Abstract
The aim of this research was transdermal delivery of 5-fluorouracil (5-FU) using dextran-coated cellulose acetate phthalate (CAP) nanoparticulate formulation. CAP nanoparticles were prepared using drug-polymer ratio (1:1 to 1:3) and surfactant ratio (2.5, 5 and 10%). Dextran coating was made using aminodextran. The results showed that the optimized CAP nanoparticles (CNs) and dextran-coated CAP nanoparticles represented core-corona nanoparticles with the mean diameter of 75 ± 3 and 79 ± 2 nm, respectively, and entrapment efficiency was 82.5 ± 0.06 and 78.2 ± 0.12, respectively. Dextran-coated nanoparticles (FDCNs) and CAP nanoparticles (FCNs) showed in vitro 5-FU release upto 31 h and 8 h, respectively. Moreover, the cumulative amount of 5-FU penetrated through excised skin from FDCNs was 2.94 folds than that of the FU cream. Concentration of 5-FU in epidermis and dermis were also studied. In dermis, concentration of 5-FU was found higher in case of FDCN formulation than plain FU cream. FDCNs were found more hemocompatible in comparison to FCNs. The hematological data recommended that FDCNs formulation was less immunogenic compared to FU creams formulation. In blood level study, FDCNs exhibited 153, 12, 16.66 and 16.24-fold higher values for area under the curve, Tmax, Cmax and mean residence time (MRT) compared with those of FU cream, respectively. The in-vitro cytotoxicity was assessed using the MCF-7 by the MTT test and was compared to the plain 5-FU solution. All the detailed evidence showed that FDCNs could provide a promising tuning as a transdermal delivery system of 5-FU.
Introduction
Cancer is one of the leading causes of casualty in the globe. According to WHO, the figure of new cancer cases will increase to more than 15 million in 2020. Generally preferred techniques for the treatment of cancer are surgery, radiotherapy and chemotherapy. Delivery of chemotherapeutic agent to cancer cells depends upon various factors like, slow and poor perfusion rate of tumors, if chemotherapeutic agents represent poor protein binding they may possibly be readily excreted in the urine via glomerular filtration, on the other hand, the protein binding ability of many chemotherapeutic agent is generally high and rapid, this may lead to a decreased drug bioavailability and, in many cases, irreversible drug inactivation (Yadav et al., Citation2010).
To conquer such boundaries, a new and more promising technology, transdermal technology has been introduced for cancer drug therapy. The transdermal drug delivery has various limitation to deliver the drug because the outer most layer stratum corneum which generally consists of lipids and has no blood flow, and thus the limited amount of drug reaches to the blood circulation; in this way, the stratum corneum plays a key role in limiting the diffusion of drugs to the bloodstream and shows barrier properties of the outermost layer of skin. For the effective transdermal drug delivery system, the drugs must obviously be able to penetrate this skin barrier and reach the targeted cancer cells (Hadgraft & Guy, Citation1989; Guy et al., Citation1996). Transdermal drug delivery avoids the first-pass metabolism of the drug and provides sustained and controlled drug release. It is a non-invasive drug delivery which does not cause trauma or any type of risk of infection also increases patient. Whenever intravenous infusion provides rapid plasma levels, but it is more invasive route of drug delivery than transdermal drug delivery (Flynn, Citation1996).
Transport of lipophilic drug molecules is facilitated by their dissolution into intercellular lipids around the cells of the stratum corneum. Absorption of hydrophilic molecules into skin can occur through “pores” or openings of the hair follicles and sebaceous glands, but the relative surface area of these openings is barely 1% of the total skin surface (Surber, Citation1990). Once the drug molecules passes the stratum corneal barrier, and reaches to the dermal layer, systemic uptake occurs relatively hurriedly and fluently. The drug absorption through the skin generally occurs by passive diffusion. An indulgent of the transport performance of the drugs is crucial for crafty and effective transdermal product, as well as convincingly predicting and comparing drug performance in assorted formulations (Kydonieus, Citation1992; Ranade & Hollinger, Citation1996).
5-Fluorouracil (5-FU) is a pyrimidine analogue. It is indicated for the therapy of proliferative skin diseases, such as superficial basal cell carcinoma (Ghoshal & Jacob, Citation1997; Patrick et al., Citation1997). Normal as well as tumor cells metabolize 5-FU to 5-fluoro-2′-deoxyuridine monophosphate (FdUMP) and 5-fluorouridine triphosphate (5-FUTP). 5-FU has been shown to be functioning against a number of solid tumors of colon, breast, cervix and rectum (Ansifield et al., Citation1962). 5-FU is poorly absorbed when administered from oral route, with noteworthy deviation in bioavailability found between 0 and 80%, and after parenteral administration of 5-FU, the rapid elimination of the 5-FU was found with possible half life of approximately 8-20 min (Diasio & Harris, Citation1989).
According to skin penetration studies using diffusion cells and excised animal skin, it was establish that the aforesaid polymer (dextran) was effective for stunning the skin penetration of model permeants, such as 5-FU, antipyrine and indomethacin. Dextran shows the enhancement of the permeability of drug through skin and has negative charge (Fukushima et al., Citation2001; Akimoto & Nagase, Citation2003).
The present study has been designed for the development and evaluation of dextran conjugated cellulose acetate phthalate (CAP) nanoparticles for topical delivery of 5-FU. CAP nanoparticles loaded with 5-FU were first prepared and dextran was attached on these nanoparticles by covalent coupling method. Further these nanoparticles were characterized by in vitro and in vivo.
Materials and methods
Materials
5-Fluorouracil was obtained as generous gift sample from Neon Laboratories Ltd., Andheri (E), Mumbai (MH) India. Cellulose acetate phthalate (CAP) was purchased from Central Drug House, New Delhi, India. Dextran, Pluronic F-68 and dialysis membranes were purchased from Himedia, Mumbai (MH) India. Acetonitrile, isopropyl alcohol and acetone were purchased from Spectrochem Mumbai (MH) India. All other chemicals which used were of analytical reagent grade and were used as received.
Preparation of 5-FU-loaded CAP nanoparticles
Cellulose acetate phthalate (10 mg) was dissolved in 10 mL of isopropyl alcohol and acetone (9:1). Then 5-FU (10 mg) was added on the CAP solution. Pluronic F-68 (5 mg) is dissolved in water (100 mL) separately. The organic phase was then poured into Pluronic F-68 solution with magnetic stirring (Remi, Mumbai, India) at 1000 rpm for 2 h. Consequently, the solvent was evaporated at normal room temperature. The eventuated suspension of nanoparticles was filtered through 0.45 µm membrane filter (Millipore, Billerica, MA) and then centrifuged for 40 min at 10 000 rpm (Remi, Mumbai, India). The nanoparticles were resuspended in water after discarding supernatant.
Preparation of 5-FU-loaded dextran-coated CAP nanoparticles
5-FU-loaded CAP nanoparticles (FCNs) with smaller particle size and high entrapment efficiency were chosen for dextran coating. Amino-dextran (100 mg) (Amino dextran were synthesized as per method reported by Zalipsky et al., Citation1983) was dissolved in 100 mL of distilled water and added to 100 mg activated FCNs (FCNs: NHS: EDAC in stoichiometric molar ratio of 2:5:5) under constant stirring. These nanoparticles' dispersion was lyophilized and kept at 5 ± 3 °C for further use.
FTIR analysis
IR Spectra of FDCNs were recorded with a FT-IR spectrophotometer (Cary-630 FTIR, Agilent Technologies).
Morphology
The surface and shape morphology of FCNs and FDCNs were recognized by atomic force microscopy (AFM) (AIST-NT, Smart SPM 1000, Novato, CA). AFM of the nanoparticles was carried out at different magnifications using glass substrate in AC mode at room temperature.
Particle size, polydispersity index and zeta potential
A well diluted suspension of FCNs and FDCNs were crammed in the chamber of a laser diffraction particle size analyzer (DTS Ver. 4.10, Malvern Instruments, Malvern, UK). PDI and the average particle size were determined. The zeta potential of the nanoparticles was obtained by using a Malvern Zetasizer (DTS Ver. 4.10, Malvern Instruments, Malvern, UK).
Entrapment efficiency
Ten milligrams of FCNs and FDCNs were dissolved in acetone/isopropyl alcohol (9:1), separately. The mixture was firstly centrifuged and then the supernatant was diluted with methanol-PBS (pH 6.8) mixture. Further, the quantity of entrapped drug was analyzed with HPLC (Agilent Technologies, Santa Clara, CA).
The HPLC system (Agilent Technologies) which consist of variable wavelength detector, and a Zorbax 5 μ C18 column (Agilent Technologies Limited , Santa Clara, CA) (250 × 4.60 mm) was used in the analysis. The mobile phase was prepared with acetonitrile:acetate buffer pH 4.4, (ratio 15:85) with a detection limit of ∼20 ng, with a flow rate of 0.8 mL/min and 5-FU was detected at 260 nm. Firstly, linear curve was constructed and peak area was calculated, concentration and the equation of the line was obtained, which is y = 0.1078x + 0.0226 with correlation coefficient of 0.9999. The linearity was in the range between 0.1 and 40 μg/mL. Validation and calibrations was performed before and during the analysis.
Differential scanning calorimetry
Differential scanning calorimetry (DSC) analysis of samples was done using DSC 60 instruments (Shimadzu, Tokyo, Japan). The samples were weighted into aluminum pan and closed with a pin-holed lid. Thermograms were recorded under nitrogen atmosphere from ambient to 300 °C at a heating rate of 10 °C per min.
Powder X-ray diffractometry
Powder X-ray diffractometry (XRD) diffraction analyses were carried out in order to characterize crystalline nature of polymer and drug. Powder X-ray diffraction patterns of samples were obtained using power X-ray diffractometer (Bruker, Munich, Germany). X-ray diffraction analysis was performed using a nickel-filtered Cu-Kα radiation (a voltage of 40 kV and a current of 20 mA). The scanning rate was 2°/min over a 2θ range of 0–40° and with an interval of 0.02°.
In-vitro release study
Ten milligrams of FCNs and FDCNs were suspended into 2 mL of PBS (pH 6.8) and put into a dialysis tube (MWCO 2000 Da). The dialysis tube was placed into 50 mL of aqueous recipient PBS medium (pH 6.8) with continuous stirring at 100 rpm and 37 ± 2 °C, for the complete separation of drug in PBS. At various time intervals, the whole medium (50 mL) was replaced with the same volume of fresh PBS (pH 6.8) (50 mL) and samples were analyzed by HPLC.
Hemolytic toxicity
Whole human blood was collected and stored in HiAnticlot blood collection vials using the method described in our previous paper (Bhadra et al., Citation2005). The human blood was centrifuged then red blood cells were alienated and resuspended in normal saline solution (10% hematocrit). One milliliter of the red blood cell suspension was separately incubated with 5 mL of distilled water (taken as 100% hemolytic standard) and 5 mL of normal saline (taken as blank for spectrophotometric estimation). Further, 1 mL of adequately diluted CAP nanoparticles and dextran-coated CAP nanoparticles (negative control), FCN and FDCN formulation, and plain 5-FU was added to 5.0 mL of normal saline and interacted with RBC suspension. The suspensions were centrifuged for 10 min at 2000 rpm, and the absorbance of supernatant were measured at 540 nm, which was used to estimate percentage hemolysis using distilled water as 100% hemolytic standard.
Preparation of cream containing 5-FU-loaded dextran-coated nanoparticles
Cream containing FCNs and FDCNs formulation were prepared as reported by Jenning et al., (Citation2000) with sight modification. In brief, the oil phase of the cream consisted of 10% cetyl alcohol, 1.5% Tween-80, 1.5% Span-60, 12.0% liquid paraffin, 0.04% propylparaben and 0.2% butylated hydroxytoluene (BHT). All the ingredients added together and the formulate mixture was heated to approximately 80 °C. Methyl paraben (0.2%) was added to 74.06 g distilled water to produce the water-phase. This mixture was also heated to approximately 80 °C. When both phases reached approximately 80 °C, then the oil phase was mixed in water phase and homogenizing the mixture at 13 500 rpm, until the temperature of cream reached 40 °C. The cream was cooled down to approximately 25 °C while stirring at 200 rpm. Finally, FCNs and FDCNs dispersion was added under constant stirring.
Primary skin irritation studies of cream containing nanoparticles (FDCNs and FCNs)
The optimized cream formulation containing FCNs and FDCNs were evaluated for skin irritation study. The protocol approved by the Institutional Animal Ethics Committee of the Guru Ramdas Khalsa Institute of Science and Technology, Pharmacy, Jabalpur (MP), India with approval number GRKIST/406/02/IAEC/16A dated 16/01/2013 (Registration Number 1471/PO/a/11/CPCSEA, India). The hairs of the dorsal portion were physically removed with the help of surgical scissors and the skin was washed properly one day prior to use. Four groups of animals (albino rats) were formed having six rats in each group: First, second, third group of animals FDCNs, FCNs, cream (5-FU) were secured, respectively, and the fourth group of animals were kept as control. Applied cream was timely removed after 48 h from the skin and the applied area was examined for any signs of skin sensitivity or irritation. The fresh creams were secured at the same site at 2nd, 4th and 6th day. All the respective treatments were continued till 7 days and finally application sites were monitored.
In vitro permeation study cream containing nanoparticles (FDCNs and FCNs)
Permeation of 5-FU cream, FDCNs cream and FCNs cream formulations were evaluated using Franz diffusion cells (Permeager, Riegelsville, PA). Pieces of male, hairless full-thickness rat skin (2 cm2) was placed between receptor and donor compartments of the Franz diffusion cells and allowed to equilibrate for an hour, then the receptor compartment of the Franz diffusion cells was filled with PBS (pH 6.8) and continuously stirred to ensure the uniform distribution and maintain sink conditions and the temperature was maintained at 32 °C ± 0.5 °C. One gram of drug formulation (FDCNs cream, FCNs cream, 5-FU cream and Control) was applied on the surface of skin. At the definite time intervals, 300 μL samples were taken from each receptor and replaced with the same volume of fresh isotonic buffer after each sampling (Djordjevic et al., Citation2003). Every step of the experiment was repeated four times, and receptor samples were frozen at -30 °C prior to HPLC (Agilent Technologies) method.
Permeation data analysis
The cumulative amount of 5-FU permeated through the skin (micrograms per square centimeter) was plotted as a function of time (hours) for each formula. Drug flux (permeation rate) at steady-state (Jss) was calculated from the slope of the linear portion of the graph. The permeability coefficient (KP) was calculated using Equation (1):
where, Jss is the drug flux at steady state and C0 is the initial drug concentration in the donor cell. Finally, the enhancement ratio (Er) was calculated by dividing the Jss of the respective formulation by the Jss of the control solution.
In vitro cell line study
Cellular cytotoxicity was assessed by tetrazolium dye-based MTT assay following a previously reported procedure (Kolhe et al, Citation2003; Yadav et al., Citation2010). MCF-7 cells were maintained in RPMI-1640 medium supplemented with 10% heat-inactivated fetal bovine serum and antibiotics at 37 °C in a humidified incubator containing 5% CO2. The cells were treated with plain 5-FU and FDCNs nanoparticles in various concentrations (0.01, 0.1, 1.0, 10.0 and 100 μM) for 24 h. The amount of formulation needed to prepare molar equivalents of 5-FU was calculated, based on the drug content in the formulation. Control was taken without any drug treatment. Subsequently, MTT was added and plates were then incubated for another 3 h, the media was pippetted off and 250 μL DMSO was added. The absorbance of individual wells was noted at 570 nm via an ELISA plate reader at 25.8 °C. Average values from triplicate were subtracted from average value of control and the survival fraction of cells was calculated by the formula.
Blood level study
Healthy male albino rats having uniform body weight (100 ± 20 g) with no prior drug treatment were used for the study of drug (5-FU). The rats were stabilized on standard diet and water. The albino rats were divided into four groups having six animals each in each group. Blood samples (0.1 mL) were withdrawn from retro-orbital plexus in every 1, 2, 4, 6, 8 h for up to 24 h. The blood samples were centrifuged at 2000 rpm for 15 min and serum was collected and deproteinized with acetonitrile (1 mL/mL of serum). Then obtained samples were centrifuged and supernatants were analyzed for drug content.
Afterwards, the albino rats were sacrificed and the administrated skins were stripped. The excised skins were thoroughly rinsed with alcohol and distilled water to eliminate the 5-FU remains on the surface. A heat-separated membrane separation was carried out to separate epidermis and dermis (Kligman & Christophers, Citation1963). Briefly, the skin samples were spread out on a large watch glass (60 ± 1 °C) for 2 min, and then the epidermis was scraped from skin using a dull scalpel blade. Following, the epidermis or dermis sample pieces were mixed with 1 mL of physiological saline and subjected to homogenization for 3 min. The amount of 5-FU in the blood, epidermis or dermis samples was analyzed by HPLC method (Agilent Technologies) (Alsarra & Alarifi, Citation2004), respectively.
Hematological study
Healthy male albino rats having uniform body weight (100 ± 20 g) with no prior drug treatment were used for the in vivo studies. The rats were stabilized on standard diet and water. First, second, third group of animals' FDCNs, FCNs, cream (5-FU) were secured, respectively, and fourth group of animals were kept as control. The animals were taken and the hair removed with the help of a razor, and FCNs and FDCNs containing cream spread on the shaved area every day. Blood was withdrawn after 7 days from the animals and analyzed for hemoglobin (Hb) content, WBCs, RBCs, differential lymphocytes, monocytes and neutrophils in pathology lab.
Statistical analysis of data
The relevance of differences in the pharmacokinetic parameters and in vitro dissolution rate profile were evaluated statistically. The data were tested by the two-way analysis of variance.
Results and discussion
The present study was designed as an attempt to facilitate transdermal delivery of 5-FU by FDCNs. Dextran-coated nanoparticles (DCNs) were prepared via reaction of coupling of amine group of amino-dextran with carboxylic group of CAP (). Coating of amino-dextran on CAP nanoparticles was confirmed by FTIR spectroscopy. The FTIR spectrum depicted a typical absorption band of stretch at 1048 cm-1 due to C-O stretching. The absorption band at 3308.44 cm−1 is determined to be the terminal hydroxyl groups in dextran and C=O stretch absorption at 1639.66 cm−1 of amide bond.
Preparation and optimization of FDCNs and FCNs
FDCNs and FCNs were prepared by nanoprecipitation method. As results shown in , the drug entrapment efficiency and particle size of nanoparticles were mainly influenced by polymer concentration and concentration of surfactants. With increasing concentration of polymer (10 to 20 mg), particle size was increased and drug encapsulation efficiency of nanoparticles was decreased. Further, increase in polymer concentration (20 to 30 mg) increased the particle size and slightly decreased the entrapment efficiency but not significantly. It may be attributed that the viscosity of dispersed phase increases, which causes poorer dispersability of the cellulose acetate phthalate solution into the aqueous phase (Quintanar-Guerrero et al., Citation1996).
Table 1. Ingredients and concentration using in the formulation of nanoparticles (Mean ± S.D., n = 3).
Pluronic F-68 concentration influences the nanoparticle characteristics, and is used in different concentrations and some batches were prepared. The results are shown in , the size of nanoparticles was decreased when the concentration of surfactant was increased (2.5 to 5.0 mg). The higher concentration of surfactant reduced the size of the nanoparticles. When the amount of Pluronic F-68 was increased it was found that the granulometric distribution became narrower. This phenomenon can be expected from the stabilizing function of a surfactant upto a definite concentration, further increase in amount of surfactant exerts little or no effect on particle and entrapment efficiency. It was also observed that when a small concentration of surfactant is used, it causes aggregation of the particles and the size of particles may increase (Quintanar-Guerrero et al., Citation1996; Feng & Huang, Citation2001).
In-vitro characterization of nanoparticles
Morphology
The atomic force microscopy technique has been widely used to determine the size, shape and surface morphology of nanoparticles. Height of the peak corresponds to size of nanoparticles. The nanoparticles were found in nanometric-size range (70-100 nm) and to be spherical in shape as observed in AFM image (). Using the AFM, CAP nanoparticles and dextran-coated CAP nanoparticles were visualized and the height was found to be relatively constant, which revealed the size of nanoparticles. Unlike other microscopic techniques, the AFM offers visualization in three dimensions. gives the tapping mode atomic force microscopic images of freshly prepared CAP nanoparticles.
Particle size, PDI and zeta potential determination
The average particle size of FCNs and FDCNs were found to be 75 ± 3 nm and 79 ± 2 nm with PDI of 0.044 and 0.052, respectively (). The results are in agreement with AFM studies, which also depicted the nanometric size of nanoparticles. Moreover, the zeta potential of FCNs and FDCNs were -2.17 and -15.4 mV, respectively. Thus, on dextran coating there was only a slight increase in particle size, PDI. Zeta potential was significantly increased. Normally, particle aggregation chances are less likely to occur for charged particles (high zeta potential) due to electric repulsion. Lower zeta potential facilitates aggregation (Potineni et al., Citation2003). The data clearly indicate that nanoparticles are stable on drug loading and hence may be suitably used as carriers for transdermal delivery of 5-FU. Particles size distribution pattern plays an important role in measuring the drug release behavior, their feasibility for transdermal administration and their fate after in vivo administration.
Table 2. Optimization study of different formulations of nanoparticles (Mean ± S.D., n = 3).
Differential scanning calorimetry
In case of 5-FU, a sharp endothermic peak was observed at 286 °C, which may be corresponding to its melting transition temperature (). In the case of CAP, endothermic peak was found at 160 ºC (). In the case of dextran, broad endothermic peak was observed between 25 and 110 °C, which may be due to the evaporation of residual water. An endothermic peak was also observed at 240 °C, corresponding to its glass transition temperature (). However, the melting endotherm of 5-FU is observed at 300 ºC in the DSC thermogram of uncoated nanoparticles (FCNs) and coated nanoparticles (FDCNs), indicating that the drug is present in crystalline form inside the nanoparticles (). The results are in good agreement with previous studies (Jelvehgari et al., Citation2011; Zu et al., Citation2011; Seema & Monika, Citation2014).
X-ray powder diffractometry
The XRD of 5-FU, dextran, CAP, uncoated and coated nanoparticles formulation are shown in . 5-FU has shown characteristic intense peaks between 2θ values of 14 °, 18 °, 19 °, 21 °, 30 ° and 35 °, which confirmed its crystalline nature (). The XRD diffractogram of dextran and CAP has no sharp peak and indicates the amorphous nature of dextran and CAP (). However, the diffractograms of FCNs and FDCNs show the typical crystalline peaks of 5-FU associated with the crystalline drug molecule, it is suggested that the FDCNs are suitable for sustained and prolonged release (). The results are in good agreement with previous studies (Jelvehgari et al., Citation2011; Zu et al., Citation2011; Seema & Monika, Citation2014).
In-vitro drug release
The release of the 5-FU from the nanoparticles showed sustained release characteristics (). The results found that much time prolongation of drug release from FDCNs than the FCNs nanoparticles system. FDCNs were sustained 5-FU more than 31 h while uncoated (FCNs) nanoparticles formulation approximately 8 h. This may be attributed to the swelling capacity of dextran and formed very strong molecular interaction between the polymer chains. The existing hydrogen bonds likely partially hinder the separation of polymer chains, allowing the entry of water, and also obstruct the outflow of the drug, leading to a prolonged drug release (Campos et al., Citation2013).
Hemolytic toxicity
Hemolytic toxicity study was measured to assess the hemotoxic effect of the FCNs and FDCNs. The hemolysis of RBCs was increased in case of FCNs in comparison with FDCNs (). Hemolysis was reduced on coating with dextran because of hydrophilic nature of dextran that leads to long circulatory and more hemocompatible nanoparticles (Bhadra et al., Citation2005). The results also may be attributed to dextran molecules penetration between the carbohydrate groups on the red cell surface and that they interact with phospholipid membranes resulting in a coating of the red cell surface with dextrans. Thus, the mechanism of dextran protection may be the interaction with incipient defects, which is facilitated by the close proximity of dextrans to defect sites (Cudd et al., Citation1989).
Primary skin irritation studies of cream containing nanoparticles (FDCNs and FCNs)
A notable difference (p <0.01) in edema or erythema was seen between the cream containing nanoparticles and the cream containing 5-FU treated group. Edema and erythema was observed lesser in order of FDCNs ≥ FCNs ≥ plain FU cream.
In-vitro permeation study cream containing nanoparticles (dextran coated and plain CAP nanoparticles)
The permeation of 5-FU from all cream formulations are illustrated in , as the definite amount of 5-FU that permeated across full-thickness to the skin over the time. The cream containing FCNs and FDCNs, were compared with the permeation of 5-FU cream. Each formulation showed slowly but continuous permeation of 5-FU through the skin over the 24 h. The permeated percentage of 5-FU from the FDCN cream, FCNs cream and 5-FU cream were found to be 95.2, 61.24 and 30.12%, respectively, through the rat skin after 24 h. The difference in the drug permeation patterns to penetrate skin layers could be related to lower ability of the FCNs and 5-FU cream in comparison to FDCNS. In fact, the Jss of cream containing FDCNs, DCNs and FU were 9.497, 6.586 and 3.228, respectively. Permeability coefficient (KP) also followed similar trend of 9.497 (FDCNs), 6.586 (FCNs) and 3.228 (FU cream) and enhancement ratio was found to be 2.94 (FDCNs), 2.04 (FCNs) and 1.0 (FU Cream) ( and ). The results are in good agreement with previous studies (ElMeshad & Tadros, Citation2011). Skin permeation results proved that the aforementioned polymer (dextran) was effective for enhancing the skin penetration of 5-FU. Dextran easily penetrated to the stratum corneum layer of the skin. Dextran shows the enhanced permeability of drug through skin (Fukushima et al., Citation2001; Djordjevic et al., Citation2003).
Table 3. In vitro permeation data of 5-FU from the FDCNs, FCNs and FU cream through newly born albino rats (Mean ± S.D., n = 3).
In-vitro cytotoxicity
The growth inhibition of FDCNs on MCF-7 cells was investigated by MTT assay. The results clearly suggested a dose-dependent cytotoxicity that is reduced cellular viability upon increasing the concentration of 5-FU (both plain 5-FU and FDCNs nanoparticles). The survival fraction of cells upon incubation of plain 5-FU and FDCNs nanoparticles in varying concentration is shown in . After 24 h of incubation, plain 5-FU as well as FDCNs nanoparticles did not show any inhibitory effect on cell growth, when the concentration of 5-FU was below 0.01 μM/mL. Further, the cell viability decreased when the concentration of 5-FU either in free form or inside the nanoparticles was increased. Almost all the cells were killed when the concentration of 5-FU was 100 μM/mL, indicating internalization of adequate 5-FU inside nuclei during this incubation period. In the concentration range of 1–100 μM/mL, FDCNs were cytotoxic to a greater degree in comparison to plain 5-FU. This is concordant with carbohydrate receptor-binding capacity exhibited by FDCNs. These results demonstrated that dextran conjugated nanoparticles' cytotoxicity induced by 5-FU to the tumor cells were dose dependent and the FDCNs had a greater cytotoxic effect on the tumor cells than did plain 5-FU. This is attributed due to dextran has excellent dispersibility in aqueous solution and endows the nanoparticles with the “stealth” property (Deng et al., Citation2010; Hao et al., Citation2013).
In-vivo study
Blood level study
Blood level study of nanoparticulate formulations were finally performed on albino rats to determine the feasibility of delivering 5-FU into blood through skin. The blood concentration versus time of FDCN and 5-FU cream are depicted in . It is attributed from the figure that FDCNs contributed remarkably towards 5-FU concentration in comparison to 5-FU cream (control) (Verma & Chandak Citation2009). Pharmacokinetic parameters for blood level study are given in . As shown, FDCNs observed higher plasma concentrations (13.50 mg/L) compared to the 5-FU cream (0.81 mg/L). There was a statistically significant difference (p < 0.05) in the AUC values between the 5-FU cream (23.82 ± 1.18 μg h/mL) and FDCNs (3652.67 ± 5.56 μg h/mL) (). This may be attributed due to hydrophilic dextran coating on CAP nanoparticles leading to enhance the penetration of drug. These results are in good agreement with earlier reported studies (Jenning et al., Citation2000).
Figure 9. Blood concentration-time profile of 5-FU after applying transdermal cream containing dextran-coated CAP nanoparticles.
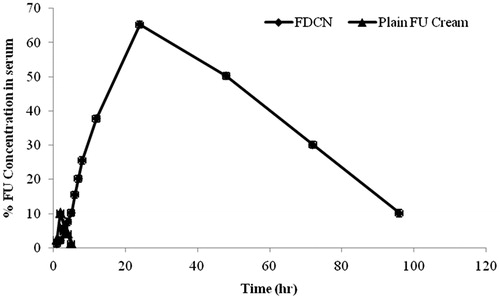
Table 4. Pharmacokinetic parameters of 5-FU from the FDCNs and FU cream through after topical administration albino rats (Mean ± S.D., n = 3).
The cumulative amounts of 5-FU in epidermis and dermis were determined to investigate the residual 5-FU within skin (). As shown in the figure, 5-FU content deposited in epidermis in case of FDCNs ranged 5–8 µg/mL, with no significant fluctuation; but 5-FU level in the case of 5-FU cream group increased significantly, (10.21 µg/mL) at 12 h (). For dermis, the 5-FU retained was 25.14 ± 0.16 µg/mL in case of FDCNs, obviously notable than that of the 5-FU cream group (10.12 ± 0.15 µg/mL) (). Inspite of achieving high level in epidermis, the relatively low level in dermis in case of 5-FU cream proved the limitation in transporting 5-FU into deep skin, let alone blood circulation.
Hematological study
The results of blood parameter show that the RBCs count was slightly decreased, WBCs count was higher in case of uncoated nanoparticles and there were no significant changes occur in case of coated nanoparticles system. Level of blood urea and serum creatinine was found lower in case of coated nanoparticles in comparison with uncoated nanoparticles (). According to the results, it was found that coated nanoparticles were shown more hemocompatible than uncoated nanoparticles. The result may be due to coating with dextran on nanoparticles, as dextran also used blood plasma expender and protective effect of dextran on RBCs (Cudd et al., Citation1989; Jeong et al., Citation2006).
Table 5. Hematological parameters in albino rats after topical administration of 5-FU-loaded CAP Nanoparticles and FU-loaded dextran-coated CAP nanoparticles (Mean ± S.D., n = 3).
Conclusion
Findings of this investigation suggest that FDCNs formulation can be considered a promising topical delivery system for 5-FU. Nanoparticles possessed a suitable size for topical route and being prepared with non-toxic and non-irritative polymer and coating agent, nanoparticles seemed to be very useful and suitable on inflamed skin and damaged skin. FDCNs formulation approach achieved the desired goal for controlled and safe delivery of drugs.
Acknowledgements
Authors are highly grateful to Principal, Guru Ramdas Khalsa Institute of Science and Technology (Pharmacy), Jabalpur, for providing necessary facility for this work. Officer-in-charge, AFM facility of Indian Institute of Technology (Indore, India) for providing AFM facilities. They are also grateful to Diya Labs Limited (Mumbai), India for providing DSC and XRD facility; ACTREC (Mumbai), India for providing in-vitro cytotoxicity assay facility.
Declaration of interest
The authors report no conflicts of interest. The authors alone are responsible for the content and writing of this article.
References
- Akimoto T, Nagase Y. (2003). Novel transdermal drug penetration enhancer: synthesis and enhancing effect of alkyldisiloxane compounds containing glucopyranosyl group. J Cont Rel 88:243–52
- Alsarra IA, Alarifi MN. (2004). Validated liquid chromatographic determination of 5-Fluorouracil in human plasma. J Chromatogr B 804:435–39
- Ansifield FJ, Schroeder JM, Curreri AR. (1962). Five years clinical experience with 5-fluorouracil. J Am Med Assoc 181:295–9
- Bhadra D, Yadav AK, Bhadra S, Jain NK. (2005). Glycodendrimeric nanoparticulate carriers of primaquine phosphate for liver targeting. Inter J Pharma 295:221–33
- Campos FdS, Cassimiro DL, Crespi MS, et al. (2013). Preparation and characterisation of dextran-70 hydrogel for controlled release of praziquantel. Braz J Pharm Sci 49:75–83
- Cudd A, Arvinte T, Schulz B, Nicolau C. (1989). Dextran protection of erythrocytes from low-pH-induced hemolysis. FEB 250:293–6
- Deng W, Li J, Yao P, et al. (2010). Green preparation process, characterization and antitumor effects of doxorubicin–BSA–Dextran nanoparticles. Macromol Biosci 10:1224–34
- Diasio RB, Harris BE. (1989). Clinical pharmacology of 5-fluorouracil. Clin Pharmacol 16:215–37
- Djordjevic J, Michniak B, Uhrich KE. (2003). Amphiphilic star-like macromolecules as novel carriers for topical delivery of nonsteroidal anti-inflammatory drugs. AAPS PharmSci 5:1–12
- ElMeshad AN, Tadros MI. (2011). Transdermal delivery of an anti-cancer drug via W/O emulsions based on alkyl polyglycosides and lecithin: design, characterization, and in vivo evaluation of the possible irritation potential in rats. AAPS PharmSciTech 12:1–9
- Feng S, Huang G. (2001). Effects of emulsifiers on the controlled release of paclitaxel (Taxol®) from nanospheres of biodegradable polymers. J Cont Rel 71:53–69
- Flynn GL. (1996). Cutaneous and transdermal delivery: processes and systems of delivery. In: Banker GS, Rhodes CT, eds. Modern pharmaceutics. New York: Marcel Dekker, 187–237
- Fukushima S, Kishimoto S, Horai S, et al. (2001). Transdermal drug delivery by electroporation applied on the stratum corneum of rat using stamp-type electrode and frog-type electrode in vitro. Biol Pharm Bull 24:1027–31
- Ghoshal K, Jacob ST. (1997). An alternative molecular mechanism of action of 5-fluorouracil. A potent anticancer drug. Biochem Pharma 53:1569–75
- Guy RH. (1996). Current status and future prospects of transdermal drug delivery. Pharm Res 13:1765–8
- Hadgraft J, Guy RH, eds. (1989). Transdermal drug delivery: development issues and research initiatives. New York: Marcel Dekker
- Hao H, Mab Q, Huang C, et al. (2013). Preparation, characterization, and in vivo evaluation of doxorubicin loaded BSA nanoparticles with folic acid modified dextran surface. Inter J Pharma 444:77–84
- Jelvehgari M, Hassanzadeh D, Kiafarar F, et al. (2011). Preparation and determination of drug-polymer interaction and in-vitro release of mefenamic acid microspheres made of cellulose acetate phthalate and/or ethylcellulose polymers. Iranian J Pharma Res 10:457–67
- Jenning V, Schafer-Korting M, Gohla S. (2000). Vitamin A-loaded solid lipid nanoparticles for topical use: drug release properties. J Cont Rel 66:115–26
- Jeong YI, Choi KC, Song CE. (2006). Doxorubicin release from core-shell type nanoparticles of poly(DL-lactide-co-glycolide)-grafted dextran. Arch Pharm Res 29:712–19
- Kligman AM, Christophers E. (1963). Preparation of isolated sheets of human stratum corneum. Arch Dermatol 88:702–5
- Kolhe P, Misra E, Kannan RM, et al. (2003). Drug complexation, in vitro release and cellular entry of dendrimers and hyperbranched polymers. Int J Pharm 259:143
- Kydonieus A. (1992). Treatise on controlled drug delivery: fundamentals, optimization and applications. New York: Marcel Dekker
- Patrick AI, Beall HD, Gilroy P, Sloan KB. (1997). Effect of vehicles on topical delivery of 5-fluorouracil (5-FU) by 1-acyl-5-FU prodrugs. Inter J Pharm 154:39–48
- Potineni A, Lynn MD, Langer R, Amiji MM. (2003). Poly(ethylene oxide)-modified poly(beta-amino ester) nanoparticles as a pH-sensitive biodegradable system for paclitaxel delivery. J Cont Rel 86:223–34
- Quintanar-Guerrero D, Fessi H, All'emann E, Doelker E. (1996). Influence of stabilizing agents and preparative variables on the formation of poly(d,l-lactic acid) nanoparticles by an emulsification-diffusion technique. Int J Pharm 143:133–41
- Ranade VV, Hollinger MA, eds. (1996). Drug delivery systems. Boca Raton, FL: CRC Press
- Seema, Monika Datta. (2014). Organoclay pluronic F-68 – montmorillonite, as a sustained release drug delivery vehicle for propranolol hydrochloride. Eur Chem Bull 3:593–604
- Surber C. (1990). Optimization of topical therapy: partitioning of drugs into stratum corneum. Pharm Res 7:1320–4
- Verma PRP, Chandak AR. (2009). Development of matrix controlled transdermal delivery systems of pentazocine: in vitro/in vivo performance. Acta Pharm 59:171–86
- Yadav AK, Agarwal A, Jain S, et al. (2010). Chondroitin sulphate decorated nanoparticulate carriers of 5-fluorouracil: development and in vitro characterization. J Biomed Nanotech 6:1–11
- Zalipsky S, Gilon C, Zilkha A. (1983). Attachment of drugs to polyethylene glycols. Eur J Polym 19:1177–83
- Zu Y, Wang D, Zhao X, et al. (2011). A novel preparation method for camptothecin (CPT) loaded folic acid conjugated dextran tumor-targeted nanoparticles. Int J Mol Sci 12:4237–49