Abstract
DNA vaccines are simple to produce and can generate strong cellular and humoral immune response, making them attractive vaccine candidates. However, a major shortcoming of DNA vaccines is their poor immunogenicity when administered intramuscularly. Transcutaneous immunization (TCI) via microneedles is a promising alternative delivery route to enhance the vaccination efficacy. A novel dissolving microneedle array (DMA)-based TCI system loaded with cationic liposomes encapsulated with hepatitis B DNA vaccine and adjuvant CpG ODN was developed and characterized. The pGFP expression in mouse skin using DMA was imaged over time. In vivo immunity tests in mice were performed to observe the capability of DMA to induce immune response after delivery of DNA. The results showed that pGFP could be delivered into skin by DMA and expressed in skin. Further, the amount of expressed GFP was likely to peak at day 4. The immunity tests showed that the DMA-based DNA vaccination could induce effective immune response. CpG ODN significantly improved the immune response and achieved the shift of immune type from predominate Th2 type to a balance Th1/Th2 type. The cationic liposomes could further improve the immunogenicity of DNA vaccine. In conclusion, the novel DMA-based TCI system can effectively deliver hepatitis B DNA vaccine into skin, inducing effective immune response and change the immune type by adjuvant CpG ODN.
Introduction
Nearly one out of every three people in the world has been infected by hepatitis B virus (HBV). HBV infections result in high rate of developing cirrhosis, which is associated with a high risk of mortality due to the development of hepatocellular carcinoma (Hoofnagle et al., Citation1997). HBV vaccines used in humans are subunit vaccines produced by recombinant DNA technology. By July 2011, 179 countries had routine infant immunization with hepatitis B vaccine (World Health Organization Fact Sheet No. 204). However, there are some disadvantages of the current HBV vaccine (Michel & Loirat, Citation2001): (i) the high cost of production has hampered delivery of recombinant HBV vaccines in countries where HBV infection is endemic; (ii) a small portion of vaccinated individuals do not develop protective immunity even after more than the recommended three doses of HBsAg and (iii) the requirement of at least three injections to achieve protection.
The above problems may be solved by the use of the potentially more potent DNA vaccines (Davis et al., Citation1996). Furthermore, the DNA vaccines have superior stability in ambient temperature. Despite the potential attractive features of DNA vaccines, their use in clinical settings remains limited because of their poor immunogenicity, especially in non-human primates and humans (Donnelly et al., Citation2003; Ferraro et al., Citation2011).
Transcutaneous immunization (TCI) is receiving increased attention as an alternative route of immunization. In the TCI procedure, the skin is the target for vaccination. The skin has important immune functions, because the epidermis and dermis are highly populated by dentritic cells (DCs) and Langerhans cells (LCs), which can capture and present the antigens (Ag) to the lymph and initiate the immune response (Kupper & Fuhlbrigge, Citation2004; Pearton et al., Citation2010). Therefore, it is possible that DNA vaccine delivery would be substantially enhanced by target DNA to the highly immunoresponsive layers of the skin. However, the outer stratum corneum layer of skin represents a significant barrier to the delivery of genes and other high molecular weight agents, so improved delivery strategies are required to overcome this skin barrier property.
Recently, development of microneedles has enabled painless cutaneous delivery of various vaccines to skin layers with resident professional APCs (Sullivan et al., Citation2010; Van der Maaden et al., Citation2012). In our previous work, we have developed a microneedle and hydrogel patch formulation combined TCI system to induce effective immune response of hepatitis B surface antigen (HBsAg) (Guo et al., Citation2013a). Also, Yin et al. (Citation2013) have reported that the immune response of hepatitis B DNA vaccine has been enhanced by percutaneous administration with microneedles using a “poke and patch” strategy. However, large dose of DNA vaccine and adjuvants would be required by the “poke and patch” method. This is because a considerable part of DNA vaccines and adjuvants would be remained in the patch and not able to be delivered into the skin. Further, the precise amount of vaccine and adjuvants delivered into the skin could be difficult to quantify and varied by little difference in the operation.
In our previous work, we have developed a TCI system consisting of dissolving microneedle arrays (DMA), where in the tips was loaded with ovalbumin (OVA) as the model vaccine. It was demonstrated that the DMA TCI system was feasible to deliver OVA into skin and enhance the immune response (Guo et al., Citation2013b). In this article, we developed a novel DMA system to improve the immunogenicity of HBV DNA vaccine. Cationic liposomes encapsulated with HBV DNA vaccines and adjuvant CpG ODN was loaded into the DMA for a further improvement of immunogenicity. The gene expression in the skin by DMA was investigated. And the immune effect was evaluated and compared with conventional intramuscular (IM) injection in vivo.
Materials and methods
Subjects and materials
Horseradish peroxidase (HRP)-labeled goat anti-mouse IgG, IgG1 and IgG2a were purchased from Santa Cruz Biotechnology (Santa Cruz, CA). The CpG-ODN1826 (5-TCC ATGACG TTC CTG ACG TT-3) was synthesized by Beijing Sun Biotech Co., Ltd (Beijing, China). 1,2-Dipalmitoyl-sn-glycero-3-phosphocholine (DPPC), cholesterol, dimethyldioctadecylammonium (DDA) were purchased from Sigma (St. Louis, MO). Polydimethylsiloxane (PDMS, Sylgard 184) was purchased from Dow Corning (Midland, MI). Polyvinylpyrrolidone-K17 (PVP-K17) and PVP-K30 were purchased from Boai NKY Pharmaceuticals Ltd. (Henan, China).
Female Balb/c mice (6–8 weeks old) used in this study were purchased from Beijing Xinglong Experimental Animals Ltd. Co. (Beijing, China). All mice were housed under a special pathogen-free condition. All research protocols followed the eighth edition of Guide for the Care and Use of Laboratory Animals (2011).
Construction of recombinant plasmid and preparation of DNA vaccine
The plasmid DNA vaccine VR-E2E that containing a plasmid vector VR2012 encoding the middle (pre-S2 plus S) envelope proteins of HBV was prepared as follows: the target gene encoding middle (pre-S2 plus S) envelope proteins of HBV was obtained by PCR using plasmid G683-1 as the template (gift from Prof. Jun Cheng) and S2-5, S5, S3 as the primer (SBS Genetech Co., Ltd, Beijing, China). Then insert the target gene into a pGEM-T Easy vector (Promega Corporation, Beijing, China) to transform the competent DH5α. The plasmid DNA was extracted and double digested by Pst I and BamH I (Promega Corporation, Beijing, China). At the same time, the vector VR1012 (gift from Prof. Xiaofang Yu) was also double digested. The gene segment and vector was recombined to form the DNA vaccine VR-E2E. The reporter plasmids pGFP was amplified and purified from previously transformed stocks of Escherichia coli DH5α using antibiotic selective conditions and a QIAGEN Mega Kit (QIAGEN Ltd, Crawley, UK).
Encapsulation of DNA and CpG ODN in the cationic liposomes
The cationic liposomes containing VR-E2E (Lip+VR-E2E) were prepared as modified by previously described (Guo et al., Citation2013b). Briefly, the lipid phase consisting of DPPC, cholesterol and DDA was dissolved in ethanol in a round-bottom flask. The ethanol was removed by rotary evaporation and a thin lipid film on the bottom remained. The lipid film was hydrated by 10 mM phosphate-buffered saline (PBS) (pH 7.4). Then, PBS-containing VR-E2E was added and mixed for 10 min. The resulting liposomes suspensions were passed through a high-pressure homogenizer (EmulsiFlex-C5, Avestin, Ottawa, Canada) for three times and then extruded through two stacked 100-nm pore size polycarbonate filters 10 times to produce unilamellar vesicles with a uniform size (Li et al., Citation2012). The cationic liposomes containing VR-E2E plus CpG ODN (Lip+VR-E2E+CpG) or pGFP (Lip+pGFP) were prepared following the same method. The final concentrations of VR-E2E, pGFP and CpG ODN in the liposomes were 0.5, 0.5 and 1 mg/ml, respectively ().
Table 1. Characterizations of different liposome formulations.
Fabrication of DMA
Previously, we have fabricated microneedles using conical master-molds (Guo et al., Citation2013b). The microneedles were too thin that the tips were easy to broken. A critical departure from previously demonstrated fabrication approach is that in this study master-molds with tetrahedral structure were used which were more solid than the conical structure. The microneedle master-molds were made of aluminum with 36 (6 × 6) microneedles over an area of 6 × 6 mm2. Each microneedle has a tetrahedral structure, with a base length of ∼250 μm. The microneedles are 650 μm in height and have center-to-center spacing of 450 μm. A PDMS mold containing inverted wells was fabricated by pouring DMS over the microneedle master-mold and incubating it at 80 °C for 1 h to make the polymer cure.
The dissolving microneedles were prepared as follows: a solution for forming the tip of microneedles (tip solution) was prepared by dissolving PVP-K17 in the solution loaded with VR-E2E, or VR-E2E+CpG ODN, or Lip+VR-E2E, or Lip+VR-E2E+CpG ODN, or Lip-pGFP. The concentration of PVP-K17 in the tip solution was 5% (w/w). And the concentration of VR-E2E, pGFP and CpG ODN in the tip solution was 0.5, 0.5 and 1 mg/ml, if any. About 10 μl of the tip solution was applied to the PDMS mold and placed inside a pressure vessel with 300 kPa for 20 s to make the liquid formulation fill into the holes of microneedle mold. After the tip solution dried, a 50% (w/w) PVP-k30 solution was added to the mold and a vacuum was applied to remove air bubbles from the solution. Then, the mold was placed in the confined space with drying agent at 4 °C for 7 h to make it thoroughly dry. Finally, the microneedle arrays were gently peeled off the mold.
Mechanical testing of DMA
DMA were subjected to mechanical tests for compression and skin insertion. The mechanical properties were evaluated using an analyser combined by a side-shaking test stand (HCS-500) and a thrust meter (HF-50; Wenzhou Tripod Instrument Manufacturing Co Ltd, Zhejiang, China). DMA were visualized before and after application of the compression load using a digital camera (Canon, Tokyo, Japan).
To investigate insertion properties, the porcine ear skin were used as the model for human skin. The skin was placed, stratum corneum side up, on a 500-μm thick sheet of dental wax topped with parafilm and this assembly was then secured on a wooden block for support. Using double-sided adhesive tape, DMA were carefully attached to the moveable cylindrical probe. The probe was lowered onto the skin at a speed of 0.5 mm/S. After removal of DMA, the surface of skin was stained with Trypan blue and then photographed using the digital camera.
Stability of DNA vaccine following microneedle preparation and storage
The biological functionality of the recovered VR-E2E was accessed by the transfection of cells in vitro. About 5 μg of VR-E2E freshly prepared and VR-E2E loaded DMA were dissolved in 200 μl PBS, respectively. The above solution was mixed with 6-μl Lipofect Transfection Reagent (Beijing Tiangen Biotech, Beijing, China). After placed at room temperature for 15 min, the transfection complexes were added to cos-7 cells that had been cultured to 70% confluency. After cell culture, the amount of HBsAg in supernatant of cell culture fluid was detected by enzyme-linked immunosorbent assay (ELISA) as previously reported (Guo et al., Citation2013a).
Dissolution kinetics analysis of DMA in vivo
The hair on the abdominal skin of mice was removed before the experiment. The DMA was applied to mice skin for 0, 1, 2 and 3 min and then removed. The remained length of microneedles was measured using microscopy.
Delivery of plasmid DNA to mouse skin using DMA
For effective DNA vaccination, delivered DNA should transfect skin cells. Therefore, we first tested delivery of the reporter plasmid DNA expressing green fluorescent protein. Mice were treated with DMAs loaded with or without Lip+pGFP. The mice were anesthetized by intraperitoneal (i.p.) injection of Pelltobarbitalum natricum (80 mg/kg), and then the hair of abdominal skin was carefully shaved using electric clippers and rested for 24 h. The shaved area of the abdominal skin was carefully wiped with 75% ethanol to make the skin clear before vaccination. Finally, the DMA were applied to the shaved skin with a force of ∼2 N and held for 3 min. The treated skin area was stripped from the mice at 0, 1, 4 and 7 days, and immediately imaged using a multiphoton confocal microscopes (Nikon, A1RMP, Tokyo, Japan).
Vaccine protocol
In order to investigate the immune response, female Balb/c mice (six in each group) were vaccinated. Before microneedle treatment, the hair of abdominal skin was carefully shaved using electric clippers and rested for 24 h. DMAs were inserted into the abdominal skin of mice for 3 min. This procedure was repeated again after 3 weeks.
Determination of immunity response
All mice were bled from the tail vein 2 weeks after final vaccination, and the serum was separated for antibody titers analysis. The anti-HBsAg antibody titer in serum was measured by ELISA as previously reported (Guo et al., Citation2013a).
Statistical analysis
Statistical analysis was performed on the data from in vitro and in vivo studies by analysis of variance (ANOVA) used SPSS software (SPSS Inc., Chicago, IL). The level of significance was chosen a p value of ≤0.05 (two-tailed).
Results and discussion
Fabrication and characterization of DMA
To investigate the localization of vaccine, we used Trypan blue as the model drug loaded only in the microneedle tips. The micrographs of DMA containing Trypan Blue are shown in . shows a 6 × 6 microneedle array containing Trypan blue only in the microneedle tips. shows a typical microneedle, with a length of ∼650 μm, and the mean length of the drug-loaded space was ∼470 μm.
Mechanical testing of DMA
A critical departure from previously demonstrated fabrication approach is that in this study master-molds with tetrahedral structure were used which were more solid than the conical structure. The images of DMA and stained skin after mechanical testing are shown in . As shown in , when the applied force was 4 N, the tips of DMA began to be damaged. As applied force was increased, there was a progressive decrease in DMA height. When the applied force was increased to 20 N, there was nearly half of the DMA height decreased. At the same time, as shown in , increasing the applied force resulted in an increase in the penetration efficiency of DMA. When the applied force was 1 N, only 60% of microchannels were created in the skin. At insertion forces of 2 and 4 N, the percentage of microchannels created in the skin were 100%. From the above, the force using to insert DMA into skin was 2 N in the following experiments.
Stability of DNA following microneedle preparation
The concentration of HBsAg obtained from the transfection of freshly prepared VR-E2E was 9.90 ± 0.10 ng/ml. And the concentration of HBsAg obtained from the transfection of freshly prepared DMA loaded with VR-E2E was 9.76 ± 0.26 ng/ml. It indicated that VR-E2E was stable during the preparation of DMA.
Dissolution kinetics of DMA
The dissolution kinetics of DMA after applied to the mice skin is shown in . The drug-loaded space was mostly dissolved in 2 min. After 3 min, the microneedles were completely dissolved. These data showed that the DMA could rapidly dissolve in mice skin within 3 min. So in the following animal immunity tests, the DMA should be applied to the mice for only 3 min.
Expression of pGFP in skin
The expression of pGFP loaded in DMA after inserted into skin was accessed in vivo. We inserted two types of DMAs; one as a negative control without DNA loaded and the other loaded with the Lip+pGFP. Zero, 1, 4 and 7 days after microneedle delivery, the treated skin area were visualized by multiphoton confocal microscopy. The excitation wavelength was 405 and 488 nm. The skin was imaged from the stratum corneum side and up to the depth of ∼200 μm.
Three-dimensional graphs of the visualized area are shown in . The negative control showed similar images at each point of time, so only one image is shown () that there were regular blue fluorescent columns through the skin. The blue fluorescent columns were also shown in other time point of images (), which were suggested to be the autofluorescence of mouse hair. From Day 0–4, the GFP level was increasing. The highest GFP level was observed at Day 4 (), almost the entire skin area was green. And at Day 7, the GFP expression level in the skin significantly decreased. Yan et al. (Citation2014) had reported a DNA delivery method with a motorized microneedle device. They observed the highest gene expression level in the skin at Day 1, and after that, the gene expression level decreased over the time quickly. Similar transient gene expression in the skin was also observed in the delivery of DNA into rat skin with an electroporation method (Pavselj & Preat, Citation2005) and in the delivery of DNA into ex vivo human skin with a tattooing device (Van den Berg et al., Citation2009). The long duration of gene expression in this study might due to the sustained release of cationic liposomes (Christensen et al., Citation2009).
Immune response
Administration route and adjuvant
We first investigate the influence of adjuvant and delivery routes to the immune response of VR-E2E. The immune groups were as follows: (i) DMA containing only VR-E2E; (ii) DMA containing VR-E2E and CpG ODN; (iii) DMA containing Lip+VR-E2E; (iv) DMA containing Lip+VR-E2E+CpG ODN; (v) IM immunization with Lip+VR-E2E; (vi) IM with Lip+VR-E2E+CpG ODN and (vii) DMA containing Lip+CpG ODN. Among these groups, Groups V and VI were positive control and Group VII was negative control. VR-E2E and CpG ODN were both 10 μg/dose, if any.
shows the antibody response generated by different groups. It was shown that using Lip+VR-E2E or Lip+VR-E2E+CpG ODN, DMA vaccination induced similar anti-HBsAg IgG titers with IM immunization (p > 0.05). It might due to two reasons: (i) during the DMA preparation, a fraction of VR-E2E was diffused from the tip to the base and (ii) during the DMA insertion, although the microneedles were totally dissolved in 3 min (data not shown), part of DMA might be left on the skin around the microchannels. Actually, we have used interferon α-2b as the model drug, and found the proportion of the total dose retained in needle tips was 52.9 ± 4.2%, and the proportion delivered into skin was 31.2 ± 1.9%, respectively. Therefore, comparing to total dose entered in body in IM immunization, only a fraction of the dose loaded in DMA could be delivered into body. It implied that inducing the same immune response, the actual dose delivered into body by DMA was less than that by IM (Moon et al., Citation2013). We are currently investigating to decrease the diffusion during DMA preparation and reduce the loss during DMA insertion, and improve the efficiency of the vaccine.
In the TCI groups, the group immunized with free VR-E2E showed the lowest IgG titer. Cationic liposomes or CpG ODN alone showed similar adjuvant effect. The level of IgG in the group immunized with DMA containing Lip+VR-E2E+CpG ODN was the highest IgG titer compared with other groups (p < 0.05). It suggested adjuvant effect of cationic liposomes and CpG ODN could be mutually reinforcing by this delivery method. It was consistent with previous reports that the adjuvant activity of CpG ODNs was improved by co-encapsulating them in liposome vesicles (Gursel et al., Citation2001).
Dose and adjuvant
Lip+VR-E2E containing 2.5, 5 and 10 μg VR-E2E with or without CpG ODN adjuvant were applied by DMA vaccination. The data are shown in .
The groups with 10 μg VR-E2E/dose showed the highest IgG titers among the three doses. The groups with CpG ODN adjuvant showed higher IgG titer than those without CpG ODN at the same VR-E2E dose except the Lip+VR-E2E (2.5) group (p < 0.05). And it suggested that at least 5 μg VR-E2E was needed to achieve a log 10 IgG titer >3. As previously reported, TCI with FMA and hydrolgel combination needed at least 25 μg HBsAg/dose to achieve a log 10 IgG titer of 3.08 ± 0.20 [10], and the dose of HBsAg used in conventional TCI was ∼100 μg/dose (Gill & Prausnitz, Citation2007). Overall, HBV DNA vaccination using DMA could elicit effective immune response with a small dose.
Immune types
We also analyzed the IgG1 and IgG2a antibody subtypes as the marker to evaluate the Th2 and Th1 immune response, respectively (Coffman et al., Citation1993). For mice, IgG1 present the Th2-type immune response (humoral immunity) and IgG2a present the Th1-type immune response (cellular immunity). It was observed that all groups had the ability to induce both IgG1 and IgG2a subclasses antibodies ().
Figure 7. Anti-HBs specific IgG1 and IgG2a titers. (A) The log10 IgG1 and IgG2a titers. (B) The IgG2a/IgG1 ratio.
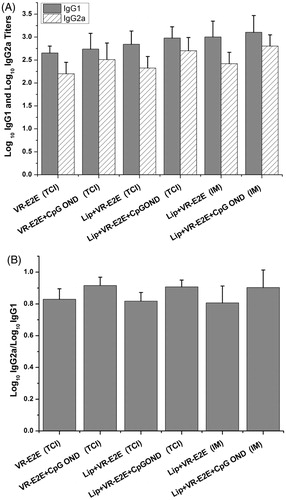
In the DMA groups, CpG ODN could significantly improve the IgG2a titers (p < 0.05). Further, as shown in , CpG ODN could significantly improve the IgG2a/IgG1 ratios, so that the ratios were closer to 1. It indicated that using the CpG ODN adjuvant could induce potent Th1 responses and get a more balanced Th1/Th2 immune response.
There was no significant difference between the IgG2a/IgG1 ratios obtained by VR-E2E group and Lip+VR-E2E group (p > 0.05). Also, there was no significant difference between the VR-E2E+CpG ODN group and Lip+VR-E2E+CpG ODN (p > 0.05). It suggested that the cationic liposomes were not able to regulate the type of immune response.
As shown in , using the same vaccine components, there was no significant difference between the IgG2a/IgG1 ratios obtained by DMA and IM vaccination (p > 0.05). Using Lip+VR-E2E, the IgG2a/IgG1 ratios were both ∼0.8. Likewise, using Lip+VR-E2E+CpG ODN, the IgG2a/IgG1 ratios were both ∼0.95. It indicated that CpG ODN could significantly improve the IgG2a/IgG1 ratios, and subsequently regulate Th1/Th2 balance, whether by DMA or IM vaccination. The mechanism and outcome for the subtype differences may be as follows: the differentiation of an antigen specific CD4 T helper subset (Th1 or Th2) takes place at the time of priming and the type of CD4 subset stimulated will depend on a number of factors. One of the main factors that influence the differentiation of Th subsets is the cytokine environment at the time of priming (Constant et al., Citation1997). The adjuvant used can alter the cytokine environment at the site of the primary immune response. CpG ODN are capable of up-regulating the secretion of cytokines such as TNF-α, IL-18, IL-12 and IL-6 and production of immunoglobolins by B cells, and subsequently induce more Th1 biased immune responses (Klinman et al., Citation1996).
Conclusions
In this study, we showed for the first time that DMA could be prepared for TCI of HBV DNA vaccine. The skin insertion test showed DMA had enough rigidity to penetrate skin with the tips loaded with cationic liposomes and CpG ODN. The encapsulation of DNA vaccine into cationic liposomes obtained a sustained release and subsequent long duration of gene expression in skin. The in vivo immune test showed effective immune response by this delivery method, and the adjuvant could improve immunogenicity when the dose of DNA vaccine was >5 μg. The antibody subtype tests showed CpG ODN could significantly improve the IgG2a/IgG1 ratios and get a more balanced Th1/Th2 immune response. Overall, DMA present beneficial delivery method to the TCI of HBV DNA vaccine. And the adjuvant cationic liposomes and CpG ODN provides a promising immune response for the TCI of HBV DNA vaccine.
Declaration of interest
The authors report no conflicts of interest. The authors alone are responsible for the content and writing of this article.
This work was supported by the Natural Science Foundation of China (31306909) and Science Foundation of The Chinese Academy of Sciences.
References
- Christensen D, Agger EM, Andreasen LV, et al. (2009). Liposome-based cationic adjuvant formulations (CAF): past, present, and future. J Liposome Res 19:2–11
- Coffman RL, Lebman DA, Rothman P. (1993). Mechanism and regulation of immunoglobulin isotype switching. Adv Immunol 54:229–70
- Constant SL, Bottomly K. (1997). Induction of Th1 and Th2 CD4+ T cell responses: the alternative approaches. Annu Rev Immunol 15:297–322
- Davis HL, McCluskie MJ, Gerin JL, Purcell RH. (1996). DNA vaccine for hepatitis B: Evidence for immunogenicity in chimpanzees and comparison with other vaccines. Proc Natl Acad Sci USA 93:7213–18
- Donnelly J, Berry K, Ulmer JB. (2003). Technical and regulatory hurdles for DNA vaccines. Int J Parasitol 33:457–67
- Ferraro B, Morrow MP, Hutnick NA, et al. (2011). Clinical applications of DNA vaccines: current progress. Clin Infect Dis 53:296–302
- Gill HS, Prausnitz MR. (2007). Coated microneedles for transdermal delivery. J Control Release 117:227–37
- Guo L, Chen JM, Qiu YQ, et al. (2013b). Enhanced transcutaneous immunization via dissolving microneedle array loaded with liposome encapsulated antigen and adjuvant. Int J Pharmaceut 447:22–30
- Guo L, Qiu YQ, Chen JM, et al. (2013a). Effective transcutaneous immunization against hepatitis B virus by a combined approach of hydrogel patch formulation and microneedle arrays. Biomed Microdevices 15:1077–85
- Gursel I, Gursel M, Ishii KJ, Klinman DM. (2001). Sterically stabilized cationic liposomes improve the uptake and immunostimulatory activity of CpG oligonucleotides. J Immunol 167:3324–8
- Hoofnagle JH, DiBisceglie AM. (1997). The treatment of chronic viral hepatitis. New Engl J Med 336:347–56
- Klinman DM, Yi AK, Beaucage SL, et al. (1996). CpG motifs present in bacteria DNA rapidly induce lymphocytes to secrete interleukin 6, interleukin 12, and interferon gamma. Proc Natl Acad Sci USA 93:2879–83
- Kupper TS, Fuhlbrigge RC. (2004). Immune surveillance in the skin: mechanisms and clinical consequences. Nat Rev Immunol 4:211–22
- Li N, Zhuang CY, Wang M, et al. (2012). Low molecular weight chitosan-coated liposomes for ocular drug delivery: In vitro and in vivo studies. Drug Deliv 19:28–35
- Michel ML, Loirat D. (2001). DNA vaccines for prophylactic or therapeutic immunization against hepatitis B. Intervirology 44:78–87
- Moon S, Wang YH, Edens C, et al. (2013). Dose sparing and enhanced immunogenicity of inactivated rotavirus vaccine administered by skin vaccination using a microneedle patch. Vaccine 31:3396–402
- Pavselj N, Preat V. (2005). DNA electrotransfer into the skin using a combination of one high- and one low-voltage pulse. J Control Release 106:407–15
- Pearton M, Kang SM, Song JM, et al. (2010). Influenza virus-like particles coated onto microneedles can elicit stimulatory effects on Langerhans cells in human skin. Vaccine 28:6104–13
- Sullivan SP, Koutsonanos DG, Martin MD, et al. (2010). Dissolving polymer microneedle patches for influenza vaccination. Nat Med 16:915–20
- Van den Berg JH, Nuijen B, Beijnen JH, et al. (2009). Optimization of intradermal vaccination by DNA tattooing in human skin. Hum Gene Ther 20:181–9
- Van der Maaden K, Jiskoot W, Bouwstra J. (2012). Microneedle technologies for (trans)dermal drug and vaccine delivery. J Control Release 161:645–55
- Yan G, Arelly N, Farhan N, et al. (2014). Enhancing DNA delivery into the skin with a motorized microneedle device. Eur J Pharm Sci 52:215–22
- Yin DF, Liang WQ, Xing SX, et al. (2013). Hepatitis B DNA vaccine-polycation nano-complexes enhancing immune response by percutaneous administration with microneedle. Biol Pharm Bull 36:1283–91