Abstract
Context: Loxoprofen (LOXO) is a non-steroidal anti-inflammatory drug. Repeated oral administrations induce gastrointestinal side effects. Patches are a promising alternative.
Objective: The aim of this study was to investigate the effects of organic amines on the skin permeation of LOXO and finally design a patch with a comparable permeation profile and pharmacodynamic effects to the commercial LOXONA® plaster.
Materials and methods: The effects of organic amines were assessed by flux values of LOXO from isopropyl myristate (IPM), using horizontal diffusion cell and rabbit skin. FTIR spectroscopy was used to confirm ion-pair formation. Anti-inflammatory and analgesic activity assessments were performed in the adjuvant arthritis rat model and acetic acid-induced writhing syndrome in mouse, separately.
Results and discussion: Results showed that triethylamine (TEA) was the most potential candidate in IPM, with the highest flux of 499.75 ± 32.40 µg/cm2/h. In patch, the highest flux of 369.37 ± 34.32 µg/cm2/h was still obtained by LOXO–TEA. Combined with penetration enhancers, the cumulative amounts were further increased in presence of 5% IPM, which exhibited a flux of 840.04 ± 66.38 µg/cm2/h as two times of the commercial one. Ultimately, anti-inflammatory and analgesic activity assessment presented that a comparable pharmacodynamic activity with the commercial one could be obtained by the patch we designed. Additionally, we also found that LOXO patch applied topically exerted a systemic effect, and the effect was dose-dependent.
Conclusion: It was feasible for LOXO patch design by combination of ion-pair technology and chemical enhancers.
Introduction
Loxoprofen (LOXO), a prodrug in the class of short-acting non-steroidal anti-inflammatory drugs (NSAIDs) (), which needs to be converted to its active metabolite () in the liver or partly in the blood and dermal layer of the skin (Koo et al., Citation2005; Murakami, Citation2008), is widely prescribed for the treatment of pain and inflammation in Japan and 14 other countries (Ichikawa et al., Citation2008). Although clinical studies have suggested that it is safer than other NSAIDs, repeated oral administrations induce adverse events yet such as the main side effect of gastroduodenal mucosal damage (Nakase et al., Citation1999), which mainly due to its high-plasma concentration and lack of selectivity for cyclooxygenase.
Transdermal drug delivery system (TDDS) is just a promising alternative for oral therapy of LOXO. The advantages of TDDS are well summarized by Marwah et al. (Citation2014). There have already been two percutaneous absorption products of loxoprofen sodium (LOXONa) in commercial that are LOXONA® plaster of Korea and Loxonin® Tape of Japan. However, with a hydrophilic quality, the skin permeability of LOXONa (log Ko/w = −1.13) is open to doubt, as the barrier of skin, stratum corneum (SC), provides a lipophilic environment (Barry, Citation2001). Thus, to achieve effective blood concentration, increasing the patch size or adding a lot of chemical enhancers may be implemented, but that will inevitably affect patients’ compliance and increase the incidence of skin irritation. Practically, the commercial products have an area of 70 cm2, which is far beyond the reasonable size (that is, a surface area of ≤40 cm2) of an ideal patch (Tan & Pfister, Citation1999).
Many strategies have been used to overcome the barrier of skin. Among them, ion-pair is a modest chemical approach to enhance the skin penetration of ionized drugs with counter ions by coulomb attraction rather than a covalent bond. Counter ions can provide a direct influence on physicochemical properties of the parent drug, such as lipophilicity. And some changes may be beneficial for a drug to across the skin (Fang et al., Citation2003; Ma et al., Citation2009; Xi et al., Citation2012; Pawar et al., Citation2014). Additionally, chemical enhancers are also a conventional method to enhance the skin penetration of drugs (Barry, Citation2001). Combined ion-pair technology and penetration enhancers may in some extent maximize the flux of ionized drugs. Cheong & Choi (Citation2003) have utilized ethanolamine salts in combination with various enhancers to maximize the flux of piroxicam from a pressure sensitive adhesive (PSA) matrix across hairless mouse skin.
In this study, the effect of ion-pair formation between LOXO and six different organic amines on the percutaneous absorption of LOXO from isopropyl myristate (IPM) and PSA matrix across rabbit skin would be further discussed. Combined with penetration enhancers, a patch with a comparable permeation profile and pharmacodynamic effects to the commercial LOXONA® plaster was designed.
Materials and methods
Materials
LOXONa was purchased from Weihai Disu Pharmaceutical Co., Ltd. (Shandong, China). LOXO was prepared in our laboratory by a method described in the patent US5532259, and the purity was greater than 96% by HPLC. LOXONA® plaster (50 mg/7 × 10 cm2) was provided by DAE HWA Pharmaceutical Co., Ltd. (Seoul, Korea). N-(2'-hydroxyethanol)-piperidine (HEPP), ethanolamine (EtA), diethanolamine (DEtA), triethanolamine (TEtA), diethylamine (DEA), triethylamine (TEA), menthol (MT), Span 80 (SP), 2-(2-ethoxyethoxy) ethanol (TP), laurocapram (AZ), oleic acid (OA) were purchased from Yuwang Pharmaceutical Co., Ltd (Shandong, China). Isopropyl myristate (IPM) was supplied by China National Medicines Co., Ltd. (Shanghai, China). Phosphate-buffered saline (PBS, pH 7.4) containing 1.36 g KH2PO4 and 0.316 g NaOH in 200 ml distilled water was prepared according to the Chinese Pharmacopoeia (Citation2005). Acrylic pressure sensitive adhesives containing carboxyl group were synthesized in our laboratory. Complete Freund’s adjuvant (CFA) was obtained from Sigma-Aldrich Co., LLC (St. Louis, MO). All the other chemicals were of the highest reagent grade available.
Animals
Rabbits (male, 2.0–2.2 kg), Wistar rats (male, 180–220 g) and KM mice (male, 18–22 g) were supplied by the Experimental Animal Center of Shenyang Pharmaceutical University (Shenyang, China). All animal experiments were performed in accordance with the NIH Guidelines for the Care and Use of Laboratory Animals and also with the guidelines for animal use published by the Life Science Research Center of Shenyang Pharmaceutical University. All efforts were made to limit the amount of animals used.
The preparation of organic amine salts
Equimolar amount of LOXO and each organic amine were dissolved in acetone and stirred for 2 h at room temperature. The solvent was then removed by vacuum rotary evaporation. Subsequently, the products were dried in a vacuum for 12 h.
FTIR characterization
The interactions between LOXO and organic amines were investigated by dissolving 20 mg/mL aforementioned LOXO and organic amines products in anhydrous chloroform and stirring for 12 h. The samples were centrifuged at 16 000 rpm for 10 min, and the supernatant was analyzed using a validated Fourier transform infrared spectroscopy (FTIR) method. Spectra were recorded with 5 scan number on a Spectrum 100 FTIR spectrometer (PerkinElmer, Waltham, MA) on line with a computer. The resolution was 4 cm−1.
Apparent partition coefficient
Apparent partition coefficient described as log Ko/w was determined by a shake-flask method. N-octanol and water were pre-saturated for 24 h and then separated them from each other. LOXO and organic amines products were dissolved in the water and mixed with the organic phase. Subsequently, the two phase solution was shaken at 25 ± 0.5 °C for 48 h. Finally, the aqueous phase and organic phase were centrifuged separately at 16 000 rpm for 10 min. Drug concentrations in these two phases were analyzed using a validated HPLC method.
Apparent solubility measurement
An excess amount of LOXO or its amine salt was added to IPM and shaken at 32 ± 0.5 °C for 48 h. Then the solutions were centrifuged at 16 000 rpm for 5 min, and the supernatant was analyzed by HPLC after appropriate dilution.
Skin sample preparation
The preparation of skin sample was in accordance with Xi et al. (Citation2012). After anesthetized with urethane (20% w/v, i.v.), the hair of rabbits in abdomen was carefully removed. After sacrificed, the full thickness of skin was excised, followed by carefully removing the sub-dermal tissue. The side of SC was wiped gently with absorbent cotton dipped in distilled water. The excised skin was stored at −70 °C within 1 week after preparation. Before the experiments, the skin was thawed to room temperature for at least 10 h.
Skin permeation test
The experiment was conducted according to Jiang et al. (Citation2014). The prepared skin sample was mounted between the two half-cells of a horizontal diffusion cell (Volume = 3.0 mL and Effective diffusion area = 0.95 cm2), with the dermal side faced the receptor compartment. The receptor compartment was filled with pH 7.4 phosphate buffer saline (PBS) and the donor compartment with the suspensions of LOXO with or without organic amines about twice the solubility in IPM, respectively. The excess solid of drug in the suspension ensured that drug was always in saturation conditions. Both compartments were continuously stirred at about 600 rpm, and the temperature was maintained constantly at 32 ± 0.5 °C using a thermostatic water pump. For solution system, samples of 2 mL were withdrawn every hour for 8 h from the receptor compartment and replaced with fresh receptor medium immediately to maintain a constant volume, while for patches sampling time were exchanged to every 2 h for 12 h, and the patches were regarded as the donor compartments. The samples were analyzed using a validated HPLC method.
All in vitro experiments were carried out in triplicate or quadruplicate. The cumulative amount of drug permeated per unit area (Q) versus time was plotted. The steady-state flux (J, μg/cm2/h) was obtained from the slope of the linear portion of the plot. The enhancing effect in terms of enhancement ratio (ER) was described as the ratio of Q resulted from adding various organic amines or chemical enhancers against that without them.
Preparation of patches
The drug-in-adhesive patches were prepared by dissolving the drug, PSA, penetration enhancers in ethanol and then stirred thoroughly to obtain a homogeneously coating solution. The PSA containing carboxyl group was synthesized in our laboratory according to Kotiyan & Vavia (Citation2002). 2-ethyl acrylate and acrylic acid were selected as the monomers. The dry weight of the final product was 36% (w/w), and the viscosity was 14 000 MPa·s. Using a laboratory coating unit (TB-1, KaiKai Co. Ltd., Shanghai, China), the obtained solution was coated onto a silicone-coated release liner (Shanghai Fupeng Adhesive Products Co. Ltd., Shanghai, China). After removal of the solvent by oven-dried at 60 °C for 10 min, a fabric backing film (Hangzhou Xiaoshan Johnson Cloth Factory, Zhejiang, China) was laminated on it. In order to compare with the LOXONA® plaster, drug content and thickness were designed equally to it.
Quantitative analysis
The quantitative determination of LOXO was according to JP15 with a little modification. A Hitachi HPLC system (pump L-2130, UV–Vis detector L-2420, autosampler L-2200, T2000L workstation) and a Diamonsil ODS (5 μm, 200 × 4.6 mm) were used. The flow rate was 1.0 mL/min. External standard method was performed in this study. Other conditions were the same as JP15.
Pharmacodynamics study
Assessment of anti-inflammatory activity
The procedure of anti-inflammatory activity assessment was based on Xi et al. (Citation2013). Wistar rats were divided into four groups each consisting of six animals. Group A was subcutaneously injected with 0.1 mL saline on bilateral hind paws of each rat as control, while Groups B, C and D were injected with 0.1 mL CFA. The volumes (V) of bilateral hind paws of each rat were measured by a water plethysmometer (YLS-7B, Jinan Yiyan Technology Co. Ltd., Shandong, China) before injection, at 0.5, 4 and 6 days after injection and every 2 days for 8 days after patches of 10 cm2 application (Group A with no patches, Group B with placebo patches, Group C with self-designed optimal patches and Group D with LOXONA® plasters) at left hind paws. As the commercial one was used once a day, the patches used for anti-inflammatory activity assessment were also changed once a day. The paw swelling degree (S) was calculated using the following formula:
where Vn and Vt were the paw volumes before and after the injection, respectively.
Acetic acid-induced writhing syndrome
KM mouse were divided into six groups each consisting of ten animals. The test was performed according to the method of Jin et al. (Citation2001). Abdomen hair was removed before the experiment. Administration scheme was as follows: Group A was as a control; Group B each mice was applied with placebo patch (6 cm2); Groups C and D were administered with self-designed patch (6 cm2) and LOXONA® plasters (6 cm2), respectively. Groups E and F were administered with self-designed patch (3 cm2) and LOXONA® plasters (3 cm2), separately. The patches were removed after 2 h later, and then each mouse was injected with acetic acid diluted with saline (0.6%, v/v). Five minutes later, the numbers of writhing within 20 min were counted. The pain inhibition ratio (PIR, %) was calculated from the following formula:
where WRF was the writhing response frequency.
Statistical data analysis
For all the studies, the data were calculated and presented as mean ± SD. Statistical analyses of the data were performed using ANOVA and the Student’s t test. The level of significance was taken as p < 0.05.
Results and discussion
The influence of organic amines
In this study, we investigated the effects of six different organic amines (DEA, TEA, EtA, DEtA, TEtA and HEPP) on the permeation of LOXO through rabbit skin from IPM. The LOXO acid obtained was a white solid, while the organic amine salts of LOXO were all in a sticky liquid state. The permeation profiles and relevant parameters of interest were presented in and , respectively. It was worth noting that the permeation rate of LOXO after forming ion-pair is much greater than that of LOXONa. The order, in terms of Js, was TEA > DEA > HEPP > TEtA > EtA > DEtA. Results showed that only TEA and DEA showed a positive effect on the skin permeation of LOXO (p < 0.05), with an enhancement ratio of 2.17 and 1.17, respectively. While HEPP had no effect, as well as other amines with –OH group even exerted inhibiting effects.
Figure 2. Effect of various organic amines on in vitro permeation of LOXO across the rabbit skin from IPM (n = 4).
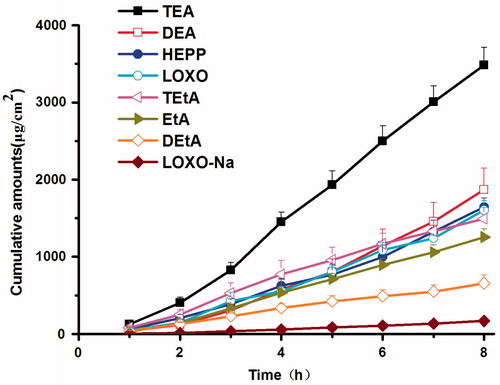
Table 1. Permeation parameters of LOXO with and without organic amines in IPM through rabbit skin and corresponding physicochemical properties (n = 3 or 4).
The results indicated that there was a complex mechanism for the effect of ion-pairs which was related to the physicochemical properties of both the parent drug and the counter-ions. As ER values increased with the increasing log Ko/w of LOXO with organic amines (), while the log Ko/w of LOXO with organic amines changed as a function of the log Ko/w of organic amines (), except for HEPP, which indicated a criteria for the selection of organic amines used to enhance skin permeability. It was worth noting that all the organic amines with –OH group could drive the log Ko/w of ion-pair complex below zero, which may be due to the intrinsic hydrophilicity of –OH group, but had no direct correlation with the number of –OH group.
Figure 4. Relationship between the log Ko/w of LOXO with organic amines and log Ko/w of organic amines.
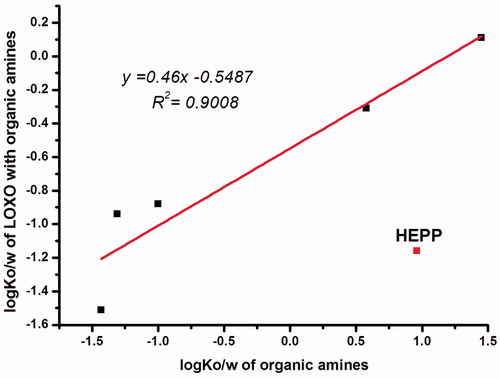
This results can be explained by drug partition between the stratum corneum (SC), epidermis and dermis (ED). Skin model can be regarded as composed of a lipophilic stratum corneum (SC) and an aqueous medium epidermis (ED). A drug must have a suitable log Ko/w, so that it could be firstly assigned to the stratum SC from the IPM, and then distributed and diffused from SC to ED. Once the drug reaches the derma layer, it enters blood circulation. The LOXO–TEA, which has a lower log Ko/w than LOXO (log Ko/w = 1.13), is more suitable to distribute from SC to ED. For LOXO–DEA, the log Ko/w is −0.31, it is too hydrophilic to distribute from the IPM to the SC.
As for HEPP, although lead to a lower log Ko/w of LOXO, permeability showed no difference with LOXO, suggesting that the influence of HEPP on LOXO was instability, that is the complex of HEPP and LOXO dissociated in IPM when skin permeation test was conducted. From the point of chemical structure of molecules, this was due to the lower electron cloud density of N atom in HEPP, as the nitrogen lone pair electrons was delocalized by the five carbon ring, and hydroxyethyl group would also provide an electron-withdrawing inductive effect. On the other hand, TEA had three electron-donating groups, which provided a greater electron cloud density for N atom compared with other organic amines. Thereby, the lone pair electrons were more easily to be given, indicating a firmly interaction between TEA and LOXO, which was just in accordance with our results.
Evidence of ion-pair formation
FTIR has already been utilized in many researches to confirm the formation of ion-pairs (Habeeb, Citation1997; Arunan et al., Citation2011; Xi et al., Citation2012). As shown in , LOXO showed two strong narrow peak around 1708 and 1733 cm−1. Both of them were stretching vibration of C=O group, but the former was due to the carboxyl group (Barthel & Deser, Citation1994), for carboxylic acids, dimeric carboxyl groups were commonly encountered, and C=O band showed an absorption around 1710 cm−1, while for carbonyl group caused by reducing the ring size of cyclic ketones to four or five raised the absorption position to 1750 cm−1.
However, for the samples with organic amines, the peak around 1708 cm−1 disappeared while the peak around 1733 cm−1 still existed. It indicated that organic amines had an electron attracting effect on the carbonyl group of –COOH group. And the O–H band of the carboxyl group often gives rise to a very broad absorption over the range 2500–3300 cm−1. As reported by Hatanaka et al. (Citation2000), if –OH group was interfered by an electron attracting group, the stretching vibration of –OH group would become slow. From , except HEPP, the stretching vibration of –OH group all changed after adding organic amines. From the above observation, a classical hydrogen bonded complex, a weak interaction, may have formed (Barrow, Citation1956; Hudson et al., Citation1972).
Formulation design
First of all, the percutaneous absorption of LOXO–amine complexes were investigated in PSA, a more complex environment than IPM, and the results were summarized in and . In PSA, LOXO–TEA complex still showed the best percutaneous performance, while LOXO became the worst one (p < 0.05). For patches, there were two steps for drug molecules to enter the systemic circulation: releasing from the PSA and penetrating across the skin. If the drug molecules strongly interacted with the functional groups of PSA, the releasing process may be suppressed. In this study, both LOXO and the PSA had carboxyl groups that can form intermolecular hydrogen bonds between these two molecules. For LOXONa, the hydrogen atom of carboxyl group was replaced by sodium atom, the intermolecular interaction became weak and thereby the percutaneous absorption increased.
Figure 6. Effect of various organic amines on in vitro permeation of LOXO across the rabbit skin from PSA (n = 4).
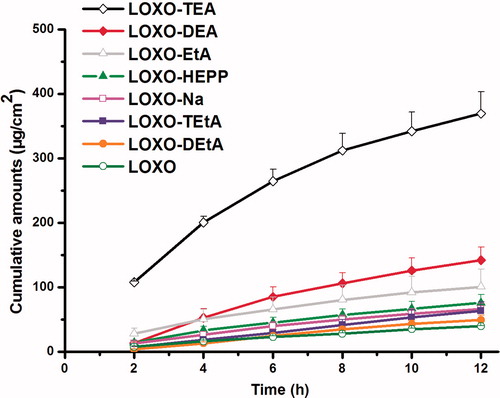
Table 2. Permeation parameters of LOXO with and without organic amines in PSA through rabbit skin (n = 4).
For further increasing the cumulative amounts, penetration enhancers were introduced to the system. As shown in and , the more lipophilic of the enhancer, such as OA and IPM, the better permeation amounts would be obtained. This may be attributed to the enhancement of lipophilicity of PSA environment, which was favorable to the release of relatively hydrophilic drug, LOXO–TEA. Subsequently, the influence of IPM concentration was further studied, and LOXONA® plaster was used as a positive control. The results were shown in and . The consequence indicated that the formulation containing 5% IPM could show an excellent permeation performance (p < 0.05), and the ER value was two times greater than that of the LOXONA® plaster. For 10% IPM contained patch, cumulative amounts were not as twice as that of 5% IPM, which may be due to the lipophilicity of PSA reached a limit when 5% IPM added.
Figure 7. Effect of LOXO–TEA combination with penetration enhancers on the permeation through rabbit skin from PSA (n = 4).
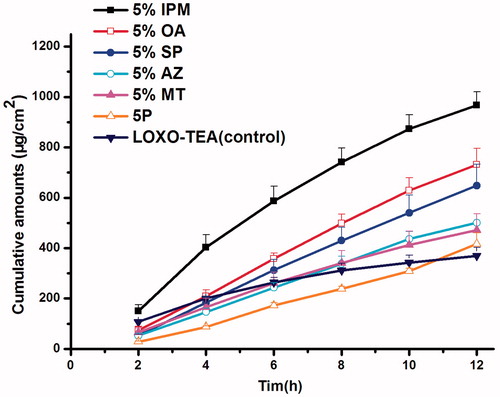
Figure 8. Effects of different concentration of IPM on percutaneous absorption of LOXO–TEA patch compared with the LOXONA® plaster (n = 4).
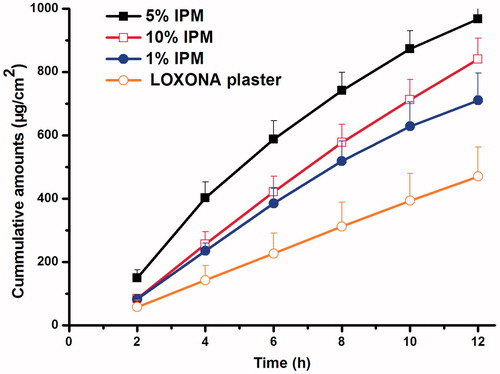
Table 3. The permeation parameters of LOXO–TEA with various enhancers (n = 3 or 4).
Table 4. The permeation parameters of LOXO–TEA with different concentration of IPM, compared with the listed LOXONa® plaster (n = 3 or 4).
Pharmacodynamics study
Assessment of anti-inflammatory activity
The anti-inflammatory activity was evaluated in the CFA-induced adjuvant arthritis (AA) rat model, and the results were illustrated in . According to , both the optimized patch and the LOXONA® plaster showed significant anti-inflammatory effects after applied for 8 days, with no significant difference detected between these two formulations (p < 0.05), suggested that the patch we designed was successful. And both the left and right hind paws of rats showed similar swelling degree at the same time point, which indicated that the patches exerted a systemic effect although they were applied topically.
Figure 9. The profiles of swelling degree (%) of different groups in the experiment of adjuvant induced arthritis in rats (A) left side; (B) right side.
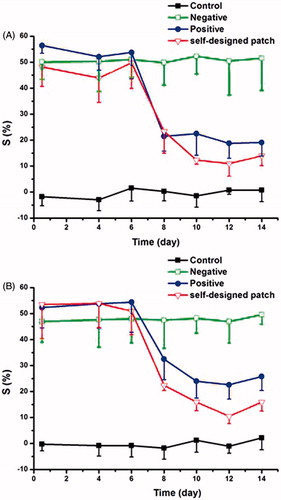
These findings may be as a result of the particularity of LOXO, which was a prodrug and needed to be converted to its active metabolite for the treatment. It was reported that liver was the major metabolism site for most of LOXO, and the extrahepatic metabolism occurred in blood and dermal layer of the skin (Koo et al., Citation2005; Murakami, Citation2008). In previous study, most of LOXO molecules entered the systemic circulation after topical application and were converted to their active forms in the liver, and then the active forms were distributed into the joint synovium, the major action site of NSAIDs (Firestein, Citation2003). And only a small amount of LOXO could metabolize in the local site and access to the synovium (Jin et al., Citation2001).
There have been a lot of studies about how NSAID was delivered into the joint synovial fluid after topical administration (Bannwarth et al., Citation1991; Richard et al., Citation1995; Lapicque et al., Citation1996; Richard et al., Citation1999). Radermacher et al. (Citation1991) indicated that any direct transport of across the skin about diclofenac accounts for only a small fraction of drug reaching the joint, and at least 85% of the dose must penetrate into the systemic circulation. A similar result was received with ibuprofen (Dominkus et al., Citation1996). Recently, our group also confirmed that the transdermal patches applied topically exerted a RA therapeutic activity by drug delivery via systemic circulation (Xi et al., Citation2013).
However, there was still an interesting problem in this study, that was the cumulative amounts of the optimized patch for 12 h were twice as the commercial one, but their anti-inflammatory activities were almost the same. This may be due to the saturation phenomena of the metabolic enzymes, which can be further demonstrated by the next study.
Acetic acid-induced writhing syndrome
The analgesic effect of the optimized patch and the LOXONA® plaster was evaluated in the acetic acid-induced writhing syndrome model, and the results were presented in . When given a patch of 6 cm2, the pain inhibition ratios of the optimized patch and the LOXONA® plaster were 71.35 and 72.84%, respectively, and no significant difference was detected between these two formulations (p < 0.05). This result was the same as that of the anti-inflammatory activity assessment. However, the pain inhibition ratios were significantly different between these two formulations, when the dosage was reduced to 3 cm2. The inhibition ratios were 59.91 and 39.99% for the optimized patch and the LOXONA® plaster, separately, which indicated that the analgesic effect of LOXO was dose-dependent. The saturation of the metabolic enzymes was thought to be responsible for the similar analgesic effect of the two formulations when topically applied at a dose of 6 cm2.
Table 5. The result of analgesic effect of LOXO patch on the pain induced by acetic acid in mice (mean ± SD, n = 10).
From above results, we could design our patch in half size, that is to say, the cumulative amounts and the anti-inflammatory activities were adjusted the same as that of the commercial one, while the bioavailability was enhanced.
Conclusions
The results of this article showed that some of the organic amines, especially the TEA, can significantly increase the skin penetration of LOXO in IPM. The mechanism of ion-pairs was due to the modification of the octanol-water partition coefficient of the parent drug. Finally, a LOXO patch with a higher percutaneous absorption was prepared using the combination of ion-pair technology and chemical enhancers. The in vitro cumulative amounts of the new designed LOXO patch were about twice as that of the LOXONA® plaster and presented a comparable pharmacodynamic effects with the commercial one.
Declaration of interest
The authors declare that they have no conflicts of interest to disclose.
References
- Arunan E, Desiraju GR, Klein RA, et al. (2011). Defining the hydrogen bond: an account (IUPAC Technical Report). Pure Appl Chem 83:1619–36
- Bannwarth B, Netter P, Lapicque F. (1991). Tenoxicam concentrations in synovium and joint cartilage in humans. Agents Actions Suppl 32:295–8
- Barrow GM. (1956). The nature of hydrogen bonded ion-pairs: the reaction of pyridine and carboxylic acids in chloroform. J Am Chem Soc 78:5802–6
- Barry BW. (2001). Novel mechanisms and devices to enable successful transdermal drug delivery. Eur J Pharm Sci 14:101–14
- Barthel J, Deser R. (1994). FTIR study of ion solvation and ion-pair formation in alkaline and alkaline earth metal salt solutions in acetonitrile. J Sol Chem 23:1133–46
- Cheong HA, Choi HK. (2003). Effect of ethanolamine salts and enhancers on the percutaneous absorption of piroxicam from a pressure sensitive adhesive matrix. Eur J Pharm Sci 18:149–53
- Chinese Pharmacopoeia. (2005). National Pharmacopoeia Committee. Pharmacopoeia of People's Republic of China [M]. Part 2. Beijing: Chemical Industry Press (Appendix 177)
- Dominkus M, Nicolakis M, Kotz R. (1996). Comparison of tissue and plasma levels of ibuprofen after oral and topical administration. Arzneimittel Forsch 46:1138–43
- Fang L, Numajiri S, Kobayashi D, Morimoto Y. (2003). The use of complexation with alkanolamines to facilitate skin permeation of mefenamic acid. Int J Pharm 262:13–22
- Firestein GS. (2003). Evolving concepts of rheumatoid arthritis. Nature 423:356–61
- Habeeb MM. (1997). Spectroscopic studies of proton transfer equilibria in hydrogen bonded complexes. Appl Spectrosc Rev 32:103–40
- Hatanaka T, Kamon T, Morigaki S, et al. (2000). Ion pair skin transport of a zwitterionic drug, cephalexin. J Control Release 66:63–71
- Hudson RA, Scott RM, Vinogradov SN. (1972). Hydrogen-bonded complex-ion-pair equilibriums in 3,4-dinitrophenol-amine-aprotic solvent systems. J Phys Chem 76:1989–93
- Ichikawa T, Sato H, Kaira K, et al. (2008). Prolonged intrahepatic cholestasis after exposure to loxoprofen. Clin Therap 30:2402–6
- Jiang J, Quan P, Chen Y, Fang L. (2014). Mechanistic investigation and reversible effect of 2-isopropyl-5-methylcyclohexyl heptanoate on the in vitro percutaneous absorption of indomethacin. Drug Deliv 21:26–33
- Jin EH, Da WL, Eun BL. (2001). Anti-inflammatory, analgesic and antipyretic activities of loxoprofen sodium given intramuscularly in animals. Arch Pharm Res 24:541–5
- Koo TS, Kim DH, Ahn SH, et al. (2005). Comparison of pharmacokinetics of loxoprofen and its active metabolites after an intravenous, intramuscular, and oral administration of loxoprofen in rats: evidence for extrahepatic metabolism. J Pharm Sci 94:2187–97
- Kotiyan P, Vavia P. (2002). Synthesis and characterization of an acrylate pressure adhesive for transdermal drug delivery. Polym Adv Technol 13:137–43
- Lapicque F, Mainard D, Gillet P. (1996). Stereoselective distribution of tiaprofenic acid in synovium and cartilage in osteoar-thritic patients. Eur J Clin Pharmacol 50:283–7
- Marwah H, Garg T, Goyal AK, Rath G. (2014). Permeation enhancer strategies in transdermal drug delivery. Drug Deliv 1–15. 2014 Jul 9. [Epub ahead of print]
- Murakami T, Kawasaki T, Takemura A, et al. (2008). Identification of degradation products in loxoprofen sodium adhesive tapes by liquid chromatography-mass spectrometry and dynamic pressurized liquid extraction-solid-phase extraction coupled to liquid chromatography-nuclear magnetic resonance spectroscopy. J Chromatogr A 1208:164–74
- Ma X, Fang L, Guo JP, et al. (2009). Effect of counter-ions and penetration enhancers on the skin permeation of flurbiprofen. J Pharm Sci 99:1826–37
- Nakase H, Itani T, Mimura J, et al. (1999). Colonic ulceration caused by administration of loxoprofen sodium. Intern Med 38:249–51
- Pawar V, Kumar HY, Giridhar R, Yadav MR. (2014). Enhanced skin permeation of 6-methoxy-2-naphthylacetic acid by salt formation. Drug Deliv 2014 Mar 27. [Epub ahead of print]
- Radermacher J, Jentsch D, Scholl MA. (1991). Diclofenac concentrations in synovial fluid and plasma after cutaneous application in inflammatory and degenerative joint disease. Br J Clin Pharmacol 31:537–41
- Richard OD, Francis H, Vial J. (1995). Naproxen concentrations in plasma and synovial fluid and effects on prostanoid concentrations. J Rheumatol 22:2295–303
- Richard OD, Andrew JM, Garry GG, Kenneth MW. (1999). Pharmacokinetics of nonsteroidal anti-inflammatory drugs in synovial fluid. Clin Pharmacokinet 36:191–201
- Tan HS, Pfister WR. (1999). Pressure-sensitive adhesives for transdermal drug delivery systems. Pharm Sci Technol Today 2:60–9
- Xi HL, Cun DM, Wang ZY, Shang L. (2012). Effect of the stability of hydrogen-bonded ion pairs with organic amines on transdermal penetration of teriflunomide. Int J Pharm 436:857–61
- Xi HL, Cun DM, Xiang RW, et al. (2013). Intra-articular drug delivery from an optimized topical patch containing teriflunomide and lornoxicam for rheumatoid arthritis treatment: does the topical patch really enhance a local treatment? J Control Release 169:73–81