Abstract
The aim of this research was to formulate a liposomal preparation of DOX to be applied topically, and to investigate the in vitro and in vivo performance of the prepared liposomes. DOX liposomes were prepared by the solvent evaporation method, and then modified with bioadhesive material HA. Through MTT assay, we found that the safe concentration of liposomes delivered would hit 1 mg/mL. Cellular uptake studies showed that DOX liposomes coated with HA are much more targetable to cell nucleus. Their ocular pharmacokinetics in rabbits were investigated through the comparison with those obtained after dosing with non-modified liposomes and DOX solution. The in vitro transcorneal permeability of DOX in both kinds of liposomes was found to be slower than that of the solution because of sustained release. After in vivo instillation in rabbits, HA-modified liposomes had the longest retention time, following with naked liposomes. Significantly, the area under the curve of the aqueous humor concentration–time profiles of DOX liposomes was found to be 1.7-fold higher than that of DOX solution. The confocal experiment confirmed that HA-modified liposomes were able to maintain a higher DOX concentration and residence time than that of non-modified liposomes and free DOX. These results suggest that our liposomal preparation was of great help to improve the bioavailability of DOX.
Introduction
Doxorubicin (DOX) is mostly known as an anthracycline agent for the treatment of variety of malignancies (Fetterly et al., Citation2013; Lin et al., Citation2014). DOX can also be applied on proliferative vitreoretinopathy (PVR) (Sunalpa et al., Citation1985), which manifests itself by the formation of fibrocellular membranes on the retinal and posterior hyaloid surfaces (Hiscott & Wong, Citation2010). Intravitreal injection of DOX was an effective way to heal experimental PVR (Sunalp et al., Citation1984; Ebrahim et al., Citation2005), whereas it is invasive and may cause even endophthalmitis (Urtti, Citation2006). With the advantages of convenience and safety, eye drops are commonly considered to be an improvement to deal with PVR. (Peyman & Ganiban, Citation1995). After instillation, the flow of lacrimal fluid removes drugs from the surface of the eye. Another source of non-productive drug removal is its systemic absorption instead of ocular absorption. These lead to a low bioavailability of less than 5% (Baba et al., Citation2011).
Various ophthalmic drug delivery strategies have emerged to solve problems mentioned above. Methods such as hydrogels, polymeric micelles, and nanocarriers were widely used in this area (Li et al., Citation2008; Shen et al., Citation2009; Gupta et al., Citation2010; Li et al., Citation2012; Liu et al., Citation2012; Gan et al., Citation2013). Liposomes, a kind of closed vesicles composed of a phospholipid bilayer and an aqueous phase (Honda et al., Citation2013), have been serving as a promising ocular drug delivery method for the past decade (Li et al., Citation2009). Studies have demonstrated that by applying this method, a controlled drug release as well as membrane permeability can be achieved. Therefore, the half-life of the drug inside the vitreous body can be prolonged, and the toxicity of the drug can be reduced (Llopis et al., Citation1992; Cheng et al., Citation2000; Kaiser et al., Citation2013). We feel obliged to promote the bioavailability of drugs in the treatment on posterior segment of the eyes. Therefore, it will be necessary to modify the liposomes to achieve better performance.
Hyaluronic acid (HA), one of the main components of the extracellular matrix in the body, has characters including biocompatible, biodegradable (Choi et al., Citation2008), and non-toxic (Jiang et al., Citation2012). It is also a nonirritating substance with high water-binding capacity, viscous flow, and pseudoplastic behavior, and has the potential to act as a mucoadhesive polymer that can increase residence time on ocular surface (Yenice et al., Citation2008). To our knowledge, there are few published reports on the use of HA in ophthalmology as a coating material of liposomes. The highlight of our study is to apply the HA-modified liposomes into the treatment of ophthalmology. Drug therapy for some eye diseases such as PVR may be easier to operate. HA-modified liposomes can be delivered with fewer intervals and even at lower doses since vehicles coated with HA provide longer retention time on the surface of the eyeball, thus improving the bioavailability of ophthalmic medications.
The objective of this study was to evaluate whether the ocular bioavailability of DOX could be improved by delivering it in liposomes modified with HA. Our study provides a new pharmaceutical preparation method to the preclinical development of treatments on posterior segment of the eyes.
Materials and methods
Materials
DOX was purchased from Beijing HuaFeng United Technology CO., Ltd, Beijing, China. Phospholipids were obtained from Shanghai Advanced Vehicle Technology Pharmaceutical Ltd, Shanghai, China. Cholesterol is purchased from Sinopharm Chemical Reagent Co., Ltd, Mainland, China. Octadecylamine is purchased from Sigma Chemical Corporation (St. Louis, MO). Dialysis membrane (MW cut-off 14 kDa) was purchased from Shanghai Green Bird Science & Technology Development Co., Ltd. Embedding Medium for Frozen Tissue Specimens (O.C.T. compound) is purchased from Sakura Finetek USA Inc., Torrance, CA. All other chemicals used in the study were of analytical reagent grade.
Animals
New Zealand albino rabbits of either sex, weighing 2.5–3 kg, were obtained from and cared for in accordance with the Guide for the Care and Use of Xiamen University Laboratory Animal Center. The study was approved by the Institutional Animal Use and Care Committee of Xiamen University, China.
Preparation of liposomes
DOX liposomes were prepared by the solvent evaporation method (Wu et al., Citation2004). In brief, 70 mg of PC/CH/Stearyl amine (SA) mixture (15:3:2, wt/wt), dissolved in 4 mL of chloroform/methanol (1:3, vol/vol) and sonicated for 1 min using the Ultrasonic cell crusher (Scientz Biotechnology Polytron Technologies Inc, Ningbo, China) to form the supernatant liquid. The organic solvent was slowly removed at reduced pressure, 40 °C water bath on a rotary evaporator to form a thin film of the phospholipid mixture on the inner wall of the flask. About 2 mL of ammonium sulfate solution (0.2% wt/vol) were added and vortexed to produce multi-lamellar vesicles (MLVs). The liposomal dispersions were sonicated and filtrated through a 0.22-μm microporous filter (Purifying Equipment Manufacturing, Shanghai, China) to obtain more homogeneously sized vesicles. Followed by incubation with DOX solutions, the dialysis method was performed to exclude free DOX. Finally, HA was added to the liposome by electrostatic interaction with sterylamine to form bioadhesive preparation.
Determination of DOX encapsulation efficiency
DOX encapsulation efficiency (EE%) was determined by size exclusion chromatography (SEC). In brief, 100 μL of DOX liposome preparation were separated by Sephadex G-50, and PBS was used as the elution solution. Separation was monitored by determining the fluorimetric absorbance of the solution at 488/530 nm using the Multimode Plate Reader (Envision, Miami, FL). The encapsulated DOX was determined after emulsion breaking of liposomes with Triton X-100 (final concentration 1% vol/vol). The DOX EE % was calculated as follow:
Particle size and zeta potential
The particle size and the zeta potential of liposome were determined using photon correlation spectroscopy (Zetasizer, HAS 3000; Malvern Instruments, Malvern, UK). The size distribution analysis was performed at a scattering angle of 90° and at a temperature of 25 °C using samples appropriately diluted with pure water (AJC-0501-P; Aquapro, Landrum, SC), whereas the zeta potential was measured using a disposable zeta cuvette. For each sample, the mean diameter/zeta potential ± standard deviation of three determinations was calculated applying multimodal analysis.
Morphology of NPs
The morphology of the DOX-loaded liposomes was investigated by transmission electron microscopy (TEM; JEM-2100, JEOL Ltd, Tokyo, Japan). The samples were placed on carbon-coated copper grids for viewing by TEM.
In vitro release from liposomes
The release of doxorubicin from the liposomal formulation was determined using the dialysis method. Briefly, 0.5 mL of the HA-modified liposomal suspension, 0.5 mL of the non-modified liposomal suspension, and 0.5 mL of free DOX solution were placed into a dialysis bag (molecular weight cut-off 8000–14 000 Da). The bag was placed in 200 mL of PBS (pH 7.4) maintained at 37 °C and stirred with a magnetic stirrer. At specific time intervals, samples were withdrawn for the determination of drug concentrations by the fluorescence method, and replaced with 200 mL of fresh PBS to maintain a constant volume.
Cell culture studies
Human corneal epithelial cells (HCE cells) were cultured in 75 cm2 culture flasks containing the DMEM F12 medium (Thermo Scientific Hyclone®, Beijing, China) supplemented with 10% fetal bovine serum (Hyclone, Logan, UT), 5 μg/mL insulin (Sigma, St. Louis, MO), and 10 ng/mL epidermal growth factor (Gibco®, Grand Island, NY) (Sasaki et al., Citation2013) at 37 °C in a humidified incubator (Thermo, Waltham, MA) containing 5% CO2 air. Confluent cell monolayers were trypsinized and cells in the exponentially growing phase were used in the cytotoxicity experiments.
Analysis of in vitro cytotoxicity
The cell viability was measured by the MTT assay (Boncler et al., Citation2014). HCE cells were seeded on 96-well plate at the initial density of 1 × 104 cells per well. After a 24 h stabilization of the cells, fresh medium containing blank or loaded liposomes (0. 5, 1, 2, 4, 6.25, 12.5, 25, 50, 100, 200, 400, and 800 PPM) was added. And these cells were grown in a humidified atmosphere of 5% CO2 in air at 37 °C, for 24 h in order to compare the cytotoxicity of different concentrations on HCE cells.
After the incubation, the medium was removed and 100 μL fresh medium and 13 μL MTT solution (5 μg/mL diluted with DMED F12) were added to each well. Incubation was allowed for another 4 h in darkness at 37 °C. Since living cells metabolize the MTT and form blue formazan crystals, 100 μL/well dimethyl sulfoxide was added to dissolve the formazan crystals. Absorbance values were measured by reading the plates at 570 nm on an enzymes labeling instrument (Perkin-Elmer, Victor 3 V, Waltham, MA), and the percentage of viability was calculated. The viability of the treated cell cultures was expressed as a percentage of control-untreated cell cultures assumed to be 100%.
Cellular uptake studies
HCE cells (2.5 × 10−3) were seeded in 6-well plates, incubated overnight at 37 °C with 5% CO2 in a humidified atmosphere. Free DOX solution and liposomes were diluted separately with cell culture medium in order to obtain a concentration of 40 PPM and were incubated at 37 °C for 0.5, 3, and 6 h. The experiment was stopped by washing the cells three times with sterile PBS to eliminate excess DOX which were not entrapped by the cells. Following that is the nuclear staining of DAPI. And then the observation of the cells was performed using a laser scanning confocal microscope.
In vitro transcorneal experiments
The corneas used were excised from rabbit eyes, which were collected immediately after the animals were sacrificed. Cornea was tied to one side of the open tube (donor compartment) ensuring that its epithelial surface faced the donor compartment. The donor compartment was filled with 0.5 mL of drug solution with a concentration of 0.4 mg/mL DOX while the receiving compartment was filled with 5 mL of glutathione bicarbonate Ringer’ s solution (GBR), which has the same composition with that in the study done by Yan Shen et al. (Shen & Tu, Citation2007). Both are performed in a water bath of 34 °C with a magnetic stirring at 400 rpm/min. About 0.4 mL of aliquot solution was withdrawn from the receiving solution and replaced hourly with 0.4 mL of fresh PBS for 10 h. The collected samples were quantified by the fluorescence method.
In vivo precorneal retention experiment
Forty-eight unanesthetized rabbits, free of gross ocular defects, were positioned into restraining boxes. Rabbits were then randomly assigned to eight groups equally. About 50 μL liposome preparation (0.8 mg/mL) or DOX-PBS solution (0.8 mg/mL) was instilled directly onto the corneal surface and the eye manually blinked to distribute the solution over the cornea. Tear fluid samples of the eyes were collected by Schirmer test strips at time intervals of 5, 10, 20, 30, 60, 120, 180, and 240 (Tianjin Jingming New-Tech Co, Tianjin, China). The amount of tear collected was calculated by taking the difference in weight of each strip before and after the sampling. The Schirmer strip was dried by an N2 stream, and then PBS was added. The sample was vortexed thoroughly to dissolve DOX into the solution and centrifuged before determination by the fluorescence method.
In vivo pharmacokinetics study
Forty-two rabbits were divided into seven groups, which was instilled 50 μL DOX liposome preparation (0.8 mg/mL) or 50 μL DOX-PBS solution (0.8 mg/mL). At time intervals of 5, 30, 45, 60, 120, 180, and 240 min after drug delivery, one rabbit was sacrificed. Samples of aqueous humor were extracted from the anterior chamber using a needle syringe.
Transcorneal visualization studies of the colloidal carriers
Corneas were harvested and snap-frozen in liquid nitrogen. The tissues are sliced up to 6 μm in depth by freezing microtome (Leica CM1900, Nuβloch, Germany). The cryosections are visualized by confocal microscopy (Zeiss, German) after DAPI nuclear staining.
Statistical data analysis
Pharmacokinetic parameters were calculated by the WinNonlin professional software (Pharsight Corporation, Mountain View, CA). All data present in this study were expressed as mean ± SD.
Results
Characterization of liposomes
Particle size is a crucial factor for ophthalmic application via eye drop administration (Fujisawa et al., Citation2012). It should not exceed 10 μm (Das et al., Citation2010). The effect of phospholipid/cholestenone ratio has an immense effect on particle size, distribution, and entrapment efficiency. All batches showed a small mean size, well suited for possible ocular application. The mean particle size for these liposome formulations varied from 134 to 517 nm. All their particle sizes are qualified. Entrapment efficiency was increased from 8.15% to 86.5% as phospholipid/cholestenone ratio changes from 3:1 to 6:1. Hence, the method was optimized to produce liposomes of small size and narrow size distribution with high entrapment efficiency, we choose S3 to continue with the rest of our experiments. The results are shown in .
Table 1. Effect of the phospholipid/cholestenone ratio on physicochemical properties of DOX-loaded liposomes.
As shows, the zeta potential of the blank liposomes is neutral. It is shifted to positive side with the addition of SA, and then turned to negative side when modified with HA, indicating that HA has already adhered to the liposomes.
Morphology
Transmission and scanning electron micrographs of the liposomes showed that the particles are multi-layer liposomes which may carry more drugs to posterior segment of the eyes ().
In vitro release studies
Biphasic release pattern characterized by an initial burst release of DOX was observed. After the initial burst effect, the drug was released slowly. shows that HA-modified liposomes (HA-LP) were able to sustain the DOX release as well as non-modified liposomes (LP) while the free DOX almost release 90% in 24 h. This may be attributed to the hydrophobic and the hydrophilic nature of HA, which increased the hydrophilic chain of liposomes hence increased longer retention time.
Cytotoxicity of liposomes
An ideal drug delivery system intended for ophthalmic use must be capable of releasing sufficient levels of active agent without weakening the viability of the host cells. Cationic polymers are known to exhibit cytotoxic effects by inducing cell membrane damage (Aksungur et al., Citation2011).
The survival rate of HCE cells decreased as a result of increasing the liposomes concentration applied (). No significant difference in survival rate for the cells treated with the concentrations range from 0.5 PPM to 12.5 PPM liposomes was observed. Through experiments, we found that there was about 4–5 mL aqueous humor in rabbits’ anterior chamber. Supposing the bioavailability of the liposomal preparation could reach 100%, the safe concentration of liposomes delivered would hit 1 mg/mL by calculation.
Cellular uptake studies
All the photographs were taken under consistent condition with detection parameters unchanged. The nucleus of each cell was labeled blue by DAPI. DOX molecules were highly fluorescent. We could take advantage of this property to monitor drug release through fluorescence signals. An obvious time-dependent increase in uptake was observed from 0.5 to 6 h (). At given time points, both liposome groups showed higher uptake in cell nucleus after 0.5 h. The intranuclear uptake of DOX loaded in liposomes was significantly greater than that of free DOX at 3 h. At this time point, HA-LP displayed stronger fluorescence intensity in the nucleus than that of LP. However, DOX was still distributed evenly in the whole cell. When it goes to 6 h, DOX almost concentrated in the nucleus of both liposome groups, while the proportion of DOX that entered the nucleus of the free DOX group was around 50% as before. Cellular uptake studies showed that DOX liposomes coated by HA is much more targetable to cell nucleus.
Figure 5. CLSM of DOX uptakes by human corneal epithelia cell after incubated alternately with 40 PPM (A1–A3) free DOX group, (B1–B3) non-modified liposome group, and (C1–C3) HA-modified liposome group after (A1, B1, and C1) 0.5 h, (A2, B2, and C2) 3 h, and (A3, B3, and C3) 6 h. (Up) blue filter; (middle) red filter; (down) overlay of both (up and middle) sections.
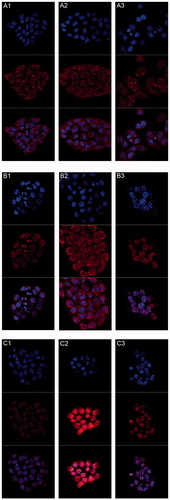
In vitro corneal penetration
illustrates the in vitro corneal penetration experimental results of the DOX-loaded liposomes and DOX PBS solution. It is clearly observed that about 2.2 μg DOX penetrated in 10 h for free DOX. The DOX-loading liposomes presented a lower corneal penetration – 0.8 μg and 1.3 μg penetrated in 10 h. In this case, the liposomes prolonged the penetration of DOX across the cornea, which corresponded to the result reported by the SL Law (Law et al., Citation2000).
In vivo precorneal retention evaluation
As shown in , following instillation of DOX PBS solution and non-modified liposomes, the concentrations of the drug can last for only 0.5 h around. However, HA-modified liposomes provided a significant sustained drug release in the rabbit eyes.
Figure 7. Kinetics of DOX disappearance from tear fluid following topical administration of the liposome and the control solution (X ± SD, n = 4).
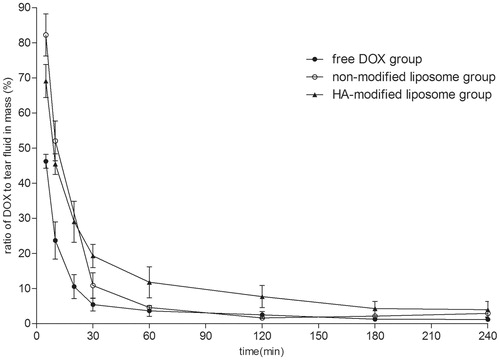
Ocular drug absorption from the lacrimal fluid to the anterior ocular tissues via trans-corneal absorption is determined by two major factors: drug permeability in the cornea and ocular contact time of the delivery system (Mannermaa et al., Citation2006). Precorneal retention can be used to evaluate the bioadhesion of ophthalmic formulation, so that it may provide useful information for the prediction of ocular bioavailability (Cao et al., Citation2011).
In vivo pharmacokinetics study
indicates the in vivo corneal absorption of DOX concentrations in aqueous humor after topical instillation of DOX PBS solution and DOX-loading liposomes. The pharmacokinetic parameters of DOX in the aqueous humor after instillation of both groups are summarized in . It is evident that the maximum concentration (Cmax) of DOX in the rabbit aqueous humor after instillation of DOX-loaded liposomes was 1.4-fold higher than that of administration of free DOX, also suggesting that liposome preparations could greatly enhance the absorption of DOX after instillation. Meanwhile, the greater DOX bioavailability was produced by the liposome group – approximately a 1.7-fold bioavailability than that of the DOX PBS solution (AUC 61 155 ± 29 366.76 and 36 462.6 ± 14 925.04 ngmL−1 min−1). Moreover, a later Tmax of the liposome group than that of the free DOX group was observed (Tmax 48.75 ± 12.43 min and 33.75 ± 6.49 min for the liposome group and the free DOX group, respectively), this result confirmed the former in vitro corneal penetration experiment. Liposome preparation had a smaller Cl/F and a longer MRT than that of free DOX, indicating that HA-modified liposomes could greatly increase the residence time by adhesion effect, resulting a better bioavailability.
Figure 8. Concentration–time profles of DOX in aqueous humor after instillation of 0.5 mg/mL DOX liposome preparation and DOX solution in rabbits (ng/mL, X ± SD, n = 4).
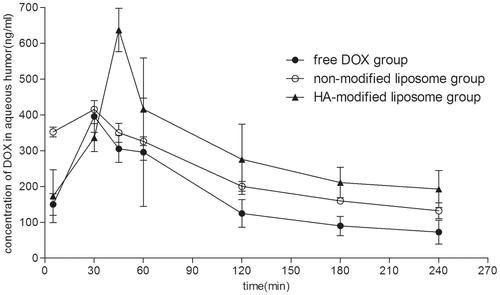
Table 2. Pharmacokinetic parameters of DOX in the aqueous humor of rabbits (n = 4).
Confocal experiment of corneal tissues
As revealed by fluorescence micrographs (), intense fluorescence signals were observed inside the tissues to express the procedure of DOX-loading liposomes and DOX PBS solution getting through the cornea. All the images were taken under the same condition with detection parameters unchanged. Blue color in the images is the consequence of DAPI staining and the red color is from DOX itself. At 0.5 h after topical instillation, a small amount of DOX has entered the cornea, while the light intensity of DOX for liposome preparations in the cornea increased, indicating that DOX-loading liposomes have a better absorption than that of free DOX. As to 2 h, DOX for the two groups both reached the stroma layer. When it goes to 3 h, we can still see quite a lot of DOX in the cornea which is instilled DOX liposomes, however, there is no longer red color appearing in the other group. The reason is that free DOX is almost metabolized at 3 h. But DOX encapsulated in the liposomes is able to achieve a sustained delivery owing to the adhesive effect HA, which prolong the retention time on the epithelium.
Figure 9. CLSM of DOX absorption by corneal tissues after rabbits’ eyes in vivo treated alternately with free DOX (A1–A3), (B1–B3) non-modified liposome, and (C1–C3) HA-modified liposome after (A1, B1, and C1) 0.5 h, (A2, B2, and C2) 2 h, and (A3, B3, and C3) 3 h. (Up) blue filter; (middle) red filter; (down) overlay of both (up and middle) sections.
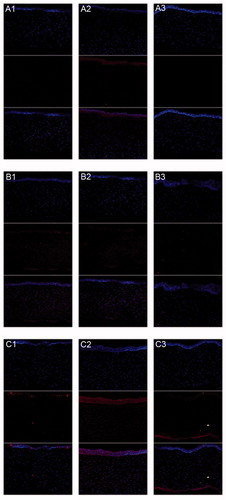
Discussion
In the in vitro study, the release profile was prominently prolonged by the liposome`s encapsulation, and further by HA coating. The drug was completely entrapped in the liposome without any free drug in solution or absorbed on the liposome surface, and therefore, there was no burst release at the beginning. In this case, the drug release rate depends on the membrane permeability which is affected by the fluidity of lipid bilayer (Volodkin et al., Citation2007). At the experimental condition, the lipid bilayer is in liquid–crystalline phase which permits the drug permeation. For the case of HA-modified liposomes, the drug permeation rate was reduced by the presence of HA coating. The mechanism might be explained that HA molecule is closely absorbed to the liposome surface and constructs an intense shell, which probably restricts the bilayer fluidity. Consequently, the membrane was consolidated and the permeability was decreased.
For most drugs in eye drops, passive diffusion along their concentration gradient as well as the transcellular route or paracellular route are the main permeation mechanisms across the cornea (Li et al., Citation2012). It may be one of the factors that the liposomes attained to be a long-term sustained release system owing to the adhesion of the HA encircling the liposomes. The desired consequence was that our clingy preparation successfully prevented the burst permeation of DOX through the cornea and washed out quickly. It indeed helped to reduce topical toxicity caused by high drug concentration and maintain effective concentration for a longer time.
This result suggested that for both non-modified liposomes and DOX solution, DOX clearance was caused mainly by tear drainage and turnover. Because both DOX preparations did not contain any viscous materials, it is possible that both could be eliminated from the precorneal area sufficiently rapidly so that the distribution process would not affect precorneal clearance. However, the use of HA which is an ideal bioadhesive material made it longer for the drugs to stay at the surface of the eyes.
The in vitro transcorneal experiments suggest that more free DOXs entered into the receptor cell as it would not be scoured by tears. However, in the in vivo pharmacokinetics study, the flow of lacrimal fluid removes drugs from the surface of the eye. Another source of non-productive drug removal is its systemic absorption instead of ocular absorption. Therefore, the advantage of the bioadhesion of HA was highlighted.
Conventional study holds that positively charged liposome has the most uptake by the cornea, following with negatively charged liposomes, and the least for neutral, as positively charged liposomes could increase electrostatic interaction between the particles and the negatively charged mucosal surface. HA promotes the ability to adhere the conjunctival tissues and the mucin layer because of its high water-binding capacity, viscous flow, and pseudoplastic behavior which is different from former mechanism. In this study, even though the zeta potential of the liposomes was negative as a result of modification of HA, adhesion helps it to achieve good bioavailability.
Hsi-Kung Kuo showed that DOX-loaded liposomes can attenuate the severity of experimental PVR and reduces the glial cell expression of intermediate filaments in PVR retinas. The liposomes have a wider safe dosage range and a longer half-life in the vitreous than does primary DOX (Kuo et al., Citation2012). In our study, HA-modified liposomes performed better than DOX-loaded liposomes in all the experiments conducted above, indicating that HA-liposome indeed has potential in ocular treatment and deserves further investigation.
Conclusions
In this study, the potential of HA-modified liposomes as a drug vehicle for ophthalmic delivery was investigated. Doxorubicin, as an anti-tumor drug being clinically applied, was successfully encapsulated into liposomes by the active loading method. The preparation provides a consecutive release. Meanwhile, a better absorption to the aqueous humor is attained. In vitro penetration studies across the rabbit cornea demonstrated that the liposome formulations exhibited a sustained release in permeation as compared with that of DOX PBS solution. When DOX-loading liposomes were administered to the rabbit eyes, the retention time was prolonged, Cmax and AUC were approximately 1.4-fold and 1.7-fold greater than those of DOX PBS solution, respectively. Moreover, the confocal experiment confirmed that HA-modified liposomes have the ability to maintain a higher DOX concentration than that of free DOX. These results suggest that the novel ocular drug delivery system was a very promising tool to improve the bioavailability of the drugs.
Acknowledgements
The authors wish to thank Eye Institute of Xiamen University for kindly providing HCE cells.
Declaration of interest
The authors report that they have no conflicts of interest. The authors alone are responsible for the content and writing of the paper.
Reference
- Aksungur P, Demirbilek M, Denkbaş EB, et al. (2011). Development and characterization of cyclosporine A loaded nanoparticles for ocular drug delivery: cellular toxicity, uptake, and kinetic studies. J Control Release 151:286–94
- Baba K, Tanaka Y, Kubota A, et al. (2011). A method for enhancing the ocular penetration of eye drops using nanoparticles of hydrolyzable dye. J Control Release 153:278–87
- Boncler M, Różalski M, Krajewska U, et al. (2014). Comparison of PrestoBlue and MTT assays of cellular viability in the assessment of anti-proliferative effects of plant extracts on human endothelial cells. J Pharmacol Toxicol Methods 69:9–16
- Cao F, Wang Y, Ping Q, Liao Z. (2011). Zn-Al-NO(3)-layered double hydroxides with intercalated diclofenac for ocular delivery. Int J Pharm 404:250–6
- Cheng L, Hostetler KY, Chaidhawangul S, et al. (2000). Intravitreal toxicology and duration of efficacy of a novel antiviral lipid prodrug of ganciclovir in liposome formulation. IOVS 41:1523–32
- Choi KY, Lee S, Park K, et al. (2008). Preparation and characterization of hyaluronic acid-based hydrogel nanoparticles. J Phys Chem Solids 69:1591–5
- Das S, Suresh PK, Desmukh R. (2010). Design of Eudragit RL 100 nanoparticles by nanoprecipitation method for ocular drug delivery. Nanomedicine 6:318–23
- Ebrahim S, Peyman GA, Lee APJ. (2005). Applications of liposomes in ophthalmology. Surv Ophthalmol 50:167–82
- Fetterly GJ, Aras U, Murphy DL, et al. (2013). Development of a preclinical PK/PD model to assess antitumor response of a sequential aflibercept and doxorubicin-dosing strategy in acute myeloid leukemia. AAPS J 15:662–73
- Fujisawa T, Miyai H, Hironaka K, et al. (2012). Liposomal diclofenac eye drop formulations targeting the retina: formulation stability improvement using surface modification of liposomes. Int J Pharm 436:564–7
- Gan L, Wang J, Jiang M, et al. (2013). Recent advances in topical ophthalmic drug delivery with lipid-based nanocarriers. Drug Discov Today 18:290–7
- Gupta H, Aqil M, Khar RK, et al. (2010). Sparfloxacin-loaded PLGA nanoparticles for sustained ocular drug delivery. Nanomed-Nanotechnol 6:324–33
- Hiscott P, Wong D. (2010). Proliferative vitreoretinopathy. In: Dartt DA, Besharse JC, Dana R, eds. Encyclopedia of the eye. USA: Academic Press, 526–34
- Honda M, Asai T, Oku N, et al. (2013). Liposomes and nanotechnology in drug development: focus on ocular targets. Int J Nanomed 8:495–503
- Jiang T, Zhang Z, Zhang Y, et al. (2012). Dual-functional liposomes based on pH-responsive cell-penetrating peptide and hyaluronic acid for tumor-targeted anticancer drug delivery. Biomaterials 33:9246–58
- Kaiser JM, Imai H, Haakenson JK, et al. (2013). Nanoliposomal minocycline for ocular drug delivery. Nanomedicine 9:130–40
- Kuo HK, Chen YH, Wu PC, et al. (2012). Attenuated glial reaction in experimental proliferative vitreoretinopathy treated with liposomal doxorubicin. Invest Ophthalmol Vis Sci 53:3167–74
- Law S, Huang K, Chiang C. (2000). Acyclovir-containing liposomes for potential ocular delivery Corneal penetration and absorption. J Control Release 63:135–45
- Li N, Zhuang C, Miwanga XS, et al. (2009). Liposome coated with low molecular weight chitosan and its potential use in ocular drug delivery. Int J Pharm 379:131–8
- Li X, Nie S, Kong J, et al. (2008). A controlled-release ocular delivery system for ibuprofen based on nanostructured lipid carriers. Int J Pharm 363:177–82
- Li X, Zhang Z, Li J, et al. (2012). Diclofenac-biodegradable polymer micelles for ocular applications. Nanoscale 4:4667–73
- Lin C, Marjan Javadi B, Belnap DM, et al. (2014). Ultrasound sensitive eLiposomes containing doxorubicin for drug targeting therapy. Nanomedicine 10:67–76
- Liu S, Jones L, Gu FX. (2012). Nanomaterials for ocular drug delivery. Macromol Biosci 12:608–20
- Llopis MD, Martos MJ, Espana E, et al. (1992). Liposomally-entrapped ganciclovir for the treatment of cytomegalovirus retinitis in AIDS patients. Doc Ophthalmol 82:297–305
- Mannermaa E, Vellonen K-S, Urtti A. (2006). Drug transport in corneal epithelium and blood–retina barrier: emerging role of transporters in ocular pharmacokinetics. Adv Drug Deliv Rev 58:1136–63
- Peyman G, Ganiban G. (1995). Delivery systems for intraocular routes. Adv Drug Deliv Rev 16:107–23
- Sasaki H, Karasawa K, Hironaka K, et al. (2013). Retinal drug delivery using eyedrop preparations of poly-l-lysine-modified liposomes. Eur J Pharm Biopharm 83:364–9
- Shen J, Wang Y, Ping Q, et al. (2009). Mucoadhesive effect of thiolated PEG stearate and its modified NLC for ocular drug delivery. J Control Release 137:217–23
- Shen Y, Tu J. (2007). Preparation and ocular pharmacokinetics of ganciclovir liposomes. AAPS J 9:371–7
- Sunalp M, Wiedemann P, Sorgente N, Ryan S. (1984). Effects of Cyto-toxic drugs on proliferative vitreoretinopathy in the rabbit-cell injection model. Curr Eye Res 3:619–23
- Sunalpa MA, Wiedemanna P, Sorgentea N, Ryan SJ. (1985). Effect of adriamycin on experimental proliferative vitreoretinopathy in the rabbit. Exp Eye Res 41:105–15
- Urtti A. (2006). Challenges and obstacles of ocular pharmacokinetics and drug delivery. Adv Drug Deliv Rev 58:1131–5
- Volodkin D, Mohwald H, Voegel JC, Ball V. (2007). Coating of negatively charged liposomes by polylysine: drug release study. J Control Release 117:111–20
- Wu P, Tsai Y, Liao C, et al. (2004). The characterization and biodistribution of cefoxitin-loaded liposomes. Int J Pharm 271:31–9
- Yenice I, Mocan MC, Palaska E, et al. (2008). Hyaluronic acid coated poly-epsilon-caprolactone nanospheres deliver high concentrations of cyclosporine A into the cornea. Exp Eye Res 87:162–7