Abstract
Hepatocarcinoma animal models, such as the induced tumor model, transplanted tumor model, gene animal model, are significant experimental tools for the evaluation of targeting drug delivery system as well as the pre-clinical studies of liver cancer. The application of antitumor drug-targeting models not only furnishes similar biological characteristics to human liver cancer but also offers guarantee of pharmacokinetic indicators of the liver-targeting preparations. In this article, we have reviewed some kinds of antitumor drug-targeting models of hepatoma and speculated that the research on this field would be capable of attaining a deeper level and expecting a superior achievement in the future.
Introduction
With the development of bioscience, biomaterials and molecular biology, the investigations of targeting drug delivery system (TDDS) have been turned into research focus. Among them, great importance should be attached to the research of TDDS in the application of anti-cancer area (Nasongkla et al., Citation2006; Liu et al., Citation2007; Peer et al., Citation2007; Brannon-Peppas et al., Citation2012). In the traditional treatment of cancer by chemotherapy drugs, there are various adverse effects such as the tendency of in-vivo drug degradation and diffusion after regional administration, which could cause a relatively average tissue distribution and a certain amount of damage on the entire body (Murali et al., Citation2015). Furthermore, free drug could not form an effective antitumor drug concentration in the tumor region. On the contrary, the TDDS refers to the drug carrier that selectively concentrates on the medicine target area (Sudimack & Lee, Citation2000), which could effectively deliver antitumor drugs to the target site and simultaneously reduce the dosage as well as the possibility of side effects. Especially in liver cancer therapy, preliminary studies have suggested that the use of targeting agents possess many desirable properties, such as good specificity and selectivity, the capacity of reducing the drug dosage and frequency, as well as decreasing the cell toxicity and improving the drug efficacy (Seymour et al., Citation2002; Brillet et al., Citation2005).
In recent decades, valid approaches have been exploiting for treating cancer and the rapid development of targeting agents has brought a breeze of fresh air in the tumor therapy (Semenza, Citation2003; Thorpe, Citation2004; Weigelt et al., Citation2010; Gao et al., Citation2014). During the study of liver-targeting agents, animal models supply significant methods on the evaluation of the anti-hepatoma TDDS (Ko et al., Citation2001; Qian et al., Citation2007; Kota et al., Citation2009). The study of animal models of liver cancer provides a powerful means of the pharmaco-dynamics and pharmacokinetics experiments of targeting agents (Ho & Chien, Citation2014).
Development of anti-tumor models
About 90% amount of cancer pre-clinical studies are by the assistance of a variety of animal models (Kapadia et al., Citation1998; Kaplan-Lefko et al., Citation2003; Van der Spoel et al., Citation2011), including the spontaneous tumor models (Rosol et al., Citation2003), induced tumor models (Talmadge et al., Citation2007), transplanted tumor models (Peterson et al., Citation1994), gene animal models, and so on (Van Dyke & Jacks, Citation2002; Khanna & Hunter, Citation2005).
Tumor animal models originally appeared in the 18th century, when researchers established homologous transplantation models by transplanted homograft tumor cells into animals and first performed the artificial manipulation of tumor (Waxman & Anderson, Citation2001). This appearance marked the onset of the experimental oncology and made up the defects of spontaneous tumor models, namely, it was difficult to collect abundant research materials on oncology in a very short time due to different incidences of tumors (Babbs, Citation1990). The study of induced tumor models made it possible to better understand the etiology of tumor. In 1915, Japanese scientist Katsusaburo Yamagiwa continuously painted crude coal tar on the inner surface of 137 rabbits’ ears in every 2 days; 1 year later, seven rabbits appeared invasive tumors on the tar-covered region (Fujiki, Citation2014). This is the first time that human had ever produced cancer successfully metastasis was rarely happened even been born the subcutaneous tumor for a long period. Subsequently, various sorts of chemical substances were used to establish induced tumor models, meanwhile, lay the foundation of chemical carcinogenesis theory (Mahfoozur et al., Citation2015).
In 1969, Rygaard & Povlsen successfully transplanted xenograft human malignant tumor cells into nude mice, discovering that the biological characteristics of the created animal models accorded better with the human body. Until today, using human cells or primary tumor tissues to produce xenograft models have become the main method of current in-vivo tumor research (Rygaard & Povlsen, Citation1969).
In 1984, Brinster & Palmiter first transduced SV40’s T antigen (Tag) oncogene into mice fertilized eggs, resulting the transgenic mice be enabled to form brain choroid plexus papilloma, which turned to be the first genetically engineered mouse tumor model (Brinster & Palmiter, Citation1984). Thereafter, the technology of gene knock-out and gene insertion was also popularly applied to cancer researches. Genetically engineered animal models not only display a revolutionary position in fundamental researches, such as the function of genes, the synergies between genes, the mechanism of tumor formation and the law of tumor development, but also play an innovation role in practical researches like the prevention and treatment of cancer (Hansen & Khanna, Citation2004; Sharpless & DePinho, Citation2006). At the end of 1990s, the successful construction of bcr-abl gene-deficient mice prompted the birth of the first molecularly targeting drug, imatinib (Zheng et al., Citation2008; De Carvalho et al., Citation2010); and then, an increasing number of molecular-targeting agents was approved and available after the year of 2000 (Tsuruo et al., Citation2003; Huang et al., Citation2004; Ferrari et al., Citation2005; Weinstein & Joe, Citation2006).
At present, the studies of tumor animal models have been improved and progressed constantly. As can be seen from the development law of tumor animal models during the past years, the purpose of the studies focus more on the clinical research, the content has been changed from mechanism to application, the methodology has been turned from passive observation into active manipulation, and the practical applications were grown stronger.
Targeting models of liver cancer
Induced animal models of liver cancer
Induced liver cancer model refers to that, under experimental conditions, using chemical, physical or biological carcinogens onto animals to induce liver canceration (Qian et al., Citation2007), which is a frequently used method in experimental oncology research (Kim et al., Citation1991; Dawson et al., Citation2002). Of which, chemical carcinogens are the most common, such as nitrosamines (DEN) (Williams, Citation1980), aflatoxins (AFB1) (Ghoshal & Farber, Citation1984), amino azo fuels (3'-Me-DAB), aromatic amines (2-AAF) and so on (Naugler et al., Citation2007). The induction can be completed by a single sort of chemical drugs or by combinations of multiple drugs with different tumor promoters and liver resections in a certain sequence to form diverse comprehensive-induced liver cancer schemes (Fisher et al., Citation1978; Sun, Citation1990).
As far as the present state of study, this sort of model is mainly applied for the research of the etiology, embryology, pathogenesis and the genetic and biological characteristics of liver cancer (Toyokuni, Citation1999). Silveira et al. (Citation2001) constantly gave DEN (200 mg/kg) to the male Wistar rats (50–60 g) by gavage for 2 weeks, and then switched to 2-AFF dissolved in dimethyl sulfoxide and corn oil at a dose of 20 mg/kg for 4 days. After 24 h of the last feed of 2-AFF, 70% liver was resected, then continuously gave 2-AFF 20 mg/kg for 2–4 days. 10 months later, the animal model was established successfully.
Current studies of this kind of animal model remain inconsistent perspectives of the choice of mice gender (Antonio et al., Citation2015). It is generally believed that, compared to female mice, male mice have higher success rate in cancer induction (Nakatani et al., Citation2001). However, others insist that the choice should be female mice (Ornstein et al., Citation2000). The features of induced liver cancer models denominate the similarities in terms of characteristics and processes of human liver cancer (Gerin, Citation1990; Yang et al., Citation2014). Generally, the whole carcinogenesis process is divided into four stages: the activation of liver cells, the proliferation of liver cells, the hyperplasia nodules of liver cells and the hepatocellular carcinoma (Sell & Leffert, Citation1982). In addition, such model also showed its defects in practice including long inducing period (3–5 months, even 1–2 years) and individual differences in aspects of time, location, lesions number of the occurrence of hepatoma.
The induced animal models of liver cancer have been used in the research of targeting agents. Uehara et al. (Citation2002) allowed Wistar rats fed with 0.01% DEN for 12 weeks and then started to give liposomes OK-432 (OK-lipo) and non-liposomal (Non-OK) at the 5th and 9th week, respectively. At the 13th week, five rats were treated per group to compare the immune parameters (NK and IFN-γ), while the remaining rats were given water to observe their survival time. Results indicated that the two groups given OK-lipo were superior to the Non-OK group in life span and the immune biochemistry aspects ().
Homograft animal models of liver cancer
Transplanted hepatic cancer model implies that this type of tumor strains is transplantable, serially grow and passage in inbred animals and the same species, possesses more stable histological types and growth characteristics. Besides, the invasion, metastasis and the sensitivity to chemotherapeutic drugs of such model have not been identified yet (Feray et al., 1990). The features of transplanted liver cancer models refer to the simple model reproduction, the conform tumor’s growth rate, the tiny individual differences, the similar effects on the host and the high-survival rate of inoculation, and it is easier to estimate the curative efficacy of targeting agents objectively; in addition, tumor cell lines could be saved in liquid nitrogen for long-term experimental usage (Freise et al., Citation1999). Nevertheless, the shortcomings of transplanted models mainly represent the differences with human tumors on growth characteristics, such as the higher growth speed and proliferation rate, but shorter volume doubling time than human cancer cells. In addition, there is no single tumor strain that is sensitive to all anticancer drugs (Sakai et al., Citation2005). Generally, the transplanted liver cancer models are irreplaceable in the research of applications of targeting preparations.
At present, the H22 tumor model is the most commonly used mice transplanted liver model (Yao et al., Citation2006; Bishayee et al., Citation2010). This model has been generally utilized in the screening of liver-targeting agents, and also has been a well-acknowledged model in the study of the curative effect and mechanism of anti-tumor traditional Chinese medicine (Wiesner et al., Citation2001). The transplantation of H22 hepatoma cells could be operated in three ways: the ascites passage, hypodermic inoculation and the orthotopic liver transplantation. These three methods have been applied to the screening of the antitumor-targeting agents of hepatoma (Tian et al., Citation2012). According to previous reports (Tardi et al., Citation2000; Peer & Margalit, Citation2004), Yuan et al. adjusted the concentration of H22 cell to 1 × 107/mL, given each BALB/c mouse 0.1 mL of the cell suspension by intraperitoneal injection to establish ascites model, then administrated respectively and observed the changes of survival time, body weight and peritoneal permeability of mice. Results showed that 100 mg/kg of quercetin nanoliposomes could prolonged the survival time of liver cancer mice, decreased the peritoneal permeability, inhibited the carcinomatous ascites and accumulated in tumor tissues effectively; hence, it was expected to be a kind of promising antitumor drug (Ren et al., Citation2003).
As for the sites where liver tumor transplanted, there are still no unified comprehensions. The intrahepatic tumor reflects the malignant behaviors and the growing status of human primary infiltration (Yang et al., Citation1992), and the transplanted tumor obtained shows much closer biological characteristics to the clinical liver carcinoma (Yao et al., Citation2001). Moreover, neither the subcutaneous transplanted tumor nor the ascites hepatoma could possess the above-mentioned properties (Makino, Citation1956).
The reported experimental results have demonstrated that the metastasis was rarely happened even been born the subcutaneous tumor for a long period (more than 70 days), mainly due to the invasion and low-metastasis rate caused by the poor blood supply and the lymphatic drainage inside the tumor (Wu et al., Citation2013). The blood supply condition is a key factor that affects the biological behavior of the tumor. Therefore, the orthotopic model of liver cancer is essential for verifying the pharmacodynamics of antitumor-targeting agents (Blakey et al., Citation2002). Lu et al. (Citation2006) administrated in Kunming mice with 2.88 × 108/mL ascitic hepatoma H22 cells injected under the liver capsule at a dose of 0.05 mL per mouse, then closed abdominal cavity. Mice were previously abdominal anesthetized by pentobarbital natrium. After grouping, mice were respectively delivered DHAQ-PBCA-NS and DHAQ for three times at the 1st, 5th and 9th day after inoculation. The result of the experiment indicated that the chemotherapeutic index of DHAQ-PBCA-NS was 3.42 times higher than the level of DHAQ (). From this pharmacodynamics experiment, it can be drawn that the agents presented obvious hepatic-targeting and tumor inhibiting efficiency.
Table 1. Relationship of dose-effect of DHAQ and DHAAQ-PBCA-NS on liver tumor H22.
Xenograft models of human hepatoma
The ultimate purpose of the establishment of xenotransplanted tumor models is to provide an in-vivo method in order to directly explore the biological characteristics and pathogenesis of human tumor (Goldstein et al., Citation1995). The orthotopic transplantation tumor models of human hepatoma not only maintain the structure of the primary tumor, but also reserve the most biological characteristics of human tumors, especially the property of metastasis, which enables tumors to present similar malignant behavior in the host body to that in patient’s body (Kubota, Citation1994). This feature is particularly supportive of the targeting agents’ study (Furukawa et al., Citation1993). At present, nude mice are the best models of human liver cancer (Flatmark et al., Citation2004). The complete human hepatoma tissues of orthotopically implanted tumors in nude mice retain the structural characteristics of human hepatoma tissues, as well as the potential of invasion and metastasis. Thus, this modeling approach is most close to the biological evolution and characteristics of human liver cancer let alone it takes less time to establish, but with a higher success rate. Therefore, it is a kind of desirable animal model of the study on anti-hepatoma-targeting agents (Kukowska-Latallo et al., Citation2005). On the other side, xenograft models of human liver cancer have to face following disadvantage factors: nude mice are incapable of performing the immune-related anti-tumor metastasis research for the lack of T-cell immunity function. In addition, nude mice are expensive, difficult to rear and uncommonly used (Cai et al., Citation2005). As a result, using orthotopic nude models to validate targeting agents is rarely reported (Leenders et al., Citation2008).
Nude mice models are typically divided into subcutaneous transplantation model and orthotopic transplantation model. Hasegawa et al. (Citation2001) and Hirano et al. (Citation2001) adjusted the concentration of HuH7 (human hepatocellular carcinoma) to 5 × 106/mL and subcutaneously injected it into male SCID mice, and 3 weeks later, consecutively injected mice with HVJ liposomes for 5 days (). As a consequence, HVJ liposomes acted pretty solid improvement on the body weight and pathological indicators of nude mice. Moreover, researchers subcutaneously inoculated human hepatoma cells into BALB/c nude mice to investigate targeted ultrasound contrast agents, and the outcome demonstrated that the agents are able to specifically enhance the targeting efficiency of hepatocellular carcinoma (Hamilton et al., Citation2002; Leong-Poi et al., Citation2003).
Figure 2. Scheme of the in-vivo study design. (A) Single-injection model. (B) Repeat injection model. About 3 weeks after the tumor cells injection, HVJ liposomes solution was injected once a week. GCV was injected i.p. once a day for 5 days from the day after each injection of HVJ liposome solution.
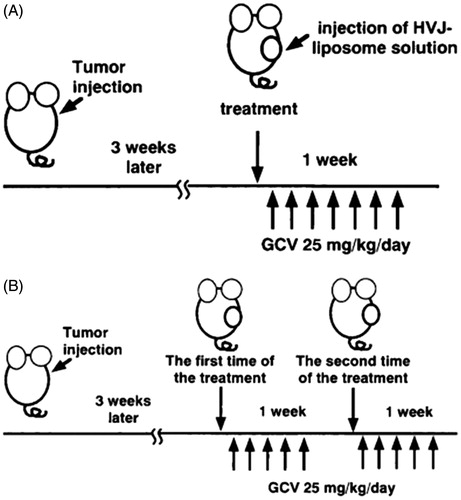
Gene animal models
With the increasingly deep acknowledgement of the cell biology characteristics and the progression of genetic engineering, the research of animal methods had improved greatly (Ellinwood et al., Citation2005). However, the above-mentioned tumor models cannot meet the demand of the comprehensive study on liver cancer. As a consequence, the gene model emerged at the right moment (Lampson et al., Citation2001). Currently used gene models are mainly in the two patterns, one is transgenic tumor model and the other is knock-out tumor model.
Transgenic tumor models
Transgenic tumor animal model is established by virtue of the genetic engineering techniques, transferring certain tumor exogenous genes into the host chromosomes through germ cells or early embryonic stem cells, stably integrating the exogenous tumor genes in genomes and being passed onto future generations (Guy et al., Citation1992; Gingrich et al., Citation1996). The essential reason that leaded normal cells to display malignant lesions is the gene mutation (Stephen et al., Citation2015). Exploring the formation, development and evolution of tumors at the gene level has created a new era of cancer research (Adams & Cory, Citation1991). The emergence of some hi-techs, like molecular cloning technology and micro-injection technique, paved the way for the establishment of transgenic tumor models. Mouse is the most commonly used animal species in transgenic experiment (Sharpless & DePinho, Citation2006). Since the location, structure and function of oncogene and proto-oncogene are still unclear by far and require continuous exploration, thus, the success rate of established transgenic animals is remaining to be low (Hogan & Lyons, Citation1988).
Knock-out tumor models
Based on the principle of homologous recombination, the knock-out technology transfers the exogenous DNA into cells for producing a recombination with the specific homologous sequence on endogenous chromosomes, in order to modify the genome (Jackson et al., Citation1995; Serra et al., Citation2004). Although the models’ incidence is a bit low and there are obstacles remained to be solved in the practical operation (Zhang et al., Citation2004), this kind of model still shows us the promising future (Matalon et al., Citation2000; Asahi et al., Citation2001).
The establishment of transgenic and knock-out models is on the basis of the combination of in-vitro and in-vivo studies (Ristevski, Citation2005). While operating on the molecular and cellular levels, and generating effects at the overall animal level, enable us to more completely investigate the mechanism of tumor development, the immunological relationship between tumor cells and the organism, as well as the evolution and apoptosis of tumor (Keffer et al., Citation1991).
In current studies, there are mainly two ways to produce knock-out mice: gene targeting and gene trapping (Sundaresan et al., Citation1995; Leighton et al., Citation2008). The gene-targeting technology, including gene knock-out and knock-in (Kuhn et al., Citation1995), is on account of the success of embryonic stem cell culture and the development of in-vitro homologous recombination, so as to discover the function deficits of mouse genomes caused by gene mutation (Capecchi, Citation1989). In 1987, the world’s first mice gene-targeting experiment was successfully completed (Thomas & Capecchi, Citation1987; Mansour, Citation1988). In the past two decades, gene-targeting technology has accelerated the research of gene function, leading to three Nobel Prize winners in 2007 for contributing this method (Mak, Citation2007) (). Gene trapping technology was formed as an alternative plan of gene targeting to induce the mutation (Abuin et al., Citation2007), with the properties of high-throughput and random mutation (Kothary et al., Citation1988; Gossler et al., Citation1989). This method is not as specific as gene targeting. However, it takes less time to knock out larger amounts of genes (Takeuchi, Citation1997; Zambrowicz & Friedrich, Citation1998). The combination of gene targeting and gene trapping is expected to knockout all of the genes in mice.
Figure 3. A flowchart for the large-scale acquisition storage and cataloguing of gene traps in mouse ES cell lines.
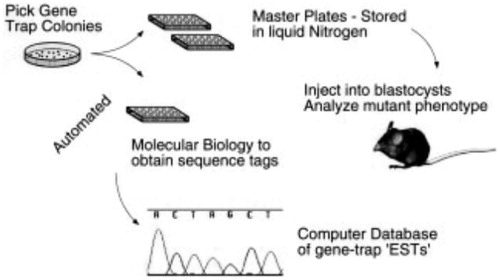
Morris et al. (2014) assessed the effect of the TGF-β signaling pathway on liver tumors induced by phosphatase and tensin homolog (Pten) loss. Interestingly, deletion of both Pten and Tgfbr2 (PtenLKO;Tgfbr2LKO) in the mouse liver resulted in a dramatic shift in tumor type to predominantly CC. However, phosphorylation of p70 S6 kinase was observed in the liver of all three phenotypes indicating that the loss of Tgfbr2 and/or Pten leads to an increase in this signaling pathway. Furthermore, Scf and EpCam expressions were also increased in the double knock-out mice. These results suggested that the alteration in tumor types between the PtenLKO mice and PtenLKO;Tgfbr2LKO mice is secondary to the altered regulation of stem-cell features induced by the loss of TGF-β signaling.
Zheng et al. (Citation1996) determined whether the mRNA expression of the proto-oncogene c-jun as well as the tumor suppressor gene p53 is increased by Cd in the target organ for Cd toxicity, namely, the liver. The effect of CdCl2 on the mRNA levels of c-jun and p53 was studied in livers of C57BL/6J (control) and MT-null mice by northern- and slot-blot analyses. The experimental data demonstrate that Cd induces the mRNA levels of c-jun and p53 in liver of mice, and MT-null mice are more sensitive to this effect; the Cd-induced increases in proto-oncogene/tumor suppressor gene expression may be mechanistically important for its carcinogenicity and hepatotoxicity ().
Figure 4. Liver Cd concentration (top) and serum alanine amino transferase levels (bottom) 12 h after Cd administration in C57BL/6J control and MT-null mice.
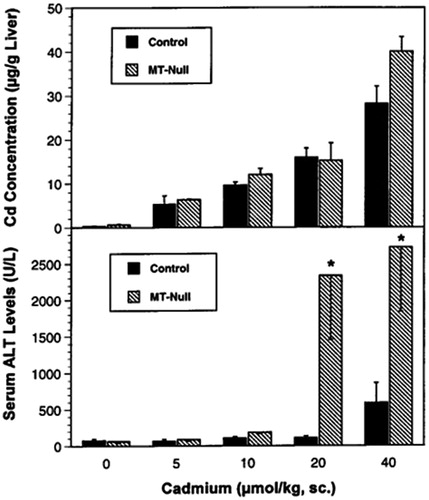
Kennedy et al. (Citation2014) employed a triple knock-out (TKO) mouse model with null alleles at the loci encoding the three relevant receptors for tumor necrosis factors α and β and IL-1α and IL-1β (i.e. null alleles at the Tnfrsf1a, Tnfrsf1b and Il-1r1 loci). The observation that TKO mice were resistant to the tumor promoting effects of dioxin in liver suggests that inflammatory cytokines play an important step in dioxin-mediated liver tumor promotion in the mouse (). The results support that the mechanism of dioxin acute hepatotoxicity and its activity as a promoter in a mouse two stage liver cancer model may be similar.
Figure 5. Decrease of dioxin-induced tumor multiplicity in the TNF/IL-1receptors triple null mice. (A) Dioxin-induced liver tumor multiplicity in the DEN-initiated WT and TKO mice. (B) Levels of 7-methylguanine in liver DNA of WT and triple-null (TKO) mice.
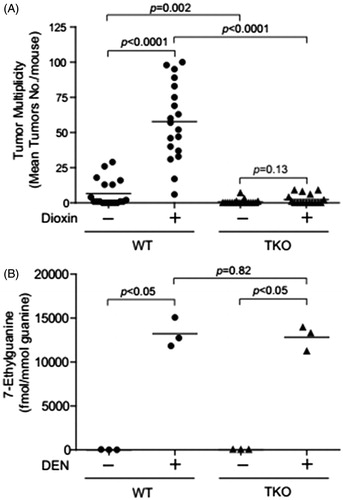
Chen et al. (Citation2014) established PIWIL2-specific transcript mono-allele and bi-allele knockout HepG2 cell lines using transcription activator-like effector nuclease (TALEN) technology. It was found using the cell line models that specific transcript knockdown of full length PIWIL2 could suppress cell proliferation (), while ectopic expression of PIWIL2 could enhance proliferation of HepG2 via suppressing the TGF-β pathway. Taken together, the study revealed critical negative regulation of TGF-β signaling by PIWIL2 in HepG2 tumor cells and provided an effective strategy to study specific gene transcript functions in cells.
Prospects
Selecting and researching a preferable animal model are the crux in evaluating the targeting agents and the pharmacodynamics of preparations. Although subcutaneously transplanting the H22 cells into nude mice is one of the most widely used liver cancer models by far, the orthotopic transplantation mice model is more appropriate for judging the pharmacodynamics of antitumor-targeting agents since it is better to imitate the in-vivo blood supply and growth environment of human hepatocellular carcinoma with low cost and short period. However, orthotopic transplantation mice model still could not meet the requirement of fully simulating the human hepatoma. An ideal liver cancer model should possess the following characteristics: the tumor cells simply grow on liver and mainly present in solitary; the occurrence and development of hepatoma are similar to the natural process of human liver cancer; the pathological features in carcinoma cells resemble that of human hepatocytes; the detection index of tumor enzymology and the serum alpha-fetoprotein would be positive; and animal models should be stable as well as easy to copy and operate within short experiment cycle. Collectively, it is necessary to exploit more desirable liver cancer models for the sake of validating the anti-hepatoma-targeting agents.
With the appearance of gene models, especially knock-out model, the research has attained unprecedented development. However, this technology still exists some drawbacks, and in recent years, the key of contention seems to be focused on whether the phenotype of knock-out animal is caused by the mutation of target gene. Gerlai (Citation2003) pointed out that the current knock-out model ignored the effect of background genotype, and due to the loss of knock-out gene, animal organism was likely to produce an avalanche of compensatory process and lead to the second alteration of genes. Therefore, it is reasonable to speculate that certain complex change of phenotype may not be on account of one particular causality. Furthermore, Fülöp et al. (Citation2003) noted that, besides the genetic background, some other factors would also complicate the results of gene knock-out. Since the gene mutant would probably delete in every tissue’s development processes, the rest of organ defects are likely to involve in the phenotypic changes as well. Taken together, the investigation of animal models is an essential aspect of the study on antitumor-targeting agents (Arap et al., Citation1998; Kukowska-Latallo et al., Citation2005). With the further studies of pharmacology, pharmacodynamics, biopharmaceutics and pharmacokinetics (Callegari et al., Citation2014; Denzler et al., Citation2014), it is expected that the research level of the antitumor-targeting models would attain a deeper level and accomplish more superior achievements (Chen & Calvisi, Citation2014; Verhaegh et al., Citation2014).
Declaration of interest
This work was financially supported by China Postdoctoral Science Foundation Funded Project (2014M562429) and Fundamental Research Funds for the Central University (xjj2013054 and xj08142016). The authors have no conflict of interest. The authors alone are responsible for the content and writing of this article.
References
- Abuin A, Hansen GM, Zambrowicz B. (2007). Gene trap mutagenesis. Handb Exp Pharmacol 178:129–47
- Adams JM, Cory S. (1991). Transgenic models of tumor development. Science 254:1161–7
- Antonio RJ, Angela C, Antonio R. (2015). Pharmacokinetic and pharmacodynamic evaluation of telaprevir for the treatment of hepatitis C. Drug Metab Toxicol 11:1157–65
- Arap W, Pasqualini R, Ruoslahti E. (1998). Cancer treatment by targeted drug delivery to tumor vasculature in a mouse model. Science 279:377–80
- Asahi M, Wang X, Mori T, et al. (2001). Effects of matrix metalloproteinase-9 gene knock-out on the proteolysis of blood–brain barrier and white matter components after cerebral ischemia. J Neurosci 21:7724–32
- Babbs CF. (1990). Free radicals and the etiology of colon cancer. Free Radic Biol Med 8:191–200
- Bishayee A, Politis T, Darvesh AS. (2010). Resveratrol in the chemoprevention and treatment of hepatocellular carcinoma. Cancer Treat Rev 36:43–53
- Blakey DC, Westwood FR, Walker M, et al. (2002). Antitumor activity of the novel vascular targeting agent ZD6126 in a panel of tumor models. Clin Cancer Res 8:1974–83
- Brannon-Peppas L, Blanchette JO. (2012). Nanoparticle and targeted systems for cancer therapy. Adv Drug Deliv Rev 64: 206–12
- Brillet PY, Gazeau F, Luciani A, et al. (2005). Evaluation of tumoral enhancement by superparamagnetic iron oxide particles: comparative studies with ferumoxtran and anionic iron oxide nanoparticles. Eur. Radiol 15:1369–77
- Brinster RL, Palmiter RD. (1984). Transgenic mice containing growth hormone fusion genes. Philos Trans R Soc Lond B Biol Sci 307:309–12
- Cai SR, Garbow JR, Culverhouse R, et al. (2005). A mouse model for developing treatment for secondary liver tumors. Int J Oncol 27:113–20
- Callegari E, Gramantieri L, Domenicali M, et al. (2014). MicroRNAs in liver cancer: a model for investigating pathogenesis and novel therapeutic approaches. Cell Death Differ 22:46–57
- Capecchi MR. (1989). The new mouse genetics: altering the genome by gene targeting. Trends Genet 5:70–6
- Chen X, Calvisi DF. (2014). Hydrodynamic transfection for generation of novel mouse models for liver cancer research. Am J Pathol 184:912–23
- Chen Y, Hu W, Lu Y, et al. (2014). A TALEN-based specific transcript knock-down of PIWIL2 suppresses cell growth in HepG2 tumor cell. Cell Prolif 7:448–56
- Dawson LA, Normolle D, Balter JM, et al. (2002). Analysis of radiation-induced liver disease using the Lyman NTCP model. Int J Radiat Oncol Biol Phys 53:810–21
- De Carvalho DD, Binato R, Pereira WO, et al. (2010). BCR–ABL-mediated upregulation of PRAME is responsible for knocking down TRAIL in CML patients. Oncogene 30:223–33
- Denzler R, Agarwal V, Stefano J, et al. (2014). Assessing the ceRNA hypothesis with quantitative measurements of miRNA and target abundance. Mol cell 54:766–76
- Ellinwood NM, Vite CH, Haskins ME. (2005). Gene therapy for lysosomal storage diseases: the lessons and promise of animal models. J Gene Med 6:481–506
- Feray C, Zignego AL, Samuel D, et al. (1990). Persistent hepatitis B virus infection of mononuclear blood cells without concomitant liver infection: the liver transplantation model. Transplantation 49:1155–7
- Ferrari M. (2005). Cancer nanotechnology: opportunities and challenges. Nat Rev Cancer 5:161–71
- Fisher PB, Weinstein IB, Eisenberg D, et al. (1978). Interactions between adenovirus, a tumor promoter, and chemical carcinogens in transformation of rat embryo cell cultures. Proc Natl Acad Sci USA 75:2311–14
- Flatmark K, Mælandsmo GM, Martinsen M, et al. (2004). Twelve colorectal cancer cell lines exhibit highly variable growth and metastatic capacities in an orthotopic model in nude mice. Eur J Cancer 40:1593–8
- Freise CE, Ferrell L, Liu T, et al. (1999). Effect of systemic cyclosporine on tumor recurrence after liver transplantation in a model of hepatocellular carcinoma1. Transplantation 67:510–13
- Fujiki H. (2014). Gist of Dr. Katsusaburo Yamagiwa’s papers entitled “Experimental study on the pathogenesis of epithelial tumors” (I to VI reports). Cancer Sci 105:143–49
- Fülöp AK, Földes A, Buzás E, et al. (2003). Hyperleptinemia, visceral adiposity, and decreased glucose tolerance in mice with a targeted disruption of the histidine decarboxylase gene. Endocrinology 144:4306–14
- Furukawa T, Fu X, Kubota T, et al. (1993). Nude mouse metastatic models of human stomach cancer constructed using orthotopic implantation of histologically intact tissue. Cancer Res 53:1204–08
- Gao Y, Xie J, Chen H, et al. (2014). Nanotechnology-based intelligent drug design for cancer metastasis treatment. Biotechnol Adv 32:761–77
- Gerin JL. (1990). Experimental WHV infection of woodchucks: an animal model of hepadnavirus-induced liver cancer. Gastroenterol Jpn 25:38–42
- Gerlai R. (2003). Zebra fish: an uncharted behavior genetic model. Behav Genet 33:461–8
- Ghoshal AK, Farber E. (1984). The induction of liver cancer by dietary deficiency of choline and methionine without added carcinogens. Carcinogenesis 5:1367–70
- Gingrich JR, Barrios RJ, Morton RA, et al. (1996). Metastatic prostate cancer in a transgenic mouse. Cancer Res 56:4096–102
- Goldstein NI, Prewett M, Zuklys K, et al. (1995). Biological efficacy of a chimeric antibody to the epidermal growth factor receptor in a human tumor xenograft model. Clin Cancer Res 1:1311–18
- Gossler A, Joyner AL, Rossant J, et al. (1989). Mouse embryonic stem cells and reporter constructs to detect developmentally regulated genes. Science 244:463–5
- Guy CT, Cardiff RD, Muller WJ. (1992). Induction of mammary tumors by expression of polyomavirus middle T oncogene: a transgenic mouse model for metastatic disease. Mol Cell Biol 12:954–61
- Hamilton A, Huang SL, Warnick D, et al. (2002). Left ventricular thrombus enhancement after intravenous injection of echogenic immunoliposomes studies in a new experimental model. Circulation 105:2772–8
- Hansen K, Khanna C. (2004). Spontaneous and genetically engineered animal models: use in preclinical cancer drug development. Eur J Cancer 40:858–80
- Hasegawa H, Shimada M, Yonemitsu Y, et al. (2001). Preclinical and therapeutic utility of HVJ liposomes as a gene transfer vector for hepatocellular carcinoma using herpes simplex virus thymidine kinase. Cancer Gene Ther 8:252–8
- Hirano T, Kaneko S, Kaneda Y, et al. (2001). HVJ-liposome-mediated transfection of HSVtk gene driven by AFP promoter inhibits hepatic tumor growth of hepatocellular carcinoma in SCID mice. Gene Ther 8:80–3
- Ho RJ, Chien J. (2014). Trends in translational medicine and drug targeting and delivery: new insights on an old concept-targeted drug delivery with antibody–drug conjugates for cancers. J Pharm Sci 103:71–7
- Hogan B, Lyons K. (1988). Gene targeting: getting nearer the mark. Nature 336:304–5
- Huang S, Armstrong EA, Benavente S, et al. (2004). Dual-agent molecular targeting of the epidermal growth factor receptor (EGFR) combining anti-EGFR antibody with tyrosine kinase inhibitor. Cancer Res 64:5355–62
- Jackson NSH, Gallin JI, Holland SM. (1995). The p47phox mouse knock-out model of chronic granulomatous disease. J Exp Med 182:751–8
- Kapadia RD, Stroup GB, Badger AM, et al. (1998). Applications of micro-CT and MR microscopy to study pre-clinical models of osteoporosis and osteoarthritis. Technol Health Car 6:361–72
- Kaplan-Lefko PJ, Chen TM, Ittmann MM, et al. (2003). Pathobiology of autochthonous prostate cancer in a pre-clinical transgenic mouse model. Prostate 55:219–37
- Keffer J, Probert L, Cazlaris H, et al. (1991). Transgenic mice expressing human tumour necrosis factor: a predictive genetic model of arthritis. J EMBO 10:4025–31
- Kennedy GD, Nukaya M, Moran SM, et al. (2014). Liver tumor promotion by 2,3,7,8-tetrachlorodibenzo-p-dioxin is dependent on the aryl hydrocarbon receptor and TNF/IL-1 receptors. Toxicol Sci 140:135–43
- Khanna C, Hunter K. (2005). Modeling metastasis in vivo. Carcinogenesis 26:513–23
- Kim CM, Koike K, Saito I, et al. (1991). HBx gene of hepatitis B virus induces liver cancer in transgenic mice. Nature 351:317–20
- Ko YH, Pedersen PL, Geschwind JF. (2001). Glucose catabolism in the rabbit VX2 tumor model for liver cancer: characterization and targeting hexokinase. Cancer Lett 173:83–91
- Kota J, Chivukula RR, O’Donnell KA, et al. (2009). Therapeutic microRNA delivery suppresses tumorigenesis in a murine liver cancer model. Cell 137:1005–17
- Kothary R, Clapoff S, Brown A, et al. (1988). A transgene containing lacZ inserted into the dystonia locus is expressed in neural tube. Nature 335:435–7
- Kubota T. (1994). Metastatic models of human cancer xenografted in the nude mouse: the importance of orthotopic transplantation. J Cell Biochem 56:4–8
- Kuhn R, Schwenk F, Aguet M, et al. (1995). Inducible gene targeting in mice. Science 269:1427–9
- Kukowska-Latallo JF, Candido KA, Cao Z, et al. (2005). Nanoparticle targeting of anticancer drug improves therapeutic response in animal model of human epithelial cancer. Cancer Res 65:5317–24
- Lampson LA. (2001). New animal models to probe brain tumor biology, therapy, and immunotherapy: advantages and remaining concerns. J Neuro-Oncol 53:275–87
- Leenders MW, Nijkamp MW, Rinkes IHB. (2008). Mouse models in liver cancer research: a review of current literature. World J Gastroenterol 14:6915–23
- Leighton PA, Van de Lavoir MC, Diamond JH, et al. (2008). Genetic modification of primordial germ cells by gene trapping, gene targeting, and фC31 integrase. Mol Reprod Dev 75:1163–75
- Leong-Poi H, Christiansen J, Klibanov AL, et al. (2003). Noninvasive assessment of angiogenesis by ultrasound and microbubbles targeted to αv-integrins. Circulation 107:455–60
- Liu Y, Miyoshi H, Nakamura M. (2007). Nanomedicine for drug delivery and imaging: a promising avenue for cancer therapy and diagnosis using targeted functional nanoparticles. Int J Cancer 120:2527–37
- Lu B, Xiong SB, Yang H, et al. (2006). Mitoxantrone-loaded BSA nanospheres and chitosan nanospheres for local injection against breast cancer and its lymph node metastases. II. Tissue distribution and pharmacodynamics. Int J Pharm 307:175–81
- Mahfoozur R, Sohail A, Javed A, et al. (2015). Nanomedicine-based drug targeting for psoriasis: potentials and emerging trends in nanoscale pharmacotherapy. Drug Deliv 12:635–52
- Mak TW. (2007). Gene targeting in embryonic stem cells scores a knockout in Stockholm. Cell 131:1027–31
- Makino S. (1956). Further evidence favoring the concept of the stem cell in ascites tumors of rats. Ann NY Acad Sci 63:818–30
- Mansour SL, Thomas KR, Capecchi MR. (1988). Disruption of the proto-oncogene int-2 in mouse embryo-derived stem cells: a general strategy for targeting mutations to non-selectable genes. Nature 336:348–52
- Matalon R, Rady PL, Platt KA, et al. (2000). Knock-out mouse for Canavan disease: a model for gene transfer to the central nervous system. J Gene Med 2:165–75
- Morris SM, Carter KT, Baek JY, et al. (2014). TGF-β signaling alters the pattern of liver tumorigenesis induced by Pten inactivation. Oncogene 34:3273–82
- Murali MR, Jackson DD, Jebasingh D, et al. (2015). Anti-hyperlipidemic effect of methanol bark extract of Terminalia chebula in male albino Wistar rats. Drug Deliv 53:1133–40
- Nakatani T, Roy G, Fujimoto N, et al. (2001). Sex hormone dependency of diethylnitrosamine-induced liver tumors in mice and chemoprevention by leuprorelin. Jpn J Cancer Res 92:249–56
- Nasongkla N, Bey E, Ren J, et al. (2006). Multifunctional polymeric micelles as cancer-targeted, MRI-ultrasensitive drug delivery systems. Nano Lett 6:2427–30
- Naugler WE, Sakurai T, Kim S, et al. (2007). Gender disparity in liver cancer due to sex differences in MyD88-dependent IL-6 production. Science 317:121–4
- Ornstein DK, Englert C, Gillespie JW, et al. (2000). Characterization of intracellular prostate-specific antigen from laser capture microdissected benign and malignant prostatic epithelium. Clin Cancer Res 6:353–6
- Peer D, Karp JM, Hong S, et al. (2007). Nanocarriers as an emerging platform for cancer therapy. Nat Nanotechnol 2:751–60
- Peer D, Margalit R. (2004). Loading mitomycin C inside long circulating hyaluronan targeted nano-liposomes increases its antitumor activity in three mice tumor models. Int J Cancer 108:780–9
- Peterson DL, Sheridan PJ, Brown Jr WE. (1994). Animal models for brain tumors: historical perspectives and future directions. J Neurosurg 80:865–76
- Qian X, Peng XH, Ansari DO, et al. (2007). In vivo tumor targeting and spectroscopic detection with surface-enhanced Raman nanoparticle tags. Nat Biotechnol 26:83–90
- Ren W, Qiao Z, Wang H, et al. (2003). Flavonoids: promising anticancer agents. Med Res Rev 23:519–34
- Ristevski S. (2005). Making better transgenic models. Mol Biotechnol 29:153–63
- Rosol TJ, Tannehill-Gregg SH, LeRoy BE, et al. (2003). Animal models of bone metastasis. Cancer 97:748–57
- Rygaard J, Povlsen CO. (1969). Heterotransplantation of a human malignant tumor to “Nude” mice. Acta Pathol Microbiol Scand 77:758–60
- Sakai D, Mochida J, Iwashina T, et al. (2005). Differentiation of mesenchymal stem cells transplanted to a rabbit degenerative disc model: potential and limitations for stem cell therapy in disc regeneration. Spine 30:2379–87
- Sell S, Leffert HL. (1982). An evaluation of cellular lineages in the pathogenesis of experimental hepatocellular carcinoma. Hepatology 2:77S–86S
- Semenza GL. (2003). Targeting HIF-1 for cancer therapy. Nat Rev Cancer 3:721–32
- Serra R, Villani M, Semeria A. (2004). Genetic network models and statistical properties of gene expression data in knock-out experiments. J Theor Biol 227:149–57
- Seymour LW, Ferry DR, Anderson D, et al. (2002). Hepatic drug targeting: phase I evaluation of polymer-bound doxorubicin. J Clin Oncol 20:1668–76
- Sharpless NE, DePinho RA. (2006). The mighty mouse: genetically engineered mouse models in cancer drug development. Nat Rev Drug Discov 5:741–54
- Silveira ER, Naves MMV, Vannucchi H, et al. (2001). Vitamin A and all-trans and 9-cis retinoic acids inhibit cell proliferation during the progression phase of hepatocarcinogenesis in Wistar rats. Nutr Cancer 39:244–51
- Stephen LC, Anthony WC, Winnie Y. (2015). Novel therapeutic targets and predictive markers for hepatocellular carcinoma. Therapeutic Targets 19:973–83
- Sundaresan V, Springer P, Volpe T, et al. (1995). Patterns of gene action in plant development revealed by enhancer trap and gene trap transposable elements. Gene Dev 9:1797–810
- Sudimack J, Lee RJ. (2000). Targeted drug delivery via the folate receptor. Adv Drug Deliv Rev 41:147–62
- Sun Y. (1990). Free radicals, antioxidant enzymes, and carcinogenesis. Free Radic Biol Med 8:583–99
- Takeuchi T. (1997). A gene trap approach to identify genes that control development. Dev Growth Differ 39:127–34
- Talmadge JE, Singh RK, Fidler IJ, et al. (2007). Murine models to evaluate novel and conventional therapeutic strategies for cancer. J Am Pathol 170:793–804
- Tardi P, Choice E, Masin D, et al. (2000). Liposomal encapsulation of topotecan enhances anticancer efficacy in murine and human xenograft models. Cancer Res 60:3389–93
- Tian Y, Guo B, Jia H, et al. (2012). Targeted therapy via oral administration of attenuated Salmonella expression plasmid-vectored Stat3-shRNA cures orthotopically transplanted mouse HCC. Cancer Gene Ther 19:393–401
- Thomas KR, Capecchi MR. (1987). Site-directed mutagenesis by gene targeting in mouse embryo-derived stem cells. Cell 51:503–12
- Thorpe PE. (2004). Vascular targeting agents as cancer therapeutics. Clin Cancer Res 10:415–27
- Toyokuni S. (1999). Reactive oxygen species-induced molecular damage and its application in pathology. Pathol Int 49:91–102
- Tsuruo T, Naito M, Tomida A, et al. (2003). Molecular targeting therapy of cancer: drug resistance, apoptosis and survival signal. Cancer Sci 94:15–21
- Uehara K, Ichida T, Sugahara S, et al. (2002). Systemic administration of liposome-encapsulated OK-432 prolongs the survival of rats with hepatocellular carcinoma through the induction of IFN-gamma-producing hepatic lymphocytes. J Gastroen Hepatol 17:81–90
- Van der Spoel TI, Agostoni P, Van Belle E, et al. (2011). Human relevance of pre-clinical studies in stem cell therapy: systematic review and meta-analysis of large animal models of ischaemic heart disease. Cardiovasc Res 91:649–58
- Van Dyke T, Jacks T. (2002). Cancer modeling in the modern era: progress and challenges. Cell 108:135–44
- Verhaegh W, Van Ooijen H, Inda MA, et al. (2014). Selection of personalized patient therapy through the use of knowledge-based computational models that identify tumor-driving signal transduction pathways. Cancer Res 74:2936–45
- Waxman S, Anderson KC. (2001). History of the development of arsenic derivatives in cancer therapy. Oncologist 6:3–10
- Weigelt B, Lo AT, Park C, et al. (2010). HER2 signaling pathway activation and response of breast cancer cells to HER2-targeting agents is dependent strongly on the 3D microenvironment. Breast Cancer Res Treat 122:35–43
- Weinstein IB, Joe AK. (2006). Mechanisms of disease: oncogene addiction – a rationale for molecular targeting in cancer therapy. Nat Clin Pract Oncol 3:448–57
- Wiesner RH, McDiarmid SV, Kamath PS, et al. (2001). MELD and PELD: application of survival models to liver allocation. Liver Transplant 7:567–80
- Williams GM. (1980). The pathogenesis of rat liver cancer caused by chemical carcinogens. Biochem Biophys Acta 605:167–89
- Wu M, Frieboes HB, McDougall SR., et al. (2013). The effect of interstitial pressure on tumor growth: coupling with the blood and lymphatic vascular systems. J Theor Biol 320:131–51
- Yang JA, Kong WH, Sung DK, et al. (2014). Hyaluronic acid – tumor necrosis factor-related apoptosis-inducing ligand conjugate for targeted treatment of liver fibrosis. Acta Biomater 7061:444–9
- Yang R, Rescorla FJ, Reilly CR, et al. (1992). A reproducible rat liver cancer model for experimental therapy: introducing a technique of intrahepatic tumor implantation. J Surg Res 52:193–8
- Yao FY, Ferrell L, Bass NM, et al. (2001). Liver transplantation for hepatocellular carcinoma: expansion of the tumor size limits does not adversely impact survival. Hepatology 33:1394–403
- Yao Z, Lu R, Jia J, et al. (2006). The effect of tripeptide tyroserleutide (YSL) on animal models of hepatocarcinoma. Peptides 27:1167–72
- Zambrowicz BP, Friedrich GA. (1998). Comprehensive mammalian genetics: history and future prospects of gene trapping in the mouse. Int J Dev Biol 42:1025–36
- Zhang J, Schweers B, Dyer MA. (2004). The first knockout mouse model of retinoblastoma. Cell Cycle 3:952–9
- Zheng H, Liu J, Choo KH, et al. (1996). Metallothionein-I and –II knock-out mice are sensitive to cadmium-induced liver mRNA expression of c-jun and p53. Toxicol Appl Pharmacol 136:229–35
- Zheng H, Matte-Martone C, Li H, et al. (2008). Effector memory CD4 + T cells mediate graft-versus-leukemia without inducing graft-versus-host disease. Blood 111:2476–84