Abstract
The purpose of this study is to synthesize a novel galactosylated cholesterol derivative, cholesterol-diethenyl decanedioate-lactitol (CHS-DD-LA) through lipase-catalyzed esterification in non-aqueous and to evaluate the preparation, pharmacokinetics and biodistribution of docetaxel (DOC) liposomes modified with CHS-DD-LA (G-DOC-L), which may actively gather at the liver compared with the conventional DOC liposomes (DOC-L) and commercial dosage form of DOC injection (DOC-i). A rapid and simple liquid chromatography-tandem mass spectrometry (LC-MS/MS) assay was developed for the determination of the DOC concentration in plasma and tissues with Taxol as the internal standard (IS). To measure the liver-targeting effect of the G-DOC-L, relative uptake rate (Re), peak concentration ratio (Ce), targeting efficiency (Te) and relative targeting efficiency (RTe) were reduced as the evaluation parameters. The results showed that the entrapment efficiency, particle size and Zeta potential of G-DOC-L was 76.8 ± 3.5%, 95.6 nm and 27.19 mV, respectively. After i.v. administration at the dose of 2.5 mg/kg in rats, a decrease in the AUC, MRT and an increase in CL (p < 0.05) were observed in the G-DOC-L group compared with DOC-L. All these results suggested that galactose-anchored liposomes could rapidly be removed from the circulation in vivo. The tissue distribution of G-DOC-L was widely different from that of DOC-L. The Re of G-DOC-L, DOC-L on liver was 4.011, 0.102; Ce was 3.391, 0.111; Te was 55.01, 3.08, respectively, demonstrating that G-DOC-L had an excellent effect on liver-targeting, which may help to improve the therapeutic effect of hepatic diseases.
Introduction
Galactoside-containing cluster ligands have high affinity for asialoglycoprotein receptors (ASGP-r) (Ashwell & Morell, Citation1974), which are found in abundance in mammalian parenchymal liver cells. ASGPR plays a key role of binding, internalization and subsequent clearance from the circulation of glycoproteins that contain terminal galactose or N-acetylgalactosamine residues (asialoglycoproteins) (Clarenburg, Citation1983; Kaufman et al., Citation1990; Spiess, Citation1990) As an efficient endocytic receptor specifically present in the liver parenchymal cell, it becomes the best liver-oriented receptor providing a good way for the treatment of liver cancer, liver function tests and liver gene directional expression (Gao et al., Citation2003; Rigopoulou et al., Citation2012). According to the physiological characteristics of the receptor, a novel active transport ligand combining the ASGPR with galactose residues as a drug carrier material can be designed, which has the advantages of a selective accumulation of higher drug concentrations, a longer duration of action and a lower dose and toxicity when applied to transport drugs in liver cells (Chou et al., Citation2010; Ueno et al., Citation2011).
In this study, a new “cholesterol-galactosidase” (CHS-DD-LA) carrier material was designed by the enzymatic coupling method. The drug carrier material consists of three parts. The first was an “Anchor” cholesterol (CHS) that was an important component of cell membranes and had good biocompatibility, and was always applied in liposomes with its function of increasing membrane fluidity and reducing the viscosity of the bilayer membrane and the permeability of membrane to phospholipids or water-soluble solute (Testylier et al., Citation1984; Ayanoglu et al., Citation1990; Ohvo-Rekila et al., Citation2002). The second, a “Targeted group” lactitol (LA) that bears a galactosyl group on one end, which was usually used as a recognition moiety for the hepatocyte-targeting carrier (Kim et al., Citation1996; Guo et al., Citation2011), a straight-chain active alcoholic hydroxylan on the other end that can be connected with the “Chain” sebacate vinyl ester (DD). The third part acted as a “cable chain” to connect CHS and the galactosidase. The “Chain” was a hydrophobic group, which can increase the affinity between galactoside and the receptor. By extending the “chain” length, the same effect can also be obtained (Biessen et al., Citation1995; Kawakami et al., Citation1998).
Many studies suggested that all macromolecules have the liver targeting features after being galactosylated. Liposomes, which have been widely used as a pharmaceutical carrier with the advantages of low immunogenicity and biodegradation, can also be seen as macromolecules, which have the characteristics of liver-targeting after being modified by our newly designed CHS-DD-LA (Zhang et al., Citation2001). The lipophilic portion of CHS-DD-LA was inserted into the liposome bilayer to orient the galactose residue to the surface of liposome bilayer. In recent decades, different types of glycolipids with lipophilic anchor moieties have been synthesized for incorporation into liposomes (Murahashi et al., Citation1997; Shimada et al., Citation1997; Rensen et al., Citation2001). Synthesized branched type galactosyl lipid derivatives to modify the liposome, which demonstrated significant liver-targeting. Kwaakmai reported two derivatives of ethyl formamide (Gal-C4-Chol), and hexyl formamide (Gal-C6-Chol), both of which had liver-targeting effect (Kawakami et al., Citation2001). Wang prepared the resveratrol ordinary liposomes and galactosylated liposomes and compared their liver-targeting. Results showed that the targeting efficiency for resveratrol liposome and liposomes modified by a galactoside and resveratrol solution was 0.29, 0.46 and 3.87, respectively, demonstrating that the liposomes modified by a galactoside showed a good liver-targeted efficiency in vivo (Wang et al., Citation2006).
There are various methods to synthesize ligand and covalent coupling (coupling the ligand with head-chain fatty acids or hydrophobic phospholipid, then reacting with a pharmaceutical carrier and forming a ligand-modified carrier) is the most common method to attach ligands to the liposome. However, most of the modified methods are chemical. And these chemical modifications require complicated and controlled multiple steps reactions and may pollute the environment. In this study, we induced enzymatic synthesis with lipase in non-aqueous medium (Zaks & Klibanov, Citation1984). The enzymatic synthesis has advantages in surface modification, such as high initial rate, high substrate conversion, high regioselectivity, environmental protection and a reduction in energy consumption. Therefore, facile and effective surface glycomodification by lipase-catalyst has become of great interest in drug-targeting carrier systems.
The aim of this study is to synthesize a new “cholesterol-galactosidase” (CHS-DD-LA), through enzymatic methods and incorporated it into liposomes containing docetaxel which is for liver targeting (Earhart, Citation1999). Investigators could establish the detection method of DOC by LC-MS/MS and compared the pharmacokinetic parameters and biodistribution after injecting DOC-i, DOC-L, G-DOC-L into rats and mice in order to verify the liver-targeting.
Materials and methods
Materials
Chemicals and reagents: Docetaxel (DOC, assay 99%, Shenzhen, China) was obtained from China Resources Sanjiu Medical & Pharmaceutical Co., Ltd. Taxol and Norethindrone (the internal standard, Guangzhou, China) was purchased from the National Institutes for Food and Drug Control (NIFDC). Egg phosphatidylcholine (PC, assay 98%), cholesterol (CH, assay 95%) were purchased from Advanced Vehicle Technology Ltd Co. (Shanghai, China). DOC injection (40 mg:1 mL) was purchased from Medicine Co., Ltd. (Jiangsu, China). Sebacic acid (assay 99%), vinylacetate (assay 98%), Candida rugosa lipase (RCL), Molecular sieves, 4 Å were all purchased from Aladdin Industrial Corporation (Shanghai, China). Novozym 435 were purchased from Novozymes Biotechnology Co., Ltd. (Tianjin, China). Lactitol Powder (lactitol purity > 98%) was provided by CTTQ Pharmaceutical Group Co., Ltd. (JiangSu, China). Pyridine, acetone and other solvents were purchased from Guangzhou Chemical Reagent Factory (Guangzhou, China). HPLC/spectral-grade reagents were used as the mobile phase in HPLC analysis, and the other reagents were of analytical grade and used without further purification. Watsons’ water was used in all experiments.
Stock solution: Stock solution of DOC and Taxol (IS) were prepared at 1 mg/mL by dissolving 1 mg drug in 1 mL methanol and then stored at −20 °C. Standard solutions were obtained by diluting the solution with methanol to give concentrations over the range of 3.25–26 000 ng/mL for preparation of the standard curve. Phosphate buffer saline (PBS) pH 6.8 was prepared with KH2PO4, 6.8045 g, NaOH 0.944 g diluted in 1000 mL of water and then through a 100 nm filter without any particulate matter.
Animals
Sprague–Dawley (SD) rats (200–250 g, male and female rats in equal number) and Kun-ming (KM) mice (18–30 g, male and female rats in equal number) obtained from the laboratory animal center of Guangzhou University of Chinese Medicine, were maintained under uniform experimental conditions (temperature 25 ± 2 °C; humidity 60 ± 5%, 12 h dark/light cycle) for one week prior to experiments. All the animals were pathogen free and allowed access to food and water freely. The experiments were carried out in compliance with the regulations of the Administration of Affairs Concerning Experimental Animals.
Lipase-catalyzed esterification of CHS-DD-LA
The synthetic substrates of CHS-DD-LA were lactitol and cholesteryl vinylhemi-sebacate (CHS-DD) which was described previously by the synthesis method (Nie et al., Citation2013; Luo et al., Citation2014). We just need to conjugate the lactitol to the CHS-DD through the method of lipase-catalyzed esterification (Zhang et al., Citation1996; Mu, Citation2003; Cheng et al., Citation2010). Prescription volume of lactitol and CHS-DD mixed in 100 mL-capped conical flask and was dissolved in non-aqueous phase reaction medium (mixed solvent of acetone and pyridine). Then, Novozym435 was added into the above solution. Detailed procedures of synthesis, isolation and purification can be referred in the paper by Nie (2014).
Preparation of Docetaxel liposomes (DOC-L) and CHS-DD-LA-modified liposomes (G-DOC-L)
The liposomes were prepared by the film dispersion method.
A mixture of PC, Ch, DOC (weight: 20, 2, 1.5 mg) was dissolved in 5 mL chloroform to form a solution, then the solvent was evaporated under vacuum using a rotator at 40–45 °C to form a thin film on the inner walls of the round-bottomed flask. The film was dried to remove residual organic solvent and vacuum dried overnight. The lipid film was finally hydrated with PBS (pH 6.8) at 50 °C until the lipid film was hydrated and a liposome was formed. Liposomes obtained were applied to the probing ultrasonication for 10 min at 220 W, and then passed through the 0.45, 0.22, 0.1 μm filter three times, respectively, at 55 °C. G-DOC-L was prepared at a similar way with an additive of 2.2 mg CHS-DD-LA (10% weight of the lipid film) (Managit et al., Citation2005).
Characterization of the liposomes
Particle size and zeta-potential of liposomes: The particle size of liposomes were measured in a dynamic light scattering spectrophotometer (Malvern Instruments, Malvern, UK) and zeta potential of liposome was determined with a laser electrophoresis zeta-potential analyzer (Malvern Instruments, UK). The concentration of DOC was determined by HPLC (Dionex, Sunnyvale, CA, USA), which consists of P680 HPLC pumps, a ASI-100 autosampler, a UV (PDA-100 Photodiode Array, Dionex, Sunnyvale, CA, USA) detector at 230 nm, and a Luna 5 μ C18(2)100A New Column (4.6 × 250 mm, Phenomenex, Torrance, CA, USA) was used for DOC separation maintained at 35 °C. Norethindrone was used as the IS (Wu et al., Citation2014). The composition of the mobile phase was acetonitrile and water (55:45) and was delivered at a flow rate of 1 mL/min with an injection volume of 10 µL.
Encapsulation efficiency (EE) was measured by Sephadex filtration method and calculated by the percentage of drug encapsulated into liposomes (Edrug) relative to the total amount of drug (Tdrug) in liposome suspension. EE% = . To determine the Tdrug in liposome suspension, 0.2 mL of DOC liposome was disrupted by an addition of 10 mL of methanol to form a clear solution. The concentration of drug was measured by using the HPLC analysis, as described above.
Edrug was evaluated after collecting liposome incorporated drug from the colloidal suspension by passing it through a Sephadex G-50 minicolumn (1.3 × 25 cm) equilibrated with distilled water. Because the liposomes containing drugs have a larger molecular and flow out firstly, we can collect the liposomes containing drugs and calculate the Edrug by HPLC (Yang et al., Citation2012).
Animal experiments
Pharmacokinetic studies
Animal experiments were performed according to the Guidelines for Animal Center of Guangzhou University of Chinese Medicine. More than 15 healthy Wistar rats were divided into three groups, including DOC-i, DOC-L and G-DOC-L. In the experiment, each rat received a 2.5 mg/kg dose of preparations by tail vein injection. Doses were based on individual animal body weight. Because the size of the liposomes would directly affect the uptake of ASGPR, controlling the particle size must be needed (Rensen et al., Citation2001). By reducing drug concentration of liposomes to some extent might help to reduce the particle size. In order to determine the drug concentration of liposome, we regarded the lower limits of quantification (LLOQ) of LC-MS/MS as the minimum concentration (Cmin) of DOC in plasma and regarded it as the plasma drug concentration after 99% of which was metabolized in vivo of rat. Calculated the initial drug concentration of DOC in plasma (C0) according to the equation: Cmin = 99% × C0, and with the formula of C0 = , the apparent volume of distribution (Vd) was calculated by related references (Tong et al., Citation2006; Liang et al., Citation2007; Yuan et al., Citation2010; Li et al., Citation2011, Citation2012; Qu et al., Citation2012; Raju et al., Citation2013). Expanding 10-fold on the basis of the calculated dose. We made decision of the viable drug concentration of lipsomes (0.15 mg/mL, being consistent with the prescription of liposome preparation) and determined the injection dose of animals. After injection, about 500 μL blood samples were taken from the retro-orbital plexus at various times (0, 0.083, 0.25, 0.5, 1, 2, 4, 6, 8, 10, 24 h) and immediately centrifuged at 3000 rpm for 10 min then stored at −70 °C until analysis. The DOC concentration was detected by LC-MS/MS, and the parameters was measured by a non-compartmental analysis using the DAS2.0 computer program (the Committee of Mathematic Pharmacology, the Chinese Society of Pharmacology, Hefei, China).
Tissue distribution studies
More than 100 KM mice were divided into three groups randomly, including DOC-i, DOC-L and G-DOC-L, and then received an i.v. dose (3.5 mg/kg) of preparations. Each group of mice was sacrificed at 10, 20, 30 and 60, 120, 240 min after intravenous injection. Tissues including heart, liver, spleen, lung and kidney were collected, rinsed in saline, blotted excess fluid, weighed and stored at −70 °C until analysis.
Plasma and tissue samples preparation
Precisely 150 µL of rat plasma or 100 µL of mice plasma were added to 100 µL (800 ng/mL) taxol solutions as IS and 3 mL methyl tert-butyl ether (Kuyukina et al., Citation2001). The mixture was vortexed for 3 min, and then centrifuged at 8000 r/min for 10 min. Quantitative amount of the supernatant was then transferred in 5 mL tubes and dried in a vacuum oven at low temperature. The residue was re-dissolved in 100 μL methanol: 0.1% formic acid (80:20), then centrifuged at 13 000 r/min for 15 min. 10 μL of the supernatant was sucked out for the analysis.
Tissue samples were homogenized in saline (tissue–water ratio of 1:5, w/v). Aliquots of the homogenate (300 μL) were sucked, and prepared as plasma. Finally, an aliquot of 10 μL of the supernatants was injected into the LC-MS/MS system for the analysis.
Analysis method
LC-MS/MS conditions
The HPLC system comprised 4-mode Surveyor LC pumps, a Surveyor autosampler, a CBM-20 system controller and a Surveyor PDA. A BDSHYPERSIL C18 column (5 μm, 2.1 × 50 mm, Thermo Scientific, Boston, MA, USA) was used for separation. The mobile phase were methanol:0.1% formic acid (80:20) at the rate of 0.3 mL/min, and the temperature of column was 40 °C.
Mass analysis was conducted by a Thermo Scientific TSQ Quantum MS/MS system equipped with electrospray ionization (ESI) interface operated in the positive ionization mode. Quantification was accomplished in selective reaction monitoring (SRM) by monitoring the transition of m/z 830.4 → 549.2 for docetaxel and m/z 876.4 → 307.8 for taxol (the IS). The spray voltage, the temperature of capillary, sheath gas pressure and auxiliary gas pressure were set at 3500 °C, 320 °C, 35 psi and 10 psi, respectively. The collision energy was 30 V, and scanning time was 0.5 s (Mortier et al., Citation2005; Tong et al., Citation2006; Yamaguchi et al., Citation2012; Wang et al., Citation2013).
Statistical analysis
All rat pharmacokinetic parameters, including AUC0–∞, MRT0–∞, Vd, CL, T1/2 were analyzed by using a non-compartment model with DAS2.0 and forming plasma concentration–time (C–T) curve from which we can get the tendency of the preparations in vivo.
Tissue distribution data were also evaluated by the DAS2.0. From the parameters we can compare the targetability of each organ. There were four parameters for the evaluation of liver targetability according to the Pharmacopoeia of the People's Republic of China 2010.
Relative uptake rate (Re) =
. Re > 1 indicates targetability, while Re ≤ 1 indicates no targetability. The bigger Re is, the better the targeted effect is; i refers to the no. i tissue, p and s refer to the liposomes preparation and drug solution, respectively. AUC, area under concentration–time curve was calculated using the trapezoidal method according to the C–T curve.
Targeting efficiency (Te%) =
*100. Te means the selectively rate of the targeted tissue.
AUCtargeting refers to AUC of the targeted tissue and AUCno-targeting means other tissues except targeted tissues.
Relative targeting efficiency (RTe) =
. RTe shows the multiples of targeting enhancement of liposome formulations compared to drug solution.
Peak concentration ratio (Ce) =
. Ce means the change in degree of preparation of tissue distribution. Large value of Ce means big change in biodistribution.
Cmax refers to the maximum concentration of DOC in tissue sample according to the C–T curve of tissue.
Statistical comparison of the pharmacokinetic parameters was conducted using the one-way analysis of variance (ANOVA). Significance level was set at 0.05 (p < 0.05).
Results and discussion
Method validation
It was possible for us to choose the IS and optimize the MS and chromatography conditions according to previous studies. Before detection, the method validation was needed.
Specificity
Specificity was assessed by analyzing six different samples of blank matrix with and without DOC and IS. There was no endogenous interference from plasma, tissue homogenates of heart, liver, spleen, lung and kidney.
Lower limit of quantitation and standard curve
The linearity of LC-MS/MS method for the determination of DOC was evaluated by a calibration curve in the range of 3.25–26 000 ng/mL. The calibration curve was obtained by plotting the ratio of chromatographic peaks area (DOC/IS) versus the concentration of DOC. Least squares linear regression analysis was used to determine the slope, intercept and correlation coefficient (r2). And r2 ≥ 0.999 was required. shows linear equations and r2 of plasma and all tissues. The LLOQ was 3.25 ng/mL
Table 1. Standard curves, correlation coefficient and linear range of DOC in plasma and tissues of mice.
To evaluate the precision, at least five QC samples of three different (low, middle, high) concentrations of DOC were processed and injected on a single day (intra-day) and on different days (inter-day). The variability of DOC determination was expressed as the relative standard deviation (R.S.D.%) which should not exceed 15% at all concentrations. The results were shown in . The intra-day precision (RSD) ranged from 2.4 to 12.1% and the inter-day precision ranged from 3.8 to 14.2%. Both of them were qualified. Extraction recoveries of DOC were determined on three levels of concentrations and measured by comparing the analyte/IS peak areas obtained from extracted samples with post-extracted samples at the same concentrations, which are also shown in .
Table 2. The inter-(n = 5) and intra (n = 5)-day precision and recovery of the method for determination of DOC in plasma and tissues samples.
Synthesis of cholesterol-diethenyl-decanedioate-lactitol (CHS-DD-LA)
The CHS-DD-LA was synthesized from substrates CHS, DD, LA by a two-step trans-esterification under the condition of lipase-catalytic. First, lipase-catalytic trans-esterification was CHS-DD from cholesterol and divinyl sebacate in non-aqueous phase with Candida Rugosa Lipase (RCL). The 3-OH of the CHS has the characteristics of esterification that can form a relatively stable structure with a P-π conjugated system and because of the excessive substrate, the formation of diester compound can be inhibited. Second, lipase-catalytic trans-esterification was conjugating the lactitol to the CHS-DD with Novozym435 in non-aqueous phase which belongs to the synthesis of sugar ester (Barenholz, Citation2001). The basic structure of LA was 4-O-β-D-galactopyranosyl-D-sorbitol comprising galactose residues that can be specifically recognized by the ASGPR. Both steps generated vinyl alcohol, which can turn into acetaldehyde through enol tautomerism. Acetaldehyde with a low boiling point can easily be volatile, so the reaction was not reversible and the enzymatic reaction can be more thoroughly taken place. The synthesis route can be understood by referring to the PhD thesis of Nie (2014). And the chemical formula is (5-Cholesten-3β-yl)[(4-O-β-d-galactopyranosyl)d-Glucitol-6] Decanedioic acid. The chemical structure of CHS-DD-LA has been confirmed by IR, 1H-NMR, 13C-NMR and ESI-MS. All the data proved it was the object substance.
Characterization of liposomes
For both liposome formulations prepared, the drug entrapment efficiency was more than 70%, and the mean particle size was about 100 nm (). This suggested that the incorporation of CHS-DD-LA had small effect on the characteristic parameters of G-DOC liposomes.
Table 3. Entrapment efficiency, particle size and zeta-potential of freshly prepared liposome suspensions.
Table 4. Pharmacokinetic parameters of DOC-i, DOC-L and G-DOC-L in rat plasma following intravenous administration (n = 5).
Pharmacokinetics
The mean plasma C–T curves of DOC after i.v. administration of DOC-i, DOC-L and G-DOC-L are shown in . There was a steep drop of plasma concentrations in G-DOC-L during 5 min, and the other two show slow decline curves, though the DOC-L decreased a little more rapid. Compared with DOC-i, DOC-L formulations were removed more slowly from the circulation, which can validate the conclusions that liposomes prolong the active time (Ishida et al., Citation2004). However, there was a rapid clearance from the systemic circulation of G-DOC-L. The key pharmacokinetic parameters of intravenous administration of different docetaxel formulations calculated from these plasma concentration–time data are presented in . As it was shown, DOC-L resulted in an increase in the AUC, MRT, and correlated with a decrease in the CL (p < 0.05). This means that it has a longer circulation and better bioavailability than DOC-i. These results were ascribed to the protection of the lipid bilayer membranes and slow drug release from liposomes. But for the G-DOC-L, the parameters were irregular. The parameters AUC, MRT declined with a rising value of CL, which demonstrated that there was a rapid removal of plasma concentration from blood. A further experiment of biodistribution was needed to figure out whether the drug was metabolized or rapidly gathered in the organs.
Tissue distribution
Theoretically, the galactocylated lipids with galactose cluster on the surface of liposomes have a higher uptake rate by liver parenchymalcells, and many studies have demonstrated the same (Sliedregt et al., Citation1999; Opanasopit et al., Citation2001). For the purpose of proving whether the new ligand liposomes have a liver targetability or not, the in vivo fate of DOC was evaluated after administration of DOC-i, DOC-L and G-DOC-L. The C–T profile of different tissues of KM-mice after intravenous administration of preparations at a dosage of 3.5 mg/kg is depicted in . It shows the tendency of preparations in vivo of mice. It can be known that the DOC of G-DOC-L rapidly distributed in tissue and reach a maximum value at 10 min. The plasma concentration of G-DOC-L decreased more significantly than the other two. That was consistent with the results of pharmacokinetics.
Figure 2. Concentration–time profile of DOC in heart (A), liver (B), spleen (C), lung (D), kidney (E) and plasma (F) of mice after i.v. administration of DOC-i, DOC-L and G-DOC-L at dose to 3.5 mg/kg of DOC (n = 5).
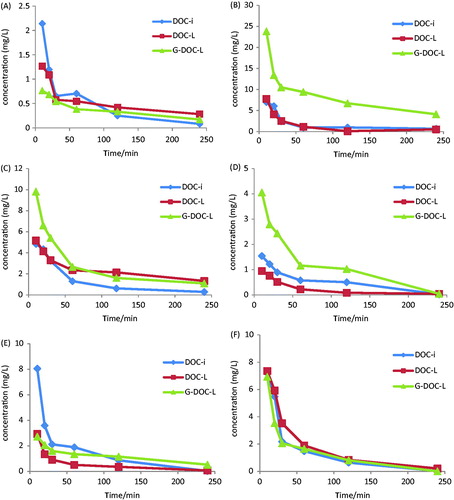
The concentrations of DOC in tissues at 10, 20, 30, 60, 120, 240 min are depicted in . At various time the concentration of DOC released from G-DOC-L in liver was the highest, demonstrating the highest uptake rate by liver and providing the possibility of liver targetability. The induced four parameters can illustrate the liver targetability. Re, Te, Rte, Ce were calculated with the parameters of AUC0–240 and Cmax in and the results are listed in .
Figure 3. Concentration of DOC in heart (A), liver (B), spleen (C), lung (D), kidney (E) and plasma (F) of mice at various time points after i.v. administration of DOC-i, DOC-L and G-DOC-L at dose to 3.5 mg/kg of DOC (n = 5).
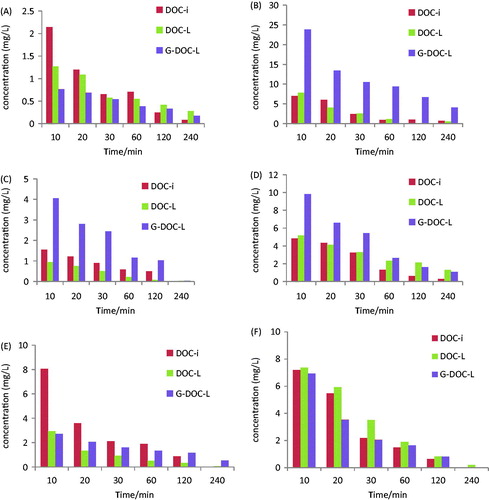
Table 5. Pharmacokinetic parameters of DOC-i, DOC-L and G-DOC-L in plasma and various tissues of mice after intravenous administration (n = 5).
Table 6. The Te (%) of DOC-i in plasma and various tissues of mice.
Table 7. Targeting parameters of DOC-L in plasma and various tissues of mice.
Table 8. Targeting parameters of G-DOC-L in plasma and various tissues of mice.
G-DOC-L showed excellent liver-targeting efficiency with Re: 4.011; Te(%): 55.01; Rte:2.115 and Ce: 3.391 compared with DOC-L. The biodistribution studies have illustrated that galactose residues bearing liposomes were cleared more rapidly from circulation and recognized by hepatic ASGPR than were uptaken into the liver parenchymal cell after intravenous infusion (Nishikawa et al., Citation1995).
Conclusion
In this research, we have demonstrated through the pharmacokinetics and biodistribution studies of G-DOC-L, which incorporate CHS-DD-LA in DOC-liposomes, that are effectively selected by liver compared with the conventional injection and liposomes, with the verification of Re, Te(%), RTe, Ce in liver, all of which were the maximum values comparing other tissues. This will make it possible to improve the cure rate of liver disease. The use of LC-MS/MS method for the determination of DOC in plasma and tissues is sensitive, rapid and reliable. The synthesis system of CHS-DD-LA through lipase-catalysts is not only cost-effective, environment friendly but also regioselective, simple, reproducible and non-toxic.
This research provides the basis for evaluating the clinical potential of G-DOC-L.
Declaration of interest
This work was financially supported by the National Nature Science Foundation of China (no. 30772790) and the Ministry of Education funds tutor project (no. 20134425110010).
References
- Ashwell G, Morell AG. (1974). The role of surface carbohydrates in the hepatic recognition and transport of circulating glycoproteins. Adv Enzymol Relat Areas Mol Biol 41:99–128
- Ayanoglu E, Chiche BH, Beatty M, et al. (1990). Cholesterol interactions with tetracosenoic acid phospholipids in model cell membranes: role of the double-bond position. Biochemistry 29:3466–71
- Barenholz Y. (2001). Liposome application: problems and prospects. Curr Opin Colloid Interface Sci 6:66–77
- Biessen EA, Beuting DM, Roelen HC, et al. (1995). Synthesis of cluster galactosides with high affinity for the hepatic asialoglycoprotein receptor. J Med Chem 38:1538–46
- Cheng Y, Wu W, Zhang DQ, et al. (2010). Enzyme-catalyzed synthesis of ASGPR ligand-targeted modifier in non-aqueous medium. Acta Pharmaceutica Sinica 45:1134–8
- Chou YT, Lin HH, Lien YC, et al. (2010). EGFR promotes lung tumorigenesis by activating miR-7 through a Ras/ERK/Myc pathway that targets the Ets2 transcriptional repressor ERF. Cancer Res 70:8822–31
- Clarenburg R. (1983). Asialoglycoprotein receptor is uninvolved in clearing intact glycoproteins from rat blood. Am J Physiol 244:G247–53
- Earhart RH. (1999). Docetaxel (Taxotere): preclinical and general clinical information. Semin Oncol 26:8–13
- Gao S, Chen J, Xu X, et al. (2003). Galactosylated low molecular weight chitosan as DNA carrier for hepatocyte-targeting. Int J Pharm 255:57–68
- Guo BH, Cheng Y, Lin LP, et al. (2011). Preparation and characterization of galactose-modified liposomes by a nonaqueous enzymatic reaction. J Liposome Res 21:255–60
- Ishida E, Managit C, Kawakami S, et al. (2004). Biodistribution characteristics of galactosylated emulsions and incorporated probucol for hepatocyte-selective targeting of lipophilic drugs in mice. Pharm Res 21:932–9
- Kaufman SS, Blain PL, Park JH, et al. (1990). Role of microfilaments in asialoglycoprotein processing in adult and developing liver. Am J Physiol 259:G639–45
- Kawakami S, Munakata C, Fumoto S, et al. (2001). Novel galactosylated liposomes for hepatocyte-selective targeting of lipophilic drugs. J Pharm Sci 90:105–13
- Kawakami S, Yamashita F, Nishikawa M, et al. (1998). Asialoglycoprotein receptor-mediated gene transfer using novel galactosylated cationic liposomes. Biochem Biophys Res Commun 252:78–83
- Kim KM, Kim C, Min K, et al. (1996). Preparation and lectin binding characteristics of N-stearyl lactobionamide liposomes. Int J Pharm 128:65–71
- Kuyukina MS, Ivshina IB, Philp JC, et al. (2001). Recovery of Rhodococcus biosurfactants using methyl tertiary-butyl ether extraction. J Microbiol Methods 46:149–56
- Li J, Xu H, Ke X, et al. (2012). The anti-tumor performance of docetaxel liposomes surface-modified with glycyrrhetinic acid. Drug Target 20:467–73
- Li X, Tian X, Zhang J, et al. (2011). In vitro and in vivo evaluation of folate receptor-targeting amphiphilic copolymer-modified liposomes loaded with docetaxel. Int J Nanomedicine 6:1167–84
- Liang G, Jia-Bi Z, Fei X, et al. (2007). Preparation, characterization and pharmacokinetics of N-palmitoyl chitosan anchored docetaxel liposomes. J Pharm Pharmacol 59:661–7
- Luo LH, Nie H, et al. (2014). Modeling and optimization of lipase-catalyzed synthesis of cholesteryl vinyl hemi-sebacate by response surface methodology. Chin Pharm J 49:1426–31
- Managit C, Kawakami S, Yamashita F, et al. (2005). Effect of galactose density on asialoglycoprotein receptor-mediated uptake of galactosylated liposomes. J Pharm Sci 94:2266–75
- Mortier KA, Renard V, Verstraete AG, et al. (2005). Development and validation of a liquid chromatography-tandem mass spectrometry assay for the quantification of docetaxel and paclitaxel in human plasma and oral fluid. Anal Chem 77:4677–83
- Mu XL. (2003). Synthesis of iso-amyl acetate catalyzed by enzymes. Hubei Chemical Industry 20:12–14
- Murahashi N, Ishihara H, Sasaki A, et al. (1997). Hepatic accumulation of glutamic acid branched neogalactosyl lipid modified liposomes. Biol Pharm Bull 20:259–66
- Nie H, Zheng PJ, Luo LH. (2013). Lipase-catalyzed synthesis of cholesteryl vinyl hemi-sebacate for selective targeting of liposomes in organic media. Chin Trad Herb Drugs 44:3289–95
- Nishikawa M, Miyazaki C, Yamashita F, et al. (1995). Galactosylated proteins are recognized by the liver according to the surface density of galactose moieties. Am J Physiol 268:G849–56
- Ohvo-Rekila H, Ramstedt B, Leppimaki P, et al. (2002). Cholesterol interactions with phospholipids in membranes. Prog Lipid Res 41:66–97
- Opanasopit P, Shirashi K, Nishikawa M, et al. (2001). In vivo recognition of mannosylated proteins by hepatic mannose receptors and mannan-binding protein. Am J Physiol Gastrointest Liver Physiol 280:G879–89
- Qu G, Wu X, Yin L, et al. (2012). N-octyl-O-sulfate chitosan-modified liposomes for delivery of docetaxel: preparation, characterization, and pharmacokinetics. Biomed Pharmacother 66:46–51
- Raju A, Muthu MS, Feng SS. (2013). Trastuzumab-conjugated vitamin E TPGS liposomes for sustained and targeted delivery of docetaxel. Expert Opin Drug Deliv 10:747–60
- Rensen PCN, Sliedregt LAJM, Ferns M, et al. (2001). Determination of the upper size limit for uptake and processing of ligands by the asialoglycoprotein receptor on hepatocytes in vitro and in vivo. J Biol Chem 276:37577–84
- Rigopoulou EI, Roggenbuck D, Smyk DS, et al. (2012). Asialoglycoprotein receptor (ASGPR) as target autoantigen in liver autoimmunity: lost and found. Autoimmun Rev 12:260–9
- Shimada K, Kamps JA, Regts J, et al. (1997). Biodistribution of liposomes containing synthetic galactose-terminated diacylglyceryl-poly(ethyleneglycol)s. Biochim Biophys Acta 1326:329–41
- Sliedregt LA, Rensen PC, Rump ET, et al. (1999). Design and synthesis of novel amphiphilic dendritic galactosides for selective targeting of liposomes to the hepatic asialoglycoprotein receptor. J Med Chem 42:609–18
- Spiess M. (1990). The asialoglycoprotein receptor: a model for endocytic transport receptors. Biochemistry 29:10009–18
- Testylier G, Clement G, Leterrier F. (1984). Photochemical interactions of chlorpromazine with phospholipids and cholesterol in artificial and natural membranes. Photochem Photobiol 39:277–80
- Tong X, Zhou J, Tan Y. (2006). Liquid chromatography/tandem triple-quadrupole mass spectrometry for determination of paclitaxel in rat tissues. Rapid Commun Mass Spectrom 20:1905–12
- Ueno S, Mojic M, Ohashi Y, et al. (2011). Asialoglycoprotein receptor promotes cancer metastasis by activating the EGFR-ERK pathway. Cancer Res 71:6419–27
- Wang L, Zhang SQ, Gu Y. (2006). Liver-targeted Resveratrol Liposome. Pharmaceut J PLA 22:241–5
- Wang X, Song L, Li N, et al. (2013). Pharmacokinetics and biodistribution study of paclitaxel liposome in Sprague-Dawley rats and Beagle dogs by liquid chromatography-tandem mass spectrometry. Drug Res (Stuttg) 63:603–6
- Wu W, Cheng Y, Guo BH, et al. (2014). Pharmacokinetics of liver-targeted docetaxel liposomes modified with 6-O-acyl-D-galactose esters in rabbits. Biomed Rep 2:545–8
- Yamaguchi H, Fujikawa A, Ito H, et al. (2012). A rapid and sensitive LC/ESI-MS/MS method for quantitative analysis of docetaxel in human plasma and its application to a pharmacokinetic study. J Chromatogr B Analyt Technol Biomed Life Sci 893–894:157–61
- Yang S, Chen J, Zhao D, et al. (2012). Comparative study on preparative methods of DC-Chol/DOPE liposomes and formulation optimization by determining encapsulation efficiency. Int J Pharm 434:155–60
- Yuan Z, Chen D, Zhang S, et al. (2010). Preparation, characterization and evaluation of docetaxel-loaded, folate-conjugated PEG-liposomes. Yakugaku Zassh 130:1353–9
- Zaks A, Klibanov AM. (1984). Enzymatic catalysis in organic media at 100 degrees C. Science 224:1249–51
- Zhang NX, Cao SG, et al. (1996). The research of sugar esters synthesized by lipase-catalyzed in organic phase. Chem J Chin Univ 17:1404–7
- Zhang X, Simmons CG, Corey DR. (2001). Liver cell specific targeting of peptide nucleic acid oligomers. Bioorg Med Chem Lett 11:1269–72