Abstract
This study aimed to evaluate transdermal delivery of vancomycin hydrochloride using the combination of ethosomes as an encapsulating vesicle and iontophoresis. Ethosomes were prepared and evaluated in terms of electrochemical stability. Cathodal iontophoresis of negatively charged ethosomes and anodal iontophoresis of free drug solution and positively charged vesicles were conducted. The effect of current mode, density, concentration of drug and ionic strength was studied. In vivo study was performed by inducing mediastinitis in Sprague-Dawley rats using methicillin-resistant Staphylococcus aureus as infected pathogen, the mean bacterial count was compared between groups of rats, one of the treated groups received drug intramuscularly while the other group received vancomycin using iontophoretic delivery of optimized ethosomal formula. Ethosomes showed efficient electrochemical stability, cathodal iontophoresis of negatively charged vesicle (F2) showed maximum transdermal flux (550 µg/cm2/h) compared to free drug solution and other ethosomal formulae, transdermal flux was reduced by altering current mode from continuous to ON/OFF mode, reducing current density and by using normal saline as drug solvent; on the other hand, flux was potentiated by increasing drug concentration from 25 to 75 mg/ml. In vivo study revealed that there was a significant difference in terms of bacterial count between untreated and treated groups, while there was no statistically significant difference between the I.M. vancomycin treatment and treatment conducted by iontophoretic delivery of vancomycin encapsulated in ethosomal formula. Combination between ethosomes and iontophoresis had succeeded in delivering vancomycin transdermally.
Introduction
Vancomycin hydrochloride is a glycopeptide antibiotic with a relatively high molecular weight (1485.7 Da), used in the prophylaxis and treatment of infections caused by gram-positive bacteria (Lin & Hayden, Citation2010). Adjustment and targeting of specific serum concentrations of vancomycin in patients has been the subject of debate for many years. The primary premise for monitoring and adjustment of serum vancomycin concentrations is based on the perceived need to achieve serum concentrations above the minimum inhibitory concentration (MIC) for the offending organisms and the avoidance of potential adverse effects, such as ototoxicity or nephrotoxicity. Therefore, designing a delivery system capable of providing sustained serum level of vancomycin hydrochloride above MIC of target pathogen for prolonged duration is a desirable target.
Iontophoresis is a physical non-invasive method that involves the application of a low electrical potential gradient across the skin to enhance molecular transport. Iontophoresis can provide rapid delivery of drug molecules both locally and systemically (Kumar & Banga, Citation2012). However, iontophoresis only improves small molecular drugs transdermal delivery. In the cases of big molecules, such as protein and DNA, iontophoresis had to be combined with the other transdermal enhancement methods to improve trans-dermal delivery (Liu et al., Citation2014).
Several researchers (Gu et al., Citation2003; Chakraborty et al., Citation2012; Muppidi et al., Citation2012) noted that vancomycin encapsulation into liposomal or nano-sized vehicle offered an enhancement in pharmacodynamics, pharmacokinetics characteristics of drug and decreased its toxicity compared to standard preparations. The use of nanoparticle for vancomycin delivery was limited not only to enhancing antimicrobial activity of drug via enhancing drug penetration into bacterial cell but also extended to enhance drug permeation across biological barriers, where Zakeri-Milani et al. (Citation2013) formulated PLGA nanoparticles that could provide a delivery system for vancomycin, with enhanced intestinal permeability.
The present work is focused on evaluating the potential of ethosomes combined with iontophoresis in transdermal delivery of macromolecules, and to formulate a new delivery system of vancomycin drug capable of providing sustained and controlled release of the drug.
Materials and methods
Materials
Vancomycin hydrochloride was a gift from Sigma Tec, Giza, Egypt. Soy phosphatidylcholine and stearylamine were purchased from Sigma-Aldrich, St. Loius MO. Potassium dihydrogen phosphate, disodium hydrogen phosphate and sodium chloride were purchased from El-Nasr Pharmaceutical Co., Qalyubiya, Egypt. All other reagents and solvents were of analytical grade.
Methods
Preparation of ethosomes
Vancomycin hydrochloride ethosomes were prepared by cold method (Prasanthi & Lakshmi, Citation2012). The ethosomal system of vancomycin hydrochloride composed of 1% w/v soy phospholipids (SPC), 45% v/v of ethanol, 10% v/v propylene glycol (PG) and water up to 100% v/v. SPC was dissolved in ethanol and heated to 30 °C in water bath. Distilled water containing 250 mg of vancomycin hydrochloride was added slowly in a fine stream with a constant mixing using mechanical stirrer, at 700 rpm in a closed vessel. The temperature was maintained at 30 °C during the experiment. The mixing was continued for 5 min. Ethosomes suspensions were transferred to a 15 ml centrifuge tube. The suspension was centrifuged at 13 000 rpm for 1 h at 4 °C using a cooling centrifuge (Sigma 3 K 30, Osterode, Germany), then resuspended in PBS. The prepared solution was stored at 4 °C.
Preparation of surface modified ethosomes
For the selected formulae, 0.4% stearylamine (SA) was added as shown in . stearylamine was mixed with SPC and dissolved in ethanol before adding aqueous phase. The remaining steps of the preparation process were followed as mentioned above.
Table 1. Composition of ethosomal formulae.
Electrochemical stability of vancomycin hydrochloride ethosomal formulae
The two electrode wires were perpendicularly immersed in ethosomal dispersion. The distance between the two electrodes was 15 mm. A constant current of 0.5 mA was passed through the system for 12 h at 37 °C. The system was agitated with magnetic stirrer and the encapsulation efficiency of vancomycin hydrochloride ethosomes was examined before and after this experiment to determine the electrostability of ethosomes (Fang et al., Citation1999).
In vitro skin permeation study
The in vitro skin permeation of vancomycin hydrochloride was studied using locally fabricated Franz’s diffusion cell with an effective permeation area and receptor cell volume of 3.14 cm2 and 30 ml, respectively. The temperature of the receiver vehicle (PBS, pH 7.4) was maintained at 37 ± 1 °C and was constantly stirred by a magnetic stirrer at 100 rpm. The skin was mounted on a receptor compartment with the SC side facing toward the donor compartment. The cathode electrode was immersed in the donor compartment filled with 6 ml (25 mg/ml) of negatively charged ethosomal formulae (F1 and F2) containing to 150 mg of vancomycin hydrochloride, while the anode was placed in the receiver compartment containing phosphate buffer saline pH 7.4, the position of the electrodes was reversed in case of positively charged formulae (F3, F4 and free drug solution). The current density was adjusted to predetermined value. Aliquots of 0.5 ml samples were withdrawn every hour for 12 h, then immediately analyzed by UV spectrophotometer. The same amount of fresh media was added to the receptor compartment to replace what had been removed to ensure sink conditions, the results are the mean values of three runs.
Effect of iontophoresis mode
The effect of iontophoresis mode on the drug delivery was studied by performing continuous current and ON/OFF mode (30 min ON and 30 min OFF) iontophoresis at drug concentration 25 mg/ml and using deionized water as a solvent for F2.
Effect of current density
The effect of current density on delivery was studied to explore the relation between the different current densities applied (0 mA/cm2, 0.25 mA/cm2 and 0.5 mA/cm2) and the amount of drug delivered using iontophoresis at drug concentration 25 mg/ml and using deionized water as a solvent for F2.
Effect of drug concentration
The effect of vancomycin hydrochloride on the delivery was studied at 25, 50 and 75 mg/ml at current density 0.5 mA/cm2 and using deionized water as a solvent for F2.
Effect of drug solvent type (ionic strength)
Effect of vancomycin hydrochloride solvent type was studied by using deionized water and normal saline as drug solvents in donor compartment for F2 at 25 mg/ml drug concentration at current density 0.5 mA/cm2.
Permeation data analysis
The permeation profile was conducted by plotting the cumulative amount of permeated drug (μg/cm2) versus time (h), the steady state flux Jss (μg/cm2·h) was calculated from the slope of the linear portion, permeability coefficient [kp] was calculated by dividing [Jss] by the employed concentration of the drug (Co) according to the equation: kp = Jss/Co.
In-vivo study
Twenty-four male Sprague-Dawley rats were divided into four groups, each of six; first group was set as negative control while the other groups were subjected to mediastinitis using methicillin-resistant Staphylococcus aureus (MRSA) as infected pathogen. The procedure was approved by the Ethical Committee of Faculty of Pharmacy, Cairo University. In brief, the animals were anesthetized through an I.M. injection of ketamine (30 mg/kg) and secured in supine position. The hair on sternal region was shaved and skin was cleaned with 70% isopropyl alcohol and then covered with sterile drape. Sternum was exposed through a midline skin incision, and an upper median sternotomy was performed using a sterile blade. The mediastinal layers and exposed sternal edges were inoculated with 0.5 ml × 108 colony-forming unit (CFU)/ml MRSA (ATCC33591). The subcutaneous tissue and skin were primarily closed with nylon monofilament sutures (Ozcan et al., Citation2006).
Group II was set as positive control where animals were infected and no treatment was given. While Group III vancomycin was given in a dose equal to 40 mg/kg/day via intramuscular injection for the treatment of mediastinitis for 3 days and Group IV vancomycin was given by iontophoretic delivery of ethosomal formula using IontoTM Phoresor II (Chattanooga, TN), where the cathodal patch (IOMED Company, Salt Lake City, UT) with an effective area of 13.4 cm2 was filled with 2 ml of vancomycin ethosomal formula (4 mg/ml), then the patch was placed on shaved dorsal rat skin and the anodal patch was placed at 1 cm2 away from the cathodal patch and wetted with normal saline solution. The patch for 24 h was applied on rat dorsal skin.
These procedures were repeated for 3 days, and on the fourth day the rats were scarified. A sternotomy was performed, and a tissue samples including a part of sternum and the adjacent mediastinal soft tissue were aseptically obtained. Mediastinal tissue samples were weighed and homogenized under sterile conditions in 1 ml of sterile phosphate-buffered saline. The serial diluted homogenates were inoculated (0.01 ml) onto blood agars, incubated at 37 °C for 48 h, and the quantity of growing bacteria was determined in terms of CFU/g tissue.
Statistical analysis of data
In order to investigate the significant difference between the results of studied formulae, the one-way analysis of variance (ANOVA) test was used. The level of significance was set at 0.05, and ‘*p < 0.05’ was considered to be statistically significant.
Result
The ethosomal formulae were formulated and characterized as mentioned before (Mohammed et al., Citation2014).
Electrochemical stability of vancomycin hydrochloride ethosomal formulae
The percentages of vancomycin hydrochloride in ethosomal formulae after continuous exposure for 12 h to 0.5 mA/cm2 current were ranged from 97.35 to 94.84% compared to initial concentration at zero time, as shown in .
Table 2. The % of vancomycin hydrochloride in ethosomal formulae after continuous exposure for 12 h to 0.5 mA/cm2 current.
Transdermal iontophoresis of vancomycin hydrochloride ethosomes
illustrates drug permeation profile of iontophoretic drug delivery of vancomycin hydrochloride from free drug solution and different ethosomal formulae at 0 and 0.5 mA/cm2, while shows transdermal flux (Jss) of different formulae at 0 and 0.5 mA/cm2; shows permeation parameters, where F2 showed maximum quantity of drug delivered after 12 h (Q12) and transdermal flux (Jss) at 0.5 mA/cm2.
Figure 1. Permeation profile of vancomycin hydrochloride from free drug solution and different ethosomal formulae with/without iontophoresis.
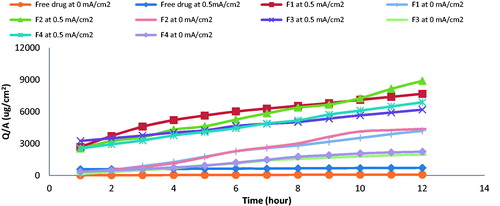
Figure 2. Transdermal flux (Jss) of free drug and ethosomal formula at 25 mg/ml drug concentration, 0 mA/cm2, 0.5 mA/cm2 and continuous current density (n = 3, error bars represent S.D. values).
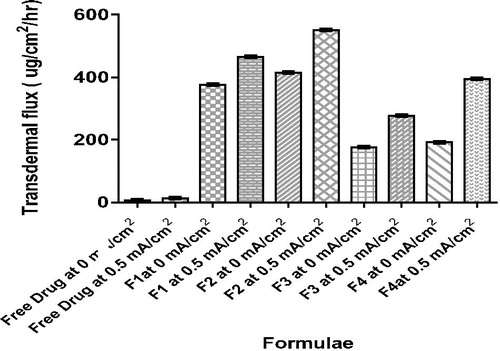
Table 3. Permeation parameters of vancomycin hydrochloride as free drug and from ethosomal formulae combined with iontophoresis at 0 and 0.5 mA/cm2 current.
Effect of current mode
The transdermal flux (Jss) obtained by applying cathodal iontophoresis of F2 using continuous current of 0.5 mA/cm2 density was 550 µg/cm2/h; by altering iontophoresis mode to be ON/OFF at same current density, the transdermal flux (Jss) decreased to 458.1 µg/cm2/h, as shown in .
Effect of current density
The transdermal flux (Jss) of the drug reached 550 µg/cm2/h by applying cathodal iontophoresis of F2 using current of 0.5 mA/cm2 density; by reducing the current density to 0.25 mA/cm2 and 0 mA/cm2, the transdermal flux (Jss) decreased to 526.4 and 414.7 µg/cm2/h, respectively, as shown in .
Effect of drug concentration
The transdermal flux (Jss) was increased from 550 µg/cm2/h to 880 and 1197 µg/cm2/h on increasing concentration from 25 mg/ml to 50 and 75 mg/ml, respectively, as shown in .
Effect of drug solvent type (ionic strength)
The addition of 0.9% normal saline to ethosomal formulae F2 led to a significant (p < 0.05) decrease in the vancomycin hydrochloride permeation across the skin, as shown in ; the transdermal flux was decreased from 550 to 325 µg/cm2/h.
In-vivo study
The study was performed and no complications related to the induction of mediastinitis or treatment techniques were observed. None of the animals in the uncontaminated group had signs of mediastinitis. On the other hand, all the rats in the untreated contaminated group exhibited apparent infection evident by pus drainage at the surgical site. The mean bacterial count was compared for the four groups as shown in and . The uncontaminated group (GP I) showed no bacterial growth of MRSA, while the other three contaminated groups (GP II, III and IV) showed bacterial growth of MRSA where microbiological examination of cultures revealed significantly higher bacterial counts in the untreated contaminated group (GP II) when compared with the treated groups (GP III and IV, p < 0.05). There was no statistically significant difference in terms of mean bacterial counts between the I.M. vancomycin treatment and treatment conducted by iontopherteic delivery of vancomycin encapsulated in ethosomal formula (p > 0.05).
Table 4. Mean bacterial count of four groups.
Discussion
Ethosomes showed efficient electrochemical stability to undergo the iontophoresis study, where the electric current may induce pores widening in phospholipid bilayers, resulting in the irreversible breakdown of lipid vesicles and the leakage of drug (Winterhalter, Citation1996). However, in order for the pore widening and irreversible breakdown of the ethosomes to happen, a higher electric current must be introduced. The 0.5 mA/cm2 current density used in our study may be too low to induce the rupture of the vesicles. These results were in agreement with Wilhelm et al. (Citation1993), who found that 0.5 mA/cm2 current density was not sufficient to induce lipid bilayer membrane breakdown.
On comparing the in-vitro permeation, parameters obtained from iontophoretic delivery of free drug solution and ethosomal formulae with its corresponding passive diffusion (at 0 mA/cm2), a significant increase (p < 0.05) in the quantity of drug delivered after 12 h (Q12) was found in addition to increase in transdermal flux (Jss), these results were in agreement with Tomoda et al. (Citation2012), who found that the concentration of indomethacin in skin was twice higher using iontophoresis combined with nano-particle compared to drug delivery without applying iontophoresis. Previous research has suggested that the epidermal alterations induced by an electric field result in pore formation and changes in epidermal membrane permeability leading to better drug permeation across the skin. Where Prasad et al. (Citation2007) studied skin alteration during iontophoresis and stated that the ATR-FTIR spectra of iontophoresis showed a phenomenal split in amide II band into 1553 and 1541 cm−1. The split could be due to the disruption in hydrogen bonding associated with the head of ceramides, resulting in loosening of lipid–protein domains, thus allowing higher flux as compared to the passive treatment.
On comparing the in-vitro permeation parameters obtained from iontophoresis of ethosomal formulae bearing vancomycin hydrochloride with anodal iontophoresis of drug aqueous solution, a significant increase (p < 0.05) in the quantity of drug delivered after 12 h (Q12) was obtained and also an increase in transdermal flux (Jss) was observed. These results were in agreement with Kajimoto et al. (Citation2011), who stated that conventional iontophoresis is only, in particular, applicable for transdermal administration of small, hydrophobic and charged molecules (Prausnitz & Langer, Citation2008).
The cathodal delivery of negatively charged ethosomes (F1 and F2) was opposed owing to the presence of a negative charge on the skin under physiological conditions and the electroosmotic solvent flow in the (opposite) anode-to-cathode direction. The cathodal delivery of negatively charged ethosomes revealed significant increase (p < 0.05) in the quantity of drug delivered after 12 h (Q12) and in transdermal flux (Jss) compared to anodal iontophoresis of positively charged ethosomes (F3 and F4) at the same experimental condition. The shielding effect of SA was considered as a contributing factor in explaining the results of anodal iontophoresis of positively charged ethosomes. These were in agreement with Fang et al. (Citation1999), who found that the incorporation of charged inducer in the liposome may result in an alteration of the transdermal behavior of enoxacin. Under anodal iontophoresis, the permeation rate and skin residue of enoxacin were greatly reduced after incorporation of SA in the liposomes; moreover, both the permeation rate and skin residue of enoxacin decreased with an increase of SA concentration in liposomes due to the shielding effect of SA. Where SA was associated strongly with the negatively charged portion of the skin at and as the concentration of SA increases, more inherent fixed charge of the skin would be effectively shielded or reversed in the ion-conducting pathways. Similar results were observed by Kirjavainen et al. (Citation2000), who found that SA partly neutralize or reverse the negative charge in the SC as it penetrated into the skin, thus leading to a decreased drug flux. Hence, the effects of SA are assumed to be mediated by the alterations in the charge of the SC structures. Same results were also observed by Li et al. (Citation2002), who found that the pretreatment of skin with cationic surfactant DTAB, during iontophoresis of R-apomorphine had an inhibition effect. DTAB is driven into the skin and compensates for the native negative charge of human stratum corneum, thereby reducing the electroosmotic flow. The overall iontophoretic flux of R-apomorphine is dominated by the electromigration force from the anode to the cathode.
Another explanation for poor permeation of positively charged ethosomes combined with anodal iontophoresis was the competition of positively charged cationic surfactant SA with positively charged vancomycin hydrochloride, where SA had relatively small molecular weight (296.5 Da) compared to vancomycin hydrochloride (1458.7 Da), the smaller molecule will be carried more easily across the skin compared to larger molecule leading to a reduced drug permeation.
The persistent use of direct current (DC) can reduce the iontophoretic flux because of its polarization effect on the skin. This can be overcome by the use of on/off mode which is a direct current delivered in a periodic manner (Wu et al., Citation2004). In the present study, continuous DC current provided significant high drug permeation from ethosomal formulae in comparison with on/off mode. These results were in agreement with Sarvaiya et al. (Citation2013), who found that the continuous DC current provided significant high lovastatin permeation from micellar composition in comparison with pulsed DC current.
Our findings support the hypothesis that the transdermal flux (Jss) is proportional to the current density applied (Guy et al., Citation2001: Femenía-Font et al., Citation2005; Schuetz et al., Citation2006). These results were in agreement with Gratieri & Kalia (Citation2013), who found that ketorolac electrotransport was dependent on current density. Also, Calatayud-Pascul et al. (Citation2011) concluded that the application of a current density of 0.5 mA/cm2 produces a statistically significant increment of almotriptan delivery not only with respect to a passive control but also to a density of 0.25 mA/cm2.
Concentration of drug is one of the most important factors affecting iontophoretic process. The effect of the concentration has been studied on a number of drugs. An increase in concentration was shown to increase the apparent steady-state flux of a number of drugs, e.g. metoprolol, butyrate, diclofenac sodium, rotigotine, atenolol and ketorolac. All these drugs showed a proportional increase in flux with an increase in concentration (Rawat et al., Citation2008).
The significant decrease in the permeation of vancomycin hydrochloride from cathodal iontophoresis of ethosomal formulae in the presence of 0.9% normal saline was attributed to the competition between the small and mobile chloride ions and negatively charged ethosomes vesicles bearing the drug leading to reduce drug flux through the skin (Kalia et al., Citation2004).
The in vivo study results indicated that iontophoretic delivery of vancomycin from ethosomal formula had succeeded in delivering the drug in concentration above the MIC of infected pathogen for a duration sufficient to induce the obtained reduction in bacterial count, also the combination of iontophoresis with ethosomes achieved a comparable results to parental delivery of vancomycin.
Conclusion
The present study aimed at designing a system capable of delivering vancomycin hydrochloride transdermally, by a combination ethosomal formulae and iontophoresis. Cathodal iontophoresis of negatively charged formulae showed enhanced permeation when compared with anodal iontophoresis of positively charged ethosomes, this may be attributed to shielding effect of SA. Transdermal flux was affected by current mode, density, drug concentration and drug solvent. In vivo study indicated that iontophoretic delivery of vancomycin from ethosomal formula succeeded in delivering drug transdermally in a magnitude above the MIC of infected pathogen. These results represent the combination between nano vesicles and iontophoresis technique as promising solution in delivering high molecular weight drug transdermally.
Declaration of interest
The authors report no conflicts of interest. The authors alone are responsible for the content and writing of this article.
References
- Calatayud-Pascual MAB-FC, Serna-Jiménez CE, Del Rio-Sancho S, et al. (2011). Effect of iontophoresis on in vitro transdermal absorption of almotriptan. Int J Pharm 416:189–94
- Chakraborty SP, Sahub SK, Pramanikb P, Roya S. (2012). In vitro antimicrobial activity of nanoconjugated vancomycin against drug resistant Staphylococcus aureus. Int J Pharm 436:659–76
- Fang JY, Sung KC, Lin HH, Fang CL. (1999). Transdermal iontophoretic delivery of enoxacin from various liposome-encapsulated formulations. J Control Release 60:1–10
- Femenía-Font A, Balaguer-Fernández C, Merino V, López-Castellano A. (2005). Iontophoretic transdermal delivery of sumatriptan: effect of current density anionic strength. J Pharm Sci 94:2183–6
- Gratieri T, Kalia YN. (2013). Mathematical models to describe iontophoretic transport in vitro and in vivo and the effect of current application on the skin barrier. Adv Drug Deliv Rev 65:315–29
- Gu H, Ho PL, Tong E, et al. (2003). Presenting vancomycin on nanoparticles to enhance antimicrobial activities. Nano Lett 3:1261–3
- Guy RH, Delgado-Charro MB, Kalia YN. (2001). Iontophoretic transport across the skin. Skin Pharmacol Appl Skin Physiol 14:35–40
- Kajimoto K, Yamamoto M, Watanabe M, et al. (2011). Noninvasive and persistent transfollicular drug delivery system using a combination of liposomes and iontophoresis. Int J Pharm 403:57–65
- Kalia Y, Naik A, Garrison J, Guy RH. (2004). Iontophoretic drug delivery. Adv Drug Deliv Rev 56:619–58
- Kirjavainen M, Urtti A, Mönkkönen J, Hirvonen J. (2000). Influence of lipids on the mannitol flux during transdermal iontophoresis in vitro. Eur J Pharm Sci 10:97–102
- Kumar V, Banga AK. (2012). Modulated iontophoretic delivery of small and large molecules through microchannels. Int J Pharm 434:106–14
- Li GL, Van der Geest R, Chanet L, et al. (2002). In vitro iontophoresis of R-apomorphine across human stratum corneum: structure-transport relationship of penetration enhancement. J Control Release 84:49–57
- Lin MY, Hayden MK. (2010). Methicillin-resistant Staphylococcus aureus and vancomycin-resistant enterococcus: recognition and prevention in intensive care units. Crit Care Med 38:S335–44
- Liu B, Zuo J, Du L, et al. (2014). Transdermal enhancement effect and mechanism of iontophoresis for non-steroidal anti-inflammatory drugs. Int J Pharm 466:76–82
- Mohammed MI, Makky AMA, Abdellatif MM. (2014). Formulation and characterization of ethosomes bearing vancomycin hydrochloride for transdermal delivery. Int J Pharm Pharm Sci 6:190–4
- Muppidi K, Pumerantz AS, Wang J, Betageri G. (2012). Development and stability studies of novel liposomal vancomycin formulations. ISRN Pharm 2:1–8
- Ozcan AV, Demir M, Onem G. (2006). Topical versus systemic vancomycin for deep sternal wound infection caused by methicillin-resistant Staphylococcus aureus in a rodent experimental model. Tex Heart Inst J 33:107
- Prasad R, Koul V, Anand S, Khar RK. (2007). Effect of DC/mDC iontophoresis and terpenes on transdermal permeation of methotrexate: in vitro study. Int J Pharm 333:70–8
- Prasanthi D, Lakshmi PK. (2012). Vesicles mechanism of transdermal permeation: a review. Asian J Pharm Clin Res 5:18–25
- Prausnitz MR, Langer R. (2008). Transdermal drug delivery. Nat Biotechnol 26:1261–8
- Rawat S, Vengurlekar S, Rakesh B, et al. (2008). Transdermal delivery by iontophoresis. Indian J Pharm Sci 70:5–10
- Sarvaiya JI, Kapse GK, Tank CJ. (2013). Iontophoresis of micellar composition of Lovastatin: study of affecting factors and in-vitro permeation. J Pharmacy Res 7:327–30
- Schuetz YB, Carrupt PA, Naik A, et al. (2006). Structure-permeation relationships for the non-invasive transdermal delivery of cationic peptides by iontophoresis. Eur J Pharm Sci 29:53–9
- Tomoda K, Terashima H, Suzukid K, et al. (2012). Enhanced transdermal delivery of indomethacin using combination of PLGA nanoparticles and iontophoresis in vivo. Colloids Surf B Biointerfaces 92:50–4
- Wilhelm C, Winterhalter M, Zimmermann U, Benz R. (1993). Kinetics of pore size during irreversible electrical breakdown of lipid bilayer membranes. Biophys J 64:121–8
- Winterhalter M. (1996). Liposomes in electric fields. In: Barenholz Y, Lasic D, eds. Handbook of nonmedical applications of liposomes. London: CRC Press, 285–307
- Wu C, Jin Y, Bao JL. (2004). Effects of on/off ratio and frequency on the iontophoresis of diclofenac sodium gel in vitro. Chin Pharm J 39:359–61
- Zakeri-Milani P, Loveymib BD, Jelvehgarid M, Valizadehe H. (2013). The characteristics and improved intestinal permeability of vancomycin PLGA-nanoparticles as colloidal drug delivery system. Colloids Surf B Biointerfaces 103:174–81