Abstract
Purpose: Lymph cancers are heterogeneous malignancies of the hematopoietic and lymphoid tissues. Doxorubicin (DOX) and vincristine (VCR) are commonly used anti-cancer chemotherapeutic drugs, but their clinical uses are associated with dose-limiting systemic toxicity.
Methods: In the present study, DOX and VCR were encapsulated into nanostructured lipid carriers (NLCs) and used them to treat B-cell lymphoma cells through the targeted delivery of DOX and VCR to lymph cancer animal model.
Results: DOX and VCR encapsulated NLCs (DOX/VCR NLCs) demonstrated controlled drug release under physiological conditions. In addition, DOX/VCR NLCs exhibited the highest cytotoxicity and synergistic effect of two drugs in B-cell lymphoma cells and the best anti-tumor effect in vivo.
Conclusion: DOX/VCR NLCs were proved to be more efficacious than the equivalent dose of free DOX and single drug (DOX or VCR) formulation in vitro and in vivo, and significantly reduced the drug-associated systemic toxicity.
Introduction
Approximately 70 000 people are diagnosed with B-cell lymphomas in the USA alone (Chen et al., Citation2010). Ninety percent are B-cell lymphomas, which cause an estimated 18 940 annual deaths (Yang et al., Citation2013). Diffuse large B-cell lymphoma (DLBCL) is the most frequently diagnosed lymphoid tumor, accounting for 40% of all non-Hodgkin lymphomas among adults in Western countries (Wang et al., Citation2015). DLBCL is also common in developing countries (Sabattini et al., Citation2010). Although traditional chemical therapies and bone marrow transplantations can increase survival rates and even cure certain patients, relapse and drug resistance remain a clinical challenge (Alzouebi et al., Citation2012). In order to enhance therapeutic efficacies and reduce adverse effects, novel therapeutics with alternative mechanisms of B-cell killing are needed (Castillo et al., Citation2008).
Nanomedicine is an emerging field that has shown great promise for the development of novel diagnostic, imaging and therapeutic agents for a variety of diseases, including tumor (Xiao et al., Citation2011). To limit some of the side effects associated with many chemotherapeutics agents such as doxorubicin (DOX) and vincristine (VCR), several nanocarrier systems have been developed (Davis & Farag, Citation2013; Wang et al., Citation2014; Cabeza et al., Citation2015; Li et al., Citation2015). These nanomaterials include lipid nanoparticles (Thakkar et al., Citation2015), liposomes (Hashimoto et al., Citation2015), polymeric nanoparticles (Prabhu et al., Citation2015), dendrimers (Setaro et al., Citation2015) and so on. It is believed that such nanocarriers will decrease the toxicity to normal organs, prolong the circulation time, slow down the metabolism of the drug and facilitate the delivery of the drug to the tumor sites via the enhanced permeability and retention effect (Keereweer et al., Citation2012).
It has been reported that lipid nanoparticles improve the drug absorption and bioavailability due to their diameter and absorption enhancing effect of lipids (Hejri et al., Citation2013). Nanostructured lipid carriers (NLCs) represent an improved generation of lipid nanoparticles (Yuan et al., Citation2013). They are advised to be the second lifetime of lipid nanocarriers. Contrasted to SLN and other lipid carriers, NLCs show a higher loading capability by blending a fluid lipid with the solid lipid, a higher element drug stacking can be achieved and a lower likelihood of drug expulsion during storage (Han et al., Citation2015).
A combination therapy with two or more chemotherapeutics for refractory cancers is a successful strategy for synergistic effects, lower toxicity and drug resistance (Zanotto-Filho et al., Citation2015). DOX and VCR are commonly used anti-B-cell lymphoma chemotherapeutic drugs, but their clinical uses are associated with dose-limiting systemic toxicity. In the present study, DOX and VCR were encapsulated into NLCs and used them to treat B-cell lymphoma cells through the targeted co-delivery of DOX and VCR. This system was expected to enhance synergistic effects for B-cell lymphoma, bring about a new combination therapy strategy for the lymph cancer.
Materials and methods
Materials
VCR was purchased from Shaanxi Pioneer Biotech Co., Ltd. (Xi’an, China). Doxorubicin hydrochloride (DOX·HCl) was supplied by Dalian Meilun Biology Technology Co., Ltd. (Dalian, China). COMPRITOL®888 ATO was generously provided by Gattefossé USA (Paramus, NJ). Polyoxyl castor oil (Cremophor ELP) was donated by BASF (Ludwigshafen, Germany). Didecyldimethylammonium bromide (DDAB) was purchased from Ziyi Reagent Factory (Shanghai, China). The human DLBCL cells (LY1 cells) were obtained from the American type culture collection (Manassas, VA). All the solvents used were of analytical reagent grade.
Animals
BALB/c mice (6–8 weeks old, 18–22 g weight) were purchased from SLAC Laboratory Animal Co., Ltd. (Shanghai, China). All animal experiments complied with the Animal Management Rules of the Ministry of Health of the People’s Republic of China
DOX/VCR NLCs construction
Prior to the NLCs preparation, the DOX·HCl was stirred with twice the molar amount of TEA in DMSO for 12 h to obtain lipophilic DOX base (Han et al., Citation2014). COMPRITOL® 888 ATO Stearic acid, Cremophor ELP, DOX base and VCR (DOX versus VCR = 1:1, w/w) were melting and dissolving at 65 °C as the lipid phase. A cold aqueous phase was prepared by dissolving DDAB in 50 mL distilled water. The lipid phase was rapidly injected into the aqueous phase. DOX/VCR NLCs were harvested by stirring in an ice bath for another 10 min. DMSO was removed by the dialyzed method. The redundant stabilizers were removed by centrifugation at 1000 g, 4 °C for 30 min. The pellet was vortexed and resuspended in Milli-Q water, washed three times, filtered through a 0.45-μm membrane. The obtained NLCs were stored at 2–8 °C.
Single DOX or VCR containing NLCs (DOX NLCs or VCR NLCs) or blank NLCs were prepared by the same method described earlier.
NLCs characterization
The particle size and zeta potential of different NLCs were measured using a Zetasizer Nano-ZS (Malvern Instruments, Worcestershire, UK). The entrapment efficiency (EE) of DOX (EEDOX) in NLCs was determined by high performance liquid chromatography (HPLC, Agilent 1100, Santa Clara, CA) with a Zorbax SB-C18 column (150 mm× 4.6 mm, 5 μm). The mobile phase consisted in a mixture of 0.05 mol/L potassium dihydrogen phosphate solution (pH adjusted to 7.5) with acetonitrile (70:30 v/v). The flow rate was maintained at 1.0 mL/min. The effluents were monitored at λEx = 480 nm and λEm = 560 nm.
The EE of VCR (EEVCR) in NLCs was determined by inductively coupled plasma optical emission spectrometry (ICP-OES). The detection was carried out at λ = 275 nm.
EE was calculated by the following equation:
NLCs release study
DOX/VCR NLCs and other kinds of NLCs were added into dialysis bags (Shao et al., Citation2015). Then, the dialysis bags were incubated with 20 mL release medium at 37 °C with stirring at 100 rpm. At predetermined time intervals, the NLCs suspensions were centrifuged and the amount of drug released in the supernatant was analyzed. The amount of DOX was measured by using HPLC, and the DDP content was determined by ICP-OES mentioned in the section “DOX/VCR NLCs construction”.
Cytotoxicity
The cytotoxicity of DOX/VCR NLCs with the different DOX to VCR ratios (5/1; 2/1; 1/1; 1/2; 1/5; w/w), DOX NLCs, VCR NLCs, DOX solution and VCR solution were evaluated by MTT assay (Namvar et al., Citation2015). The LY1 cells were grown in the RPMI-1640 medium supplemented with 10% fetal bovine serum at 37 °C in 5% CO2 humidified atmosphere. The confluent monolayer cells were harvested and seeded into microplates for 24 h in the incubator for cell attachment and cell recovery after trypsinization before subjected to NLCs treatment and incubation for 48 h. Then, MTT solution (5 mg/mL) was added to each well and cells were incubated for 4 h. Two hundred microliters DMSO was added to each well to dissolve the MTT formazan crystals. The optical density (OD) of formazan product was measured at 570 nm. Cells without the addition of MTT reagents were used as a blank control. Cell viability was expressed as the percentage of MTT counts of treated cells relative to those without MTT treatment. The IC50 was the concentration that caused 50% inhibition of cell viability and was calculated.
In vivo anti-tumor efficacy
LY1 cells were inoculated into the right armpit of BALB/c mice. When tumor volume reached 90–110 mm3, transplanted mice were randomly divided into six groups (six per group) separately.
Mice were injected intravenously every 3 days (d) with DOX/VCR NLCs, DOX NLCs, VCR NLCs, DOX solution, VCR solution and 0.9% saline as control. Twenty-one days later, all the mice were sacrificed by cervical dislocation and the tumor tissue samples were taken out.
Tumor size was measured by a digital caliper every 3 d. Tumor volume (mm3) was calculated by V = L × W2/2, where L and W are the longest and shortest diameter of tumors.
The weight of each mouse was measured every 3 d after injection to determine the body weight lost of different groups.
Statistical analysis
Results were presented as mean ± SD. Statistical comparisons were made by Student’s t-test or ANOVA analysis. The accepted level of significance was p < 0.05.
Results and discussion
NLCs characterization
The size of DOX/VCR NLCs was 96 nm. Blank NLCs was 94 nm. These data illustrated that the loading of drugs did not increase the size significantly. It has been reported that the nanoparticles with the size less than 200 nm can significantly accumulate in tumor by “filtration” mechanism (Xu et al., Citation2009; Duan et al., Citation2015). Small particles are also minimal endocytosis by macrophages, so destruction and clearance could be minimized, and the nanocarriers were desirable for in vitro and in vivo drug delivery (Accardo et al., Citation2012; Wang et al., Citation2013). The zeta potentials of DOX/VCR NLCs were +21 mV. The cationic property the NLCs is due to the DDAB as surfactant used during preparation. Positive charge of the nanocarriers could exploit the negative charged cells due to the electrostatic interactions, thus increase the cellular uptake (Xu et al., Citation2012; Vighi et al., Citation2012). The EE of DOX and VCR loaded in DOX/VCR NLCs and single drug NLCs were above 80%. High EE could guarantee the therapeutic efficiency of the nanomedicine ().
Table 1. NLCs characterization.
NLCs release study
DOX and VCR release profiles of DOX/VCR NLCs and single drug NLCs are shown in and . NLCs showed the sustained-release behavior of both drugs. We consider over 80% of accumulated releases as the complete release of drugs. DOX releases were completed at 16 h, while VCR release was slower, at 48 h. The sustained-release of drugs from NLCs can continuously contribute anti-tumor effect for relatively long period of time (Momoh et al., Citation2013; Wang & Xia, Citation2014). This may help with the anti-tumor progress.
DOX to VCR ratio determination in DOX/VCR NLCs
To determine the best DOX to VCR ratio of the DOX and VCR loaded in NLCs, the best synergy effect of these two drugs in the formulations must be achieved. The cytotoxicity was evaluated by MTT assay in LY1 cells, and the IC50 values of DOX/VCR NLCs with the different DOX to VCR ratios (5/1; 2/1; 1/1; 1/2; 1/5; w/w) are summarized in .
Table 2. DOX to VCR ratio determination in DOX/VCR NLCs.
The DOX/VCR NLCs showed the lowest IC50 (0.26) when DOX to VCR ratio was 1/1, showing the best synergism effect. It could be concluded that the synergistic effect of DOX and VCR contained in NLCs at the weight ratio of 1/1 was the best formulation in the present study.
Cytotoxicity assays
In vitro cytotoxicity of DOX/VCR NLCs, DOX NLCs, VCR NLCs, DOX solution and VCR solution was evaluated by MTT assay in LY1 cells. shows the IC50 values of drugs solutions and NLCs formulations in LY1 lymph cancer cells. The IC50 values of DOX and VCR solution were 7.51 and 9.82 μg/mL, respectively. The IC50 values of DOX and VCR NLCs were 2.63 and 3.31, respectively. These results were threefold dose advanced than the solutions. This can contribute to the advantage of NLCs that improved the drug bioavailability. DOX/VCR NLCs has the IC50 of 0.26/0.26 of the two drugs. More than 10-fold of IC50 values of DOX/VCR NLCs over DOX NLCs and VCR NLCs in reducing viability of lymph cancer cells was observed, accounting for the highest anti-tumor activity and exhibited the significantly synergy effect.
Table 3. DOX to VCR ratio determination in DOX/VCR NLCs.
In vivo anti-tumor efficacy
According to the results of in vitro cytotoxicity assays, the in vivo anti-tumor studies of different formulations were applied in mice bearing LY1 cells lymph cancer model. shows the tumor growth curves. The results could be summarized as follows: On the one hand, NLCs formulations showed better efficacy than free drug solutions (p < 0.05); on the other hand, tumor growth was more significantly inhibited by DOX/VCR NLCs than other NLCs groups (p < 0.05). The most obviously tumor regressions were observed in the double drug containing NLCs group, showing the synergistic effect and the excellent delivery ability of the novel NLCs.
Figure 3. Tumor volume changes after treatment with different formulations in mice bearing lymph cancer xenograft.
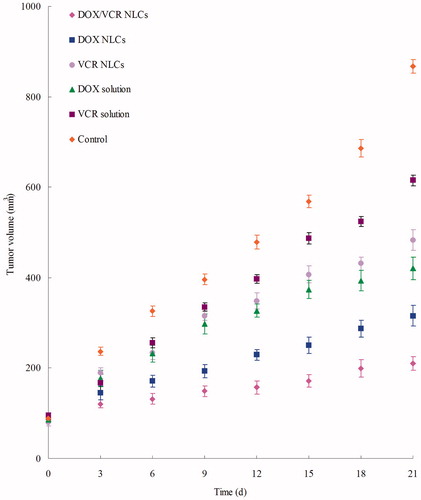
Systemic toxicity during the cancer treatment is a severe side effect. Changes in the body weight of tumor-bearing mice after injections can indicate the systemic toxicity. The body weights of DOX/VCR NLCs, DOX NLCs and VCR NLCs groups slightly increased, which might due to the low toxicity of NLCs and slight increase of the tumor weight (). Noticeable body weight losses were observed in DOX solution, VCR solution and saline control groups. Also, reduction in food intake, energy sag and inactive in moving were observed in these three groups during the test. The results demonstrated the reduced systemic toxicity in the NLCs formulations.
Conclusions
DOX/VCR NLCs exhibited the highest anti-tumor activity and the significantly synergy anti-lymph cancer effect in vitro and in vivo. This system could achieve targeted co-delivery of DOX and VCR, overcome the drug resistance of the single drug preparation and reduce the systemic toxicity. Also, NLCs formulation could target the lymph cancer site due to its small particle size and positive surface charge. The resulting nanomedicine could function as a promising targeted delivery system for the treatment of lymph cancers.
Declaration of interest
The authors report no conflicts of interest. The authors alone are responsible for the content and writing of this article.
References
- Accardo A, Salsano G, Morisco A, et al. (2012). Peptide-modified liposomes for selective targeting of bombesin receptors overexpressed by cancer cells: a potential theranostic agent. Int J Nanomedicine 7:2007–17
- Alzouebi M, Goepel JR, Horsman JM, Hancock BW. (2012). Primary thyroid lymphoma: the 40 year experience of a UK lymphoma treatment centre. Int J Oncol 40:2075–80
- Cabeza L, Ortiz R, Arias JL, et al. (2015). Enhanced antitumor activity of doxorubicin in breast cancer through the use of poly(butylcyanoacrylate) nanoparticles. Int J Nanomedicine 10:1291–306
- Castillo J, Winer E, Quesenberry P. (2008). Newer monoclonal antibodies for hematological malignancies. Exp Hematol 36:755–68
- Chen WC, Completo GC, Sigal DS, et al. (2010). In vivo targeting of B-cell lymphoma with glycan ligands of CD22. Blood 115:4778–86
- Davis T, Farag SS. (2013). Treating relapsed or refractory Philadelphia chromosome-negative acute lymphoblastic leukemia: liposome-encapsulated vincristine. Int J Nanomedicine 8:3479–88
- Duan Y, Wang J, Yang X, et al. (2015). Curcumin-loaded mixed micelles: preparation, optimization, physicochemical properties and cytotoxicity in vitro. Drug Deliv 22:50–7
- Han Y, Li Y, Zhang P, et al. (2015). Nanostructured lipid carriers as novel drug delivery system for lung cancer gene therapy. Pharm Dev Technol . [Epub ahead of print]. doi: 10.3109/10837450.2014.996900
- Han Y, Zhang Y, Li D, et al. (2014). Transferrin-modified nanostructured lipid carriers as multifunctional nanomedicine for codelivery of DNA and doxorubicin. Int J Nanomedicine 9:4107–16
- Hashimoto Y, Shimizu T, Abu Lila AS, et al. (2015). Relationship between the Concentration of anti-polyethylene glycol (PEG) immunoglobulin M (IgM) and the intensity of the accelerated blood clearance (ABC) phenomenon against PEGylated liposomes in mice. Biol Pharm Bull 38:417–24
- Hejri A, Khosravi A, Gharanjig K, Hejazi M. (2013). Optimisation of the formulation of β-carotene loaded nanostructured lipid carriers prepared by solvent diffusion method. Food Chem 141:117–23
- Keereweer S, Mol IM, Kerrebijn JD, et al. (2012). Targeting integrins and enhanced permeability and retention (EPR) effect for optical imaging of oral cancer. J Surg Oncol 105:714–18
- Li M, Tang Z, Zhang D, et al. (2015). Doxorubicin-loaded polysaccharide nanoparticles suppress the growth of murine colorectal carcinoma and inhibit the metastasis of murine mammary carcinoma in rodent models. Biomaterials 51:161–72
- Momoh MA, Kenechukwu FC, Attama AA. (2013). Formulation and evaluation of novel solid lipid microparticles as a sustained release system for the delivery of metformin hydrochloride. Drug Deliv 20:102–11
- Namvar F, Rahman HS, Mohamad R, et al. (2015). Cytotoxic effects of biosynthesized zinc oxide nanoparticles on murine cell lines. Evid Based Complement Alternat Med 2015:593014
- Prabhu RH, Patravale VB, Joshi MD. (2015). Polymeric nanoparticles for targeted treatment in oncology: current insights. Int J Nanomedicine 10:1001–18
- Sabattini E, Bacci F, Sagramoso C, Pileri SA. (2010). WHO classification of tumours of haematopoietic and lymphoid tissues in 2008: an overview. Pathologica 102:83–7
- Setaro F, Brasch M, Hahn U, et al. (2015). Generation-dependent templated self-assembly of biohybrid protein nanoparticles around photosensitizer dendrimers. Nano Lett 15:1245–51
- Shao Z, Shao J, Tan B, et al. (2015). Targeted lung cancer therapy: preparation and optimization of transferrin-decorated nanostructured lipid carriers as novel nanomedicine for co-delivery of anticancer drugs and DNA. Int J Nanomedicine 10:1223–33
- Thakkar A, Chenreddy S, Wang J, Prabhu S. (2015). Evaluation of ibuprofen loaded solid lipid nanoparticles and its combination regimens for pancreatic cancer chemoprevention. Int J Oncol 46:1827–34
- Vighi E, Montanari M, Ruozi B, et al. (2012). The role of protamine amount in the transfection performance of cationic SLN designed as a gene nanocarrier. Drug Deliv 19:1–10
- Wang J, Jia J, Liu J, et al. (2013). Tumor targeting effects of a novel modified paclitaxel-loaded discoidal mimic high density lipoproteins. Drug Deliv 20:356–63
- Wang J, Xia Q. (2014). Alpha-lipoic acid-loaded nanostructured lipid carrier: sustained release and biocompatibility to HaCaT cells in vitro. Drug Deliv 21:328–41
- Wang L, Li X, Liu X, et al. (2015). Enhancing effects of indirubin on the arsenic disulfide-induced apoptosis of human diffuse large B-cell lymphoma cells. Oncol Lett 9:1940–6
- Wang Y, Dou L, He H, et al. (2014). Multifunctional nanoparticles as nanocarrier for vincristine sulfate delivery to overcome tumor multidrug resistance. Mol Pharm 11:885–94
- Xiao K, Luo J, Li Y, et al. (2011). PEG-oligocholic acid telodendrimer micelles for the targeted delivery of doxorubicin to B-cell lymphoma. J Control Release 155:272–81
- Xu W, Cui Y, Ling P, Li LB. (2012). Preparation and evaluation of folate-modified cationic pluronic micelles for poorly soluble anticancer drug. Drug Deliv 19:208–19
- Xu Z, Chen L, Gu W, et al. (2009). The performance of docetaxel-loaded solid lipid nanoparticles targeted to hepatocellular carcinoma. Biomaterials 30:226–32
- Yang S, Damiano MG, Zhang H, et al. (2013). Biomimetic, synthetic HDL nanostructures for lymphoma. Proc Natl Acad Sci USA 110:2511–16
- Yuan L, Liu C, Chen Y, et al. (2013). Antitumor activity of tripterine via cell-penetrating peptide-coated nanostructured lipid carriers in a prostate cancer model. Int J Nanomedicine 8:4339–50
- Zanotto-Filho A, Braganhol E, Klafke K, et al. (2015). Autophagy inhibition improves the efficacy of curcumin/temozolomide combination therapy in glioblastomas. Cancer Lett 358:220–31