Abstract
Silicon-containing nanoparticles (NPs) are considered promising drug carriers for targeted drug delivery. In this study, we investigated the physical and chemical properties of silicon-containing NPs, including silica and organomodified silica NPs (SiO2NPs and OrSiO2NPs, respectively), with different surface modifications, with the aim of increasing drug-loading efficiency. In addition, we described the original synthesis methods of different sizes of OrSiO2NPs, as well as new hybrid OrSiO2NPs with a silica core (SiO2 + OrSiO2NPs). Animal experiments revealed that the silicon-containing NPs investigated were non-toxic, as evidenced by a lack of hemodynamic response after intravenous administration. Bioelimination studies showed rapid renal excretion of OrSiO2NPs. In drug release kinetics studies, adenosine was immobilized on SiO2NPs using three different approaches: physical adsorption, ionic, and covalent bonding. We observed that the rate of adenosine desorption critically depended on the type of immobilization; therefore, adenosine release kinetics can be adjusted by SiO2NP surface modification technique. Adsorption of adenosine on SiO2 + OrSiO2NPs resulted in a significant attenuation of adenosine-induced hypotension and bradycardia.
Introduction
Nanoparticles (NPs) with various physicochemical properties are widely used for medical applications. One of the most important uses of NPs in medicine is their application as drug delivery vehicles targeted to an area of interest, which augments their therapeutic effect and minimizes the side effects (Galagudza et al., Citation2010a,Citationb). The ideal NP for drug delivery should fulfill the following criteria: have complete biodegradation, minimal toxicity, optimal drug loading efficiency, and predictable drug release kinetics after accumulation in the area of interest (De Jong et al., Citation2008). Biodegradable organic NPs, such as liposomes and micelles, loaded with chemotherapeutic agents have been successfully used in the treatment of cancer (Abdelkader et al., Citation2014; Lyseng-Williamson et al., Citation2013; Puntawee et al., Citation2015).
Accumulation of organic or inorganic NPs in damaged tissue as a result of increased microvascular permeability and/or diminished lymphatic drainage is usually referred to as passive targeting (Bae, Citation2009; Galagudza et al., Citation2010a,Citationb). In active targeting systems, the selectivity of drug-loaded NP accumulation in the area of interest is further increased by the engraftment of targeting ligands to the NP surface, which results in a specific interaction between the targeting ligand and the unique disease marker expressed on the injured cells. Therapeutic NPs are therefore retained in the area of interest (Bae, Citation2009; Levchenko et al., Citation2012).
While passive- and active-targeted drug delivery have been intensively investigated in cancer models, the possibility of delivering cardioprotective drugs to the area of myocardial ischemia-reperfusion is much less studied (Galagudza et al., Citation2012; Levchenko et al., Citation2012; Li et al., Citation2015). To date, few studies that show a reduction in irreversible myocardial ischemia–reperfusion injury after the administration of liposome-embedded adenosine (Takahama et al., Citation2009), or adenosine adsorbed on the surface of the silica NPs (SiO2NPs) (Galagudza et al., Citation2012) have been reported. We have previously shown that SiO2NPs are non-toxic during acute intravenous administration (Galagudza et al., Citation2010a,Citationb). However, a single intravenous injection of SiO2NPs resulted in their accumulation in the organs of the reticuloendothelial system, and caused the formation of multiple granulomas in the liver and spleen (Ivanov et al., Citation2012). Although some studies have demonstrated the complete biodegradation of SiO2NPs (Malvindi et al., Citation2012), most studies, in agreement with our data, reveal a delayed pattern of SiO2NP biodegradation (Nishimori et al., Citation2009; Xie et al., Citation2010). However, one cannot exclude the possibility that surface modification, including sulfonation, of SiO2NPs may affect the rate of their biodegradation. We hypothesized that surface modification and functionalization of SiO2NPs would result in the enhancement of biodegradation and sorption properties, and would provide a gradual release of the drug. In this regard, sulfonated as well as organomodified silica NPs (OrSiO2NP) with potentially improved biodegradability are of particular interest. The next step in engineering of nanocarriers for drug delivery is choosing the method of drug immobilization. The physical adsorption, ionic, and covalent bonding are the most widely used methods (Chittasupho et al., Citation2013; Rasouli et al., Citation2014). Comparison studies of various methods of drug immobilization are often used in order to predict in vivo properties of drug-loaded NPs.
The purpose of this study was to describe techniques of OrSiO2NP synthesis, as well as examine the physical and chemical properties of OrSiO2NPs and SiO2NPs with different surface modifications. In addition, we investigated the acute toxicity of silicon-containing NPs and the bioelimination of OrSiO2NPs in order to assess the possibility of using these materials for drug delivery. In our drug release kinetics studies, adenosine was immobilized on SiO2NPs using three different approaches: physical adsorption, ionic bonding, and covalent bonding.
Materials and methods
Materials
All chemicals were supplied by Sigma Aldrich (Sigma-Aldrich Rus LLC, Moscow, Russia) unless otherwise stated.
Synthesis and modification of NPs
Aminated SiO2NPs
Standard fumed highly dispersed silica (Aerosil of A-380 mark, Vekton Ltd., Russia; specific surface area: 370 m2 × g−1) was aminated by chemisorption of 3-aminopropyltriethoxysilane in the gas phase using a continuous flow reactor. The technique of synthesis included (a) the removal of physically bound water from the surface of silica at 220 °C for 2 h, (b) the chemisorption of 3-aminopropyltriethoxysilane at the same temperature for 3 h, and (c) the removal of excess of reagents and byproducts for 2 h (). The unreacted alkoxy groups of the chemisorbed aminopropyltriethoxysilane were then fully hydrolyzed by treating the sample with water vapor for 1 h at 200 °C. The amine number, as determined by means of titration, was 0.720 mmol/g. The specific surface area of aminated SiO2NPs determined by Brunauer–Emmett–Teller (BET) surface area analysis (ASAP 2020 MP, Micromeritics, Norcross, GA) was 260 m2 × g−1.
Functionalization of aminated SiO2NPs
Functionalization of SiO2NPs was performed with succinic anhydride in order to obtain acid radicals for ionic bonding of drug. To immobilize succinic anhydride, a 10-fold excess dissolved in dimethylformamide (DMF) was used relative to the aminated SiO2NPs. The resulting solution containing 50 mg of succinic anhydride was added to 100 mg pre-dried aminated SiO2NPs. The reaction was performed in an ultrasonic bath over 2 h without heating (). The product was washed sequentially with DMF, ethanol, and water in order to obtain a neutral pH. To determine whether amino groups were present on the surface of the SiO2NPs, a test reaction was performed, which showed a negative result. As determined by titration, the carboxyl group content was 0.5 mmol/g, which corresponds to the quantity of amino groups on aminated SiO2NPs. The specific surface area of succinic anhydride modified SiO2NPs determined by BET was 250 m2 × g−1.
To produce binding sites for covalent immobilization of drugs on the SiO2NP surface, a glutaraldehyde reaction was used (Osek, Citation2003). For this reaction, 0.5 ml glutaraldehyde was mixed with 0.5 ml distilled water, and added to aminated SiO2NPs pre-dried at 80 °C (). Synthesis was carried out for 2 h at room temperature on a mixer (LOIPLS 110, LOIP Ltd., St. Petersburg, Russia). After the reaction, the modified SiO2NPs were washed with 10 volumes of distilled water and centrifuged. BET surface area was 245 m2 × g−1.
Strategies for adenosine immobilization
In order to study the drug release kinetics, adenosine was used as a model drug. Three methods of adenosine immobilization on SiO2NP surface were studied: physical adsorption, covalent bonding, and ionic bonding. The kinetics of adenosine release from the surface of unmodified SiO2NPs were determined by its residual concentration in the solution after complete adsorption. Adenosine (10 mg) was dissolved in distilled water, followed by the addition of 100 mg SiO2NPs. After that, the solution was mixed for 2 h, centrifuged, and the upper phase was analyzed by spectrophotometry (Shimadzu UV-2450, Shimadzu Corp., Tokyo, Japan) at λ = 260 nm. The quantity of adsorbed adenosine was 12.6 mg/g.
Сovalent binding of adenosine to glutaraldehyde-modified SiO2NPs was performed under continuous mixing for 2 h. Thirty milligrams of adenosine dissolved in 1 ml of distilled water was added to 100 mg of modified SiO2NPs. The solution was then centrifuged and washed with water to remove the excess reactants. The grafted adenosine content was determined to be 3.26 mg/g. Ionic binding of adenosine to succinic anhydride-treated SiO2NPs containing carboxyl groups was performed as described above. The content of the immobilized adenosine was determined to be 1.43 mg/g.
OrSiO2NPs
The synthesis reaction was carried out in acetone (10 ml) previously dissolved in a two times excess of succinic anhydride, followed by an addition of 1 ml of 3-aminopropyltriethoxysilane, using mixing for 1 h. Then, the unreacted ethoxy groups were hydrolyzed by adding 0.5 ml water. After 30 min, the acetone was completely evaporated, and an additional 10 ml of water was added. To remove the succinic acid formed by the hydrolysis of excess succinic anhydride, the reaction was purified by filtering through a strongly basic anion exchange column (Amberlite IRA-402 Cl, The Dow Company, Midland, MI) in a gravity mode. This resulted in the formation of three-dimensionally cross-linked polymer polyorganosiloxane (OrSiO2NPs3, number 3 in superscript indicates the size of the NP). An overview of the synthesis is shown in .
Figure 2. Synthesis of OrSiO2NPs with a silica core. (a) Scheme of the synthesis of the OrSiO2NPs; (b and c) scheme of synthesis SiO2 + OrSiO2NPs.
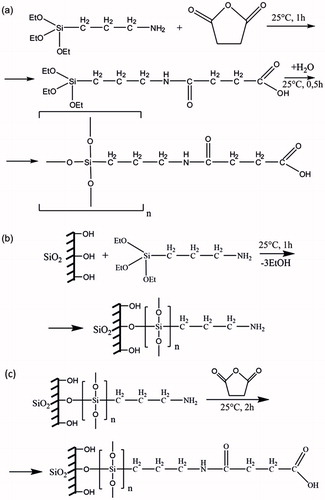
For larger NPs, the synthesized OrSiO2NPs3 gel was completely dried at elevated temperatures. In this process, condensation causes the formation of longer chains, and consequently, larger NPs. Their size depends on the temperature. In the present study, OrSiO2NPs were obtained at 70 °C. The dried precipitate was sonicated to produce a saturated solution. Undissolved residual material was discarded after centrifugation. Particle size analysis showed that the mean diameter of OrSiO2NPs was 150 nm (OrSiO2NPs150). As determined by titration, the content of carboxyl groups in OrSiO2NPs was 3.01 mmol/g.
OrSiO2NPs with silica core (SiO2 + OrSiO2NPs)
Aerosil A-380 was treated with 5% aqueous solution of 3-aminopropyltriethoxysilane in ultrasonic bath for 1 h. Then, aerosil with immobilized aminopropyl silyl groups was washed to remove excess reagent, and suspended using an ultrasonic bath. After that, the NPs were washed several times with acetone, and suspended in a solution of succinic anhydride in acetone for 2 h, followed by washing with water, and sonication. BET surface area was 230 m2 × g−1. The synthesis method is shown in and .
Sulfonated SiO2NPs (SSiO2NPs)
The method of SiO2NP modification by attaching sulfonate groups included the following stages: modification of SiO2NPs by 2-phenylethyltrichlorosilane and sulfonation of the modified SiO2NPs. Modification of SiO2NPs was carried out as follows. Two grams of SiO2NPs were suspended in 10 ml of 10% 2-phenylethyltrichlorosilane in toluene and 0.5 ml of triethylamine, a well-known catalyst (Foldvari et al., Citation2008) that speeds up the rate of chlorosilane chemisorption and enhances grafting density. The mixture was placed in a flask equipped with a reflux condenser, and chemisorption was carried out at 80 °C for 3 h. Excess solution was decanted, and the product was washed five times with dry chloroform. Chlorosilyl groups underwent hydrolysis in hot water, and the product was washed with water until no acid reaction was observed. Then, sulfonation of the modified SiO2NPs was performed as described by Foldvari et al. (Citation2008) and Cai et al. (Citation2005). The sample was placed in a flask with reflux condenser containing 20 ml dry chloroform, after which 5 ml chlorosulfonic acid was added. The mixture was heated for 7 h, cooled, filtered off, and then washed successively with chloroform, acetone, 1 M sulfuric acid, and distilled water. Surface reactions with the sequential processing of silica with 2-phenylethyltrichlorosilane, water, chlorosulfonic acid, and water are illustrated in . The content of sulfonate groups determined by acid–base titration was 0.47 mmol/g. The specific surface area was 310 m2 × g−1.
Physical properties of NPs
The size distribution of silicon-containing NPs was measured by dynamic light scattering technology with a non-invasive backscatter (Zetasizer Nano, Malvern Instruments, Malvern, UK). Infrared (IR) spectra were obtained on a Shimadzu FTIR-8400S (Shimadzu Corp., Tokyo, Japan), using the following parameters: Happ-Genzel apodization, with 45 scans at a resolution of 4.0 cm−1. Spectra are shown in the wavenumber range from 4000 to 400 cm−1; the samples were prepared with dry KBr.
Adenosine release kinetics
The samples were washed to remove excess active substance, and dried at 50–60 °C. Then, conical tubes with samples were filled with Krebs–Henseleit buffer, similar to blood plasma in terms of electrolyte composition, followed by stirring at 200 rpm (LOIPLS 110, LOIP Ltd., St. Petersburg, Russia). After predetermined time intervals, the content was centrifuged and the adenosine concentration was determined by spectrophotometry (Shimadzu UV-2450, Shimadzu Corp., Tokyo, Japan). Samples were poured into semi-demountable quartz cuvettes, and the spectra were recorded at λ = 260 nm. Optical path length was adjusted so that the maximum absorption optical density was in the range of 0.1–1.0 (ν = 38.6 × 103 cm−1, which corresponds to λ = 260 nm).
Acute toxicity of NPs
The procedures were performed in accordance with the Guide for the Care and Use of Laboratory Animals and were approved by the ethics committee at North-West Federal Medical Research Centre, St. Petersburg, Russian Federation. The animals were maintained on a 12 h light/dark cycle and were provided food and water ad libitum. Male Wistar (weight, 200–250 g) rats were anesthetized with sodium pentobarbital (60 mg/kg). In spontaneously breathing animals, the right carotid artery and femoral vein were cannulated for blood pressure monitoring and anesthesia maintenance, respectively. Rats were randomly divided into five groups: SiO2NPs (n = 6), SSiO2NPs (n = 6), OrSiO2NPs3 (n = 6), OrSiO2NPs150 (n = 6), and SiO2 + OrSiO2NPs (n = 6). Suspensions of NPs in 0.9% NaCl solution were prepared and administered intravenously at a concentration of 2 mg/ml (SiO2NPs, SSiO2NPs, and SiO2 + OrSiO2NPs) or 16 mg/ml (OrSiO2NPs). NP suspensions were administered intravenously for 10 min. Control animals received intravenous infusion of a 0.9% NaCl solution. Heart rate and mean arterial pressure were measured immediately before infusion, at the end of infusion, and 5 min after infusion.
Hemodynamic effects of NP-bound and free adenosine
Adenosine adsorption on the surface of SiO2NPs was achieved by mixing the suspension of SiO2 + OrSiO2NP in 0.9% sodium chloride and adenosine solution in the same vehicle. These suspensions were prepared extempore, and samples were left at room temperature for 2 h. SiO2 + OrSiO2NP-bound adenosine was administered intravenously for 5 min at a dose of 300 µg/kg/min. Before infusion of adenosine-loaded NPs, the animals received a 5-min intravenous infusion of free NPs and free adenosine at the same dose. After infusion of adenosine-loaded NPs, the animals received a second 5-min intravenous infusion of free adenosine at the same dose. Mean blood pressure was registered before drug infusion, at the 1st and 5th min of infusion, and at the 5th min of recovery.
Bioelimination of organic nanoparticles
In vivo bioelimination of OrSiO2NPs was studied in male Wistar rats weighing 200–250 g. OrSiO2NPs of two different diameters (3 and 150 nm) were infused intravenously at a dose of 16 mg/ml and volume of 1 ml, followed by sampling of the heart, liver, spleen, brain, kidney, and lung at 1 h, 3 h, 24 h, and 2, 3, and 7 d post-infusion. The controls were animals receiving an intravenous infusion of 1 ml of vehicle. Organ samples were dried at 90 °C over 24 h to obtain a constant weight. A quantitative analysis of silicon content within the samples was performed using atomic absorption spectroscopy.
Results
Physical properties of silicon-containing NPs
The size distribution of NPs is shown in . The average primary particle size of OrSiO2NPs3 was 3 nm. A significant increase in particle size was observed after condensation of the primary NPs, up to 150 nm. The average sizes of SiO2 + OrSiO2NPs and SSiO2NPs were 75 nm and 196 nm, respectively ().
Figure 4. Distribution of nano-objects by size. (a) Primary OrSiO2NPs (OrSiO2NPs3), (b) OrSiO2NPs obtained after condensation (OrSiO2NPs150); (c) SiO2 + OrSiO2NPs; (d) SSiO2NPs.
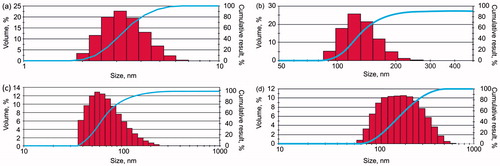
Table 1. The average size of nano-objects.
The NPs have been characterized by IR spectra (). In the spectrum of SiO2NPs, absorption bands were 480, 810, 990, 1110, 1645, 1875, 2000, and 3318 cm−1. Absorption bands at 480 and 810 cm−1 can be attributed to the stretching vibrations of Si–O–Si in the silicate systems. The 990 cm−1 band corresponds to the stretching vibrations of Si–O groups in Si–OH. The 1110 cm−1 band can be attributed to the asymmetric fluctuations of Si–O–Si. The absorption band at 1645 cm−1 is the bending vibration of OH in physically adsorbed water; the bands at 1875 and 2000 cm−1 correspond to overtones and skeletal vibrations of the silica matrix, respectively. The band at 3318 cm−1 is attributed to H-bonded water molecules and physically adsorbed water molecules on the silica surface.
Figure 5. IR spectra of silicon-containing NPs. (a) IR spectra of (1) SiO2NPs, (2) aminated SiO2NPs, and (3) SiO2 + OrSiO2NPs; (b) IR spectra of (1) SiO2NPs, (2) OrSiO2NPs, and (3) SSiO2NPs.
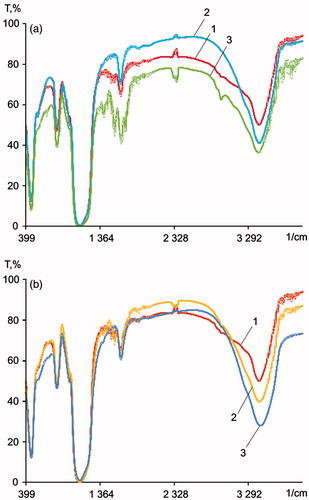
In the spectrum of aminated SiO2NPs, there are five absorption bands of aminated silica at 1490, 1570, 1659, 2930, and 3200 cm−1. The band at 1490 cm−1 corresponds to a deformation of medium intensity of the symmetric vibrations of the C–H bond. The absorption bands at 1570 and 1659 cm−1 correspond to the vibration of the N–H bond of primary amines. The absorption band at 2930 cm−1 is the stretching vibrations of the C–H bond. It should be noted that the broad band at 3200 cm−1 substantially decreased in intensity, due to exposure to surface organic radicals, which reduce the hydrophilicity of the surface. There was no band at 990 cm−1, an absorption characteristic of silanol groups. This is due to the occurrence of surface reactions between the silanol groups and 3-aminopropyltriethoxysilane.
Additional bands at 1540 and 1700 cm−1 were identified in the spectrum of SiO2NPs, which are correlated with the characteristic IR absorption bands of amide I and amide II, respectively. Also, there is a clear band at 3320 cm−1, which is characteristic of primary amino groups. The appearance of an intense band at 1360 cm−1 is due to the stretching vibrations of C–N in amides (amide band III).
Kinetics of adenosine desorption from the surface of the silica
The fastest release rate was observed for adsorption immobilization (), and the slowest was observed for ion binding. We further investigated the kinetics of adenosine desorption from the surface of SSiO2NPs, which may have a different kind of physicochemical interaction with adenosine. Measurements of the concentration of adenosine in the solution are shown in . We observed that the kinetics of adenosine desorption from the surface of SSiO2NPs coincided with the order of the kinetics of desorption of adenosine from the conventional silica with ionic binding of adenosine. Probably, the primary drug–carrier interaction of SSiO2NPs is ionic bonding with adenosine.
Figure 6. The kinetics of adenosine desorption from the surface of the silica. (a) The relation between adenosine release into the suspension of SiO2NPs and time for different immobilization methods: (1) adsorption (unmodified SiO2NPs); (2) covalent bonding (glutaraldehyde-modified SiO2NPs); (3) coordination-ion bonding (succinic anhydride-treated SiO2NPs); (b) the relation between adenosine concentration in the suspension of SSiO2NPs versus time.
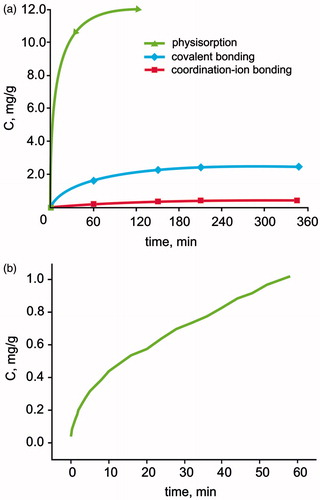
Bioelimination of OrSiO2NPs
The data on the dynamics of the silicon content in various organs following infusion of a suspension of OrSiO2NPs3 are shown in . Increased silicon levels were found in the kidney at 10 and 60 min post-infusion. The silicon content in other organs was not different from that of controls. A similar pattern was noted after infusion of OrSiO2NPs150, but the peak value of silicon concentration in the kidney was registered 24 h after administration ().
Acute toxicity of NPs
The effect of intravenous administration of silicon-containing NPs on hemodynamic parameters in rats is summarized in . There were no intra- or inter-group differences in the mean blood pressure and heart rate.
Table 2. The effect of intravenous administration of Si-containing nanoparticles on hemodynamic parameters in rats.
Hemodynamic response to free and SiO2 + OrSiO2NP-bound adenosine
Infusion of adenosine at a dose of 300 µg/kg × min caused reduction of mean arterial pressure (MAP) by 36% and heart rate by 14% at the 1st minute of infusion. When adenosine was immobilized on the surface of SiO2 + OrSiO2NPs, a much smaller decrease in MAP was registered: MAP was decreased by 9% and heart rate by 5% at the 1st min of infusion ( and ). It follows that adenosine adsorption on SiO2 + OrSiO2NPs exhibited a slower release of adenosine, resulting in the attenuation of adenosine-associated hypotension and bradycardia.
Figure 8. Hemodynamic response to free and SiO2 + OrSiO2NP-bound adenosine. Adsorption of adenosine on SiO2 + OrSiO2NPs resulted in the slow release of adenosine and reduction of side effects in the form of a sharp drop in arterial pressure. (a) Infusion of free adenosine and (b) infusion of SiO2 + OrSiO2NPs loaded with adenosine.
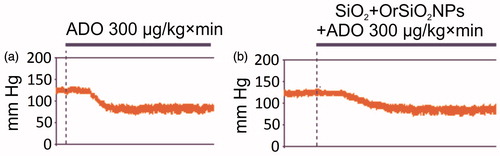
Table 3. The effect of intravenous administration of adenosine-loaded SiO2+OrSiO2NPs on hemodynamic parameters in rats.
Discussion
Recent work has shown that immobilization of adenosine on the surface of SiO2NPs resulted in the enhancement of adenosine-mediated infarct size limitation (Galagudza et al., Citation2012). In addition, immobilization of adenosine on the surface of SiO2NPs attenuated the hypotensive effect of adenosine. In a similar model, Takahama et al. (Citation2009) showed that the administration of liposome-embedded adenosine resulted in a greater infarct-limiting effect when compared with the effect of free adenosine. Moreover, liposomal adenosine caused smaller decrease in blood pressure when compared with free adenosine.
In this study, we hypothesized that functionalization of SiO2NPs would result in the enhancement of sorption properties, and would provide a gradual release of the drug. For this purpose, three different strategies of adenosine immobilization on SiO2NPs were used: physical adsorption, ionic bonding, and covalent bonding. In order to provide ionic and covalent bonding, aminated SiO2NPs were modified with succinic anhydride and glutaraldehyde. Adenosine was chosen as a model drug because of its well-recognized cardioprotective properties (Headrick et al., Citation2009; Jacobson, Citation2009). The most rapid release of adenosine was observed after adsorption immobilization (), and the slowest was observed after ion bonding. This can be explained by the instability of azomethine groups, ensuring the biding of adenosine to glutaraldehyde. The data obtained showed that the drug release rate can be adjusted through the selection of drug–carrier interaction type. It should be noted, however, that the strength of adenosine immobilization was directly proportional to the quantity of the immobilized agent. The quantity of immobilized adenosine observed was 12.6, 3.26, and 1.43 mg/g after physisorption, ion and covalent bonding, respectively. More studies are needed to develop the optimal method of NP surface modification resulting in the greatest drug loading efficiency associated with optimal release kinetics.
The major concerns associated with the use of nanocarriers are their toxicity and biodegradability. When administered intravenously, SiO2NPs were shown to accumulate in reticuloendothelial system, particularly in the liver and spleen, resulting in the formation of foreign body-type granulomas (Ivanov et al., Citation2012). Therefore, there is great interest surrounding organomodified silica NPs, which combine the properties of organic and inorganic substances. OrSiO2NPs have recently emerged as an attractive candidate for nanomedical applications (Selvestrel et al., Citation2013). Methods of OrSiO2NPs synthesis are well characterized (Roy et al., Citation2012). Roy et al. (Citation2012) describe an OrSiO2NP synthesis scheme based on the alkaline condensation polymerization of organosilanes. During the first step, microemulsion of dioctylsulfosuccinate/1-butanol/water was prepared, followed by the addition of active substances or N,N-DMF. After that, organosilanes, such as 3-ethoxyvinylsilane and 3-aminopropyl-3-ethoxysilane, were added. At the final stage of purification from an excess of surfactants, the OrSiO2NPs solution is subjected to dialysis.
OrSiO2NPs have a number of physicochemical properties that are helpful in designing targeted drug delivery systems. However, to date, there is only a single study describing the biodegradation of OrSiO2NPs, in which fluorescent and radiolabeled OrSiO2NPs with a diameter of 20–25 nm were administered to athymic nude mice (Kumar et al., Citation2010). At 24–120 h post-infusion, a preferential accumulation of OrSiO2NPs in the liver, spleen, and stomach was found, with a significantly lower accumulation in the kidney, heart, and lung. Within 15 d, there was almost a complete elimination of OrSiO2NPs through hepatobiliary excretion, with an absence of histological manifestations of organ toxicity. The results of our study suggest that OrSiO2NPs with a diameter of 3 nm and 150 nm are excreted by the kidneys; as we observed, the level of silica in the kidney in the first hours after administration of the OrSiO2NPs significantly exceeded the background value. It cannot be excluded, however, that OrSiO2NPs with a diameter of 3 nm can traverse the glomerular filter.
In recent years, the medical applications of OrSiO2NPs have been actively discussed in the literature. Several studies have explored the methods of cancer photodynamic therapy using OrSiO2NP-embedded photosensitizers (Selvestrel et al., Citation2013; Singh et al., Citation2014). Another important application of OrSiO2NPs is the targeted delivery of genetic constructs into the cell (Bharali et al., Citation2005). On one hand, transfection of target cells can be used for the modeling of central nervous system pathology. In particular, OrSiO2NPs were used as a vector for the delivery of plasmids encoding polyglutamines into the brain of mice to simulate Huntington's disease (Klejbor et al., Citation2007). On the other hand, the high tropism of OrSiO2NPs to neurons and the absence of significant changes in cell function following penetration by OrSiO2NPs (Barandeh et al., Citation2012) hold promise for the use of OrSiO2NPs in neurodegenerative disease therapies (Gunawardena, Citation2012). OrSiO2NPs can be applied not only for the delivery of drugs and genes in affected tissue but also for the visualization of damaged cells (Kumar et al., Citation2008).
To improve OrSiO2NP stability in circulation, we synthesized a new hybrid nanocarrier with a silica core and OrSiO2NPs as a shell, with an average size of 75 nm. The idea of creating hybrid NPs by mixing organic polymers and inorganic NPs opens new pathways for engineering new nanocarriers. The concept was recently applied to synthesize a hybrid NP with a Fe3O4 core and an organic shell (Zhou et al., Citation2009).
Sulfonation represents another method of SiO2NPs surface modification that has been used to produce chitosan–silica complex membranes (Liu et al., Citation2005). In the present study, sulfonation of SiO2NPs was used for the modification of the surface of SiO2NPs to study alternative methods of ionic immobilization of adenosine. The size of SSiO2NPs was 196 nm. The presence of sulfonic acid groups has changed the kinetics of adenosine desorption from the surface of SiO2NPs by providing ionic bonds with adenosine. This coincided with the order of the kinetics of desorption of ionically bound adenosine from the SiO2NPs modified by succinic anhydride.
Our previous study showed that SiO2NPs are relatively biocompatible nanomaterials, at least when considering acute toxicity (Ivanov et al., Citation2012). Here, we studied the acute hemodynamic effects of NP formulations in the rat model by intravenous infusion of suspensions of modified SiO2NPs with sulfonic acid groups: OrSiO2NPs3, OrSiO2NPs150, SiO2 + OrSiO2NP, and unmodified SiO2NPs. The parameters of systemic hemodynamics remained unaltered in all groups. Our study confirms that SiO2NPs and OrSiO2NPs are biocompatible nanomaterials that can be used as model nanocarriers in drug delivery studies. In a separate experimental series, we analyzed the hemodynamic response to SiO2 + OrSiO2NP-bound versus free adenosine. Adsorption of adenosine on SiO2 + OrSiO2NPs resulted in the delayed release of adenosine, and a reduction in hemodynamic side effects. This result supports the use of SiO2 + OrSiO2NPs as a drug carrier. More research is needed to study the biodegradation and biodistribution of this new hybrid NP. Previously, we have shown that non-modified SiO2NPs can accumulate within the anatomical area at risk after regional myocardial ischemia–reperfusion (Galagudza et al., Citation2012). In combination with the results of the present study, these data provide a solid basis for further investigation of silicon-containing NPs as drug carriers.
Conclusions
This study describes an original method of synthesis of differently sized OrSiO2NPs, and shows an absence of acute toxicity of silicon-containing NPs. OrSiO2NPs with a diameter of 3 nm undergo rapid elimination from the body by renal excretion during the first hour after infusion. Larger OrSiO2NPs (150 nm) are also likely excreted by the kidney, but the process is slower, probably being associated with the cleavage of circulating OrSiO2NPs into smaller fragments. OrSiO2NPs150 do not accumulate in the reticuloendothelial system. We also describe an original method of synthesis of new hybrid SiO2 + OrSiO2NPs. Adsorption of adenosine on SiO2 + OrSiO2NPs results in the delayed release of adenosine and reduction of hemodynamic side effects. We show that the rate of adenosine desorption critically depends on the type of immobilization; therefore, adenosine release kinetics can be adjusted by the technique of SiO2NP surface modification.
Declaration of interest
The authors report that they have no conflicts of interest. This study was supported by the Russian Science Foundation (Project 14-15-00473).
References
- Abdelkader H, Alani AW, Alany RG. (2014). Recent advances in non-ionic surfactantvesicles (niosomes): self-assembly, fabrication, characterization, drug delivery applications and limitations. Drug Deliv 21:87–100
- Bae YH. (2009). Drug targeting and tumor heterogeneity. J Control Release 133:2–3
- Barandeh F, Nguyen PL, Kumar R, et al. (2012). Organically modified silica nanoparticles are biocompatible and can be targeted to neurons in vivo. PLoS One 7:e29424
- Bharali DJ, Klejbor I, Stachowiak EK, et al. (2005). Organically modified silica nanoparticles: a nonviral vector for in vivo gene delivery and expression in the brain. Proc Natl Acad Sci USA 102:11539–44
- Cai D, Mataraza JM, Qin ZH, et al. (2005). Highly efficient molecular delivery into mammalian cells using carbon nanotube spearing. Nat Methods 2:449–54
- Chittasupho C, Jaturanpinyo M, Mangmool S. (2013). Pectin nanoparticle enhances cytotoxicity of methotrexate against HepG2 cells. Drug Deliv 20:1–9
- De Jong WH, Borm PJ. (2008). Drug delivery and nanoparticles: applications and hazards. Int J Nanomed 3:133–49
- Foldvari M, Bagonluri M. (2008). Carbon nanotubes as functional excipients for nanomedicines: II. Drug delivery and biocompatibility issues. Nanomedicine 4:183–200
- Galagudza MM, Korolev DV, Sonin DL, et al. (2010a). Passive targeting of ischemic myocardium with the use of silica nanoparticles. Nanotechnol Russia 5:844–50
- Galagudza M, Korolev D, Sonin D, et al. (2010b). Targeted drug delivery to ischemic heart with use of nanoparticulate carriers: concepts, pitfalls and perspectives. J Manuf Technol Manage 21:930–49
- Galagudza M, Korolev D, Postnov V, et al. (2012). Passive targeting of ischemic-reperfused myocardium with adenosine-loaded silica nanoparticles. Int J Nanomed 7:1671–8
- Gunawardena S. (2012). Developing nanotherapies for neurodegenerative diseases: ORMOSIL and its potential in axonal transport. Ther Deliv 3:1189–98
- Headrick JP, Lasley RD. (2009). Adenosine receptors and reperfusion injury of the heart. Handb Exp Pharmacol 193:189–214
- Ivanov S, Zhuravsky S, Yukina G, et al. (2012). In vivo toxicity of intravenously administered silica and silicon nanoparticles. Materials 5:1873–89
- Jacobson KA. (2009). Introduction to adenosine receptors as therapeutic targets. Handb Exp Pharmacol 193:1–24
- Klejbor I, Stachowiak EK, Bharali DJ, et al. (2007). ORMOSIL nanoparticles as a non-viral gene delivery vector for modeling polyglutamine induced brain pathology. J Neurosci Methods 165:230–43
- Kumar R, Roy I, Ohulchanskyy TY, et al. (2008). Covalently dye-linked, surface-controlled, and bioconjugated organically modified silica nanoparticles as targeted probes for optical imaging. ACS Nano 2:449–56
- Kumar R, Roy I, Ohulchanskkyy TY, et al. (2010). In vivo biodistribution and clearance studies using multimodal organically modified silica nanoparticles. ACS Nano 4:699–708
- Lyseng-Williamson KA, Duggan ST, Keating GM. (2013). Pegylated liposomal doxorubicin: a guide to its use in various malignancies. BioDrugs 27:533–40
- Li M, Wang G, Li H, et al. (2015). How to deliver therapeutics or imaging agents to the infracted heart? Austin Therapeutics 2:1013
- Liu YL, Hsu CY, Su YH, et al. (2005). Chitosan-silica complex membranes from sulfonic acid functionalized silica nanoparticles for pervaporation dehydration of ethanol-water solutions. Biomacromolecules 6:368–73
- Levchenko TS, Hartner WC, Torchilin VP. (2012). Liposomes in diagnosis and treatment of cardiovascular disorders. Method Debakey Cardiovasc J 8:36–41
- Malvindi MA, Brunetti V, Vecchio G, et al. (2012). SiO2 nanoparticles biocompatibility and their potential for gene delivery and silencing. Nanoscale 4:486–95
- Nishimori H, Kondoh M, Isoda K, et al. (2009). Silica nanoparticles as hepatotoxicants. Eur J Pharm Biopharm 72:496–501
- Osek J. (2003). Detection of the enteroaggregative Escherichia coli heat stable enterotoxin 1 (EAST1) gene and its relationship with fimbrial and enterotoxin markers in E. coli isolates from pigs with diarrhea. Vet Microbiol 91:65–72
- Puntawee S, Theerasilp M, Reabroi S, et al. (2015). Solubility enhancement and in vitro evaluation of PEG-b-PLA micelles as nanocarrier of semi-synthetic andrographolide analogue for cholangiocarcinoma chemotherapy. Pharm Dev Technol. [Epub ahead of print]. doi: 10.3109/10837450.2015.1016619
- Roy I. (2012). Synthesis, surface modification, characterization, and biomedical in vitro applications of organically modified silica (ORMOSIL) nanoparticles. Methods Mol Biol 906:365–79
- Rasouli S, Davaran S, Rasouli F, et al. (2014). Synthesis, characterization and pH-controllable methotrexate release from biocompatible polymer/silica nanocomposite for anticancer drug delivery. Drug Deliv 21:155–63
- Selvestrel F, Moret F, Segat D, et al. (2013). Targeted delivery of photosensitizers: efficacy and selectivity issues revealed by multifunctional ORMOSIL nanovectors in cellular systems. Nanoscale 5:6106–16
- Singh SP, Sharma M, Patel H, et al. (2014). Extra cellular pH influences uptake and photodynamic action of pyropheophorbide-a entrapped in folate receptor targeted organically modified silica nanoparticle. Photodiagn Photodyn Ther 11:156–64
- Takahama H, Minamino T, Asanuma H, et al. (2009). Prolonged targeting of ischemic/reperfused myocardium by liposomal adenosine augments cardioprotection in rats. J Am Coll Cardiol 53:709–17
- Xie G, Sun J, Zhong G, et al. (2010). Biodistribution and toxicity of intravenously administered silica nanoparticles in mice. Arch Toxicol 84:183–90
- Zhou L, Yuan J, Yuan W, et al. (2009). Synthesis and characterization of multi-functional hybrid magnetite nanoparticles with biodegradability, superparamagnetism, and fluorescence. Mater Lett 63:1567–70