimethoxy-3methyl-2,5- cyclohexadiene -1,4- dione] was obtained as gift sample from Takeda Pharmaceuticals Ltd, Rome, Italy. Precirol ATO 5 (Glyceryl palmitostearate) was gift sample from Gattefosse, St-Priest, France used as solid lipid. Liquid lipid (oil) Miglyol 840 (Propylene glycol dicaprylate/dicprate) was gift sample from Sasol Germany GmbH, Hamburg, Germany. HPLC grade methanol and 2-propanol were purchased from Molychem (Mumbai, India) and ethanol was purchased from Jiangsu Huaxi International Trade Co. Ltd., Jiangsu, China, used as solvents. Surfactant co-surfactant system comprising Tween 80 and Labrasol (Caprylocaproyl macrogol-8-glyceride) as gift samples from S. D. Fine Chemicals Ltd, Mumbai, India, and Gattefosse Cedex., St-Priest, France, respectively. Water used in whole research study was bi-distilled. All other chemicals used in the study were of analytical grade.
Methods
Preparation of IDE loaded lipid-based nanostructured carriers as drug delivery system
Preparation of IDE nanostructured lipid carrier drug delivery system were prepared by nanoprecipitation method. In this method solid lipid Precirol ATO 5 of 10 mg/ml was melted to 55–60 °C. Melted phase was added into the mixture of liquid lipid Miglyol 840 of 10 mg/ml containing drug 0.5% w/v and 2-Propanol. The resulting 3 ml of this solution was rapidly injected into distilled water of 50 ml containing surfactant system (Smix) Tween 80 and Labrasol at the concentration 0.05% w/v of each, under ultrasonication (Sonic vibra cell, Sonics & Materials, Newtown, USA) condition for 5 min at temperature of 40 °C, amplitude 90% and pulse on 30 s; pulse off 5 s. The resultant lipid nanostructured dispersions were then allowed to return at room temperature for about 45 to 60 min. Such NLCs dispersion was fast frozen under −40 °C in a deep-freezer (Sanyo Ultra Low Temperature Freezer MDF-192, Osaka, Japan) for 10 to 12 h and then the sample was subjected for lyophilization. Lyophilization was performed for 72 h (Heto Drywinner, model DW1 060E, Holten, Allerod, Denmark) using trehalose as cryoprotectant. The resultant powder of drug-loaded NLCs were used for subsequent evaluation studies.
Evaluation of IDE-loaded lipid-based nanostructured carriers as drug delivery system
Particle size determination
Particle size distribution and mean particle size of optimized batch of IDE NLCs was determined by dynamic light scattering using Nicomp 380 ZLS particle size analyzer (Particle Sizing Systems, Inc., Santa Barbara, CA) at room temperature. The width of the size distribution was indicated by the polydispersity index (PI).% Intensity distribution was taken into consideration for evaluation of whole particle size distribution data through the point of detection of even a few large particles.
Zeta potential (ζ) determination
Zeta potential analysis study was performed for the determination of stability of developed IDE lipid-based nanoformulation. Charges on drug-loaded droplet surface were measured using Zetasizer 300 HSA (Malvern Instruments, Malvern, UK). Analysis time was kept for 60 s and average ZP, charge and mobility of optimized batch of IDE NLCs was determined.
Crystallographic investigation
X-ray crystallography (XRD)
The powder X-ray diffraction patterns were recorded using an X-ray Diffractometer (PW 1729; Philips, Netherland), with Cu as anode material and crystal graphite monochromator operated at a voltage of 30 kV and a current of 30 mA. The samples were analyzed in the 2θ angle range of 2 to 50°. The range and the chart speed were 5 × 103 CPS and 10 mm/°2θ, respectively.
Differential scanning calorimetry
The differential scanning calorimetry (DSC) measurements were performed using METTLER Toledo DSC 821e module controlled by STARe software (METTLER Toledo GmbH, Gießen, Germany. The sample (4–12 mg), for each measurement was placed in sealed aluminium pans, before heating under nitrogen flow (20 ml/min) at a scanning rate of 10 °C/min, over the temperature range of 25–250 °C.
Transmission electron microscopy
Transmission electron microscopy (TEM) was used to characterize the microstructure of IDE lipid-based nanostructured carriers. Formulation was placed on a carbon-coated copper grid and then a drop of 1% phosphotungstic acid covered on layer of formulation. The superfluous phosphotungstic acid on formulation was wiped off by filter paper. After being stained by 2% phosphotungstic acid (PTA) solution, it was dried at room temperature for about half an hour, and the sample was gained for the TEM investigation. The TEM images were obtained using a Tecnai G2 20 TEM (FEI Corp., Hillsboro, OR, USA).
Entrapment efficiency
Entrapment efficiency (%EE) of IDE lipid-based nanostructured carriers were measured by minicolumn centrifugation technique which requires application of centrifugal force for the separation of the nanoparticles from the aqueous medium (Fry et al., Citation1978; Teeranachaideekul et al., 2007a). Free drug remained bound to the gel, while entrapped nanoparticulate drug traveled through the minicolumn of Sephadex® G25M which were further determined by V-630 UV-Vis Spectrophotometer (Jasco, Easton, MD, USA) spectrophotometer.
In vitro drug release study
In vitro drug release from IDE lipid-based nanostructured carrier drug delivery system was assessed by using USP Type-I dissolution test apparatus (Electrolab, TDT 08L, Mumbai, India) at 37 ± 0.5 °C and 100 rpm using 0.1 N HCl (900 ml) for first 2 h (Osterwald, Citation1990). The further procedure was performed using dissolution media as: 2 h in pH 4.5, 2 h in pH 6.8 phosphate buffer and lastly pH 7.4 maintained for 24 h at 37 ± 0.5 °C, considering the pH of the GI tract (Watts & Illum, Citation1997). Dissolution studies were carried out in triplicate, maintaining the sink conditions during the dissolution test in all dissolution media. A 5 ml aliquot of sample was withdrawn at regular time intervals, filtered and assayed by HPLC method at C9 channel wavelength of 282 nm. The cumulative% drug release was calculated for the formulations and the drug release data were curve fitted using PCP Disso V3 software (BVCP, Pune, India).
HPLC
HPLC studies were carried out by using reverse-phase C18 column (25 × 0.46 cm, pore size 5 μm; Luna, Phenomenex, Torrance, CA) connected to a LC-10AD LC pump (Shimadzu, Tokyo, Japan) with 280 nm detection. The mobile phase consisted of methanol in water (80:20 v/v) at 1.0 ml/min. NLCs (∼10 mg of IDE) were dissolved in 5 ml dimethyl sulfoxide; 50 ml of methanol were added and after sonication for 15 min the volume was brought to 100 ml with water. This solution was diluted with methanol: water (50:50 ratio) in triplicate so as to be with in the linear range of the method (∼6 μg/ml of IDE) (Amorim et al., Citation2009). The injection loop used as a 100 μl stainless steel loop and injection volumes of 50 μl were used. To demonstrate the specificity of the method, peak of IDE lipid-based nanostructured carriers was compared with pure drug.
Pharmacokinetic study in blood plasma and brain compartment
Wistar albino rats of either sex weighing 200–250 g were used for estimation of pharmacokinetic parameters of IDE NLCs and plain drug-loaded aqueous dispersions. The albino rats were kept in animal house under standard laboratory conditions with free access to diet and water as per guidelines of CPCSEA. The experimental protocol was approved by Institutional Animal Ethical Committee (Approval No. BVCPK/CPCSEA/IAEC/01/07).
Totally 18 animals were used for performance of pharmacokinetic study in blood plasma and brain compartment. Animals were separated into three groups with six animals in each group. Group I: (control) saline 3 mg/kg, Group II: (IDE lipid-based nanostructured carriers), repeated oral administration of formulation containing IDE equivalent to 3 mg/kg and Group III: (IDE aqueous dispersions) repeated oral administration of formulation containing IDE equivalent to 3 mg/kg. The rats were anaesthetized using ether anesthesia. Blood samples were withdrawn from retro orbital puncture, immediately the animals were decapitated and the brain tissue samples were collected. Both the samples of blood and brain tissue were collected at an interval of 0.25, 0.5, 1, 2, 6, 12, 15, 18, 21 and 24 h. The plasma was separated by centrifuging the blood samples using centrifuge (Remi, Mumbai, India) at 12 000 rpm for 10 min. Drug estimation in brain tissue was determined by homogenizing with ice cold saline (0.2 g of tissue per ml of saline) using tissue homogenizer at 6000 rpm (Remi, Mumbai, India). The plasma and brain tissue samples were kept frozen at −80 °C until drug analysis (Zhang et al., Citation2007; Khandadi et al., Citation2011).
Preparation of blood and brain tissue samples for HPLC analysis
Accurately 0.5 ml of 0.1 M acetic acid was added to 3 ml of blood and brain tissue samples. Samples were then centrifuged at 5000 rpm for 30 min. The supernatant was collected from all samples of used experimental animals. Accurately 1 ml of supernatant from plasma and brain tissue collections was added to series of 10 ml of volumetric flasks. Exactly 1 ml of 0.1 µg/ml standard stock solution of IDE was added into it and final volume adjusted to 10 ml with mobile phase. Precaution has been taken in each stage of sampling with avoidance of precipitation of blood and brain cells by treatment of subsequent centrifugation cycles and collection of supernatant at each and every new one stage (Zhang et al., Citation2007; Vyas et al., Citation2008; Khandadi et al., Citation2011).
Estimation of pharmacokinetic parameters
Accurately 50 μl of sample solution was injected into the chromatographic system using fixed volume loop injector. The pharmacokinetic parameters including maximum plasma concentration (Cmax), corresponding time (tmax) and area under the concentration-time curve (AUC) were obtained directly from plasma and brain tissue homogenate concentration time data which was estimated according to trapezoidal rule. The peak area obtained was used to calculate the concentration at each time interval for IDE NLCs and aqueous dispersions.
In vivo study
Total 24 Wistar albino rats of either sex (200–250 g) were used for in vivo study such as Morris water maze learning test. Animals were divided into four groups with six animals in each group. Group I: (control) treated with saline 3 mg/kg, Group II: (IDE NLCs), repeated oral administration of formulation containing IDE equivalent to 3 mg/kg, Group III: (IDE aqueous dispersions) repeated oral administration of formulation containing IDE equivalent to 3 mg/kg, Group IV: treated with scopolamine subcutaneously injected at a dose of 2 mg/kg of body weight.
Morris water maze testing was performed in a circular pool (diameter: 90 cm, height: 25 cm), filled to a depth of 12 cm of water (19 ± 1 °C). The white platform (10 cm2) was submerged approximately 1 cm below the surface of the water, and milk was then added to the water to make it opaque. The rats could escape from the water on to the platform. The time was measured from start to escape from the water onto the platform. The rats were assigned to four groups for the swimming test. All the rats first learned the location of submerged platform for two consecutive days, and were sorted out the uniform ability of rats, in which they were released from the same starting point in the maze in all sessions and given a maximum of 120 s to the platform. When the rat reached the platform, it was allowed to remain on it for 20 s. If the rat failed to find the platform within 120 s, it was removed from the water and placed on the platform for 20 s. The location of the platform remained the same for all tests. Latency was recorded for each trial. Six escape trials were given to all the rats per day for five consecutive days. All latency for the given day was calculated by averaging the six trials. Scopolamine (2 mg/kg of body weight) was given by subcutaneous injection 30 min prior to the experiments while formulations of IDE lipid-based nanostructured carriers and aqueous dispersions were administrated orally15 min prior to the experiment (Yoshiyuki et al., Citation1981; Morris, Citation1984; Hatano et al., Citation1989).
Stability study
The optimized formulation of IDE lipid-based nanostructured carriers were subjected for accelerated stability studies as per ICH guidelines (CitationQA(R2)). The IDE NLCs were observed for physical appearance, particle size, zeta potential, entrapment efficiency and in vitro release for a period of three months. Samples of optimized formulation of drug loaded NLCs were kept in refrigerator and programmable environmental chamber for three months at 5 ± 3 °C, 60% RH ± 5% RH and elevated temperature at 40 ± 2 °C, 75% RH ± 5% RH, respectively. Samples were withdrawn at 30, 60 and 90 days of intervals from the time of placing sample into the chamber where physical appearance, particle size, zeta potential, entrapment efficiency and in vitro drug release were assessed.
Results and discussion
For formulation development of IDE lipid-based nanostructured carriers screening of lipids, surfactants and solvents has been carried out on the basis of solubility, HLB (Hydrophilic lipophilic balance) theory, particle size, zeta potential and stability study. Formulation consisting of following concentration of components deemed as an optimized composition for the development of IDE NLCs as drug delivery system, which has been taken into consideration for further studies. Optimized drug-loaded NLCs as a formulation comprises 0.5% w/v of IDE, 10 mg/ml each of both lipids as Precirol ATO 5 and Miglyol 840, respectively. Surfactant combination system was optimized at concentration of 0.05% w/v each of Tween 80 and Labrasol. 2-Propanol and water were screened out as solvent and anti-solvent phase set at volume of 3 ml and 50 ml, respectively.
Particle size determination
Particle size distribution is one of the most essential characteristics through the point of stability of formulation and drug delivery at the appropriate target site in physiological system. Particle size parameters have been characterized immediately after the production of IDE lipid-based nanostructured carriers. Particle size of each batch was evaluated by laser diffractometer (LD). Particle size distribution in terms of% intensity for optimized IDE lipid-based nanostructured carriers has shown in .
Optimized formulation has shown mean particle size d 50% of 117.3 ± 3.6 nm and d 90% of 174.1 ± 2.6 nm, whereas polydispersity index (PI) and Chi squared values were observed to be 0.265 and 16.71, respectively. The particle size distribution during 3 months of storage at room temperature was shown in . Average mean particle size d 90% of IDE NLCs during 3 months of storage at room temperature was found to be 191.2 ± 4.1 nm which was beneath 200 nm. As reported in literature (Lockman et al., Citation2003; Tosi et al., Citation2008), particle size of less than 200 nm bypasses in blood circulation with inhibition in uptake of nanoparticles by RES and hepatocytes. Hence these result attributes for achieving desired therapeutic level of IDE in system with prolonged blood circulation and efficient brain transport of NLC formulation. So such substantial study could supports for enhancement in bioavailability of drug and effective delivery to brain as target site.
Table 1. Average particle size measured by LD during 3 months of storage at room temperature.
Relative standard deviation (RSD) for IDE lipid-based nanostructured carriers was observed to be ≤8.64. Henceforth by consideration of statistical evaluation study, it gives evidence for reproducibility of results and stability of prepared optimized nanoformulaion.
Zeta potential (ζ) determination
IDE lipid-based nanostructured carrier system showed a zeta potential of −18.65 ± 1.13 mV. Zeta potential was found to be negative for developed optimized IDE lipid nanocarrier formulation due to carboxylic group of Miglyol 840 and insufficiency of counter ions for neutralization within electrically double diffuse layer. In lipid-based nanostructured carriers, solid lipid Precirol ATO 5 displaces the oil Miglyol 840 as a result displacement of charged particle occurs hence it might possess less negative value. Tween 80 and Labrasol perform an additional sterical stabilization of the nanoparticles in developed formulation. It has been reported (Jacobs & Muller, Citation2002; Salunkhe et al., Citation2014), that in a combined effect of electrostatic and sterical stabilization a zeta potential of about |−20| mV can be sufficient for physical stability. Hence formulation in investigation have shown prospects for physical stability as it has zeta potential of −18.65 ± 1.13 mV which is near to |−20| mV. Values of zeta potential showed that prepared IDE lipid-based nanostructured carriers have sufficient charge and mobility to inhibit aggregation of particles.
Crystallographic investigation
X-ray crystallography (XRD)
The X-ray diffraction patterns of the IDE, Precirol ATO 5 and IDE lipid-based nanostructured carriers are as shown in .
Figure 2. X-ray diffraction patterns: (A) IDE (B) Precirol ATO 5 (C) IDE lipid-based nanostructured carriers.
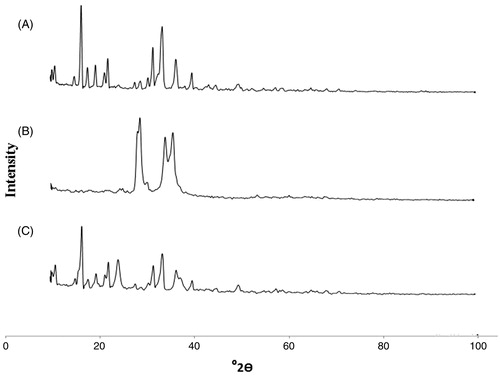
The X-ray diffraction pattern of pure drug IDE presented several diffraction peaks indicating the crystalline nature of the drug. Peaks for crystallinity were observed in pure drug at 2θ values of 16.70, 22.29, 31.87, 33.44, 33.87, 36.59, 36.84, 40 (). The solid lipid Precirol ATO 5 also showed peaks at 28.25, 29.12, 34.27, and 35.97 ). The XRD pattern of IDE lipid-based nanostructured carriers () showed the presence of peaks of drug with a decrease in intensity but effect on sharpness was not considerable. As reported by others (Teeranachaideekul et al., 2007b), results confirmed that the partial crystallinity in NLCs was observed due to molecularly dispersion of IDE in the blend of lipids Precirol ATO 5 and Miglyol 840. Remarkable lessening in intensity of peaks of solid-lipid Precirol ATO 5 in formulation gives explanation for amorphization of the lipid. Thus this result suggests that there was no significant change in chemical integrity of drug IDE.
Differential scanning calorimetry
DSC thermogram for IDE, Precirol ATO 5 and IDE lipid-based nanostructured carriers are shown in . The thermal curve of IDE showed sharp melting endothermic peak at 57.5 °C ( indicating its crystalline nature. Thermal curve for Precirol ATO 5 also shown in . However, in case of IDE lipid-based nanostructured carriers, the characteristic endothermic peak, corresponding to drug melting was observed at 52 °C . This could be attributed to presence of drug in partial crystalline state.
As reported in the literature (Montenegro et al., Citation2012), nanocrystalline size in lipid-based carriers causes lowering of the melting peak temperature with lessen in peak intensity of lipid core comprehending drug particles. Similar behavior was observed in IDE NLCs as a formulation which exhibited lowering in melting temperature of IDE from 57.5 °C to 52 °C with slight decrease in peak intensity. Hence from combined outcomes of crystallographic investigation study, it was concluded that such partial crystallinity might be due to presence of lipids and surfactant concentration leading to embedding of drug in the crust of NLCs. Moreover, the data also indicates that there seems to be no interaction between the components of system.
Transmission electron microscopy
TEM study was done in order to provide more insight about the morphology and size of the optimized IDE lipid-based nanostructured carriers. It was found that IDE-loaded NLCs have similar physicochemical properties to those as stated in previously reported studies (Montenegro et al., Citation2011). TEM photomicrographs of formulation reveals that NLCs have particle size in the range of 70–180 nm along with almost spherical shape and some free drug at the periphery of the droplets with no prominent sign of aggregation; this is due to displacement of Miglyol 840 by solid lipid Precirol ATO 5 as shown in .
The particle size as determined by the TEM was in accordance with that determined by the Nicomp 380 ZLS particle size analyzer. Hence it reveals that particle size by TEM supports for the values of d10%, d50% and d90% which were as measured by laser diffractometer.
Entrapment efficiency
The percentage of entrapped drug in NLCs were observed as 90.68 ± 2.90, 90.17 ± 2.68, 89.57 ± 2.74 after 1, 7 and 21 days, respectively when stored at room temperature. Insignificant change in entrapment efficiency during 7 days and 21 days of storage study implies that prepared drug loaded NLCs has stability over 21 days of period. The high percentage EE can be attributed to the high lipophilicity of IDE, the high solubility of the active in the oil Miglyol 840 and solid lipid Precirol ATO 5. In one of the studies (Liu et al., Citation2007), it has been reported that the combined 0.1% w/v concentration of Tween 80 and Labrasol influences on lipid nanocarriers by demonstrating high% EE with stability. So present investigation established the same findings which imparts high stability with proficient drug entrapment in NLCs due to the use of proposed concentration of surfactant system.
Thus it can be concluded that IDE NLCs comprises of binary mixture of liquid lipid and solid lipid, resulting in a highly disordered, imperfect lipid matrix structure offering grater space for drug accommodation, which confirms with the effects of high and stable entrapment of drug in lipid crust.
In vitro drug release study
In vitro drug release study of IDE lipid-based nanostructured carriers and IDE aqueous dispersions are as shown in and graphically represented in .
Figure 5. In vitro release profile of IDE from lipid-based nanostructured carriers and aqueous dispersions.
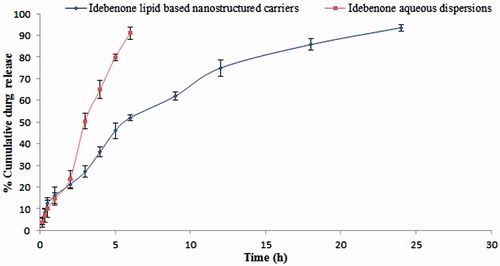
Table 2. In vitro release profile of IDE from lipid-based nanostructured carriers and aqueous dispersions.
In vitro drug release from the freeze dried powder of NLCs was carried out using empty gelatin capsule in above mentioned dissolution media. IDE lipid-based nanostructured carriers showed an initial burst release of drug, approximately 4–21% of drug releases in 0.1 N HCl during the first 2 h which can be explained by enrichment of IDE in the outer shell of particles containing an oily nanocompartment. The different melting behavior of solid and liquid lipids can lead to accumulation of the liquid lipid in the outer shell of lipid nanoparticles after lipid crystallization. So by admixing oil the viscosity of the outer lipid particle matrix is reduced leading consequently to burst drug release in an initial phase. Prolonged release in latter phase up to 24 h can be explained by slower release of IDE from higher viscous inner solid matrix of lipid nanocarriers. At the end of 24 h, drug release from developed lipid–based nanostructured carriers was observed up to the 93%, whereas IDE aqueous dispersions showed drug release approximately 3% to 23% in 0.1 N HCl during the first 2 h and about 91% at the end of 6 h. Thus results reveal that due to such release pattern of the drug, IDE NLCs have achieved the necessary effect for optimum drug dissolution profile. As reported in one of the studies (Jenning et al., Citation2000; Montenegro et al., Citation2011), polymorphic transitions of the lipid matrix plays a decisive role in the drug release from SLN. Similarly due to difference in the ability of lipid components containing Precirol ATO 5 and Miglyol 840 to accommodate host molecules resulted into change in polymorphic transitions which can exhibit effects with prolonged release of IDE from NLCs. Such behavior was observed owing to alteration in both the transitions of lipids and diffusion rate from the donor phase. As reported for lipophilic drugs in case of vitamin A loaded into SLN (Jenning et al., Citation2000), on the same ground IDE was thought to be released only from lipid phase as it has very poor water solubility (3 µg/ml) so maximum amount of drug lies in lipid crust from where the drug release can take place with sustained manner.
In vitro drug release kinetics: Drug release data were fitted to the dissolution kinetic model including the zero order Equation (Equation1(1) ), first order Equation (Equation2
(2) ), Higuchi matrix Equation (Equation3
(3) ), and Korsmeyer-Peppas Equation (Equation4
(4) ), release equations to find the equation with the best fit.
(1)
(2)
(3)
(4)
where m0 is the initial drug amount (100%, when represented as%); m the amount of drug remaining at a specific time (calculated as% of m0); k the rate constant; t is the time.
The kinetics of the release of drug from IDE NLCs and aqueous dispersions illustrated by equations such as zero order rate equation, which describes the drug dissolution of several types of modified release pharmaceutical dosage forms where equilibrium conditions are not obtained which is related to unchange in the area of dissolved species. The first order equation describes the release of drug which is proportional to the amount of drug remaining in its interior porous matrices in such a way that the amount of drug released by unit of time diminishes. Higuchi equation describes the drug release by diffusion process based on the Fick’s law where drug particles dispersed in a uniform matrix, and the rate of drug release with respect to the rate of diffusion. The Korsmeyer–Peppas equation is used to analyze the release of pharmaceutical polymeric dosage forms, when the release mechanism is not well known or when more than one type of release phenomena could be involved. To find out exact dissolution mechanism, drug release data of formulations were applied in above dissolution kinetic equations.
The correlation coefficients (value of r) for zero order, first order, Higuchi matrix, and Korsmeyer-Peppas in case of IDE NLCs were observed to be 0.8941, 0.5209, 0.9861 and 0.6819, respectively. Whereas for IDE aqueous dispersions these values were found to be 0.9735, 0.6608, 0.9445 and 0.9856, respectively.
Overall curve fitting showed that drug release from IDE lipid-based nanostructured carriers was best expressed by Higuchi’s classical diffusion equation and as the plots showed high linearity r = 0.9861 and similarity factor of 94.56, whereas drug release from IDE aqueous dispersions followed Korsmeyer-Peppas equation (non-Fickian diffusion) with linearity of r = 0.9856 and similarity factor of 38.84. Thus it can be concluded that the prolonged release of IDE from NLCs considered being a paramount effect of lipid matrix drug load which could assist for enhancement in the oral absorption by exhibiting appropriate dissolution profile.
HPLC
From HPLC study, it has been found that the retention time of IDE from NLCs was observed to be 7 min which is quite near to pure drug of 6.8 min. Thus it concludes that excipient from the lipid-based nanostructured carriers did not cause interference with the peak of IDE.
Pharmacokinetic study in blood plasma and brain compartment
Relative pharmacokinetic parameters of formulations in blood plasma and brain tissue are as shown in .
Table 3. Relative pharmacokinetic parameters of formulations containing IDE measured in blood plasma and brain compartment.
Cmax for IDE lipid-based nanostructured carriers in plasma (43.452 ± 4.479 µg/ml) represents greater improvement in the concentration achieved than the IDE aqueous dispersions (7.823 ± 1.340 µg/ml)). It was also observed that AUC0 → 24 h of IDE NLCs was 6 229 441.495 ± 264 546.334 µg h/ml and thus the difference was highly significant (p < 0.05) as compared to AUC0 → 24 h (1 349 762.084 ± 37 225.487 µg h/ml) of aqueous dispersions. The significant difference (p < 0.05) in the pharmacokinetic parameters such as Cmax and AUC of both formulations was clearly observed. tmax values observed for IDE NLCs and aqueous dispersions were 9.33 ± 0.35 h and 5.08 ± 0.18 h, respectively. Statistically, the difference in tmax of IDE NLCs was also found to be significant (p < 0.05) when compared to aqueous dispersions. In blood plasma, the% relative bioavailability of IDE lipid-based nanostructured carriers to that of plain aqueous dispersions was found to be 4.6%. Furthermore, results have been supplemented with illustrations which describes AUC of IDE NLCs is 4.6-fold greater than plain IDE aqueous dispersions (Supplementary Figure 6).
In brain distribution studies, Cmax for IDE lipid-based nanostructured carriers was observed to be 9.910 ± 0.567 µg/ml whereas for IDE aqueous dispersions it was 1.891 ± 0.243 µg/ml. tmax values for IDE NLCs and aqueous dispersions were found to be 8.89 ± 0.24 h and 4.90 ± 0.14 h, respectively. The values of AUC0 → 24 h for IDE NLCs was 1 410 879.021 ± 12 231.132 µg h/ml and for aqueous dispersions was 503 885.014 ± 3453.243. Statistical significant difference (p < 0.05) was observed between IDE NLCs and aqueous dispersions for executed experimental pharmacokinetic parameters in brain tissues. In brain compartment, the percentage of relative therapeutic bioavailability of IDE NLCs was observed as 2.8% over the plain drug-loaded aqueous dispersions. Thus results represent that prepared formulation of IDE in the form of NLCs could have potential for efficient drug delivery as well as transport in the brain.
As reported in earlier studies (Tiwari & Amiji, Citation2006; Vyas et al., Citation2008), pharmacokinetic parameters in the plasma and brain showed higher values for Cmax and AUC of saquinavir-loaded lipid nanoparticles in the form of nanoemulsions as compared to plain drug loaded aqueous suspensions when it was administered by oral route in experimental animals. So in account of above results, it depicts that enhancement in bioavailability in both the blood plasma as well as brain compartment occurs due to encapsulation of IDE in lipid-based nanostructured carriers. The pharmacokinetic studies revealed, significantly greater extent of absorption of IDE from lipid-based nanostructured carriers than the plain drug-loaded aqueous dispersions. Henceforth, this result attributed that the presence of lipids and surfactants in NLCs might have caused changes in permeability of the GI tract and the inhibition of an apically polarized efflux system, which could lead to enhancement of the oral bioavailability as well as brain transport.
In vivo study
The average escape latencies to find the platform was measured using water maze learning tests. At the end of 5th day, results reveal that scopolamine treated group showed no significant change in escape latency (72.43 ± 3.61 s). In control group, latency was slight shortened by training (70.41 ± 1.45 s). IDE aqueous dispersions treated group showed significant improvement in water maze learning deficit (67.80 ± 1.19 s). Whereas IDE NLCs treated group showed significant shortening of average latency (60.47 ± 1.10 s) and marked improvement in water maze learning deficit.
The analysis of the results by two way analysis of variance (Bonferroni test) showed significant differences amongst the groups (p < 0.05, F of 167 and R2 0.9692) especially between IDE NLCs and aqueous dispersions.
Previous study pointed out (Yamada et al., Citation2004), Z-ajoene inhibited the scopolamine-induced memory impairment in mice so it was considered as strong candidate through preview of preventive medicine in case of dementia. Thus on the same line, it concludes that developed IDE lipid-based nanostructured carriers help to lower the effect of memory impairments induced by scopolamine which will further gives strong experimental proof for pharmacological use of IDE lipid-based nanostructured carriers in the area of neurodegenerative disorders such as Alzheimer’s disease and certain memory impairments in which the stage of Dementia did not achieve.
Stability study
There was no significant change in particle size, zeta potential, entrapment efficiency and in vitro drug release of IDE lipid-based nanostructured carriers during specified storage period as shown in .
Table 4. Stability study of IDE lipid-based nanostructured carriers stored at 5 ± 3 °C and 40 ± 2 °C temperatures.
At both the temperature and humidity conditions, slightly but insignificantly decrease in% entrapment efficiency and zeta potential was observed whereas increase in particle size and in vitro drug release occurred over a period of 90 days. The use of lipid blend and surfactant combination system assists for good stability of IDE NLCs. On storage, entrapped hydrophobic drug liberates from lipid matrix which might be the reason for decrease in entrapment efficiency and increase in in-vitro drug release. In one of the reported studies (Carbone et al., Citation2014), temperature effects on crystallinity of lipid nanoparticles which were based on particle engineering and hence in present investigation it supports that temperature causes disturbance in the formed electrically double diffuse layer of formulation. Further, which might lead to increase in particle size and decrease in zeta potential. Physical appearance of the IDE NLCs shows minor changes. Thus it can be concluded that concentration of surfactants such as Tween 80 and Labrasol form an appropriate drug delivery system which compensates for required HLB of lipids. Furthermore, results depict that components stabilize the lipid nanostructured carrier drug delivery system of IDE and prevent the agglomeration, precipitation in the system.
Conclusions
Formulation and development of lipid-based nanostructured carrier system using nanoprecipitation method proven to be an inclusive approach to obtain stable optimized formulation of IDE for efficient drug delivery and transport to brain. The binary mixture of solid and liquid lipids leads for a highly disordered, imperfect lipid matrix structure leaves grater space for high and stable entrapment of drug with optimum attainment in particle size and zeta potential. Partial amorphization of drug occurs in the lipid crust which is supported by the crystallographic studies including XRD and DSC analysis. The solid lipid Precirol ATO 5, oil Miglyol 840, surfactant co-surfactant system comprising Tween 80 and Labrasol in IDE NLCs help for enhancement in bioavailability of drug by 4.6-fold in plasma and 2.8-fold in brain compartment over plain drug-loaded aqueous dispersions. Thus IDE NLCs could have the potential for effective drug delivery to brain by representing appropriate drug release profile of 93.56 ± 0.39% at the end of 24 h, which follows Higuchi dissolution kinetic model. In vivo study such as Morris water maze test indicated that the effect of drug was enhanced by prepared IDE lipid nanocarrier formulation. Hence IDE lipid-based nanostructured carrier system evidenced the potential for drug delivery and transport to brain over the conventional formulations.
Supplementary material available online
Supplementary Figure 6.
Supplemental Material.pdf
Download PDF (1,016.1 KB)Acknowledgements
The authors are very thankful to Principal, Bharati Vidyapeeth College of Pharmacy, Kolhapur for providing necessary facilities for this research work. The authors are highly thankful to Takeda Pharmaceuticals Ltd Italy for providing Idebenone as a gift sample. Authors are thankful to Gattefossé (Cedex, France) for providing solid lipid Precirol ATO 5 and surfactant Labrasol as gift samples. Authors are also thankful to Sasol Germany GmbH and S. D. Fine Chemicals Ltd, India for providing gift samples of oil Miglyol 840 and surfactant Tween 80, respectively.
Declaration of interest
The authors declare that they have no conflict of interest.
References
- Alam MI, Beg S, Samad A, et al. (2010). Strategy for effective brain drug delivery. Eur J Pharm Sci 40:385–403
- Amorim CM, Netz DJA, Couto AG, et al. (2009). Stability-indicating LC-PDA method for determination of idebenone in nanoparticles based on chitosan and N-carboxymethylchitosan. Chromatographia 70:1411–15
- Carbone C, Campisi A, Manno D, et al. (2014). The critical role of didodecyldimethylammonium bromide on physico-chemical, technological and biological properties of NLC. Colloids Surf B 121:1–10
- Carbone C, Pignatello R, Musumeci T, Puglisi G. (2012a). Chemical and technological delivery systems for idebenone: a review of literature production. Expert Opin Drug Deliv 9:1377–92
- Carbone C, Tomasello B, Ruozi B, et al. (2012b). Preparation and optimization of PIT solid lipid nanoparticles via statistical factorial design. Eur J Med Chem 49:110–17
- Eldem T, Speiser P, Hincal A. (1991). Optimization of spray-dried and congealed lipid micropellets and characterization of their surface morphology by scanning electron microscopy. Pharm Res 8:47–54
- European Medicines Agency. Evaluation of medicines for human use. London, 20 November 2008. Doc.Ref.:EMEA/387246/2009
- Fry DW, White C, Goldman DJ. (1978). Rapid separation of low molecular weight solutes from liposomes without dilution. Anal Biochem 90:809–15
- Gasco MR. (1993). Method for producing solid lipid microspheres having a narrow size distribution. US Patent 52 50 236
- Gillis JC, Benefield P, McTavish D. (1994). Idebenone. A review of its pharmacodynamics and pharmacokinetic properties, and therapeutic use in age related cognitive disorders. Drugs Aging 5:133–52
- Hatano T, Edamatsu R, Mori A, et al. (1989). Effects of the interaction of tannins with co-existing substances. VI. Effects of tannins and related polyphenols on superoxide anion radical, and on 1,1-diphenyl-pierylhydrazyl radical. Chem Pharm Bull 37:2016–21
- ICH harmonized tripartite guideline. Stability testing of new drug substances and products Q1A(R2)
- Jacobs C, Muller RH. (2002). Production and characterization of a budesonide nanosuspension for pulmonary administration. Pharm Res 19:189–94
- Jenning V, Schafer-Korting M, Gohla S. (2000). Vitamin A-loaded solid lipid nanoparticles for topical use: drug release properties. J Control Release 66:115–26
- Khandadi P, Syed MA, Goparaboina S, Veerabrahma K. (2011). Brain specific delivery of pegylated indinavir submicron lipid emulsions. Eur J Pharm Sci 42:423–32
- Liu Jie, Hu Wen, Chen Huabing, et al. (2007). Isotretinoin-loaded solid lipid nanoparticles with skin targeting for topical delivery. Int J Pharm 328:191–5
- Lockman PR, Koziara JM, Mumper RJ, Allen DD. (2004). Nanoparticle surface charges alter blood-brain barrier integrity and permeability. J Drug Target 12:635–41
- Lockman PR, Oyewumi MO, Koziara JM, et al. (2003). Brain uptake of thiamine-coated nanoparticles. J Control Release 93:271–82
- Montenegro L, Campisi A, Sarpietro MG, et al. (2011). In vitro evaluation of idebenone-loaded solid lipid nanoparticles for drug delivery to the brain. Drug Dev Ind Pharm 37:737–46
- Montenegro L, Carbone C, Condorelli G, et al. (2006). Effect of oil phase lipophilicity on in vitro drug release from O/W microemulsions with low surfactant concentration. Drug Dev Ind Pharm 32:539–48
- Montenegro L, Trapani A, Latrofa A, Puglisi G. (2012). In vitro evaluation on a model of blood brain barrier of idebenone-loaded solid lipid nanoparticles. J Nanosci Nanotech 12:330–7
- Morris R. (1984). Developments of a water-maze procedure for studying spatial learning in the rat. J Neurosci Methods 11:47–60
- Muller RH, Mader K, Gohla S. (2000). Solid lipid nanoparticles (SLN) for controlled drug delivery- a review of the state of the art. Eur J Pharm Biopharm 50:161–77
- Muller RH, Runge SA, Ravelli V. (1998). German patent application DE19819273A1
- Osterwald HP. (1990). Pharmaceutic development: mesalazine. Scand J Gastroenterol 25:43–6
- Salunkhe SS, Bhatia NM, Thorat JD, et al. (2014). Formulation, development and evaluation of ibuprofen loaded nanoemulsion prepared by nanoprecipitation technique: use of factorial design approach as a tool of optimization methodology. J Pharm Invest 44:1–18
- Souto EB, Muller RH. (2006). Polymorphic behaviour of Compritol 888 ATO as bulk lipid and as SLN and NLC. J Microencapsul 23:377–88
- Speiser P. (1990). Lipid nanopellets als Tragersystem fur Arzneimittel zur peroralen Anwendung. European Patent EP 01 67 825
- Stancampiano AHS, Acquaviva R, Campisi A, et al. (2006). Technological and biological characterization of idebenone-loaded solid lipid nanoparticles prepared by a modified solvent injection technique. J Biomed Nanotechnol 2:253–70
- Suno M, Nagaoka A. (1984). Inhibition of lipid peroxidation by a novel compound, idebenone (CV-2619). Jap J Pharmacol 35:196–8
- Teeranachaideekul V, Müller RH, Junyaprasert VB. (2007a). Encapsulation of ascorbyl palmitate in nanostructured lipid carriers (NLC) effects of formulation parameters on physicochemical stability. Int J Pharm 340:198–206
- Teeranachaideekul V, Souto EB, Junyaprasert VB, Muller RH. (2007b). Cetyl palmitate-based NLC for topical delivery of Coenzyme Q10-development, physicochemical characterization and in vitro release study. Eur J Pharm Biopharm 67:141–8
- Tiwari SB, Amiji MM. (2006). Improved oral delivery of paclitaxel following administration in nanoemulsion formulations. J Nanosci Nanotechnol 6:3215–21
- Tosi G, Costantino L, Ruozi B, et al. (2008). Polymeric nanoparticles for the drug delivery to the central nervous system. Expert Opin Drug Deliv 5:155–74
- Vyas TK, Shahiwala A, Amiji MM. (2008). Improved oral bioavailability and brain transport of saquinavir upon administration in novel nanoemulsion formulations. Int J Pharm 347:93–101
- Watts PJ, Illum L. (1997). Colonic drug delivery. Drug Dev Ind Pharm 23:893–913
- Wong HL, Wu XY, Bendayan R. (2012). Nanotechnological advances for the delivery of CNS therapeutics. Adv Drug Deliv Rev 64:686–700
- Yamada N, Hattori A, Hayashi T, et al. (2004). Improvement of scopolamine induced memory impairment by Z-ajozene in the water maze in mice. Pharmacol Biochem Behav 78:787–91
- Yoshiyuki K, Michinori K, Tadato T, et al. (1981). Studies on Scutellariae Radix. IV. Effects on lipid peroxidation in rat liver. Chem Pharm Bull 29:2610–17
- Zhang P, Chen L, Gu W, et al. (2007). In vitro and in vivo evaluation of donepezil sustained release microparticles for the treatment of Alzheimer’s disease. Biomaterials 28:1882–8